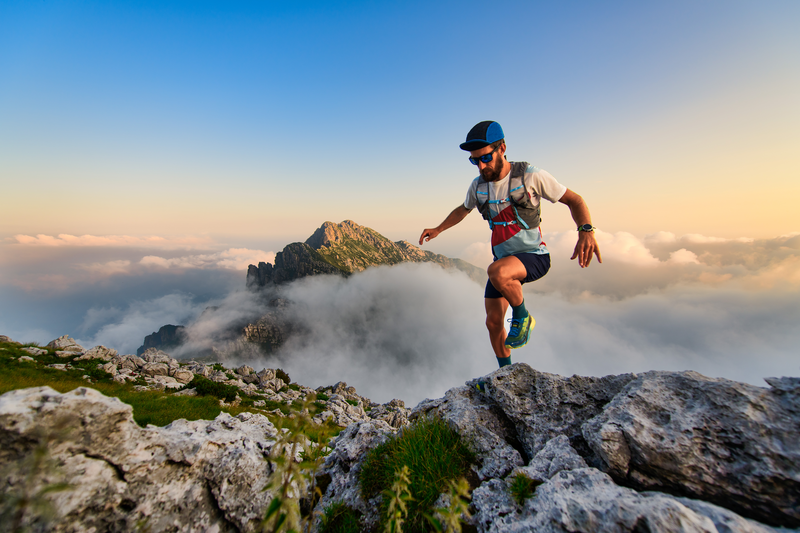
94% of researchers rate our articles as excellent or good
Learn more about the work of our research integrity team to safeguard the quality of each article we publish.
Find out more
ORIGINAL RESEARCH article
Front. Water , 04 November 2021
Sec. Water and Human Systems
Volume 3 - 2021 | https://doi.org/10.3389/frwa.2021.763358
This article is part of the Research Topic Flood Risk Perception, Vulnerability, and Risk: From Assessments to Analyses View all 6 articles
Louisiana, U.S.A., is among the world's most vulnerable places to coastal flooding. Increasing frequency/intensity of natural hazards under climate change scenarios is expected to exacerbate Louisiana's coastal flood risk. Although many factors are involved, land subsidence from marshland compaction and underground resource extraction, shoreline erosion accelerated by eustatic sea level rise (ESLR), and tropical-cyclone-induced storm surge are among the most important. While past research has focused on flood risk assessment and mitigation strategies, including climate change scenarios, few studies examine all of these factors collectively. This study shows present pluvial flood depth and the contributions of additional coastal subsidence and ESLR toward future (2050) pluvial flooding. Then, current tropical-storm-induced storm surge and future storm surge depth modeled by Louisiana's Coastal Protection and Restoration Authority (CPRA) under climate change scenarios are presented. Present and future 100-year pluvial flood and storm surge losses (separately, 2020$) to structures and their contents are estimated at the individual building level for Grand Isle, Louisiana, a barrier island town of ecological, economic, historical, recreational, cultural, and aesthetic treasure. Results suggest that the average 100-year pluvial flood depth in buildings will increase by 1.35 feet by 2050, with subsidence contributing over 82% of this total. Subsidence is projected to escalate structure and content losses by ~17% above losses in 2017, while ESLR may contribute ~3% above 2017 losses. A 100-year tropical-cyclone-induced storm surge event amid a “low” scenario of environmental change as defined by CPRA would increase Grand Isle's structure and content losses by 68–74% above the 2017 value by 2027, 141–149% by 2042, and 346–359% by 2067. The (“high”) scenario of environmental change would increase the 100-year storm surge losses by 85–91% above the 2017 value by 2027, 199–218% by 2042, and 407–415% by 2067. Outcomes from this study will offer a more realistic risk assessment model and will direct flood risk managers, property owners, and other stakeholders to build a comprehensive framework to minimize future flood risk in one of the most vulnerable sites in the U.S.A. to coastal flooding.
Flooding is an increasingly prevalent hazard and risk to coastal property and crops worldwide, including in Louisiana, U.S.A. Carter et al. (2018) suggested that by 2050, extreme coastal flood events will increase both in frequency and duration in the U.S.A. Louisiana's coastal flooding problem is exacerbated by high tides, frictionally-forced Gulf of Mexico currents, and unfavorable basin bathymetry, in an environment where river sediment nourishment is minimized due to the presence of levees. Although these and other factors intensify this hazard in Louisiana, land subsidence from compaction of marshlands, tectonism, isostasy (González and Törnqvist, 2006) and underground resource extraction; shoreline erosion accelerated by eustatic sea level rise (ESLR); and tropical-cyclone-induced storm surge are three of the most important (Yang et al., 2014). The broad-scale atmospheric circulation favorable for flooding in the U.S.A. has been described in detail (e.g., Junker et al., 1999; Shepherd et al., 2011; Warner et al., 2012; Lavers and Villarini, 2013; Wang et al., 2016), and tidal or nuisance flooding has recently received attention (Sheridan et al., 2017; Vega et al., 2020). Evidence suggests that flood frequency will increase markedly in the future and that, collectively, nuisance flooding events are likely to rival or exceed damage due to catastrophic flooding (Jacobs et al., 2018). Moreover, high-resolution modeling for the conterminous U.S.A. has uncovered that past and future fluvial and pluvial (i.e., rainfall-caused) flooding leaves increasingly more of the nation, including Louisiana, in flood hazard zones than previously realized, even without considering subsidence, ESLR, and storm surge (Wing et al., 2018). Yet a comprehensive analysis of Louisiana susceptibility to loss due to coastal flooding is lacking. This is unfortunate because, unlike so many other hazards and risks in coastal Louisiana (e.g., Mostafiz et al., 2020a,b), the predictability of flooding and its effects is relatively straightforward and could result in substantial mitigation to the hazard through inexpensive improvements in planning and forecasting.
This research represents a comprehensive approach to assessing coastal flood loss that considers the pluvial (i.e., locally-generated, rainfall-induced) flood-related variables of coastal subsidence and ESLR, and the tropical-cyclone-induced storm surge flooding, for the present and future under environmental change scenarios which include the effects of subsidence and ESLR. In this analysis, the concept of the 100-year flood event (or flood with a 100-year recurrence interval), which is defined by United States Geological Survey (United States Geological Survey, 2021a) as a flood of such magnitude that it has a 1% annual chance of being equaled or exceeded, is considered. The binomial theorem holds that, for example, a home in the 100-year floodplain has a 26% chance of being flooded at least once during a 30-year mortgage (United States Geological Survey, 2021b) and a 50% chance of being flooded during the 70-year useful life cycle of a building. This definition of 100-year flood may apply to pluvial or storm surge-generated causes. The specific objective is to evaluate current and future flood loss (due to 100-year pluvial, and in a separate analysis, 100-year storm surge) to building structures and their contents at the individual building level in the town of Grand Isle, Louisiana (Figure 1), one of the most vulnerable areas in the world to coastal flooding.
Figure 1. Grand Isle (town and barrier island), Louisiana, with dots representing building locations.
Subsidence is a major cause of the increased flood hazard in Louisiana since the 1920s, when the backswamps of the Mississippi River delta were drained and levees were built in response to the Great Mississippi Flood of 1927 (Davis, 1998). When these effects are combined with those of construction of upstream dams that trap ~50% of the sediment load (Blum and Roberts, 2009, 2012), the coastal wetlands are robbed of the sediments needed to balance the erosive power of tides and storms. Additionally, compaction and/or consolidation of Holocene sediments exacerbated by human activities contribute substantially to the problem (Dokka, 2006; Törnqvist et al., 2008) at least in some localized areas (Meckel et al., 2006). Hydrocarbon extraction is another cause of subsidence in southern Louisiana (Voyiadjis and Zhou, 2018; Zhou and Voyiadjis, 2019), but it may be a far smaller contributor than the geological factors (Olea and Coleman, 2014), especially those related to local tectonics (Dokka, 2011) and downwarping of the geosyncline on the Gulf of Mexico coast by sediments (Blum et al., 2008; Byrnes et al., 2019). Issues related to surface water drainage and management also contribute to Louisiana's subsidence problem (Jones et al., 2016), though some have questioned the significance of this factor (Olea and Coleman, 2014; Turner, 2014). Aside from the obvious effect of increased vulnerability to coastal flood hazards, subsidence along the Louisiana coast has also been shown to be detrimental to ecosystem health (e.g., Yuill et al., 2009).
