- 1Eau Terre et Environnement Research Centre, Institut national de la recherche scientifique, Quebec, QC, Canada
- 2Department of Bio-processing fermentation, BOSK Bioproducts, Quebec, QC, Canada
- 3Department of Chemistry, Université de Montréal, Montreal, QC, Canada
- 4Department of Industrial Innovation, Investissement Québec Centre de Recherche Industrielle du Québec (CRIQ), Quebec, QC, Canada
The fate of 12 pharmaceutical pollutants was investigated to understand their removal mechanism during hospital wastewater (HWW) treatment in submerged membrane bioreactor (SMBR). High concentrations of anti-depressant (venlafaxine and desvenlafaxine), analgesic (ibuprofen and hydroxy-ibuprofen), and caffeine were detected in the HWW during the entire study period. The SMBR showed high removal >70% of antibiotics (sulfamethoxazole and clarithromycin), beta-blocker (acebutolol), hormone (estrone), and caffeine via biodegradation. The partial degradation of diclofenac, venlafaxine, and desvenlafaxine in SMBR indicates the growth promoter or agent requirement, which could facilitate the metabolism and co-metabolism of these pharmaceuticals by microorganisms. The study demonstrated that the major removal mechanism of pharmaceuticals in SMBR at optimized treatment conditions was biodegradation for the majority of examined pharmaceuticals. The assessment of SMBR performance at the low temperature of 15 and 10°C resulted in the drop of biodegradation efficiency of SMBR, affecting overall pharmaceuticals removal.
Introduction
The increases in demand and production of pharmaceutical compounds resulted in the prevalence of pharmaceutical pollutants in the environment (Tiwari et al., 2016). The presence of ibuprofen, diclofenac, trimethoprim, paracetamol, and citalopram was documented in the 12 estuaries of the UK (Letsinger et al., 2019). A recent study reported the presence of 11 pharmaceuticals, namely Betamethasone, Fluconazole, Loratadine, Prednisone, Atorvastatin, Danofloxacin, Enoxacin, Norfloxacin, Ketoprofen, and Gemfibrozil in the drinking water treatment plant of Brazil, with a concentration ranging from few ng L−1 to 6,323 ng L−1 (Reis et al., 2019). The presence of pharmaceuticals in waterbodies can impact aquatic organisms; for instance, a study reported that the presence of diclofenac affects immune response and kidney and gill integrity of fish (Hoeger et al., 2005). Moreover, the synergistic effect of two or more pharmaceuticals (even at low environmental concentration) is stronger than the individual compound. Cleuvers (2003) showed that the consolidated effect of carbamazepine and clofibric acid was more substantial on Daphnia magna compared to a single compound. The presence of pharmaceuticals in the aquatic environment indicates the need to develop an efficient treatment process.
The investigation of membrane bioreactor process (MBR) and conventional activated sludge process (CAS) reported the better performance of the former technique for micropollutants and pathogens removal (Kim et al., 2014; Tiwari et al., 2016). The MBR process offers many advantages over other biological treatment techniques, which favors the efficient treatment of wastewater. Apart from the high biomass concentration and sludge retention, MBR has many benefits that result in the efficient removal of micro-pollutant. For instance, in MBR, the specific floc surface per unit reactor volume was 10 times higher than CAS, facilitating the substrate's diffusion (such as micro-pollutants) into the flocs (Cirja et al., 2007). Moreover, the MBR was reported to have a higher viable fraction of biomass compared to the CAS process due to efficient mass transfer conditions (Cirja et al., 2007). The MBR allows maintenance of long sludge retention time which increases the assimilation potential of microorganisms for micro-pollutants via adaptation and mutation.
The research investigation on removing pharmaceutical pollutants from water and wastewater using MBR reveals that the MBR could be an efficient treatment option for pharmaceutical removal (Cirja et al., 2007; Tadkaew et al., 2011; Rua-Gomez and Puttmann, 2012; Tiwari et al., 2019). Sipma et al. (2010) reviewed the pharmaceutical removal efficiency of MBR and CAS. The study demonstrated that the removal of ibuprofen, diclofenac, ketoprofen, indomethacin, naproxen, mefenamic acid, sulfamethoxazole, and others were 20–50% higher in MBR (Sipma et al., 2010). The efficiency of MBR for the elimination of pharmaceutical pollutants depends on various factors, such as hydraulic retention time (HRT), sludge retention time (SRT), pH, temperature, suspended solid concentration, physicochemical properties of the pollutant, and microbial community (Cirja et al., 2007; Tadkaew et al., 2011; Tiwari et al., 2019). The physicochemical properties of pharmaceutical pollutants, namely, hydrophobicity, hydrophilicity, and chemical structure, influence the sorption and biodegradation process in MBR. For instance, the presence of halogen and a strong electron acceptor makes the compound less susceptible to degradation. The hydrophobicity and hydrophilicity of a compound govern its sorption on the sludge solids.
