- 1School of Transborder Studies, Arizona State University, Tempe, AZ, United States
- 2School of Sustainable Engineering and the Built Environment, Arizona State University, Tempe, AZ, United States
- 3Western Geographic Science Center, U.S. Geological Survey, Tucson, AZ, United States
- 4Department of Environmental Science, University of Arizona, Tucson, AZ, United States
- 5Instituto Municipal de Investigación y Planeación (IMIP), Nogales, Mexico
Creating sustainable, resilient, and livable cities calls for integrative approaches and collaborative practices across temporal and spatial scales. However, practicability is challenged by institutional, social, and technical complexities and the need to build collective understanding of integrated approaches. Rapid urbanization along the United States-Mexico border, fueled by industrialization, trade, and migration, has resulted in cities confronted with recurrent flooding risk, extended drought, water pollution, habitat destruction and systemic vulnerabilities. The international border, which separates natural and built ecosystems, is both a challenge and an opportunity, making a unique social and institutional setting ideal for testing the integration of urban planning and water management. Our research focuses on fusing multi-functional and multi-scalar green infrastructure to restore ecosystem services through a strategic binational planning process. This paper describes this planning process, including the development and application of both a land suitability analysis and a hydrological model to optimally site green infrastructure in the Nogales, Arizona, United States—Nogales, Sonora, Mexico, cross border region. We draw lessons from this process and stakeholder feedback focused on the potential for urban green infrastructure, to allow for adaptation and even transformation in the face of current and future challenges such as limited resources, underdeveloped governance, bordering, and climate change. In sum, a cross border network of green infrastructure can provide a backbone to connect this transboundary watershed while providing both hydrological and social benefits.
Introduction
A cross-border urbanization formed by Nogales, Sonora, and Nogales, Arizona, “Ambos Nogales,” embodies the complexities and challenges of urban planning and water management on the United States-Mexico border. The two cities were settled simultaneously at the end of the ninteenth century in the eastern section of the Santa Cruz River Basin and along the narrow valley formed by the Nogales Wash (Figure 1). The steep topography includes wooded hillsides, rock outcroppings, and seasonal arroyos that are typical of the Sonoran Desert landscape. Ambos Nogales contains an urban corridor that originates at the border gateway and expands north and south for ~12 km. While the two cities are separated by the border, they are highly interconnected by geography and ecohydrology. Monsoonal storms, more than any other natural event, make this paradox evident.
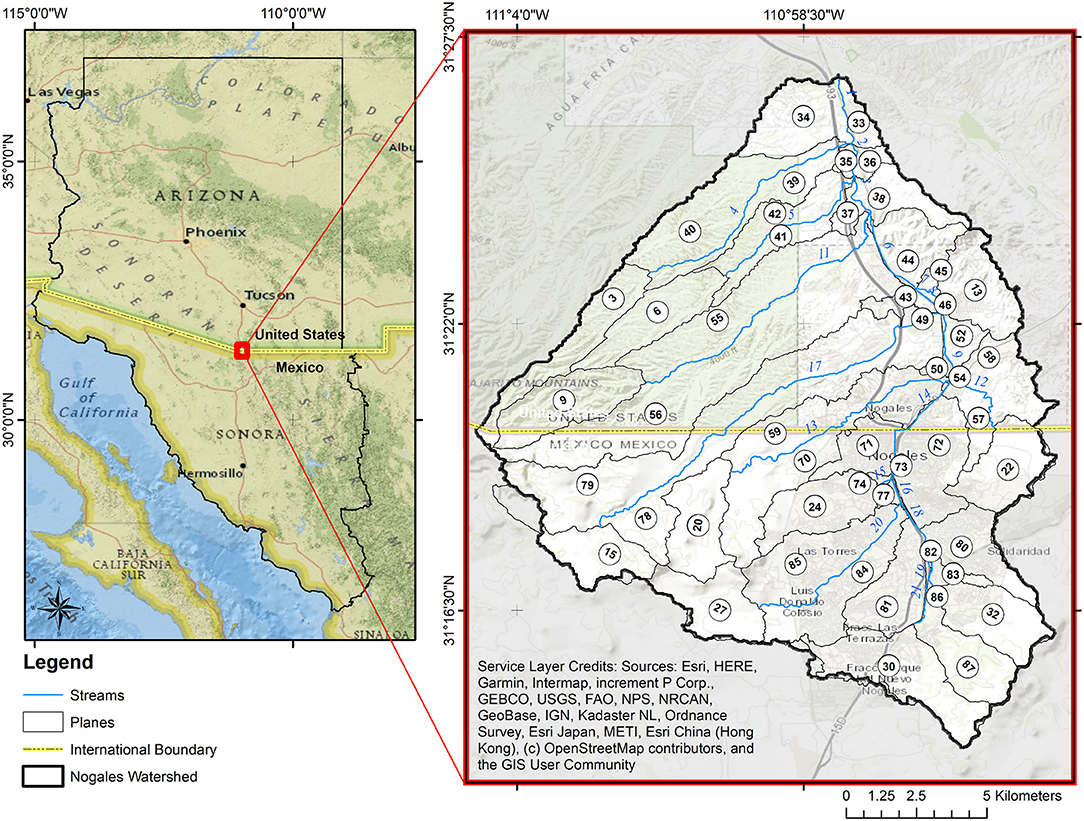
Figure 1. Nogales, Arizona, and Nogales, Sonora (Ambos Nogales), are located on the United States-Mexico border. Plane and stream numbers are labeled based on KINEROS2 outputs.
According to official reports collated by the National Oceanic and Atmospheric Administration (NOAA), documented flooding in Ambos Nogales dates back to 1887; more recently, almost every year from 1980 to 2020, the Ambos Nogales area has been impacted by one or more severe storms causing major floods and destruction (NOAA., 2021). Because of the higher altitude of Nogales, Sonora, Mexico, stormwater runoff, sediments, and pollutants travel north to lower elevations in Nogales, Arizona, United States, causing damage on their way to the lowest sections of the watershed along the major transportation corridors in both cities and the commercial business districts near the border (Norman et al., 2010a). Flood-related deaths, displacement, and destruction affect both sides of the border, yet are concentrated on the Mexican side (NOAA., 2021). In addition to the short-term costs of flooding (loss of life and public and private property damage), Ambos Nogales also has to deal with long-term impacts of increased water pollution (Norman et al., 2008), health risk (Norman et al., 2012a), and disruption to schools and social life (García-Perez and Lara-Valencia, 2021).
Urban green infrastructure (UGI) is increasingly promoted in both research and policy as a way for cities to achieve multiple benefits and become more sustainable and resilient (Ahern, 2011; Palmer et al., 2015). UGI is defined by the U.S. Water Infrastructure Act (U.S. Congress, 2019) as “the range of measures that use plant or soil systems, permeable pavement or other permeable surfaces or substrates, stormwater harvest and reuse, or landscaping to store, infiltrate, or evapotranspirate stormwater and reduce flows to sewer systems or to surface waters.” In particular, green infrastructure is used to mitigate urban hydrological modification thereby decreasing peak runoff rates, increasing groundwater recharge, mitigating the urban heat island effect, and providing other ecosystem services (Tzoulas et al., 2007; Pataki et al., 2011; Spatari et al., 2011; Lewellyn et al., 2016; Yang and Wang, 2017). In addition to providing hydrological and ecosystem services, green infrastructure also has the potential to provide social and cultural benefits (O'Brien et al., 2017). Further, the choice of where to site UGI has implications for equity and environmental justice (Hoover et al., 2021). The environmental planning literature increasingly recognizes the role that UGI can play in supporting the well-being of urban communities and the need to apply UGI for long term strategic planning advancing sustainability and equity in cities (Hansen et al., 2019; Meerow, 2020; Campbell-Arvai and Lindquist, 2021). However, how to integrate social and ecohydrological factors in a sustainable planning strategy, particularly in border regions, is a challenge for urban planning (Hansen et al., 2019). Further, while the scientific literature strongly supports integrating urban planning and the management of specific resources or hazards (e.g., water, heat), there are few applications (for exceptions see Liu and Jensen, 2018) and little specific guidance (Norton et al., 2015; Hansen et al., 2019; Van Oijstaeijen et al., 2020).
Emphasizing the role of UGI as an environmental planning tool, the European Environmental Agency (EEA, 2019) defines UGI as “a strategically planned network of natural and semi-natural areas with other environmental features designed and managed to deliver a wide range of ecosystem services.” UGI improves water quality and mitigates flooding by mimicking natural hydrology through storing water in the landscape, reducing runoff, slowing the stormwater conveyance, and facilitating infiltration (Norman, 2020). The cities of Nogales, Sonora, and Nogales, Arizona, have embraced the idea that their long-term sustainability and quality of life depend on valuing and protecting natural systems, so creating green infrastructure is central to their planning efforts (Giner et al., 2019; Freimund, 2020).
A core value of UGI planning is its multifunctionality, which relates to its potential of providing a stream of multiple benefits emanating simultaneously from a single intervention site (Hansen and Pauleit, 2014). To achieve UGI multifunctionality, a wide range of social and hydrological variables must be considered to identify feasible and priority sites for UGI implementation. However, to fully enable UGI cross-cutting capacity to provide these benefits, its multifunctionality should be explicitly considered during the planning process and the specific solutions selected deployed as part of a multi-scalar urban strategy, including interventions at the neighborhood, city, and watershed levels (Hansen and Pauleit, 2014; Surma, 2020). The project posits that a cross-border perspective is required to characterize the complex socio-ecological relationships and dynamics occurring within the Ambos Nogales watershed (Brown and Mumme, 2000). Therefore, an essential activity of the project is identifying the most appropriate sites for the placement of the components of a UGI network encompassing the Mexican and United States sections of the watershed. We conceptualize the bi-national UGI network as the backbone of a cross-border planning strategy to reconnect Nogales, Sonora, and Nogales, Arizona, with each other and with their natural environment using an approach based on principles of sustainability, resilience, and cooperation.
In this manuscript, we present an approach to integrate land suitability analysis (LSA) and hydrological modeling to identify priority sites for UGI. We apply this approach to Nogales, Sonora, and Nogales, Arizona, and assess the impact of implementing UGI on the identified sites. LSA involves evaluating spatial information by applying a series of criteria to determine which sites are more or less suitable for the placement and construction of urban infrastructure. The main input of this process is geospatial datasets that make explicit the location and spatial variability of the capabilities and limitations of natural and built systems that influence the urban hydrological cycle and the social functions taking place in these systems. The ultimate product of the analysis is a map of the relative aptitude of the different areas of the city to develop UGI interventions (Kuller et al., 2017).