Subsidence rates in coastal Louisiana have been measured accurately in recent years using high-precision instruments, but because of wide spatial variation and differences in the techniques used, rate estimates are difficult to generalize (Mallman and Zoback, 2007). For example, Shinkle and Dokka (2004) found rates as high as 52 mm year−1. Dixon et al. (2006) measured rates up to 28 mm year−1 in New Orleans and noted that rapid subsidence in the 3 years prior to Hurricane Katrina may have played a role in some levee failures during Katrina. Yet Dokka et al. (2006) reported only 7 mm year−1 atop an active allochthon in southeastern Louisiana, and Karegar et al. (2015) approximated 5–6 mm year−1 in the southern Mississippi River delta. Reed and Yuill (2016) mapped the Mississippi delta region of southeast Louisiana, showing ranges of 2–35 mm year−1. Nienhuis et al. (2017) found much more uniform subsidence rates across coastal Louisiana, with a mean of 9 mm year−1 and a standard error of the mean of only 1 mm year−1; however, they cautioned that the uncertainty in the estimations must be considered. Byrnes et al. (2019) reported that values range from 2 to 7 mm year−1 at 21 locations in the Barataria Basin of southeastern Louisiana. Harris et al. (2020) estimated rates from <1 to 16 mm year−1 in the Lower Mississippi River Industrial Corridor. Overlapping results and the rationality of substantial spatial variability and uncertainty suggest that the results noted above may all be within an acceptable range of accuracy for the areas considered, and Lane et al. (2020) offered a wide range of 17–125 mm year−1 in southeastern Louisiana uncompacted marshes. Zou et al. (2016) found that subsidence will cause the below-sea-level areas in the lower Mississippi River basin to increase from about 4% in 2004 to 31% by 2050.
The most comprehensive recent study on ESLR is by Oppenheimer et al. (2019), as part of the Intergovernmental Panel on Climate Change (IPCC), who reported a rise of 1–2 mm year−1 in most regions through the twentieth century, with current rates of 3–4 mm year−1. Predictions of future ESLR hinge on the extent to which societal changes may mitigate or increase the warming (Bushra et al., 2021). Intergovernmental Panel on Climate Change (2021) defines several “representative concentration pathways” (RCPs) scenarios, named for the number of Watts per square meter of additional radiative forcing that is assumed to occur in that scenario. For example, the most “optimistic” (i.e., “low environmental change”) scenario, RCP2.6, is based on the assumption that bioenergy combined with carbon capture/storage increasingly replaces fossil fuel combustion through the twenty-first century, leading to an increase in atmospheric CO2 to 490 parts per million (ppm) (van Vuuren et al., 2011), compared to 419 ppm today. Such a scenario would cause ESLR to increase to 4–9 mm year−1 by 2100 (Oppenheimer et al., 2019). By contrast, the most drastic (i.e., “high environmental change”) scenario considered by IPCC (RCP8.5) would produce atmospheric CO2 concentrations of 1,370 ppm by 2100 (van Vuuren et al., 2011) and generate ESLR of 10–20 mm year−1 by century's end (Oppenheimer et al., 2019). Predictions to 2050 can be made with a higher degree of confidence, with ESLR likely between 17 and 32 cm under RCP2.6 and 23–40 cm under RCP8.5 by 2050, with the latter being reported with a “medium” degree of confidence (Oppenheimer et al., 2019).
Local sea level may depart from ESLR for a variety of geophysical reasons. An early study concluded that Louisiana exhibited the highest sea level rise (SLR) rate along the U.S. Gulf of Mexico coast, with values of ~10 (at Grand Isle) to 12 mm year−1 (at Eugene Island, Louisiana) from 1946 to 1988 (Penland and Ramsey, 1990). Jankowski et al. (2017) approximated recent SLR rates on the Louisiana coast at 4–20 mm year−1. National Oceanic and Atmospheric Administration (National Oceanic Atmospheric Administration, 2021a) reported a trend at Grand Isle of 9.16 mm year−1 with a 95% confidence interval of ±0.4 mm year−1. Again, the correspondence between these results may suggest that all are plausible. The combined effect of ESLR and subsidence at a local point is often referred to as relative sea level rise (RSLR). Historical RSLR has been estimated for Grand Isle (Figure 2).
Figure 2. Historical relative sea level at Grand Isle, Louisiana. Source: National Oceanic Atmospheric Administration (2021a).
Along with the two components of RSLR, the third major consideration in this research is tropical cyclone-induced storm surge. Current 100-year storm surge depth for Grand Isle is shown in Figure 3. Not surprisingly, with the increase in sea level and coastal flooding comes an increase in the effect of storm surge by 2050. Storm surge amid ESLR in the U.S. Southeast may cost up to $60 billion/year by 2050 (Carter et al., 2018).
Figure 3. Current modeled 100-year tropical storm-induced storm surge inundation depths (feet) for Grand Isle, Louisiana. Source: Coastal Protection Restoration Authority (2017).
Modeling of storm surge for Louisiana began over 40 years ago with the Sea, Lake, and Overland Surges from Hurricanes (SLOSH; Crawford, 1979) model. Other one- and two-dimensional models were used to assess the impact of current on the storm surge on the Louisiana-Texas continental shelf (Signorini et al., 1992). Hurricane Katrina (2005) stimulated additional work on storm surge in Louisiana (e.g., Fritz et al., 2007; Dietrich et al., 2010). Developed in the early 1990s (Luettich et al., 1992), the Advanced Circulation (ADCIRC) model became an increasingly important tool for modeling storm surge in Louisiana's coastal zone (e.g., Westerink et al., 2008; Smith et al., 2010). ADCIRC was also coupled with other models (Bunya et al., 2010) to describe hurricane evolution in improved detail (Dietrich et al., 2010). Using ADCIRC to simulate storm surge near the Mississippi River mouth, Kerr et al. (2013) suggested that water levels in this area are only slightly dependent on streamflow. Building on previous research that modeled the potential of coastal wetlands for reducing storm surge (Wamsley et al., 2010), Barbier et al. (2013) calculated the economic value of southeast Louisiana wetlands for storm surge mitigation. Siverd et al. (2019) predicted increases of storm surge from 2010 to 2110 of 55–75 cm, with future changes dominated by RSLR effects, unlike historical storm surges that increased primarily due to local land and river management decisions. Most recently, Camelo et al. (2020) projected up to a 25% increase in wetted area due to tropical-cyclone-induced storm surge for the Gulf of Mexico and Atlantic coasts, including Louisiana, under climate change scenarios by 2100.
Impacts of storm surge on various aspects of the Louisiana natural and human environments have also been analyzed. For example, building structural damage (Robertson et al., 2007), agricultural pests (Beuzelin et al., 2009), power outages (Tonn et al., 2016), and bird nesting (Bolinger et al., 2018) have all been shown to be impacted negatively by storm surge. Furthermore, storm surge deposition in Louisiana has been used to reconstruct pre-historic storm surges (e.g., Williams and Flanagan, 2009; Liu et al., 2014) and marsh aggradation processes (Williams, 2012). The response of storm surge to sea level changes (Smith et al., 2010; Bilskie et al., 2014) and barrier island/marsh restoration (Wamsley et al., 2009; Grzegorzewski et al., 2011) have also been topics of recent scholarly interest.
Changes in precipitation are a potential factor that could influence future floods. However, for this study region, the literature does not suggest drastic changes in precipitation by 2050. More specifically, Ingram et al. (2013) noted that the North American Regional Climate Change Assessment Program (NARCCAP) identified precipitation projections for Louisiana that will remain similar for 2014–2070 as compared to 1971–2000. Hausfather (2018) provided multimodel averages from the Coupled Model Intercomparison Project version 5 (CMIP5) that show that, unlike most other areas of the world, Louisiana can expect only a small difference in total precipitation for 1981–2000 vs. 2081–2100, even for the RCP8.5 scenario. Kadaba (2018) suggested that by 2050, annual precipitation in Jefferson Parish (i.e., county), which contains Grand Isle, will increase by only 16 mm, as compared to baseline values from 1970 to 2000. Thus, this study does not consider changes to precipitation by 2050. However, it is noteworthy that extreme precipitation events that cause floods may increase in frequency and/or magnitude (Lall et al., 2018), as weakened steering circulation (He et al., 2014) would cause storms to remain overhead for a longer period of time. It is also important to note that compound inundation models (e.g., Santiago-Collazo et al., 2019) have been implemented to consider the effects of two or more factors in generating floods.