Apart from the physicochemical properties, the operating parameter of MBR also influences pharmaceutical removal and its mechanism. For instance, oxytetracycline was reported to have high sorption on sludge even if it exists in the form of a zwitter ion (Cirja et al., 2007). The study suggests that along with the physicochemical properties, the sludge characteristics influences the interaction of pharmaceutical pollutant to the sludge matrix (Cirja et al., 2007). Thus, to understand pharmaceutical fate during the biological treatment of hospital wastewater, the study was conducted to investigate the removal mechanism of pharmaceutical pollutants in submerged membrane bioreactor (SMBR). The operating temperature of SMBR is critical, which affects treatment efficiency by altering microbial activity. The alteration in microbial activity may affect the removal mechanism of pharmaceutical pollutants. Therefore, the study also analyzed the influence of varying operating temperatures on SMBR performance and the removal mechanism of pharmaceuticals. Apart from temperature, the other operating conditions of SMBR were also investigated and discussed in our previous publications (Tiwari et al., 2019).
Materials and Methods
Hospital Wastewater Sampling
The hospital wastewater was sampled from one of the hospitals of Quebec City (QC), Canada. The hospital generates ~200 m3 of wastewater daily. The hospitals have a separate sewer network for the wastewater generated from operating blocks. The sewer networks of hospitals discharge wastewater into the public sewer without any pre-treatment. The hospital wastewater for the study was collected from the sewer network connected to the operating blocks of the hospital by using an automatic sampler (Teledyne Isco 3710 auto-sampler).
SMBR Setup and Operation
In order to investigate the removal mechanism of pharmaceutical pollutants from hospital wastewater, a SMBR (with 6L working volume) was operated in continuous mode. The SMBR was equipped with a hollow fiber membrane (ZW-1, Zenon Environmental Inc., Canada) having a pore size of 0.04 μm. The wastewater was fed in the SMBR unit using a peristaltic pump. The hollow fiber membrane was placed vertically within the reactor, and it was connected with a second peristaltic pump for filtration and backwashing. The SMBR has a filtration cycle of 50 sec and a backwash of 10 sec. The compressed air was continuously supplied in the reactor using a perforated tube placed in the bottom of the reactor. Initially, the SMBR was inoculated with activated sludge of a municipal wastewater treatment plant (Victoriaville, Quebec, Canada). The mixed liquor suspended solids (MLSS), and mixed liquor volatile suspended solids (MLVSS) concentration of the seed sludge was 5 and 3.5 g L−1, respectively. The biomass of SMBR was developed using synthetic hospital wastewater augmented with pharmaceuticals (Tiwari et al., 2019). Once the SMBR biomass reached the MLSS concentration of 15.8 g L−1, the study on optimization of SMBR operating conditions was performed (Tiwari et al., 2019, 2021). Following the optimization, the acclimatization of SMBR biomass with hospital wastewater was performed. The pH of the SMBR was maintained at 7 ± 0.5. An SRT of 100 days and HRT of 18 h optimized previously was utilized to examine the SMBR performance for hospital wastewater treatment. The dissolved oxygen concentration of 8 mg L−1was maintained in the SMBR. To study the effect of varying operating temperature of SMBR on the fate of pharmaceuticals, the desired temperature was achieved using a lab-made thermostat. The circulating bath refrigerator was connected to the thermostat to maintain the operating temperature.
Analytical Methods
The SMBR samples (feed, effluent, and sludge) were collected regularly (twice a week) for pharmaceutical analysis. The pH and temperature of the SMBR were measured using Acumet pH meter (Ion meter excel XL2, Fisher Scientific Co.) equipped with a Cole-Palmer double junction electrode (reference Ag/AgCl). Liquid chromatography and tandem mass spectrometry analysis (LC-MS/MS) (Thermo Scientific TSQ Quantiva Triple-Stage Quadrupole Mass Spectrometer, Germany) technique was utilized for the determination of pharmaceuticals concentration. The High-Performance Liquid Chromatography (HPLC) grade water and methanol were used for the pre-concentration and elution step. The recovery of pharmaceutical was >80% in influent, and effluent of SMBR and limit of detection and limit of quantification was <1 ng L−1.