Hydrological modeling allows for a representation of the water budget, with varied inputs and outputs, that allow for understanding, predicting, and managing water resources, related to discharge and water quality. Geospatial watershed models address the spatial variation that might occur in a landscape including variations in geology, soils, vegetation, land use/land cover, and precipitation patterns. Best management practices, such as stormwater control, can be input to models to determine the optimal placement for making desired improvements (Avellaneda et al., 2017; Korgaonkar et al., 2018), including for flood mitigation (Zellner et al., 2016), water quality (Korgaonkar et al., 2021) and equity (Garcia-Cuerva et al., 2018). Watershed models of Ambos Nogales have been used to predict impacts of best management practices (Norman et al., 2008), identify hotspots of erosion (Norman et al., 2008), and situate large rock detention structures (gabions) for optimal flood detention (Norman et al., 2010a,b).
This paper addresses the interaction between urban planning and hydrological modeling for stormwater management in the context of Ambos Nogales. This research reports from an on-going bi-national effort to mitigate flooding, improve water quality, and increase green space access with UGI underpinning a cross-border planning strategy.
Study Area and Data
The Ambos Nogales watershed is the main drainage area of the Nogales Wash and its tributaries that runs from Mexico to the United States and connects Nogales, Sonora, and Nogales, Arizona (Figure 1). Ambos Nogales is the largest binational conurbation and most important trade corridor along the Arizona-Sonora border (Lara-Valencia and Herzog, 2021). The topography in this region is characterized by steep high mountain drainages in the south that create narrow canyons draining to the urban center and alluvium at the United States-Mexico border (Brady et al., 2002). The primary soil types in Nogales, Arizona, are gravelly sandy loam and in Nogales, Sonora, are generally very thin soils of average texture, commonly rocky (Norman et al., 2004). Land cover within the watershed has been dramatically transformed by urbanization and economic growth, which in turn has altered the hydrology and hydraulics of this natural system (Norman and Wallace, 2008; Myint et al., 2010; Norman et al., 2010c, 2012b). Ambos Nogales includes socio-environmentally vulnerable neighborhoods on both sides of the border, as determined by levels of education, access to resources, migratory status, housing, and number of dependents, and regulating ecosystem services (Norman et al., 2012b).
Ambos Nogales has a hot desert or arid (BWh) climate (according to the Köppen classification), which is characterized by extreme hot summers and mild short winters. The average yearly precipitation is 409 mm, while the mean high and low temperature are 26.7 and 6.7°C, respectively (NCEI, 2021). There are two wet seasons, the summer (July–September), marked by monsoon storms, and winter (November–March), characterized by large-scale frontal storms (Sheppard et al., 2002). Most of the year, the Nogales Wash has a base flow of 0.06–0.08 m3/s (2–3 ft3/s), much of which is fed by untreated sewage and potable water leaks originating in neighborhoods, industrial parks, and commercial areas in Nogales, Sonora (Huth and Tinney, 2008). During the summer and winter wet seasons, the watershed is subject to intense rainfall, producing fast-moving and concentrated runoff threatening human life, urban infrastructure, and ecosystems on both sides of the border.
Currently, Nogales, Sonora, has ~260,000 residents, and its economy is dominated by export-oriented manufacturing (INEGI, 2021). On the other hand, Nogales, Arizona, has a population of about 21,000, with jobs heavily dependent on border enforcement, retail, and transportation (US Census Bureau, 2019a). Ambos Nogales is a transportation hub for Mexican winter vegetables exported into the United States and for export-oriented industries (manufacturing/maquiladora and mining) operating in northwest Mexico (Bureau of Transportation Statistics., 2021). According to the 2019 American Community Survey (US Census Bureau, 2019a), about 95% of Nogales, Arizona, residents have Mexican ancestry, and almost 42% are foreign-born. Many families have members on both sides of the border and cross the border regularly, both ways, for work, school, health care and shopping. Despite the obvious cross-border socio-ecological connections, water management in the watershed remains fragmented and often runs in opposite directions on each side of the border (Schwartz, 2021).
The summary of data used in this study is shown in Table 1. Table 1 describes the data type (vector, raster, or time series data) and notes the source.
Methodology
In the sections below, we first describe the methodology for the LSA method. We then explain the methodology for hydrological modeling of both the existing land use and the case of UGI as prioritized by the LSA.
Land Suitability Analysis
Determining the location of the UGI network and its components involves major decisions at various levels starting from choosing the most appropriate land uses and functions for various UGI practices. In her extensive literature review, Malczewski (2006) noted that there are diverse approaches to LSA. In this project, we used a spatially explicit multicriteria assessment to identify the most suitable sites for the placement of UGI solutions in Ambos Nogales. Spatially explicit evaluations enable the design of a UGI network reflecting the locational constraints and advantages of specific sites within a planning area while responding to the range of needs characterizing complex urban landscapes such as the Ambos Nogales watershed.
Kuller et al. (2017) advocated a two-sided suitability assessment framework to determine the location of hydrologically sensitive interventions in cities, including UGI. This framework acknowledges that UGI's feasibility and effectiveness are impacted by site-specific characteristics such as soil type, hydrological features, land availability, population density and others, but also introduces the needs of a place into the locational assessment. This double-sided framework puts on the same plane the consideration of the factors that affect the effectiveness of UGI (what UGI needs from a location) and its capacity to produce change leading to desirable social and ecological outcomes (what a location needs from UGI) (Kuller et al., 2017).
According to this approach, the main goal of LSA is the identification of a series of locations within the Ambos Nogales watershed where the combination of geohydrological, social, and institutional factors: (1) allows for the optimal functioning of UGI from an engineering, ecological and urban planning perspective and (2) provides the highest probability of contributing directly and significantly to flood mitigation, water protection, and open space creation.
Figure 2 summarizes the analytical flow of the process we followed in implementing this approach and the criteria used to select potential sites comprising the Ambos Nogales UGI network. The data were obtained from a variety of Mexican and United States sources, including national censuses, the U.S. Geological Survey, Santa Cruz County and the Municipality of Nogales, Sonora (Table 1). All data sources were re-projected using ArcMap projection tools from their original coordinate system to NAD 83, UTM Zone 12 (EPSG:26912).
The first stage identifies the conditions required by UGI to function optimally: land use compatibility, slope, and soil type. Land used primarily for public functions (schools, parks, cemeteries, rights of way, etc.) and vacant and underdeveloped parcels are spaces with the highest UGI potential because of their compatibility and adaptability through retrofit infill or landscape design. Based on the ad-hoc Ambos Nogales Land-Based Classification System (LBCS) developed as part of this research, city blocks on both sides of the border were classified as most suitable if they were used primarily for public functions or were vacant or underdeveloped (Lara-Valencia, 2021). They were classified as least suitable if used for any other urban function. Because stormwater movement over the land is affected by slope, finding locations in the path of stormwater runoff and facilitating water detention and in situ usage is critical for UGI placement. Slopes were calculated for land on both sides of the border and grouped in five categories using a digital elevation model (2ft × 2ft cell size) derived from a 2019 LiDAR (Light Detection and Ranging) data covering the urban areas of the Ambos Nogales watershed and the USGS 10 m digital elevation model for areas not covered by LiDAR. Land with 0–3% slope was considered the most suitable, 3–6% suitable, 6–9% moderately suitable, 9–12% unsuitable, and land with slopes greater than 12% was the least suitable. Soil maps from Norman et al. (2016) were used in this analysis. Each soil type identified was categorized as moderately rapid, moderate, moderately slow, slow and very slow permeability based on hydraulic conductivity (Richardson et al., 1979). Because most UGI solutions are designed with infiltration in mind, the higher the soil infiltration rate in an area, the higher their suitability for UGI placement.
The second stage focused on identifying areas within the watershed where UGI could yield the highest output in terms of flooding risk mitigation, water contamination reduction, and green space provision. These three areas are a major cause of concern on both sides of the border and provide the two cities the chance of becoming more resilient and achieving environmental, social and economic benefits (Marquez Reyes, 2010; Freimund, 2020; Schwartz, 2021). Considering these areas corresponds to Kuller et al. (2017) recommendation to instill the consideration of ecosystem services in selecting sites for the implementation of UGI solutions.
Regarding flood mitigation, physical factors such as imperviousness, elevation, and roadways are critical. The inclusion of these features in the analysis was informed by the general notion that controlling stormwater runoff at the origination point (i.e., parking lots and high-altitude areas) or in the path of concentrated runoff (i.e., street network) increases the overall efficacy and cost-effectiveness of UGI solutions. For water quality, the selection of suitability factors (network of channels and streams, active water wells, and pollution hotspots) assumes that waterways and wellheads are features in need of protection from polluted stormwater runoff while pollution hotspots need to be contained from contributing pollutants to stormwater runoff. Note that UGI are not recommended for hotspot runoff as they may facilitate groundwater contamination but may be used to reduce the runoff reaching pollutant hotspots. On the other hand, the placement of UGI in easily accessible locations (proximity to major roads) or within high-density neighborhoods (residents per areal unit) increases open space opportunities and reduces socio-spatial disparities if such locations are in neighborhoods with poor access to public parks and other green areas (distance to nearest public park).
Each of the factors considered in stages 1 and 2 was mapped as a separate GIS layer to represent a gradient of suitability based on their unique characteristics and potential of contributing to the goal of the analysis. Each layer was assigned a value on an ordinal (1-5) or binary scale (1 or 5), with a score of five representing the highest suitability. ArcGIS 10.2 was used to generate initial vector layers that were ultimately transformed into raster layers. All raster data were created using a 25-m cell size and the Nogales Watershed Area polygon was used as an analysis mask. This resolution was selected for consistency in resolution across LSA input data. All the layers were weighted equally and added together to determine a partial UGI suitability score.
The third stage consisted of combining the raster layers representing the UGI effectiveness factors and the UGI service factors to obtain an overall suitability score for each cell grid within the watershed. Raster data facilitates weighted overlay on numerous layers and calculates a composite score for each cell on the grid. The purpose of weighting in LSA is to determine each factor's importance compared with another. Involving local stakeholders in the analysis is a proven approach for evaluating alternative and competing solutions to a multi-objective problem (Pereira and Duckstein, 1993). In addition, it is particularly useful in learning and building consensus with stakeholders and building a sense of stakeholder's ownership in the project's results and recommendations (Malczewski, 2006).