Grand Isle is a town that sits on both the mainland and the only inhabited barrier island in Louisiana (also named Grand Isle, see again Figure 1). Similar to other barrier islands, Grand Isle was formed as waves and the longshore current deposited sediments in a linear fashion, in this case ~5,000 years ago as sediment from the Mississippi River was carried westward. Like most of the rest of the U.S. Gulf of Mexico coast, Grand Isle has a humid subtropical climate. Mean daily maximum temperatures in July hover near 89°F, while mean daily minimum temperatures in January are near 45°F; killing frosts are rare but do occur (Vega et al., 2013). Although precipitation is abundant year-round with a mean annual total of 61 inches, minor hydrologic deficits are common in summer owing to high evapotranspiration rates, and droughts do occur (Vega et al., 2013).
Grand Isle is selected as the study area because it is particularly vulnerable to the effects of subsidence, sea level rise, and storm surge. As a popular fishing, resort, and mineral extraction area, it is well-known by many sectors of society, and therefore, the findings here would be of broad interest in both the scientific and popular sectors of society. Grand Isle is also one of the few areas where present and projected future 100-year storm surge and current 100-year pluvial flood data are available along with the subsidence rate, ESLR, and NOAA sea level rise inundation depth scenarios. A final reason for selecting this study area is that no previous study has focused on present and future flood damage to building structure and contents in Grand Isle while considering the effects of subsidence, ESLR, and tropical cyclone-related storm surge.
The U.S. Army Corps of Engineers (USACE) Hydrologic Engineering Center's Flood Inventory Analysis (HEC-FIA; Dunn, 2000) model is used to calculate the loss from the current 100-year pluvial flood event and (separately) 100-year storm surge event, and to project loss resulting from a future (i.e., in 2050) 100-year flood due to RSLR and (separately) a storm surge event 10, 25, and 50 years after the 2017 baseline. Calculation of the loss in HEC-FIA requires a building inventory for representing building characteristics such as replacement cost, number of stories, and foundation height; a digital elevation model (DEM) for identifying building elevation; and a pluvial flood and (separately) storm surge inundation depth grid of the study area. The default depth-damage function from the HEC-FIA model is employed here to express loss as a function of flood inundation depth by building.
The building inventory used here is the National Structure Inventory (NSI) (2019) 2.0, a building attribute dataset of every major structure, such as homes, commercial establishments, and educational buildings, in the conterminous United States. NSI 2.0 was developed from many sources, including Hazus (Schneider and Schauer, 2006), USACE, USGS, National Center for Education Statistics, U.S. Census Bureau, Microsoft, Esri, and Homeland Infrastructure Foundation-Level Data (Barnett, 2010). Availability of NSI 2.0 is typically limited to approved federal government applications and academic work such as this research. Attributes in NSI 2.0 used in this research include: structure value (i.e., replacement cost), building content value, number of stories, occupancy type (i.e., residential vs. commercial vs. industrial), foundation type (e.g., slab, pier on beam, basement), and foundation height.
The DEM is downloaded from National Oceanic Atmospheric Administration (2021b), where it was compiled from among the following Lidar data sets: 2017 Upper Delta Plain LA Lidar; 2015 South Terrebonne Lidar; 2013 USGS Louisiana Barataria Lidar; 2013 USGS/NPS Jean Lafitte Lidar; and 2011 USGS Louisiana Coastal Lidar Project (ARRA Region 2). The DEM is referenced vertically to the North American Vertical Datum of 1988 (NAVD88, Geoid12B) and horizontally to the North American Datum of 1983 (NAD83), with vertical units of meters and spatial resolution of ~3 m (National Oceanic Atmospheric Administration, 2021b).
To ascertain the present impact of flooding on building structure and content losses, the 100-year return period pluvial flood depth grid for Jefferson Parish, Louisiana, was developed at a scale of 3.048 × 3.048 m by FEMA through its Risk Mapping, Assessment and Planning (Risk MAP) program (FEMA, 2021). While the complexities associated with Risk MAP products have been identified (Johnson and Mahoney, 2011), these model-output data represent the best available input for understanding the flood risk. In Risk MAP, FEMA determined flood hazard areas using statistical analyses of records of river flow, storm tides, and rainfall; information obtained through consultation with the communities; floodplain topographic surveys; and hydrological and hydraulic analysis. However, because subsidence and ESLR were not considered in FEMA's Risk MAP program and because these are two important considerations regarding Grand Isle's future flood hazard, spatially-distributed subsidence rates from Zou et al. (2016) and inundation depths caused by ESLR from National Oceanic Atmospheric Administration (2021b) are collected for calculating the structure and content losses due to future flooding.
To calculate the losses, present and future 100-year storm surge data from Louisiana's Coastal Protection Restoration Authority (2017) are used. Coastal Protection Restoration Authority (2017) assesses changes involved in a 50-, 100-, and 500-year storm surge event 10 (i.e., 2027), 25 (i.e., 2042), and 50 (i.e., 2067) years after the 2017 baseline. This is done by including tropical cyclones of different storm intensities, areas, and landfall locations in and near Louisiana, including the different environmental change scenarios described in Sea Level Rise section, both with and without implementation of the protection and restoration actions. Specifically, CPRA provides spatially-distributed 100-year storm surge depth grids along Louisiana's coast, for the present and for 10, 25, and 50 years into the future. Each of these projections includes six depths: one each for low, medium, and high scenarios of environmental change (i.e., warming and sea level rise), with and without implementation of mitigation measures described in the Louisiana Coastal Master Plan. Output data from Grand Isle are used here. A summary table of data sources is shown in Table 1.
Given that local precipitation change at Grand Isle is expected to be negligible, localized future flood depths are governed by two major local factors: changes in pluvial floods due to subsidence and ESLR, and tropical cyclone-dominated changes in storm surge.
For pluvial changes, the current 100-year flood depth at Grand Isle from the Jefferson Parish Risk MAP program flood depth grids is used as the baseline for predicting future flood depths due to these two categories of factors. The mean subsidence rate (mm year−1) raster files generated by Zou et al. (2016) for coastal Louisiana are used to project the total subsidence from 2017 (i.e., when the flood depth grid was calculated) to 2050. Then, the Grand Isle elevation in 2050 is computed as the value in National Oceanic Atmospheric Administration (2021b) minus this total subsidence. The decrease in elevation is assumed to be linear over time and will increase the flood depth for buildings in Grand Isle by 2050 at a mean of 1.11 feet, but ranging from 0.63 to 1.25 feet.
The ESLR trend of 9.16 mm year−1 at Grand Isle as measured by National Oceanic Atmospheric Administration (2021a) is extrapolated linearly from 2017 (i.e., when the flood depth grid was calculated) for 33 years, to project a ESLR of 302 mm (or ~one foot) by 2050. The flood depth increase attributed to this ESLR increase is calculated using the National Oceanic Atmospheric Administration (2021b) “sea level rise depth” option for a zero (i.e., for present) and a one-foot (2050 scenario) sea level rise. The inundation depth accompanying this one-foot increase is calculated as the difference between the zero (for present) and one-foot (for 2050) inundation depth. Consequently, the building 100-year flood depth in 2050, considering the effect of ESLR only, is calculated as the sum of the present 100-year flood depth and the increase in inundation depth attributed to ESLR by 2050.
For both present and future 100-year storm surge at Grand Isle, the Coastal Protection Restoration Authority (2017) storm surge inundation depth shapefiles are converted to raster files using the “Polygon to Raster” tool in ArcGIS®. All combinations of scenarios are run (i.e., current 100-year storm surge depth, storm surge depth 10 years in the future without action for a low environmental change scenario, 25-year future storm surge without action for a high environmental change scenario,. 50-year future storm surface with action for a high environmental change scenario). The present and future 100-year storm surge scenarios are used to calculate the structure and content losses in HEC-FIA to determine the economic impact of a future 100-year storm surge in Grand Isle.