The method used for the pharmaceuticals analysis in sludge was a classic organic solvent extraction with sonication and centrifugation. The extract is pre-concentrated and diluted in water to do the purification, which is a solid-phase extraction (SPE) on Hypersep Retain polar enhanced polymer (PEP) cartridges from Thermo Scientific (200 mg, 6 mL). After purification, the clean extract was dried under nitrogen and reconstituted in the injection solvent. The analysis was done on the High-Performance Liquid Chromatography-Mass spectrometry (HPLC-MS) system.
For the determination of sample concentrations, both in the effluent and sludge, a matched-matrix calibration curve was used with the addition of an isotope-labeled internal standard. These same internal standards are added to the samples prior to pre-treatment steps (filtration, extraction, purification). Quality control solutions and solvent blanks are injected during each analytical run to ensure system stability and adequate performance.
Mass Balance
The fate of pharmaceuticals in SMBR was determined by evaluating the biodegradation and sorption to sludge solids. The fraction of mass loading of pharmaceuticals that were sorbed to sludge, bio-degraded, and remained in SMBR permeate were calculated:
Thus, MB (mg d−1) = MI – Ms- Mp
Where MB is the mass of pharmaceutical biodegrade/transformed in SMBR, MI is the mass of pharmaceutical in SMBR influent (mg d−1), MP is the mass of pharmaceutical in SMBR permeate (mg d−1), Ms is the mass of pharmaceutical sorbed to sludge (mg d−1).
Results
Distribution of Pharmaceuticals in HWW, SMBR Sludge, and Permeate
The study investigated the occurrences of 12 pharmaceuticals in HWW and their removal in SMBR. The monitored pharmaceuticals were ibuprofen, hydroxy ibuprofen, diclofenac, hydroxy-diclofenac, acebutolol, estrone, sulfamethoxazole, clarithromycin, venlafaxine, desvenlafaxine, caffeine, and carbamazepine. The detailed operating condition of SMBR and treatment efficiency was provided elsewhere (Tiwari et al., 2021). Table 1 shows the average concentration of pharmaceuticals in SMBR influent i.e., in HWW, permeate, and sludge. High concentration of ibuprofen, hydroxy-ibuprofen, venlafaxine, desvenlafaxine and caffeine were detected in the range of 1,000–70,000 ng L−1 in HWW. The concentration of diclofenac, hydroxy-diclofenac, acebutolol, estrone, clarithromycin, and carbamazepine were ranged between 20 and 350 ng L−1 in HWW. The measured concentration of ibuprofen, caffeine, hydroxy–ibuprofen in HWW was similar to those reported in wastewater treatment of Canada (Kim et al., 2014).
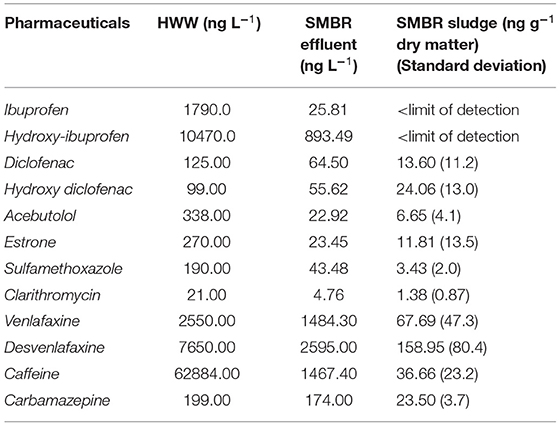
Table 1. Mean concentration of pharmaceuticals in SMBR influent, effluent, and sludge at optimized treatment condition.
In SMBR permeate, desvenlafaxine was the most abundant pharmaceutical, followed by venlafaxine, caffeine, and hydroxy ibuprofen. However, the concentration of caffeine was two orders of magnitude lower in permeate compared to its concentration in HWW, indicating its high removal in SMBR. Excluding the abundant pharmaceuticals, the concentration of acebutolol, estrone, sulfamethoxazole, clarithromycin, venlafaxine, diclofenac, hydroxy-diclofenac, and carbamazepine in permeate was in the range of low ng L−1 (2–200 ng L−1). Analgesic pharmaceutical ibuprofen and hydroxy ibuprofen were not detected in the sludge. Ibuprofen are slightly less sensitive for the analysis in solid phase, and the matrix effect of such contaminated samples (SMBR sludge) rose the background signal and affected the limit of detection. Venlafaxine and desvenlafaxine were the abundant drug residues detected in SMBR sludge. Their concentration ranged between 50 and 200 ng L−1. Apart from venlafaxine and desvenlafaxine, the concentration of other studied pharmaceuticals was <50 ng g−1 of dry sludge.