Weighting in this study was done with the help of a sample of Ambos Nogales' stakeholders identified using chain-referral sampling. The stakeholders in the sample included developers, contractors, as well as engineers, planners, and technicians working in local water departments, flood control offices, and planning agencies. The sample comprised 54 individuals evenly distributed across the border who were chosen for their involvement in urban and water planning, but also because of their professional ability to provide an actionable opinion about the feasibility of implementing UGI as a multi-functional approach to stormwater management on both sides of the border. Although this approach limits the spectrum of views included in the analysis, it was justified by the need to obtain specialized and practical insight for a study designed as part of a technical assistance project. To assess stakeholder priorities, we developed a survey which included three questions intended to elicit their level of concern regarding flood risk, water contamination, and availability of green space in their city and across the border. The questions used a five-point Likert scale ranging from “a great deal of concern” to “not concerned at all.” The responses provided data points used to calculate a weighted score to determine the relative importance assigned by the group of stakeholders to each factor of the four UGI factors considered in the LSA. Because the feasibility factors represent constraints for the emplacement of UGI solutions, it was assigned the same score as the service factor with the highest ordinal score (water protection). Finally, priority scores were transformed into weights by dividing them by the summation of all (Σ = 9).
Finally, the four composite layers were added together using a weighted linear combination procedure where each factor (Wi) was multiplied by the corresponding weight (Zi) to get a weighted suitability score based on the following formula:
where wi represents the suitability score of factor i, zi represents the relative weight of factor i, and n is the total number of factors included in the analysis.
Hydrological Modeling
In this analysis, we applied the KINematic Runoff and EROSion (KINEROS2; K2) hydrological model implemented via the Automated Geospatial Watershed Assessment (AGWA) 3.x tool GIS interface. KINEROS is an event oriented, physically based model describing the processes of interception, infiltration, surface runoff and erosion that can be applied to urban watersheds (Woolhiser et al., 1990; Wheater et al., 2007; Goodrich et al., 2012). In addition to spatial variation of inputs (land use, slope, soils and rainfall), the spatial variation of outputs (infiltration, runoff, and erosion) can be accommodated (Goodrich et al., 2012). KINEROS has been used to determine the effects of various artificial features such as small detention reservoirs, green infrastructure, and gabion rock detention structures on flood hydrographs and sediment yield (Kennedy et al., 2013; Korgaonkar et al., 2018). In AGWA 3.x the Nogales Watershed was delineated and discretized using a mosaiced 0.6 m digital elevation model (DEM modified from the Santa Cruz County LiDAR dataset). Watershed discretization breaks up the delineated watershed into planes and creates a stream feature class that drains the planes. The plane size is selected to capture the variability in the topography, soils, and land cover conditions across the watershed. Subsequently, the discretized watershed was subdivided using binational land-use-land-cover (LULC) data (Norman and Wallace, 2008) and the Food and Agriculture Organization of the United Nations (FAO/UNESCO) soil map of the World. Although the FAO soil maps have a low resolution (1:5,000,000 scale), previous studies have shown that K2 results for FAO compare well with results from higher resolution soil maps in the United States (Levick et al., 2004; Levick, 2006). A 10-year, 1-h time-depth storm event of 46.5 mm (1.83 inches) was defined based on rainfall data from the National Oceanic and Atmospheric Administration (NOAA) Atlas 14 (NOAA, 2011). A spatially uniform rainfall is less appropriate for quantitative modeling in arid regions. However, it is useful in qualitatively assessing land-use/cover changes (Norman et al., 2010a). The inputs for this model run are representative of the current land-use scenario, with no green infrastructure intervention.
KINEROS with AGWA 3.x is applied to model both the current land use and the conversion of areas identified in the LSA to UGI. To model the effects of UGI placement on stormwater management, we created a new land cover input file with the locations recommended by the LSA converted to UGI. According to Norman (2020), areas treated with rock detention structures (i.e., gabions, check dams, one-rock dams, and loosely placed stones such as trincheras or spreaders) take on characteristics normally associated with wetland-like landscapes in terms of vegetation (Norman et al., 2014; Wilson and Norman, 2018), water availability (Norman et al., 2017, 2019), and carbon sequestration potential (Callegary et al., 2021). The impacts of rock detention structures have been studied and reported on over the past 12 years as summarized by Norman (2020). To represent this alteration to the landscape in our UGI scenario analysis, we converted polygon areas being proposed as treated with UGI to the wetland land-cover class as an alternative input to K2. This land-use reclassification allows for a rapid evaluation of the different hydrologic responses to landscape changes and an assessment of maximum benefits to target mitigation activities (Norman et al., 2010a). The implementation of UGI in KINEROS with AGWA 3.x was highly simplified in order to model a large spatial area efficiently using existing model tools (Korgaonkar et al., 2018). While modeling UGI as wetlands has been previously vetted as an appropriate approach, there are important site-specific characteristics that are not captured.
To verify that model output is consistent with site specific details, we analyzed the hydrological impacts of UGI on one of the pilot sites where UGI was installed in Spring 2021 using the first rainfall-runoff model, the Rational Method (Mulvany, 1851; Koutsoyiannis et al., 1998). The Rational Method requires the contributing drainage area, rainfall intensity for a storm of a specified return period, and the runoff coefficient (which is a function of soils, slope, and land use). The contributing areas and UGI footprint and volume were field verified by the project team and the runoff coefficients were estimated using field assessment and soils data. Time of concentration was computed using the TR-55 approach (USDA., 1986). Rainfall intensity for a storm with a return period of 2-year and the computed time of concentration was drawn from NOAA Atlas 14 (NOAA, 2011). Pre- and post-UGI construction peak flow was computed using the Rational Method peak flow equation (Equation 2).
where Qp is peak flow, C is the runoff coefficient, i is the rainfall intensity, and A is the catchment area. The resulting peak flow reduction was then compared with the KINEROS peak flow reduction.
Results
LSA Results
Figure 3 shows the composite grid for each of the four factors considered in LSA for UGI: effectiveness; flooding mitigation; water quality protection; and greenspace opportunity. The spatial distribution of the grid reflects directly the urban emphasis of the approach used in the analysis. The composite grid mapping of the UGI effectiveness factor (Figure 3A), for example, identifies as most suitable areas those located in the urban cores of Ambos Nogales where public land uses (i.e., parks and schools) tend to be concentrated and slope gradient is low. The composite grids also reflect the contrast in the urban form of the two cities. Figure 3D depicting the spatial distribution of greenspace opportunities shows a grid with less area suitable for UGI in Nogales, Sonora, than in Nogales, Arizona, an outcome resulting from the most compact development and higher density characteristic of the Mexican city and the quasi-rural character of Nogales, Arizona. The overlaps between the suitability grids are significant across the four composite maps.
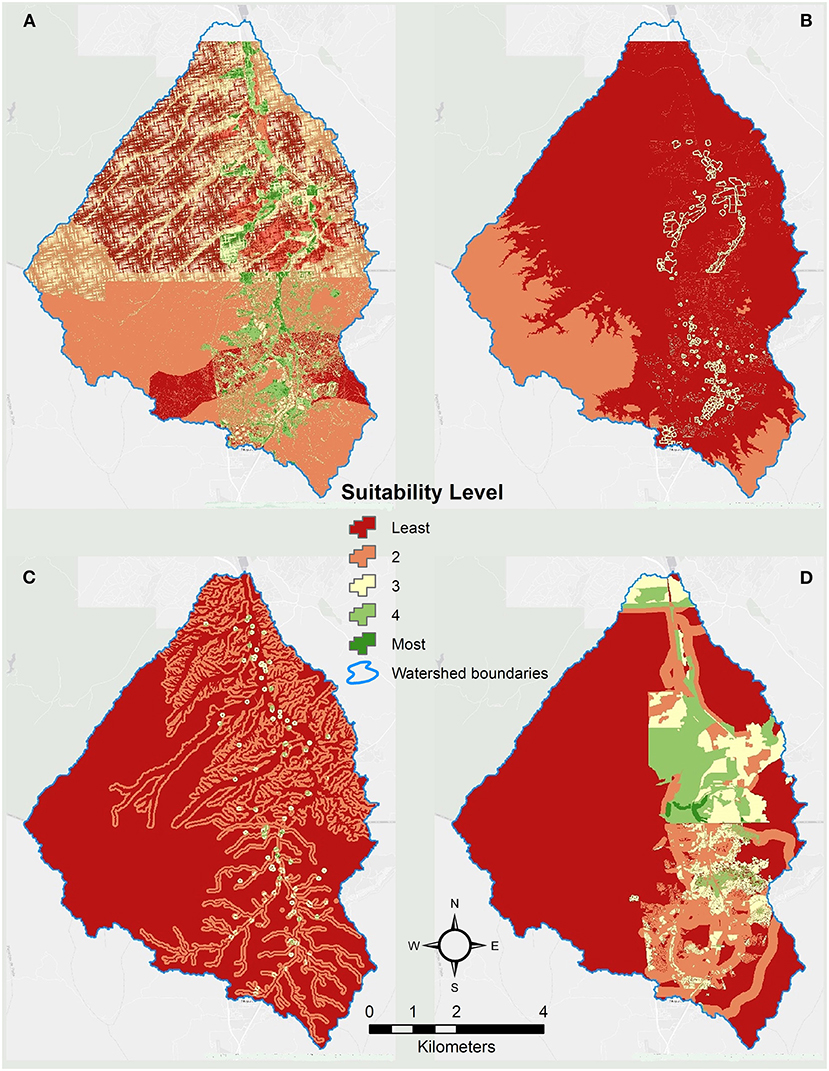
Figure 3. Composite maps showing locational distribution of factors interacting with (A) UGI effectiveness. (B) UGI flooding mitigation potential. (C) UGI water quality protection. (D) UGI greenspace opportunity.