HEC-FIA is the best model for this analysis because its method is to estimate quantitatively flood consequences with uncertainty (Lehman and Light, 2016), and because it partitions direct and indirect economic losses (only the former are of interest here) geospatially while allowing incorporation of externally-derived flood depth grids (Lehman et al., 2014) which are essential for this analysis. HEC-FIA is also advantageous because it is compatible with other USACE software, including River Analysis System (HEC-RAS) for geospatial analysis of losses from fluvial flooding (Dunn et al., 2016), making it useful in future riverine flood research while providing the same basis for comparison of results from this coastal flooding analysis. A more comprehensive review of the useful features of HEC-FIA for flood damage assessment is provided in Nafari (2013).
HEC-FIA information flow is depicted in Figure 4. The layer files (i.e., study area boundary and NSI 2.0 data for Grand Isle) are first converted into shapefiles. Likewise, all raster files (i.e., DEM, 100-year flood depth grids, and 100-year storm surge depth grids) are converted into Tag Image File Format (TIFF or TIF), and all shapefiles and raster files are projected to the same geographic projection system (WGS 1984 UTM Zone 15N).
The method involved in structure and content loss calculations follows that of Mostafiz et al. (2021). Specifically, after the study area and NSI 2.0 shapefiles are added in the “map layers” folder, the DEM data are included by selecting “add terrain model” in the “terrain grids” folder, and a new “watershed configurations” is created using the terrain grid (i.e., DEM). Then, the study area is “imported” in the “boundaries” and “impacted areas” folders separately. Next, 100-year flood and storm surge depths (present and future) are configured separately, by selecting “new” in the “inundation data” folder, “grid only” under “hydraulic data type,” “inundation grid” under “grid only,” and the watershed configuration (i.e., DEM) created earlier. Then “define event” is selected separately for each scenario. The next step is to import the depth damage functions (DDFs) using the “import from default” option in the “structure occ. types” folder. Next, “structure inventories” is selected, along with “generate from point shapefile” (i.e., NSI 2.0), and “use terrain+foundation height” under the “first floor elevation source” header. Then, the appropriate mandatory “shapefile fields” added from NSI 2.0 are uploaded into the “structure ID,” “damage category,” “occupancy type,” and “replacement value” inventory fields. The following optional inventory fields are then uploaded: “content value,” “foundation height,” “foundation type,” and “number of stories,” along with their corresponding shapefile fields.
To run the final loss calculation, the “new” option is selected within the “alternatives” folder for each scenario. The study area is clicked under “impact area set,” the appropriate inundation depth (e.g., 100-year flood for present, 100-year storm surge depth for 10 years in the future, etc.) is chosen under “inundation configuration,” and NSI 2.0 is selected under “structure inventory.” Then, “new” is clicked from the “simulation” folder, and the appropriate flood return period is selected in the “alternative” and “event” fields. Finally, the “compute” option is selected by clicking on the name given to the simulation in the previous step. The results show the aggregate and individual building damage reports, including both structure and content separately, for the selected study area.
Computation of losses using the Monte Carlo technique with inclusion of uncertainty only yields slight differences from that without uncertainty. The results without uncertainty are presented here.
A 100-year pluvial flood event in 2017 would produce over $169 million (2020$) in structure and content losses (Figure 5) to the 2,023 structures on Grand Isle, of which 1,897 are residential, 79 are commercial, 21 are industrial, and 26 are public [(National Structure Inventory (NSI), 2019)]. The mean 100-year pluvial flood depth for all structures on Grand Isle today is 8.95 feet above the ground surfaces, which explains why the structures are all elevated (Supplementary Figure 7). By 2050, 1.11 feet of additional depth is projected to have been caused by continued subsidence (Supplementary Figure 8) and another 0.24 feet of depth is projected to be due to ESLR (Supplementary Figure 9), leaving the projected mean 100-year pluvial flood depth for these structures at 10.30 feet (Supplementary Figure 10). Thus, continued subsidence and ESLR will increase the 2017 pluvial flood depth by 12.4 and 2.7%, respectively, by 2050. Continued subsidence to 2050 is projected to increase these losses to over $198 million (2020$), a 17% increase (Supplementary Appendix A), and ESLR would add another 3.1% to these losses by 2050 (Supplementary Appendix B). In both cases, losses to structure are and will likely remain greater than losses to content (Supplementary Appendices A, B), and the combined total effect of continued subsidence and ESLR will cause over $203 million (2020$) in losses to these buildings by 2050 (Figure 5; Supplementary Appendix C). Nearly 77% of these total (structure + content) losses would be to residential structures (Figure 5; Supplementary Appendix D).
Figure 5. Present (2017) and 2050-projected total (structure + content) losses (2020$) for all buildings and for only residential buildings, from 100-year pluvial flood event due to the effects of continued subsidence and eustatic sea level rise, Grand Isle, Louisiana.
Regarding tropical-cyclone-induced storm surge flooding, the 100-year event that occurs 10, 25, and 50 years into the future will cause greatly magnified total (structure + content) losses at Grand Isle compared to today's loss estimates for all buildings of $59 million (2020$) for structure and content (Figure 6). Interestingly, because the Louisiana Coastal Master Plan (Coastal Protection Restoration Authority, 2017) prioritizes rebuilding land elsewhere, the losses are estimated to be slightly greater at Grand Isle with implementing the Plan compared to the scenario without Plan implementation (Figure 6). The “low” scenarios of environmental change would increase the storm-surge-induced flood losses at Grand Isle by 68–74% in 10 years, 141–149% in 25 years, and 346–359% in 50 years (Figure 6; Supplementary Appendix E). Even more menacingly, “high” scenarios of environmental change are expected to increase the losses by ~85–91% in 10 years, 199–218% in 25 years, and 407–415% in 50 years (Figure 6; Supplementary Appendix E).
Figure 6. Total (structure + content) losses (2020$) for a 100-year storm surge flood event in Grand Isle, Louisiana, with vs. without implementation of the 2017 Louisiana Coastal Master Plan (CMP), and by low, medium, and high scenarios of environmental change, for 2017, 2027, 2042, and 2067.
The nearly $34 million (2020$) in additional damage to all buildings from a 100-year pluvial flood event that can be expected because of the continuing effects of subsidence and ESLR between 2017 and 2050 (Supplementary Appendix C) would represent an extra $23,770 (2020$) for each of the 1,429 permanent residents of Grand Isle in 2017 (United States Census Bureau, 2021). Thus, the total losses to all buildings are projected at an astounding $142,132 per person (not per household). The 2050 losses from an event of the same magnitude solely to the residential buildings would leave each person (not each household) with $108,922 in damage (Supplementary Appendix D).
An even more sobering story emerges when examining the impact of tropical-cyclone-induced storm surge. In the least severe environmental change scenario with and without implementation of the Louisiana Coastal Master Plan (Coastal Protection Restoration Authority, 2017), a storm surge in 50 years (i.e., 2067) would inflict $187,786 and $185,133, respectively, per person in losses. Even more ominously, the most severe environmental change scenario with and without implementing the Louisiana Coastal Master Plan Coastal Protection Restoration Authority (2017) would impose losses of $211,443 and $209,646 per person in 2067 (Supplementary Appendix E).
The fact that losses would likely be slightly greater without implementation should certainly not be taken as evidence that Louisiana's Coastal Master Plan (Coastal Protection Restoration Authority, 2017) should not be implemented. Instead, the direction of resources elsewhere for fortifying Louisiana's coastline in Coastal Protection Restoration Authority (2017) may simply be evidence of a triage-based approach to prioritize the areas with the greatest impact per dollar spent. Regardless, however, the overwhelming sentiment is that Grand Isle is worth saving, but this will require individual homeowners to elevate their homes and camps under the assumption that the CPRA Coastal Master Plan will provide little additional mitigating effects for Grand Isle.
According to the binomial distribution, the probability of a 100-year flood occurring in a 33-year period (e.g., between 2017 and 2050) is 28.2%, and the 50-years-in-the-future-from-2017 probability of the 100-year storm surge flood is 39.5%. Bohn (2013) provides a comprehensive set of possible mitigation measures to circumvent such an undesirable outcome. In all cases for both the 100-year pluvial flood and the 100-year storm surge, damage to structures exceeds damages to the contents within the structure (Supplementary Appendices A–E).
Even more daunting is the fact that these losses do not include those incurred to vehicles including boats, ecosystem structures and functions, recreation, industries (e.g., commercial fisheries, tourism, and mineral extraction), indirect losses (e.g., hotel expenses while a residence is being remodeled, work absences, and emotional/psychological anguish), and the priceless impacts to aesthetic resources for which Grand Isle is renowned. Nor does it include additional damage to structures, contents, or the items listed above from wind or lightning that accompanies the modeled flood-producing storms. Furthermore, it is noteworthy that the losses calculated herein assume no increase in precipitation, even though several sources suggest an increasing frequency of heavy precipitation events, as described previously. Thus, our calculations here likely underestimate the losses.
This analysis estimates loss to building structure and contents from a 100-year pluvial flood event and a 100-year tropical cyclone-induced storm surge event at the iconic resort area of Grand Isle, Louisiana, for the present and for future scenarios. The 100-year pluvial flood event certainly produces formidable flood depths at Grand Isle today—an average of almost 9 feet. However, continued subsidence and ESLR will increase this value by 12.4 and 2.7%, respectively, causing over $203 million (2020$) in structure and content damage by 2050, an increase of 20% over calculations for the 100-year pluvial flood in 2017. While the 100-year storm-surge flood causes ~$58,878,948 in structure and content damage today for all buildings in Grand Isle, future increases in flood depths caused by low, medium, and high scenarios of environmental change (Coastal Protection Restoration Authority, 2017) may produce losses 50 years into the future (i.e., 2067) of between $265 and $300 million. Because mitigation measures are targeted for areas other than Grand Isle, the losses are slightly greater with implementation as compared to without implementation of Coastal Protection Restoration Authority (2017). Thus, individual homeowners must elevate their homes and camps under the assumption that the CPRA Coastal Master Plan will provide little additional mitigating effects for Grand Isle. In all cases for both the 100-year pluvial flood and the 100-year storm surge, damage to structures exceeds damage to the contents within the structures. Municipal and parish (county) officials must make all practical efforts to mitigate these losses through effective planning and cooperation to enhance community resilience to the coastal flood hazard. Otherwise, Grand Isle may be destined to a similar fate as another famous Louisiana barrier island resort destination—Isle Dernière—where ~200 lost their lives when the island was destroyed and broken into multiple islands by a hurricane in 1856, with its subsequently unpopulated remnants renamed as Isles Dernières.
Similar methods could be used to assess potential risk in any coastal area for which sophisticated flood modeling has been done. Results from such analyses would assist community leaders in planning for the ever-present coastal flood hazard and in educating the public regarding the importance of proactive programs to mitigate the hazard. Such efforts would improve the quality of life and enhance resilience in the densely populated and frequently visited coastal zone.
While NSI 2.0 data are generally accessible with permission from USACE Hydrologic Engineering Center, other raw data supporting the conclusions of this article will be made available by the authors, without undue reservation.
RM developed the methodology, collected and analyzed the data, and developed the initial text. NB provided oversight on the numerical analysis. RR edited early and late drafts of the text. CF provided suggestions on the methodology and edited the text. MR assisted in the loss analysis. All authors contributed to the article and approved the submitted version.
This project was funded by the Louisiana Sea Grant College Program (LSG; Omnibus cycle 2020–2022, Award Number NA18OAR4170098, Project Number R/CH-03), and the U.S. Department of Housing and Urban Development (HUD; 2019–2022, Award Number H21679CA, Subaward Number S01227-1). Publication of this article was subsidized by the Louisiana State University (LSU) Libraries Open Access Author Fund and the Performance Contractors Professorship in the College of Engineering at LSU.
Any opinions, findings, conclusions, and recommendations expressed in this manuscript are those of the authors and do not necessarily reflect the views of LSG or HUD.
The authors declare that the research was conducted in the absence of any commercial or financial relationships that could be construed as a potential conflict of interest.
All claims expressed in this article are solely those of the authors and do not necessarily represent those of their affiliated organizations, or those of the publisher, the editors and the reviewers. Any product that may be evaluated in this article, or claim that may be made by its manufacturer, is not guaranteed or endorsed by the publisher.
The authors warmly appreciate the sharing of subsidence data by Lei Zou and the assistance and support of David Dunaway of LSU Libraries for assistance in acquiring reference materials.
The Supplementary Material for this article can be found online at: https://www.frontiersin.org/articles/10.3389/frwa.2021.763358/full#supplementary-material
Barbier, E. B., Georgiou, I. Y., Enchelmeyer, B., and Reed, D. J. (2013). The value of wetlands in protecting southeast Louisiana from hurricane storm surges. PLoS ONE 8:e58715. doi: 10.1371/journal.pone.0058715
Barnett Jr, J. A. (2010). Homeland Infrastructure Foundation-Level Data Working Group Bi-Monthly Meeting: Remarks of James Arden Barnett, Jr., Chief, Public Safety and Homeland Security Bureau, Federal Communications Commission, November 1, 2010, Washington DC. Available online at: https://www.hsdl.org/?abstractanddid=14184 (accessed April 8, 2021).
Beuzelin, J. M., Reagan, T. E., Akbar, W., Cormier, H. J., Flanagan, J. W., and Blouin, D. C. (2009). Impact of Hurricane Rita storm surge on sugarcane borer (Lepidoptera: Crambidae) management in Louisiana. J. Econ. Entomol. 102, 1054–1061. doi: 10.1603/029.102.0325
Bilskie, M. V., Hagen, S. C., Medeiros, S. C., and Passeri, D. L. (2014). Dynamics of sea level rise and coastal flooding on a changing landscape. Geophys. Res. Lett. 41, 927–934. doi: 10.1002/2013GL058759
Blum, M. D., and Roberts, H. H. (2009). Drowning of the Mississippi Delta due to insufficient sediment supply and global sea-level rise. Nat. Geosci. 2, 488–491. doi: 10.1038/ngeo553
Blum, M. D., and Roberts, H. H. (2012). The Mississippi delta region: past, present, and future. Annu. Rev. Earth Planet. Sci. 40, 655–683. doi: 10.1146/annurev-earth-042711-105248
Blum, M. D., Tomkin, J. H., Purcell, A., and Lancaster, R. R. (2008). Ups and downs of the Mississippi Delta. Geology 36, 675–678. doi: 10.1130/G24728A.1
Bohn, F. H. (2013). Design Flood Elevations beyond Code Requirements and Current Best Practices. M.S. thesis, Louisiana State University. Available online at: https://digitalcommons.lsu.edu/cgi/viewcontent.cgi?article=1068andcontext=gradschool_theses (accessed September 8, 2021).
Bolinger, S., Averhart, M., Duke-Sylvester, S., Johnson, E., Ray, K., and Bolinger, S. (2018). Effects of depredation and storm surge on hatching success of least tern nests on a renourished southeast Louisiana beach. Integr. Comp. Biol. 58:E20 (meeting abstract). Available online at: https://sicb.burkclients.com/meetings/2018/schedule/abstractdetails.php?id=747 (accessed May 8, 2021).