As explained earlier, rather than generate one accumulated value across the grids, we opted for a weighted approach to combining the grids. A sample of 52 individuals proportionally distributed across the border was invited to answer a survey. The survey was approved by the IRB office of the University of Arizona and completed by 27 individuals (52% response rate). The resulting scores indicated a deeper concern for water quality issues among stakeholders in Ambos Nogales. Flooding risk was the second issue of concern, followed by concerns about open space availability in third place (Table 2). This pattern was consistent across the border and was used to prioritize the three UGI service factors using an ordinal scale, where 3 corresponds to the highest priority factor and 1 to the lowest. These priorities were used to compute weights for the four suitability factors according to Equation 1 (Table 2).
The overall UGI suitability map for the watershed involved the combination of the four suitability grids using the weights derived from stakeholders' survey (Figure 4). Figure 4A shows polygons representing most suitable areas for UGI after dissolving proximal grid-cells using a 50 meters distance band. The map contains a total of 166 polygons, with 109 located in Nogales, Sonora, and 57 in Nogales, Arizona. The average size of the polygons in Nogales, Sonora, was 8.5 hectares, while in Nogales, Arizona, was 12.3 hectares. In total, the land area identified as UGI suitable is ~1,640 hectares, an extent that is equal to 6.7% of the watershed territory and 21.2% of the urban footprint of Nogales, Sonora, and Nogales, Arizona, combined.
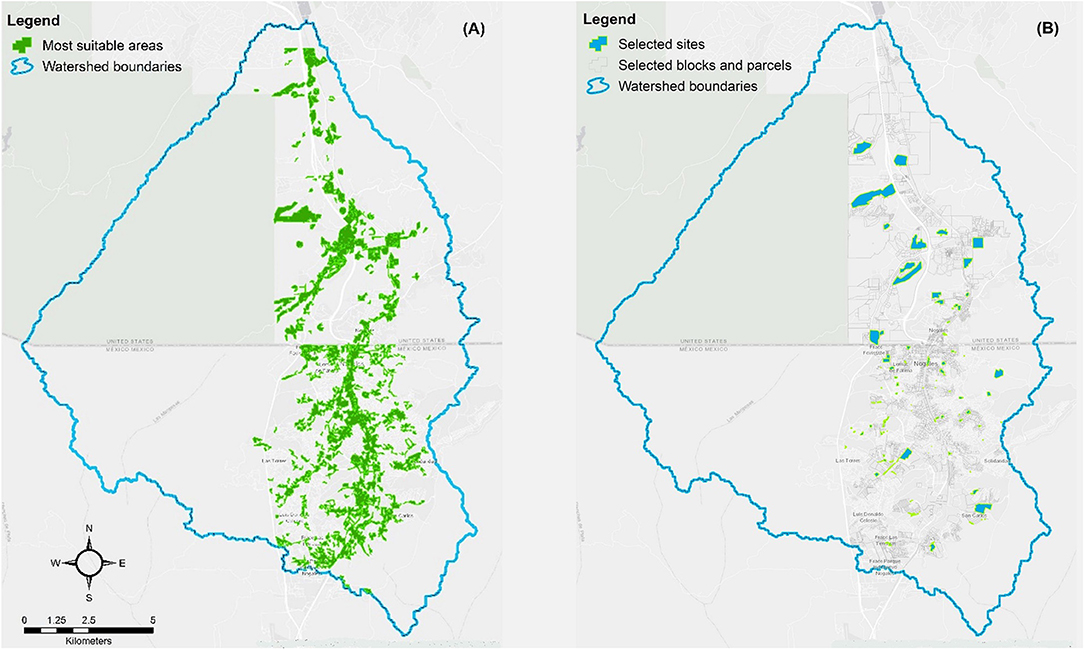
Figure 4. LSA outcomes: (A) most suitable areas for UGI in the watershed. (B) Ambos Nogales UGI network selected parcels and sites.
Although these polygons represent areas within the watershed with the highest potential for UGI placement, many of them contain land uses and functions that limit the type and features of green infrastructure practices that can be placed on them. Therefore, as a final step, the overall UGI suitability raster was intersected with the LBCS layer to evaluate parcels within or near the most suitable area with the highest potential to be incorporated into the Ambos Nogales UGI network. A decision matrix integrating the dimensions of the two-sided suitability assessment framework used in the LSA guided a process to select sites with the highest potential for retrofit, redevelopment, or development using UGI practices. This process was conducted using a combination of visual inspection using Google Earth imagery or in situ observations by the research team. The results were converted to a polygon file using the previously explained method. Figure 4A shows the selected sites in relation to the most suitable areas and the urban grid of Ambos Nogales.
A total of 103 potential UGI sites were identified in the watershed, 83 of which were located in Nogales, Sonora, and 21 in Nogales, Arizona. South of the border, potential sites averaged 0.96 hectares, significantly smaller than sites in Nogales, Arizona, that averaged 10.3 hectares. These differences in the number and extent of potential UGI sites are explained by the existing contrasts between the two cities regarding urban form and density. First, Nogales, Sonora, has an intensively developed urban core, while Nogales, Arizona, is a diffuse settlement with large interspersed hilly and underdeveloped areas. Second, in correspondence to a ten times bigger population, the number of schools, parks and other public use lands is significantly larger in Nogales, Sonora, a factor that influenced the final selection of sites. In fact, public parks, school, and sport facilities represent 52% of the sites.
The next step in the planning process was to apply urban design standards to decide which UGI practice is the best fit for each of the sites and how to connect them, so they become nodes of a cross-border green infrastructure network linking the two cities. A key feature of UGI is its functional versatility and adaptability, which allow its use of virtually any space available as part of strategy to detain and infiltrate stormwater in intensively built-up areas.
Hydrological Modeling
A decrease is observed for runoff, peak flow and most notably, sediment yield, in both planes and streams in Ambos Nogales when the proposed UGI is used (Table 3). This decrease corresponds with findings attributed to small rock check dams (Norman and Niraula, 2016; Norman et al., 2017; Tosline et al., 2020; Callegary et al., 2021). In Ambos Nogales, where rainfall runoff creates hazardous flooding and sediment deposition which can reduce the capacity of conveyance infrastructure—these changes would be most welcome. Results of running the model on the change scenarios show a slight decrease of infiltration in streams (~-2%), which we attribute to the concurrent decrease in flow and water availability there, and an increase in infiltration on the hillslopes (a.k.a., KINEROS planes; ~+2%; Table 3), which corresponds to findings associated with rock gabion influence (Norman et al., 2019) and adds to the potential recharge of the aquifer in this arid environment.
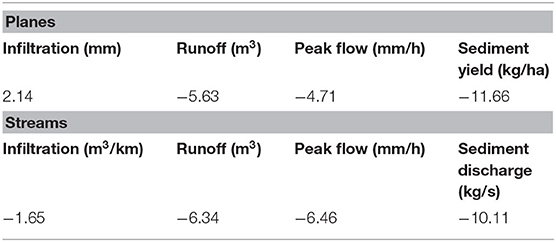
Table 3. Summary of KINEROS2 average percent change outputs results from the two scenarios (before UGI intervention and after UGI intervention) in terms of total watershed changes: average percent runoff (m3); peak flow (mm/h); and sediment yield (kg/ha) and infiltration (m3/km) for planes that intersect with a proposed UGI placement and all streams (all streams are within 2 miles of a UGI).
The uncalibrated model results are designed to evaluate relative change, not give quantitative estimates of runoff and erosion. Thus, the results give a qualitative estimate of the benefits of UGI structures. Nevertheless, when proposed UGI interventions are simulated as wetlands at suitable sites in Ambos Nogales, the watershed model predicts ecosystem services congruent with observed benefits that rock detention structures provide in similar arid and semiarid ecosystems—in terms of increased flood detention, erosion control, water provisioning and water purification (Norman, 2020). We parsed out the planes and streams in the watershed to analyze the trends associated with their outputs (Figure 5). Despite some variation in numbers, the general trends were similar for each output (runoff, infiltration, sedimentation and peak flows) and across the watershed.
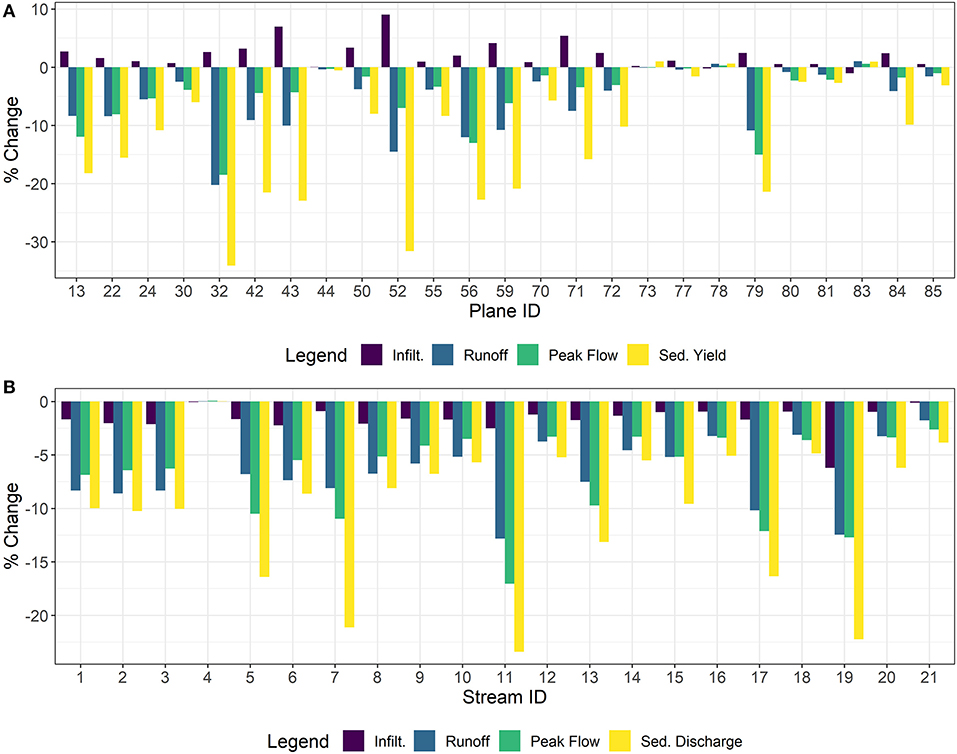
Figure 5. Percent change in KINEROS2 hydrological outputs between current land use and after implementation of UGI in (A) planes and (B) streams.
We then mapped out these trends to illustrate the spatial relationship of this variation in planes and streams, calling attention to the areas in the watershed that will be most impacted by UGI (Figure 6). Despite converting only 1.26% of the total watershed area to UGI, there are great benefits expected to be gained for Ambos Nogales. In the downtown area (DowntwnNog in Figure 6), the model predicts the largest reduction in peak flows and runoff, which is also predicted to decrease the sedimentation (Figures 6A,C,D). Increased runoff and flooding have been ongoing stormwater management challenges in the downtown area in Nogales, Sonora, and Nogales, Arizona, and the proposed UGI will help to address them. The subwatershed between Downtown Nogales (planes 24: −5%; 70: −1%; 71: −3%; 72: −3%; 73: 0%; 77: 0%) and Cuevitas (plane 22: −8%) is also predicted to greatly benefit from reductions in peak flow. In this area, UGI has been installed at the Embarcadero as a pilot as part of the broader project. Other areas that are predicted to benefit from reduced flooding and associated sediment transport include the Cuesta Blanca (just south of Capulines) and Potrero Creek subwatersheds (a collection of adjacent planes). Conversely, the neighboring subwatersheds of Mariposa and Capulines are predicted to increase these outputs. The majority of the watershed benefits from stormwater detention and erosion control associated with UGI. Increases in infiltration are predicted at all locations in the watershed (Figure 6B). As expected, decreases in runoff volume and peak flow, and increases in infiltration are greatest in planes where UGI are located. We do not have an accurate model of the aquifer underlying Nogales, but the increased infiltration observed creates the potential for watershed-wide increases in recharge, which would benefit regional water supply.
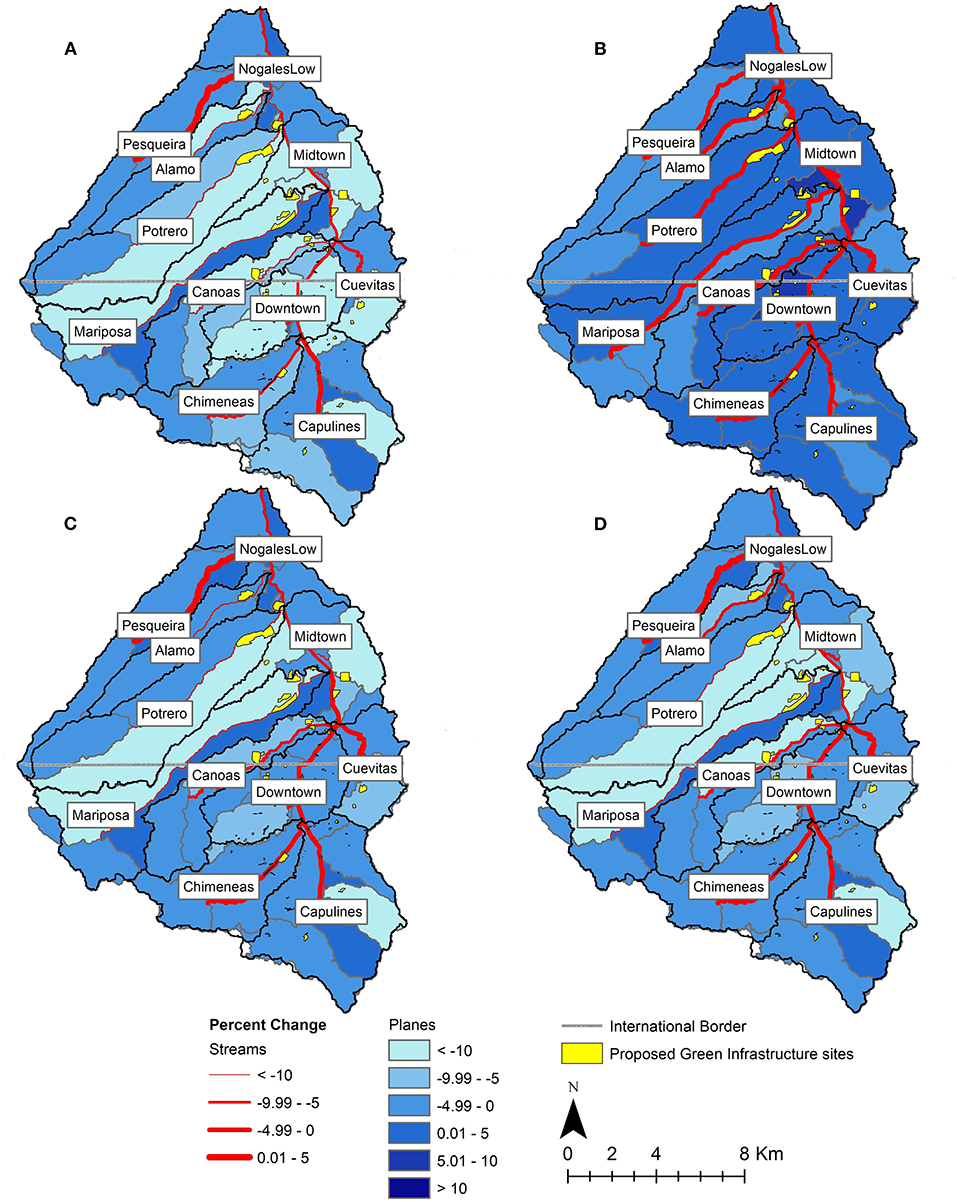
Figure 6. Maps depicting percent change for planes and streams in (A) sediment yield/discharge (kg/ha, kg/s). (B) Infiltration (mm, m3/km). (C) Peak flow (mm/h); and (D) runoff (m3) after implementation of green infrastructure.
To complement the model analysis, a rain garden (a type of UGI which stores water and facilitates infiltration) with a 25,000 L capacity was installed at Secundaria General # 3, a middle school in Nogales, Sonora, identified as a suitable site for the Ambos Nogales UGI network. The pre-implementation conditions are described in the upper half of Table 4. The rain garden consisted of three interconnected local catchments constructed using the trincheras system. Peak flow for the 10-year storm prior to UGI installation was computed, using the Rational Method, to be 221.1 l/s. After installation of UGI, the peak flow was computed to be 204.1 l/s, resulting in a 7% decrease, as described in Table 4. When modeling a 2-year 1-h time-depth storm event of 30.7 mm in KINEROS, the corresponding plane for the school (plane 85) shows a 4% decrease in peak flow when comparing pre-UGI to post-UGI.
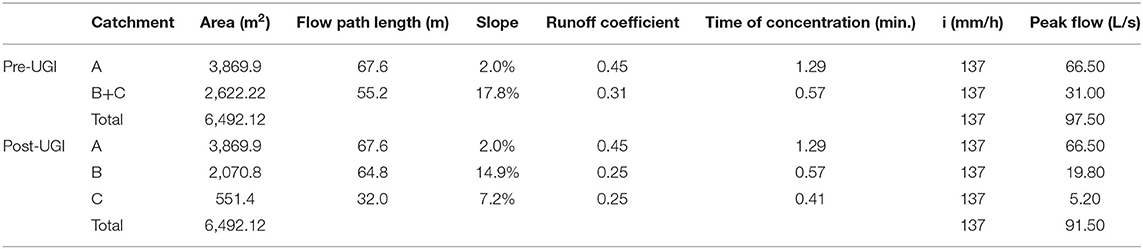
Table 4. Rational method variables and results for pre- and post-UGI implementation for the 10-year return period storm.
Discussion
The LSA conducted in this study differs from conventional approaches to locate UGI because it considers runoff control in tandem with ecological services and social equity. This framework is consistent with conceptualizations of UGI as delivering essential goods and services to urban communities while simultaneously addressing development driven hydrological modification, which results in water quality impacts and increased flood risk (Tzoulas et al., 2007; Ahern, 2011; Shi, 2020; Surma, 2020). The multifunctionality of UGI allows for the identification of places that can be retrofitted, redeveloped, or developed to accommodate different services producing a range of social, environmental, and economic benefits in the same piece of land.
In addition, UGI is flexible because it can be scaled to fit dense urban areas where land is limited and urban growth places pressure on available land. That is the case of the compact, rapidly growing, and unequal urbanization occupying the Ambos Nogales basin. As reported, the LSA produced a broad spectrum of location alternatives for UGI placement, ranging from small residual spaces in poor neighborhoods of Nogales, Sonora, to large open areas in need of preservation and protection in Nogales, Arizona. The installation of small rain gardens and trincheras to detain and infiltrate rainfall and mitigate erosion and landslides are an example of UGI interventions that can be used effectively in residual spaces to re-naturalize Ambos Nogales and promote local environmental awareness and stewardship (e.g., Buijs et al., 2019). Large areas like the wetlands at Potrero Creek (Figure 6) in Nogales, Arizona, can benefit from the construction of a series of cascading bioretention cells helping to reduce the flow of stormwater and decreasing the sediments and pollutant loads originating on nearby roads and residential areas.
The total storage capacity and area for infiltration impact performance of UGI practices. However, targeted UGI can have a significant impact despite a small footprint. Here, as an outcome of the LSA, only 1.26% of the total area in the watershed was identified for UGI treatment. The hydrologists on the team were initially skeptical of the impacts this small change in land use/land cover would have on the overall hydrologic response. However, estimated changes in the water budget were significant for the watershed and prompted one to consider how scenarios expanding the footprint of localized interventions and their interconnection might further increase infiltration, while decreasing peak flows and sediment transport in the watershed.
The results of the KINEROS AGWA 3.x at the watershed scale were compared to a site scale analysis using the Rational Method on one of the demonstration sites selected through the LSA. Both methods showed a similar magnitude of peak flow reduction. The Rational Method and the KINEROS AGWA 3.x are both models, and we have not collected any field data, so the field performance remains uncertain. However, the benefit of this comparison is that the Rational Method application considered the specific area, volume and materials used in a UGI installation in the Ambos Nogales watershed. This provides additional support for the approach to UGI modeling used within KINEROS AGWA 3.x.
In addition to impacting the surface water budget, UGI can impact subsurface hydrological processes. There is limited understanding of the Santa Cruz aquifer which underlies Ambos Nogales and is an important regional water source (Scott et al., 2012). UGI practices, which facilitate infiltration, may or may not increase recharge depending on how infiltration interacts with evapotranspiration and baseflow processes (Bhaskar et al., 2018). Future investigations to determine how infiltration impacts the aquifer are important for UGI design and regional water planning. UGI placement can also influence recharge as surface groundwater connectivity differs in space. Because of the urban emphasis of this study, many of the sites identified through the LSA are located at the outlets of many of the subwatersheds of Ambos Nogales, yet traditional restoration projects are designed to first treat headwaters of tributaries. Therefore, the effect on the hydrological budget of the proposed UGI cross-border network is subject to planning and design decisions affecting their configuration and connectivity. Future research comparing different placement scenarios of UGI in the watershed, given the same total area of land being treated, is warranted.