Bunya, S., Dietrich, J. C., Westerink, J. J., Ebersole, B. A., Smith, J. M., Atkinson, J. H., et al. (2010). A high-resolution coupled riverine flow, tide, wind, wind wave, and storm surge model for southern Louisiana and Mississippi. Part I: Model development and validation. Mon. Weather Rev. 138, 345–377. doi: 10.1175/2009MWR2906.1
Bushra, N., Mostafiz, R. B., Rohli, R. V., Friedland, C. J., and Rahim, M. A. (2021). Technical and social approaches to study shoreline change of Kuakata, Bangladesh. Front. Mar. Sci. 8:730984. doi: 10.3389/fmars.2021.730984
Byrnes, M. R., Britsch, L. D., Berlinghoff, J. L., Johnson, R., and Khalil, S. (2019). Recent subsidence rates for Barataria Basin, Louisiana. Geo-Mar. Lett. 39, 265–278. doi: 10.1007/s00367-019-00573-3
Camelo, J., Mayo, T. L., and Gutmann, E. D. (2020). Projected climate change impacts on hurricane storm surge inundation in the coastal United States. Front. Built Environ. 6:588049. doi: 10.3389/fbuil.2020.588049
Carter, L., Terando, A., Dow, K., Hiers, K., Kunkel, K. E., Lascurain, A., et al. (2018). “Southeast,” in Impacts, Risks, and Adaptation in the United States: Fourth National Climate Assessment, Volume II, eds. D. R. Reidmiller, C. W. Avery, D. R. Easterling, K. E. Kunkel, K. L. M. Lewis, T. K. Maycock, et al. (Washington, DC: U.S. Global Change Research Program), 743–808. doi: 10.7930/NCA4.2018.CH19
Coastal Protection Restoration Authority (2017). Download Data—Coastal Master Plan GIS Data. Available online at: http://cims.coastal.louisiana.gov/masterplan/GISDownload/ (accessed September 8, 2021).
Crawford, K. C. (1979). Hurricane surge potentials over southeast Louisiana as revealed by a storm-surge forecast model: a preliminary study. Bull. Am. Meteorol. Soc. 60, 422–429. doi: 10.1175/1520-0477(1979)060<0422:HSPOSL>2.0.CO;2
Davis, J. M. (1998). Rising Tide: The Great Mississippi Flood of 1927 and How it Changed America. New York, NY: Touchstone.
Dietrich, J. C., Bunya, S., Westerink, J. J., Ebersole, B. A., Smith, J. M., Atkinson, J. H., et al. (2010). A high-resolution coupled riverine flow, tide, wind, wind wave, and storm surge model for southern Louisiana and Mississippi. Part II: Synoptic description and analysis of Hurricanes Katrina and Rita. Mon. Weather Rev. 138, 378–404. doi: 10.1175/2009MWR2907.1
Dixon, T. H., Amelung, F., Ferretti, A., Novali, F., Rocca, F., Dokka, R., et al. (2006). Subsidence and flooding in New Orleans. Nature 441, 587–588. doi: 10.1038/441587a
Dokka, R. K. (2006). Modern-day tectonic subsidence in coastal Louisiana. Geology 34, 281–284. doi: 10.1130/G22264.1
Dokka, R. K. (2011). The role of deep processes in late 20th century subsidence of New Orleans and coastal areas of southern Louisiana and Mississippi. J. Geophys. Res. 116:B06403. doi: 10.1029/2010JB008008
Dokka, R. K., Sella, G. F., and Dixon, T. H. (2006). Tectonic control of subsidence and southward displacement of southeast Louisiana with respect to stable North America. Geophys. Res. Lett. 33:L23308. https://doi.org/10.1029/2006GL027250doi: 10.1029/2006GL027250
Dunn, C., Baker, P., and Fleming, M. (2016). “Flood risk management with HEC-WAT and the FRA compute option,” in E3S Web of Conferences. EDP Sci. 7:11006. Available online at: https://www.e3s-conferences.org/articles/e3sconf/pdf/2016/02/e3sconf_flood2016_11006.pdf (accessed September 8, 2021).
Dunn, C. N. (2000). “Flood damage and damage reduction calculations using HEC's Flood Impact Analysis model (HEC-FIA),” in Joint Conference on Water Resource Engineering and Water Resources Planning and Management 2000, Minneapolis, MA. doi: 10.1061/40517(2000)180
FEMA (2021). Risk Mapping, Assessment and Planning (Risk MAP). Available online at: https://www.fema.gov/flood-maps/tools-resources/risk-map (accessed June 16, 2021).
Fritz, H. M., Blount, C., Sokoloski, R., Singleton, J., Fuggle, A., McAdoo, B. G., et al. (2007). Hurricane Katrina storm surge distribution and field observations on the Mississippi Barrier Islands. Estuar. Coast. Shelf Sci. 74, 12–20. doi: 10.1016/j.ecss.2007.03.015
González, J. L., and Törnqvist, T. E. (2006). Coastal Louisiana in crisis: subsidence or sea level rise? EOS Trans. Am. Geophys. Union 87, 493–508. doi: 10.1029/2006EO450001
Grzegorzewski, A. S., Cialone, M. A., and Wamsley, T. V. (2011). Interaction of barrier islands and storms: implications for flood risk reduction in Louisiana and Mississippi. J. Coast. Res. 59, 156–164. doi: 10.2112/SI59-016.1
Harris, J. B., Joyner, T. A., Rohli, R. V., Friedland, C. J., and Tollefson, W. C. (2020). It's all downhill from here: a forecast of subsidence rates in the lower Mississippi River industrial corridor. Appl. Geogr. 114:102123. doi: 10.1016/j.apgeog.2019.102123
Hausfather, Z. (2018). Explainer: What Climate Models Tell Us About Future Rainfall. CarbonBrief . Available online at: https://www.carbonbrief.org/explainer-what-climate-models-tell-us-about-future-rainfall (accessed July 14, 2021).
He, J., Soden, B. J., and Kirtman, B. (2014). The robustness of the atmospheric circulation and precipitation response to future anthropogenic surface warming. Geophys. Res. Lett. 41, 2614–2622. doi: 10.1002/2014GL059435
Ingram, K., Dow, K., Carter, L., and Anderson, J., (2013). Climate of the Southeast United States: Variability, Change, Impacts, and Vulnerability. Washington, DC: Island Press.
Intergovernmental Panel on Climate Change (2021). Data Distribution Centre. Available online at: https://sedac.ciesin.columbia.edu/ddc/ar5_scenario_process/RCPs.html (accessed July 13, 2021).
Jacobs, J. M., Cattaneo, L. R., Sweet, W., and Mansfield, T. (2018). Recent and future outlooks for nuisance flooding impacts on roadways on the US East Coast. Transp. Res. Rec. 2672, 1–10. doi: 10.1177/0361198118756366
Jankowski, K. L., Törnqvist, T. E., and Fernandes, A. M. (2017). Vulnerability of Louisiana's coastal wetlands to present-day rates of relative sea-level rise. Nat. Commun. 8:14792. doi: 10.1038/ncomms14792
Johnson, C., and Mahoney, T. (2011). “Challenges to mapping coastal risk in the southeastern United States for FEMA's Risk MAP program,” in Solutions to Coastal Disasters 2011, 581–592. Available online at: https://ascelibrary.org/doi/abs/10.1061/41185(417)50 (accessed June 16, 2021).
Jones, C. E., An, K., Blom, R. G., Kent, J. D., Ivins, E. R., and Bekaert, D. (2016). Anthropogenic and geologic influences on subsidence in the vicinity of New Orleans, Louisiana. J. Geophys. Res. Solid Earth 121, 3867–3887. doi: 10.1002/2015JB012636
Junker, N. W., Schneider, R. S., and Fauver, S. L. (1999). A study of heavy rainfall events during the Great Midwest Flood of 1993. Weath. Forecast. 14, 701–712. doi: 10.1175/1520-0434(1999)014<0701:ASOHRE>2.0.CO;2
Kadaba, D. (2018). Interactive Map: Precipitation in the 2050s. The Revelator. Available online at: https://therevelator.org/interactive-map-precipitation-2050/ (accessed July 13, 2021).