KINEROS AGWA 3.x, like all models, has limitations; sources of error include the model structure and parameterization, and the model inputs. In the case of Ambos Nogales, the land use data is outdated (2008) and the soils data is coarse (1:5,000,000 scale), which may lead to errors in modeled infiltration and runoff. Additionally, there were no discharge measurements available to calibrate the model. In place of calibration, model parameters were selected based on the available data (e.g., soil types). Field data, such as streamflow measurements would improve this parameterization, reducing error and uncertainty. However, given that the model is applied to compare the status quo with the addition of UGI, parameters are held constant across this comparison. Therefore, even with these limitations, the model is sufficient for the purpose of the analysis: to understand the watershed's response to rainfall and how the addition of UGI alters that response.
UGI planning has the potential to be a useful approach for regulating the interaction between land use/land cover change, urban growth, and environmental management because such a network is a spatially explicit structure and its components operate as its territorial anchors. Land use change is arguably the most significant socioeconomic force altering the Ambos Nogales watershed, which drives flash floods, water quality deterioration, damage to essential urban infrastructure, public health hazards, and economic disruption on both sides of the border (Myint et al., 2010; Norman et al., 2010a).
UGI is compatible with a binational approach to integrated stormwater management because of its emphasis on restoring and maintaining the connection across scales and across the border needed for a healthy watershed. These interconnections link components and processes sustaining a variety of regulating, provisioning and cultural services important for urban resilience and sustainability. The framework used in this study relies on an integrated approach to stormwater management by using the Ambos Nogales watershed as a planning unit, rather than two watersheds artificially separated by the international border. The practice of managing the United States and Mexican sections of the watershed as two separate units is not only ill-advised from a hydrological and ecological perspective but also incongruent with the experiential knowledge of residents and water experts in Ambos Nogales, who see a benefit in cross-border coordination and exchange (Marquez Reyes, 2010; Freimund, 2020; Schwartz, 2021). In the context of this project, UGI planning is advanced as a debordering strategy laying down the components of a binational network with green nodes and corridors of varying size, shape, and function that need to be interconnected through cross-border cooperation and planning.
Finally, stormwater management has gone from a field dominated by hydrologists and engineers, to a multidisciplinary field involving the urban planners, landscape architects, sociologists, risk managers, biologists, and other specialists. This impetus for this transition comes from an expansive urbanization process across the world and the growing complexity of the interaction between the natural and built environments in the context of a changing climate. However, the slow speed at which interdisciplinarity and multifunctionality sinks into the mindset and practices of local water managers delays the adoption of multidimensional approaches like UGI. As suggested by Giner et al. (2019), a strategy that can help accelerate this process is developing technical tools that can be used to update planning and urban design practices and standards and have implications for water management along the United States-Mexico border. In response to this imperative, this study has developed an approach that is placed-based and sensitive to local needs. To develop this approach, we engaged with communities on both sides of the international boundary and formed a research team with the skills to work in a cross-cultural setting. As observed by Nalven (1986) in an early study exploring cross-border environmental cooperation, a “technical planning perspective can easily identify a common topography, meteorology, and problems of urbanization in border twin cities.” However, this solely technical approach fails to see the importance of a binational perspective and the influence of broad and professional societal values for the implementation of cross-border planning and design ideas. In response, we framed this study as a multi-perspective research project, designed as a collaborative learning process involving hydrologists, planners, architects, sociologists and practitioners from both sides of the border.
Conclusions
For decades, environmental studies focused on the United States-Mexico border have highlighted the need for better cross-border coordination and management of urban hazards and shared ecosystems (Mumme and Nalven, 1988; Morehouse, 1995; Varady et al., 2013). One of the elements that has hampered the progress of cross-border environmental cooperation in the region has been the lack of programmatic approaches to planning and implementation, addressing some of the most urgent challenges for sustainable water management in border cities. Using the case of Ambos Nogales, this article has demonstrated that UGI provides a flexible and practical approach to the various challenges of border cities and cross-border environmental planning. When UGI planning is supported by an LSA and modeling integrating geographical, hydrological, urban, and social factors, and using a cross-border lens, UGI can be designed to address concurrent urban and environmental challenges faced by border cities.
Transboundary watersheds present an added challenge in creating sustainable, resilient, and livable cities. In Ambos Nogales, as in other transboundary regions, rules, data sources, procedures, finances and standards change as you cross the national boundary. However, hydrologically, Ambos Nogales is a single watershed system. Development across the watershed has exacerbated flood hazards and water quality challenges, and, as demonstrated here, coordination across the watershed in interventions such as UGI has potential to efficiently tackle these challenges and enhance benefits. Strategic placement of UGI across the Ambos Nogales watershed, considering both the conditions required for successful UGI and where the community would benefit most from UGI placement, reduced peak flows and sediment loads while increasing green space access and using under-utilized spaces. The results demonstrate the potential benefits of taking an interdisciplinary and transboundary approach.
Creation and strengthening of institutions supporting cross-border dataset development, intervention planning, and implementation would enhance benefits on both sides of the border. As we learned through this study, the combination of strong problem-oriented research by regional universities and local leadership are the drivers behind the widespread acceptance of UGI in Ambos Nogales. Going forward, identifying ways to build a stronger institutional capacity for collaboration in addressing shared development, flooding and water quality challenges would benefit the full Ambos Nogales watershed, and other transboundary watersheds grappling with urbanization, flooding and water quality challenges.
Data Availability Statement
The original contributions presented in the study are included in the article/supplementary material, further inquiries can be directed to the corresponding author.
Ethics Statement
The studies involving human participants were reviewed and approved by University of Arizona, IRB. Written informed consent for participation was not required for this study in accordance with the national legislation and the institutional requirements.
Author Contributions
FL-V designed the study, conducted the LSA, created figures, wrote and edited. AA conducted data analysis, ran the hydrological model, created figures, and wrote and edited. MG conducted literature review, created figures, and wrote and edited. LN developed the hydrological model, created figures, and wrote and edited. EC-R conducted field work, reviewed candidate UGI location based on field condition, and conducted rational method calculations.
Funding
This research has been supported by a grant from the Border 2020 U.S.-Mexico Environmental Program through the North American Development Bank (NADB). The funding was provided to Arizona State University under Technical Assistance Agreement TAA20-004 for the project Sustainable Strategies for Stormwater and CSOS Control in Ambos Nogales.
Conflict of Interest
The authors declare that the research was conducted in the absence of any commercial or financial relationships that could be construed as a potential conflict of interest.
Publisher's Note
All claims expressed in this article are solely those of the authors and do not necessarily represent those of their affiliated organizations, or those of the publisher, the editors and the reviewers. Any product that may be evaluated in this article, or claim that may be made by its manufacturer, is not guaranteed or endorsed by the publisher.
Acknowledgments
The authors wish to thank our partners on this project including colleagues from El Colegio de la Frontera Norte; Fay Jones School of Architecture and Design, University of Arkansas; Seeds/Semillas Community Agriculture; Instituto Municipal de Investigación y Planeación de Nogales; School of Landscape Architecture and Planning, University of Arizona. Lastly, we appreciate the careful pre-submission peer reviews of this manuscript provided by Deborah Tosline and Natalie R. Wilson. Scientific modeling and hydrologic background information was provided with support from the Land Change Science (LCS) Program, under the Core Science Systems Mission Area of the US Geological Survey (USGS). Any use of trade, firm, or product names is for descriptive purposes only and does not imply endorsement by the U.S. Government.
References
ADWR. (2020). Arizona Groundwater Site Inventory of Arizona, Arizona Department of Water Resources. Available online at: https://new.azwater.gov/gis (accessed January 3, 2021).
Ahern, J. (2011). From fail-safe to safe-to-fail: sustainability and resilience in the new urban world. Landsc. Urban Plan. 100, 341–343. doi: 10.1016/j.landurbplan.2011.02.021
Avellaneda, P. M., Jefferson, A. J., Grieser, J. M., and Bush, S. A. (2017). Simulation of the cumulative hydrological response to green infrastructure. Water Resour. Res. 53, 3087–3101. doi: 10.1002/2016WR019836
Bhaskar, A. S., Hogan, D. M., Nimmo, J. R., and Perkins, K. S. (2018). Groundwater recharge amidst focused stormwater infiltration. Hydrol. Process. 32, 2058–2068. doi: 10.1002/hyp.13137
Brady, L. M., Gray, F., Castaneda, M., Bultman, M., and Bolm, K. S. (2002). Preliminary United States-Mexico border watershed analysis, twin cities area of Nogales, Arizona and Nogales, Sonora. Report 2002-112. U.S. Geological Survey, Tucson, AZ. doi: 10.3133/ofr02112
Brown, C. P., and Mumme, S. (2000). Applied and theoretical aspects of binational watershed councils (consejos de cuencas) in the U.S. Mexico borderlands. Nat. Resour. J. 40, 895–929. Available online at: https://digitalrepository.unm.edu/nrj/vol40/iss4/7
Buijs, A., Hansen, R., der Jagt, S., Ambrose-Oji, B., Elands, B., Lorance Rall, E., et al. (2019). Mosaic governance for urban green infrastructure: upscaling active citizenship from a local government perspective. Urban For. Urban Green. 40:53–62. doi: 10.1016/j.ufug.2018.06.011
Bureau of Transportation Statistics. (2021). Border Crossing/Entry Data. Washington, DC: Bureau of Transportation Statistics.
Callegary, J. B., Norman, L. M., Eastoe, C. J., Sankey, J. B., and Youberg, A. (2021). Preliminary assessment of carbon and nitrogen sequestration potential of wildfire-derived sediments stored by erosion control structures in forest ecosystems, Southwest USA. Air Soil Water Res. 14, 11786221211001768. doi: 10.1177/11786221211001768
Campbell-Arvai, V., and Lindquist, M. (2021). From the ground up: Using structured community engagement to identify objectives for urban green infrastructure planning. Urban For. Urban Green. 59, 127013. doi: 10.1016/j.ufug.2021.127013
City of Nogales. (2020a). Parks and Recreation Information, Department of Parks and Recreation. Available online at: https://nogalesaz.gov/p-r/parks-rec-information/ (accessed November 10, 2020).
City of Nogales. (2020b). Vision 2020: City of Nogales General Plan. The Planning Center, Nogales, A. Z. Retrieved from: https://nogalesaz.gov/wp-content/uploads/Files/3-Nogales-General-Plan-Adopted-8-5-2020.pdf
EEA (2019). Tools to Support Green Infrastructure Planning and Ecosystem Restoration. European Environmental Agency. doi: 10.2800/562602
Freimund, C. (2020). Perceptions of flood risk and resilience in Ambos Nogales. Tucson, AZ: The University of Arizona.
Garcia-Cuerva, L., Berglund, E. Z., and Rivers, L. (2018). An integrated approach to place Green Infrastructure strategies in marginalized communities and evaluate stormwater mitigation. J. Hydrol. 559, 648–660. doi: 10.1016/j.jhydrol.2018.02.066
García-Perez, H., and Lara-Valencia, F. (2021). Jóvenes, lluvia y vulnerabilidad en Ambos Nogales. Technical Report 1/05, Infraestructura Verde para Ambos Nogales, Arizona State University, Tempe, AZ.
Giner, M.-E., Córdova, A., Vázquez-Gálvez, F. A., and Marruffo, J. (2019). Promoting green infrastructure in Mexico's northern border: the Border Environment Cooperation Commission's experience and lessons learned. J. Environ. Manage. 248, 109104. doi: 10.1016/j.jenvman.2019.06.005
Goodrich, D. C., Burns, I. S., Unkrich, C. L., Semmens, D. J., Guertin, D. P., Hernandez, M., et al. (2012). KINEROS2/AGWA: model use, calibration, and validation. Trans. ASABE 55, 1561–1574. doi: 10.13031/2013.42264
Gorelick, N., Hancher, M., Dixon, M., Ilyushchenko, S., Thau, D., and Moore, R. (2017). Google Earth Engine: Planetary-scale geospatial analysis for everyone. Remote Sens. Environ. 31, 799–815 doi: 10.1016/j.rse.2017.06.031
Hansen, R., Olafsson, A. S., van der Jagt, A. P. N., Rall, E., and Pauleit, S. (2019). Planning multifunctional green infrastructure for compact cities: What is the state of practice? Ecol. Indic. 96, 99–110. doi: 10.1016/j.ecolind.2017.09.042
Hansen, R., and Pauleit, S. (2014). From multifunctionality to multiple ecosystem services? A conceptual framework for multifunctionality in green infrastructure planning for urban areas. Ambio 43, 516–529. doi: 10.1007/s13280-014-0510-2
Hoover, F.-A., Meerow, S., Grabowski, Z. J., and McPhearson, T. (2021). Environmental justice implications of siting criteria in urban green infrastructure planning. J. Environ. Policy Plann. 2021, 1–18. doi: 10.1080/1523908X.2021.1945916
Huth, H., and Tinney, C. (2008). “Causes and consequences of monsoonal flooding in Nogales, Sonora,” in Proceedings of a USGS Workshop on Facing Tomorrow's Challenges Along the US-Mexico Border—Monitoring, Modeling, and Forecasting Change Within Arizona, 51.
IMIP (2020a). Lista de pozos públicos urbanos 2020. Nogales, Sonora: Instituto Municipal de Investigación y Planeación.
INEGI (2016a). Conteo de Población 2015. Aguascalientes, Ags: Instituto Nacional de Estadística y Geografia. Available online at: https://www.inegi.org.mx (Sociodemographic data and boundary shape files) (accessed October 5, 2020).
INEGI (2019). Directorio Estadístico Nacional de Unidades Económicas (DENUE) 11_2018. Aguascalientes, Ags: Instituto Nacional de Estadística y Geografia. Available online at: http://www.beta.inegi.org.mx/app/descarga/?ti=6 (accessed October 5, 2020).
INEGI. (2020). Marco geoestadístico, Septiembre 2019. Aguascalientes, Ags: Instituto Nacional de Estadística y Geografia. Available online at: https://www.inegi.org.mx/app/mapas/?t=0170000000000000 (accessed October 5, 2020).
Kennedy, R., Goodrich David, C., and Unkrich Carl, L. (2013). Using the KINEROS2 modeling framework to evaluate the increase in storm runoff from residential development in a semiarid environment. J. Hydrol. Eng. 18, 698–706. doi: 10.1061/(ASCE)HE.1943-5584.0000655
Korgaonkar, Y., Guertin, D. P., Goodrich, D. C., Unkrich, C., Kepner, W. G., and Burns, I. S. (2018). Modeling urban hydrology and green infrastructure using the AGWA urban tool and the KINEROS2 model. Front. Built Environ. 4, 1–15. doi: 10.3389/fbuil.2018.00058
Korgaonkar, Y., Guertin, D. P., Meixner, T., and Goodrich, D. C. (2021). Hydrological modeling of green infrastructure to quantify its effect on flood mitigation and water availability in the high school watershed in Tucson, AZ. ISPRS Int. J. Geo-Inf. 10, 443. doi: 10.3390/ijgi10070443
Koutsoyiannis, D., Kozonis, D., and Manetas, A. (1998). A mathematical framework for studying rainfall intensity-duration-frequency relationships. J. Hydrol. 206 (1): 118–135. doi: 10.1016/S0022-1694(98)00097-3
Kuller, M., Bach, P. M., Ramirez-Lovering, D., and Deletic, A. (2017). Framing water sensitive urban design as part of the urban form: a critical review of tools for best planning practice. Environ. Modell. Softw. 96, 265–282. doi: 10.1016/j.envsoft.2017.07.003
Lara-Valencia, F. (2021). An urban function perspective on land use/land cover analysis in border conurbations: the case of Ambos Nogales. Technical Report, SOLTA-C-19-008: sustainable strategies for stormwater and CSOs control in Ambos Nogales, Arizona State University, Tempe, AZ.
Lara-Valencia, F., and Herzog, L. (2021). “Border cities: spatial and institutional expressions of bordering,” in Border Cities and Territorial Development, Regions and Cities Series, ed E. Medeiros (Routledge), 76–96.
Levick, L. (2006) USE. Automated Geospatial Watershed Assessment Tool (AGWA): Uncertainty Analysis of Common Input Data. U. S. Environmental..
Levick, L. R., Semmens, D. J., Guertin, D. P., Burns, I. S., Scott, S. N., Unkrich, C. L., et al. (2004). “Adding global soils data to the automated geospatial watershed assessment tool (AGWA),” in Proceedings of the 2nd International Symposium on Transboundary Waters Management.
Lewellyn, C., Lyons Cara, E., Traver Robert, G., and Wadzuk Bridget, M. (2016). Evaluation of seasonal and large storm runoff volume capture of an infiltration green infrastructure system. J. Hydrol. Eng. 21, 4015047. doi: 10.1061/(ASCE)HE.1943-5584.0001257
Liu, L., and Jensen, M. B. (2018). Green infrastructure for sustainable urban water management: Practices of five forerunner cities. Cities 74, 126–133. doi: 10.1016/j.cities.2017.11.013
Malczewski, J. (2006). GIS-based multicriteria decision analysis: a survey of the literature. Int. J. Geogr. Inf. Sci. 20, 703–726. doi: 10.1080/13658810600661508
Marquez Reyes, B. J. (2010). Floods, Vulnerability, and the US-Mexico Border: A Case Study of Ambos Nogales. Arizona State University.
Meerow, S. (2020). The politics of multifunctional green infrastructure planning in New York City. Cities 100, 102621. doi: 10.1016/j.cities.2020.102621
Morehouse, B. (1995). A functional approach to boundaries in the context of environmental issues. J. Borderl. Stud. 10, 53–73. doi: 10.1080/08865655.1995.9695474
Mulvany, T. (1851). On the use of self-registering rain and flood gauges in making observations of the relations of rainfall and of flood discharges in a given catchment. Proc. Inst. Civ. Eng. Irel. 4, 18–33
Mumme, S. P., and Nalven, J. (1988). National perspectives on managing transboundary environmental Hazards: the US-Mexico Border Region. J. Borderlands Stud. 3, 39–67. doi: 10.1080/08865655.1988.9695351
Myint, S. W., Jain, J., Lukinbeal, C., and Lara-Valencia, F. (2010). Simulating urban growth on the US-Mexico border: Nogales, Arizona, and Nogales, Sonora. Can. J. Remote Sens. 36, 166–184. doi: 10.5589/m10-038
Nalven, J. (1986). Transboundary environmental problem solving: social process, cultural perception. Nat. Resour. J. 26, 793.
NCEI (2021). U.S. Climate Normals. NOAA Available online at: https://www.ncei.noaa.gov/products/land-based-station/us-climate-normals (accessed 1 July 2021).
NOAA (2011). Atlas 14 Precipitation-Frequency Atlas of the United States Volume 1 Version 5.0: Semiarid Southwest (Arizona, Southeast California, Nevada, New Mexico, Utah). Washington, D.C.: National Centers for Environmental Information.
NOAA. (2021). Storm Events Database. Washington, D.C.: National Centers for Environmental Information
Norman, L., Villarreal, M., Pulliam, H. R., Minckley, R., Gass, L., Tolle, C., et al. (2014). Remote sensing analysis of riparian vegetation response to desert marsh restoration in the Mexican Highlands. Ecol. Eng. 70, 241–254. doi: 10.1016/j.ecoleng.2014.05.012
Norman, L. M. (2020). Ecosystem services of riparian restoration: a review of rock detention structures in the Madrean Archipelago Ecoregion. Air Soil Water Res. 13, 1178622120946337. doi: 10.1177/1178622120946337
Norman, L. M., Brinkerhoff, F., Gwilliam, E., Guertin, D. P., Callegary, J., Goodrich, D. C., et al. (2016). Hydrologic response of streams restored with check dams in the Chiricahua mountains, Arizona. River Res. Appl. 32, 519–527. doi: 10.1002/rra.2895
Norman, L. M., Caldeira, F., Callegary, J., Gray, F., O' Rourke, M. K., Meranza, V., et al. (2012a). Socio-environmental health analysis in Nogales, < city>Sonora < /city>, Mexico. Water Qual. Expo. Health 4, 79–91. doi: 10.1007/s12403-012-0067-x
Norman, L. M., Callegary, J. B., Lacher, L., Wilson, N. R., Fandel, C., Forbes, B. T., et al. (2019). Modeling Riparian Restoration Impacts on the Hydrologic Cycle at the Babacomari Ranch, SE Arizona, USA. Water 11, 381. doi: 10.3390/w11020381
Norman, L. M., Guertin, D. P., and Feller, M. (2008). A coupled model approach to reduce nonpoint-source pollution resulting from predicted urban growth: a case study in the ambos nogales watershed. Urban Geogr. 29, 496–516. doi: 10.2747/0272-3638.29.5.496
Norman, L. M., Guertin, D. P., Hernández, D. P., Barnett, A. S., and Ashton-Reis, K. (2004). Binational Digital Soils Map of the Ambos Nogales Watershed, Southern Arizona and Northern Sonora, Mexico. Reston, VA: US Geological Survey.