Karegar, M. A., Dixon, T. H., and Malservisi, R. (2015). A three-dimensional surface velocity field for the Mississippi Delta: implications for coastal restoration and flood potential. Geology 43, 519–522. doi: 10.1130/G36598.1
Kerr, P. C., Westerink, J. J., Dietrich, J. C., Martyr, R. C., Tanaka, S., Resio, D. T., et al. (2013). Surge generation mechanisms in the lower Mississippi River and discharge dependency. J. Waterway Port Coast. Ocean Eng. 139, 326–335. doi: 10.1061/(ASCE)WW.1943-5460.0000185
Lall, U., Johnson, T., Colohan, P., Aghakouchak, A., Brown, C., McCabe, G., et al. (2018). “Water,” in Impacts, Risks, and Adaptation in the United States: Fourth National Climate Assessment, Volume II, eds. D. R. Reidmiller, C. W. Avery, D. R. Easterling, K. E. Kunkel, K. L. M. Lewis, T. K. Maycock, et al. (Washington, DC: U.S. Global Change Research Program), 145–173. doi: 10.7930/NCA4.2018.CH3
Lane, R. R., Reed, D. J., Day, J. W., Kemp, G. P., McDade, E. C., and Rudolf, W. B. (2020). Elevation and accretion dynamics at historical plots in the Biloxi Marshes, Mississippi Delta. Estuar. Coast. Shelf Sci. 245:106970. doi: 10.1016/j.ecss.2020.106970
Lavers, D. A., and Villarini, G. (2013). Atmospheric rivers and flooding over the central United States. J. Clim. 26, 7829–7836. doi: 10.1175/JCLI-D-13-00212.1
Lehman, W., Dunn, C. N., and Light, M. (2014). “Using HEC-FIA to identify the consequences of flood events,” in 6th International Conference on Flood Management (ICFM6), São Paulo, Brasil. Available online at: http://eventos.abrh.org.br/icfm6/proceedings/papers/PAP014378.pdf (accessed September 8, 2021).
Lehman, W., and Light, M. (2016). “Using HEC-FIA to identify indirect economic losses,” in 3rd European Conference on Flood Risk Management (FLOODrisk 2016), E3S Web of Conferences 7:05008. doi: 10.1051/e3sconf/20160705008
Liu, K.-, b., McCloskey, T. A., Bianchette, T. A., Keller, G., Lam, N. S., Cable, J. E., et al. (2014). Hurricane Isaac storm surge deposition in a coastal wetland along Lake Pontchartrain, southern Louisiana. J. Coast. Res. 70, 266–271. doi: 10.2112/SI70-045.1
Luettich, R. A., Westerink, J. J., and Scheffner, N. W. (1992). ADCIRC: An Advanced Three-Dimensional Circulation Model for Shelves, Coasts, and Estuaries. Report 1, Theory and Methodology of ADCIRC-2DD1 and ADCIRC-3DL. U.S. Army Corps of Engineers Technical Report DP9. Available online at: https://erdc-library.erdc.dren.mil/jspui/bitstream/11681/4618/1/13158.pdf (accessed July 8, 2021).
Mallman, E. P., and Zoback, M. D. (2007). Subsidence in the Louisiana coastal zone due to hydrocarbon production. J. Coast. Res. SI50:443–449.
Meckel, T. A., ten Brink, U. S., and Williams, S. J. (2006). Current subsidence rates due to compaction of Holocene sediments in southern Louisiana. Geophys. Res. Lett. 33:L11403. doi: 10.1029/2006GL026300
Mostafiz, R. B., Friedland, C. J., Rahman, M. A., Rohli, R. V., Tate, E., and Bushra, N. (2021). Comparison of neighborhood-scale, residential property flood-loss assessment methodologies. Front. Environ. Sci. 9:734294. doi: 10.3389/fenvs.2021.734294
Mostafiz, R. B., Friedland, C. J., Rohli, R. V., and Bushra, N. (2020b). “Assessing property loss in Louisiana, U.S.A., to natural hazards incorporating future projected conditions,” in American Geophysical Union Conference, NH015-002. Available online at. https://ui.adsabs.harvard.edu/abs/2020AGUFMNH0150002M/abstract (accessed September 24, 2021).
Mostafiz, R. B., Friedland, C. J., Rohli, R. V., Gall, M., Bushra, N., and Gilliland, J. M. (2020a). Census-block-level property risk estimation due to extreme cold temperature, hail, lightning, and tornadoes in Louisiana, U.S.A. Front. Earth Sci. 8:601624. doi: 10.3389/feart.2020.601624
Nafari, R. H. (2013). Flood Damage Assessment with the Help of HEC-FIA Model. M.S. thesis, Department of Civil and Environmental Engineering. Politechnico Milano. Available online at: https://www.researchgate.net/publication/283043613_Flood_damage_assessment_with_the_help_of_HEC-FIA_model (accessed August 8, 2021).
National Oceanic Atmospheric Administration (2021a). Tides and Currents. Relative Sea Level Trend 8761724 Grand Isle, Louisiana. Available online at: https://tidesandcurrents.noaa.gov/sltrends/sltrends_station.shtml?id=8761724 (accessed May 8, 2021).
National Oceanic Atmospheric Administration (2021b). Sea Level Rise Data Download. Available online at: https://coast.noaa.gov/slrdata/ (accessed May 8, 2021).
National Structure Inventory (NSI) (2019). Hydrologic Engineering Center. Available online at: https://github.com/HydrologicEngineeringCenter/NSI (accessed August 6, 2021).
Nienhuis, J. H., Törnqvist, T. E., Jankowski, K. L., Fernandes, A. M., and Keogh, M. E. (2017). A new subsidence map for coastal Louisiana. GSA Today 27, 58–59. doi: 10.1130/GSATG337GW.1
Olea, R. A., and Coleman, J. L. (2014). A synoptic examination of causes of land loss in southern Louisiana as related to the exploitation of subsurface geologic resources. J. Coast. Res. 30, 1025–1044. doi: 10.2112/JCOASTRES-D-13-00046.1
Oppenheimer, M., Glavovic, B. C., Hinkel, J., van de Wal, R., Magnan, A. K., Abd-Elgawad, A., et al. (2019). “Sea level rise and implications for low-lying islands, coasts and communities,” in IPCC Special Report on the Ocean and Cryosphere in a Changing Climate, eds. H.-O. Pörtner, D. C. Roberts, V. Masson-Delmotte, P. Zhai, M. Tignor, E. Poloczanska, et al. Available online at: https://www.ipcc.ch/site/assets/uploads/sites/3/2019/11/08_SROCC_Ch04_FINAL.pdf (accessed August 5, 2021).
Penland, S., and Ramsey, K. E. (1990). Relative sea-level rise in Louisiana and the Gulf of Mexico: 1908–1988. J. Coast. Res. 6, 323–342.
Reed, D. J., and Yuill, B. (2016). 2017 Coastal Master Plan: attachment C2-2: subsidence. Coastal Protection and Restoration Authority of Louisiana, Baton Rouge, LA. Available at: https://coastal.la.gov/wp-content/uploads/2016/04/Attachment_C2-2_02012017.pdf. Last (accessed August 5, 2021).
Robertson, I. N., Riggs, H. R., Yim, S. C., and Young, Y. L. (2007). Lessons from Hurricane Katrina storm surge on bridges and buildings. J. Waterway Port Coast. Ocean Eng. 133, 463–483. doi: 10.1061/(ASCE)0733-950X(2007)133:6(463)
Santiago-Collazo, F. L., Bilskie, M. V., and Hagen, S. C. (2019). A comprehensive review of compound inundation models in low-gradient coastal watersheds. Environ. Model. Softw. 119, 166–181. doi: 10.1016/j.envsoft.2019.06.002
Schneider, P. J., and Schauer, B. A. (2006). HAZUS—its development and its future. Nat. Hazards Rev. 7, 40–44. doi: 10.1061/ASCE1527-6988(2006)7:2(40)
Shepherd, M., Mote, T., Dowd, J., Roden, M., Knox, P., McCutcheon, S. C., et al. (2011). An overview of synoptic and mesoscale factors contributing to the disastrous Atlanta flood of 2009. Bull. Am. Meteorol. Soc. 92, 861–870. doi: 10.1175/2010BAMS3003.1
Sheridan, S. C., Pirhalla, D. E., Lee, C. C., and Ransibrahmanakul, V. (2017). Atmospheric drivers of sea-level fluctuations and nuisance floods along the mid-Atlantic coast of the USA. Reg. Environ. Change 17, 1853–1861. doi: 10.1007/s10113-017-1156-y
Shinkle, K. D., and Dokka, R. K. (2004). NOAA Technical Report 50: Rates of Vertical Displacement at Benchmarks in the Lower Mississippi Valley and the Northern Gulf Coast. Silver Spring, MD: National Oceanic and Atmospheric Administration, National Geodetic Survey. Available online at: https://citeseerx.ist.psu.edu/viewdoc/download?doi=10.1.1.649.6561andrep=rep1andtype=pdf (accessed August 5, 2021).