Norman, L. M., Huth, H., Levick, L., Shea Burns, I., Phillip Guertin, D., Lara-Valencia, F., et al. (2010a). Flood hazard awareness and hydrologic modelling at Ambos Nogales, United States-Mexico border. J. Flood Risk Manag. 3, 151–165. doi: 10.1111/j.1753-318X.2010.01066.x
Norman, L. M., Levick, L., Guertin, D., Callegary, J., Guadarrama, J. Q., Anaya, C. Z. G., et al. (2010b). Nogales Flood Detention Study. Reston, VA: U. S. Geological Survey.
Norman, L. M., and Niraula, R. (2016). Model analysis of check dam impacts on long-term sediment and water budgets in Southeast Arizona, USA. Ecohydrol. Hydrobiol. 16, 125–137. doi: 10.1016/j.ecohyd.2015.12.001
Norman, L. M., Sankey, J. B., Dean, D., Caster, J., DeLong, S., DeLong, W., et al. (2017). Quantifying geomorphic change at ephemeral stream restoration sites using a coupled-model approach. Geomorphology 283, 1–16. doi: 10.1016/j.geomorph.2017.01.017
Norman, L. M., Villarreal, M. L., Lara-Valencia, F., Yuan, Y., Nie, W., Wilson, S., et al. (2012b). Mapping socio-environmentally vulnerable populations access and exposure to ecosystem services at the U.S. Mexico borderlands. Appl. Geogr. 34, 413–424. doi: 10.1016/j.apgeog.2012.01.006
Norman, L. M., Villarreal, M. L., Wallace, C. S. A., Gil Anaya, C. Z., Arcos, I. D., and Gray, F. (2010c). A High-Resolution Land-Use Map; Nogales, Sonora, Mexico. Reston, VA: USGS.
Norman, L. M., and Wallace, C. S. A. (2008). Mapping Land Use/Land Cover in the Ambos Nogales Study Area. Reston: U.S. Geological Survey.
Norton, B. A., Coutts, A. M., Livesley, S. J., Harris, R. J., Hunter, A. M., and Williams, NSG. (2015). Planning for cooler cities: a framework to prioritise green infrastructure to mitigate high temperatures in urban landscapes. Landsc. Urban Plan. 134, 127–138. doi: 10.1016/j.landurbplan.2014.10.018
O'Brien, L., De Vreese, R., Kern, M., Sievänen, T., Stojanova, B., and Atmiş, E. (2017). Cultural ecosystem benefits of urban and peri-urban green infrastructure across different European countries. Urban For. Urban Green. 24, 236–248. doi: 10.1016/j.ufug.2017.03.002
Palmer, M. A., Liu, J., Matthews, J. H., Mumba, M., and D'Odorico, P. (2015). Water manage water in a green way. Science 349, 584–585. doi: 10.1126/science.aac7778
Pataki, D. E., Carreiro, M. M., Cherrier, J., Grulke, N. E., Jennings, V., Pincetl, S., et al. (2011). Coupling biogeochemical cycles in urban environments: ecosystem services, green solutions, and misconceptions. Front. Ecol. Environ. 9, 220. doi: 10.1890/090220
Pereira, J. M. C., and Duckstein, L. (1993). A multiple criteria decision-making approach to {GIS-based} land suitability evaluation. Int. J. Geogr. Inf. Syst. 7, 407–424. doi: 10.1080/02693799308901971
Richardson, M. I., Clemmons, S. D., and Walker, J. C. (1979). Soil Survey of Santa Cruz County and parts of Cochise and Pima Counties, Arizona. Washington, DC: United States Agriculture Department, Chemistry and Soils Bureau, University of Arizona Agricultural Experiment Station.
Santa Cruz County (2020). Park and Recreation. Available online at: https://www.santacruzcountyaz.gov/ImageRepository/Document?documentID=3149 (accessed November 16, 2020).
Schwartz, P. (2021). Reclaiming a Fractured Flow: Identifying Policy Barriers and Opportunities for Green Infrastructure in Ambos Nogales. Master of Arts, University of Arizona.
Scott, C. A., Megdal, S., Oroz, L. A., Callegary, J., and Vandervoet, P. (2012). Effects of climate change and population growth on the transboundary Santa Cruz aquifer. Clim. Res. 51, 159–170. doi: 10.3354/cr01061
Sheppard, P. R., Comrie, A. C., Packin, G. D., Angersbach, K., and Hughes, M. K. (2002). The climate of the U.S. Southwest. Clim. Res. 21, 219–238. doi: 10.3354/cr021219
Shi, L. (2020). Beyond flood risk reduction: how can green infrastructure advance both social justice and regional impact? Socio-Ecol. Pract. Res. 2, 311–320. doi: 10.1007/s42532-020-00065-0
Spatari, S., Yu, Z., and Montalto, F. A. (2011). Life cycle implications of urban green infrastructure. Environ. Pollut. 159, 2174–2179. doi: 10.1016/j.envpol.2011.01.015
Surma, M. J. (2020). “Urban green infrastructure for sustainable land use planning”, in International Conference on Sustainable Development (New York, NY). Available online at: https://ic-sd.org/wp-content/uploads/2020/11/Martyna-Surma.pdf
Tosline, D., Norman, L. M., Greimann, B. P., Cederberg, J., Huang, V., and Ruddell, B. L. (2020). Impacts of Grade Control Structure Installations on Hydrology and Sediment Transport as an Adaptive Management Strategy (Science and Technology Program Research and Development Office ST-2017-1751-01). Bureau of Reclamation. Available online at: https://data.usbr.gov/catalog/4414/item/6298 (accessed June 30, 2020).
Tzoulas, K., Korpela, K., Venn, S., Yli-Pelkonen, V., Kazmierczak, A., Niemela, J., et al. (2007). Promoting ecosystem and human health in urban areas using green infrastructure: a literature review. Landsc. Urban Plan. 81, 167–178. doi: 10.1016/j.landurbplan.2007.02.001
U.S. Geological Survey (2017). 1/3rd arc-second Digital Elevation Models (DEMs)—USGS National Map 3DEP Downloadable Data Collection. Reston, VA: U.S. Geological Survey.
US Census Bureau (2019a). American Community Survey. Available online at: https://www.census.gov/programs-surveys/acs/news/data-releases.2019.html (accessed July 23, 2020).
US Census Bureau (2019b). Cartographic Boundary Files. Available online at: https://www.census.gov/geographies/mapping-files/time-series/geo/carto-boundary-file.html
US Census Bureau (2020). 2015: ACS 5-Year Estimates Data Profiles by Urban Areas. Washington, D.C: U.S. Census Bureau.
USDA. (1986). Urban Hydrology for Small Watersheds. Available online at: https://www.nrcs.usda.gov/Internet/FSE_DOCUMENTS/stelprdb1044171.pdf
Van Oijstaeijen, W., Van Passel, S., and Cools, J. (2020). Urban green infrastructure: a review on valuation toolkits from an urban planning perspective. J. Environ. Manage. 267, 110603. doi: 10.1016/j.jenvman.2020.110603
Varady, R. G., Scott, C. A., Wilder, M., Morehouse, B., Pablos, N. P., and Garfin, G. M. (2013). Transboundary adaptive management to reduce climate-change vulnerability in the western US–Mexico border region. Environmental Science and Policy 26, 102–112. doi: 10.1016/j.envsci.2012.07.006
Wheater, H., Sorooshian, S., and Sharma, K. D. (2007). Hydrological Modelling in Arid and Semi-Arid Areas. Cambridge: Cambridge University Press.
Wilson, N. R., and Norman, L. M. (2018). Analysis of vegetation recovery surrounding a restored wetland using the normalized difference infrared index (NDII) and normalized difference vegetation index (NDVI). Int. J. Remote Sens. 39, 3243–3274. doi: 10.1080/01431161.2018.1437297
Woolhiser, D. A., Smith, R. E., and Goodrich, D. C. (1990). KINEROS: A Kinematic Runoff and Erosion Model: Documentation and User Manual. United States, Agricultural Research Service.
Yang, J., and Wang, Z. H. (2017). Planning for a sustainable desert city: the potential water buffering capacity of urban green infrastructure. Landsc. Urban Plan. 167, 339–347. doi: 10.1016/j.landurbplan.2017.07.014
Keywords: urban water management, green infrastructure (GI), urban planning, border cities, land suitability analysis, hydrological modeling, cross-border cooperation
Citation: Lara-Valencia F, Garcia M, Norman LM, Anides Morales A and Castellanos-Rubio EE (2022) Integrating Urban Planning and Water Management Through Green Infrastructure in the United States-Mexico Border. Front. Water 4:782922. doi: 10.3389/frwa.2022.782922
Received: 25 September 2021; Accepted: 05 January 2022;
Published: 01 February 2022.
Edited by:
Sara Hughes, University of Michigan, United StatesReviewed by:
Anna Murveit, University of Arizona, United StatesJinsung Cho, California State Polytechnic University, Pomona, United States
Copyright © 2022 Lara-Valencia, Garcia, Norman, Anides Morales and Castellanos-Rubio. This is an open-access article distributed under the terms of the Creative Commons Attribution License (CC BY). The use, distribution or reproduction in other forums is permitted, provided the original author(s) and the copyright owner(s) are credited and that the original publication in this journal is cited, in accordance with accepted academic practice. No use, distribution or reproduction is permitted which does not comply with these terms.
*Correspondence: Margaret Garcia, M.Garcia@asu.edu