Signorini, S. R., Wei, J. S., and Miller, C. D. (1992). Hurricane-induced surge and currents on the Texas-Louisiana shelf. J. Geophys. Res. Oceans 97, 2229–2242. doi: 10.1029/90JC02191
Siverd, C. G., Hagen, S. C., Bilskie, M. V., Braud, D. H., Peele, R. H., Foster-Martinez, M. R., et al. (2019). Coastal Louisiana landscape and storm surge evolution: 1850–2110. Clim. Change 157, 445–468. doi: 10.1007/s10584-019-02575-7
Smith, J. M., Cialone, M. A., Wamsley, T. V., and McAlpin, T. O. (2010). Potential impact of sea level rise on coastal surges in southeast Louisiana. Ocean Eng. 37, 37–47. doi: 10.1016/j.oceaneng.2009.07.008
Tonn, G. L., Guikema, S. D., Ferreira, C. M., and Quiring, S. M. (2016). Hurricane Isaac: a longitudinal analysis of storm characteristics and power outage risk. Risk Anal. 36, 1936–1947. doi: 10.1111/risa.12552
Törnqvist, T. E., Wallace, D. J., Storms, J. E., Wallinga, J., Van Dam, R. L., Blaauw, M., et al. (2008). Mississippi Delta subsidence primarily caused by compaction of Holocene strata. Nat. Geosci. 1, 173–176. doi: 10.1038/ngeo129
Turner, R. E. (2014). Discussion of: Olea, RA and Coleman, JL, Jr. 2014. A synoptic examination of causes of land loss in southern Louisiana as related to the exploitation of subsurface geological resources. J. Coast. Res. 30, 1330–1334. doi: 10.2112/JCOASTRES-D-14-00076.1
United States Census Bureau (2021). City and Town Population Totals: 2010–2019. Available online at: https://www.census.gov/data/tables/time-series/demo/popest/2010s-total-cities-and-towns.html (accessed August 8, 2021).
United States Geological Survey (2021a). The 100-Year Flood. Available online at: https://www.usgs.gov/special-topic/water-science-school/science/100-year-flood (accessed: September 22, 2021).
United States Geological Survey (2021b). Floods and Recurrence Intervals. Available online at: https://www.usgs.gov/special-topic/water-science-school/science/floods-and-recurrence-intervals?qt-science_center_objects=0#qt-science_center_objects (accessed September 22, 2021).
van Vuuren, D. P., Edmonds, J., Kainuma, M., Riahi, K., Thomson, A., Hibbard, K., et al. (2011). The representative concentration pathways: an overview. Clim. Change 109, 5–31. doi: 10.1007/s10584-011-0148-z
Vega, A. J., Grymes, I. I. I., J. M, and Rohli, R. V. (2013). Louisiana Weather and Climate. Sudbury, MA: Jones and Bartlett.
Vega, A. J., Miller, P. W., Rohli, R. V., and Heavilin, J. (2020). Synoptic climatology of nuisance flooding along the Atlantic and Gulf of Mexico coasts, USA. Nat. Hazards 105, 1281–1297. doi: 10.1007/s11069-020-04354-5
Voyiadjis, G. Z., and Zhou, Y. N. (2018). Time-dependent modeling of subsidence due to drainage in bounding shales: application to a depleted gas field in Louisiana. J. Petrol. Sci. Eng. 166, 175–187. doi: 10.1016/j.petrol.2018.03.032
Wamsley, T. V., Cialone, M. A., Smith, J. M., Atkinson, J. H., and Rosati, J. D. (2010). The potential of wetlands in reducing storm surge. Ocean Eng. 37, 59–68. doi: 10.1016/j.oceaneng.2009.07.018
Wamsley, T. V., Cialone, M. A., Smith, J. M., Ebersole, B. A., and Grzegorzewski, A. S. (2009). Influence of landscape restoration and degradation on storm surge and waves in southern Louisiana. Nat. Hazards 51, 207–224. doi: 10.1007/s11069-009-9378-z
Wang, S. Y. S., Zhao, L., and Gillies, R. R. (2016). Synoptic and quantitative attributions of the extreme precipitation leading to the August 2016 Louisiana flood. Geophys. Res. Lett. 43, 11805–11814. https://agupubs.onlinelibrary.wiley.com/doi/pdfdirect/10.1002/2016GL071460
Warner, M. D., Mass, C. F., and Salathe, J.r. E. P. (2012). Wintertime extreme precipitation events along the Pacific Northwest coast: climatology and synoptic evolution. Mon. Weather Rev. 140, 2021–2043. doi: 10.1175/MWR-D-11-00197.1
Westerink, J. J., Luettich, R. A., Feyen, J. C., Atkinson, J. H., Dawson, C., Roberts, H. J., et al. (2008). A basin-to channel-scale unstructured grid hurricane storm surge model applied to southern Louisiana. Mon. Weather Rev. 136, 833–864. doi: 10.1175/2007MWR1946.1
Williams, H. F. (2012). Magnitude of Hurricane Ike storm surge sedimentation: implications for coastal marsh aggradation. Earth Surf. Process. Landforms 37, 901–906. doi: 10.1002/esp.3252
Williams, H. F. L., and Flanagan, W. M. (2009). Contribution of Hurricane Rita storm surge deposition to long-term sedimentation in Louisiana coastal woodlands and marshes. J. Coast. Res. 56, 1671–1675. Available online at: https://www.jstor.org/stable/pdf/25738074.pdf (accessed May 8, 2021).
Wing, O. E. J., Bates, P. D., Smith, A. M., Sampson, C. C., Johnson, K. A., Fargione, J., et al. (2018). Estimates of present and future flood risk in the conterminous United States. Environ. Res. Lett. 13:034023. doi: 10.1088/1748-9326/aaac65
Yang, Z., Wang, T., Leung, R., Hibbard, K., Janetos, T., Kraucunas, I., et al. (2014). A modeling study of coastal inundation induced by storm surge, sea-level rise, and subsidence in the Gulf of Mexico. Nat. Hazards 71, 1771–1794. doi: 10.1007/s11069-013-0974-6
Yuill, B., Lavoie, D., and Reed, D. J. (2009). Understanding subsidence processes in coastal Louisiana. J. Coast. Res. 54, 23–36. doi: 10.2112/SI54-012.1
Zhou, Y. N., and Voyiadjis, G. Z. (2019). Finite element modeling of production-induced compaction and subsidence in a reservoir along coastal Louisiana. J. Coast. Res. 35, 600–614. doi: 10.2112/JCOASTRES-D-18-00108.1
Keywords: future flood, risk assessment, coastal Louisiana, sea level rise, storm surge, climate change, tropical cyclones, subsidence
Citation: Mostafiz RB, Bushra N, Rohli RV, Friedland CJ and Rahim MA (2021) Present vs. Future Property Losses From a 100-Year Coastal Flood: A Case Study of Grand Isle, Louisiana. Front. Water 3:763358. doi: 10.3389/frwa.2021.763358
Received: 23 August 2021; Accepted: 11 October 2021;
Published: 04 November 2021.
Edited by:
Irfan Ahmad Rana, National University of Sciences and Technology (NUST), PakistanReviewed by:
Xianwu Shi, National Marine Hazard Mitigation Service, ChinaCopyright © 2021 Mostafiz, Bushra, Rohli, Friedland and Rahim. This is an open-access article distributed under the terms of the Creative Commons Attribution License (CC BY). The use, distribution or reproduction in other forums is permitted, provided the original author(s) and the copyright owner(s) are credited and that the original publication in this journal is cited, in accordance with accepted academic practice. No use, distribution or reproduction is permitted which does not comply with these terms.
*Correspondence: Rubayet Bin Mostafiz, cmJpbm1vMUBsc3UuZWR1
Disclaimer: All claims expressed in this article are solely those of the authors and do not necessarily represent those of their affiliated organizations, or those of the publisher, the editors and the reviewers. Any product that may be evaluated in this article or claim that may be made by its manufacturer is not guaranteed or endorsed by the publisher.
Research integrity at Frontiers
Learn more about the work of our research integrity team to safeguard the quality of each article we publish.