Fate of Pharmaceuticals in SMBR
The detailed SMBR performance and optimization of treatment conditions of SMBR were (i.e., SRT−100 d, HRT- 18h, temperature of 20°C) were provided elsewhere (Tiwari et al., 2021). The fate of pharmaceuticals in SMBR was summarized in Tables 2–4. Excluding carbamazepine and hydroxy- diclofenac, the major removal mechanism of all the studied pharmaceuticals was biodegradation. SMBR achieved >90% removal of acebutolol, estrone, and caffeine. The high biodegradation percentage of these pharmaceuticals indicates that the microbial community of SMBR was able to metabolize and/or co-metabolize these micro-pollutants readily. Although in SMBR, the total removal of sulfamethoxazole and clarithromycin were ranged between 75 and 80%, the biodegradation of both antibiotics was >70%. The biomass of SMBR was not able to degrade carbamazepine, and its degradation was negligible. The moderate biodegradation of diclofenac, venlafaxine, and desvenlafaxine (ranged 45–65%), shows the biodegradation potential of the microbial community to degrade these complex drug residues.
The SMBR exhibit negligible or low (<3 %) sorption of the anti-depressant drug venlafaxine and its metabolite desvenlafaxine. The log Kow of venlafaxine is 0.43, which explains its low sorption on sludge. The moderate biodegradation of venlafaxine in SMBR (~40%) was in agreement with the previous studies (Gasser et al., 2012; Li et al., 2012; Rua-Gomez and Puttmann, 2012; Casas et al., 2015).
The sorption of diclofenac and hydroxy diclofenac on SMBR sludge was 10.3 and 23.66%, respectively. Carbamazepine is H-dibenzo[b,f]azepine-5-carboxamide, which contains a strong electron acceptor amide group. The oxidation of carbamazepine by microbial oxygenase requires a strong electron donor; however, in carbamazepine, the presence of amide limits its degradation (Tadkaew et al., 2011). A study suggested that the electron deficiency makes carbamazepine less susceptible to oxidative catabolism under aerobic conditions (Hai et al., 2011). Investigation on carbamazepine degradation reveals the anaerobic microorganism facilitates the degradation of carbamazepine due to the presence of a reducing agent, which reduces the electron deficiency and aids in catabolism (Zhang et al., 2008; Hai et al., 2011).
Fate of Pharmaceuticals in SMBR at Varying Temperature
The performance of SMBR for the removal of pharmaceutical pollutants at varying operating temperature was studied. The SMBR showed that the decrease in operating temperature reduced the pharmaceuticals removal. The detailed impact of temperature on SMBR performance was provided elsewhere (Tiwari et al., 2021) The fate of pharmaceuticals during different operating temperatures (20, 15, and 10°C) was assessed to understand the effect of temperature on sorption and biodegradation process. The criteria behind the selection of varying temperature ranges were based on the climatic condition of the area where this study was conducted. In Canada, the operating temperature of wastewater treatment plants (WWTP) varies from 5 to 20°C (Lishman et al., 2006). The fate of pharmaceuticals in SMBR at the operating temperature of 20°C (optimized condition) was discussed in section 3.2. This section provides the fate of pharmaceuticals in SMBR at the operating temperature of 15–10°C. During the biological treatment, the temperature not only impacted the biodegradation by influencing the microbial community but also affects the sorption equilibrium (Hulscher and Cornelissen, 1996). Hulscher and Cornelissen (1996) reviewed the impact of temperature on the sorption equilibrium of organic micro-pollutant. The study indicated that an increase in temperature increases the solubility of compounds, thus decreasing the sorption. The study further states that the sorption of micro-pollutants on organic matrix occurred by enthalpy related adsorption forces. The enthalpy related adsorption forces are van der wall interaction, dipole interaction, hydrogen bond, and covalent chemical bond. A chemical compound with a polar group either interacts electrostatically or donates or accepts a hydrogen bond. This electrostatic interaction will provide an exothermic contribution to the sorption enthalpy. Thus, the change in temperature influences the equilibrium sorption enthalpy and hence the sorption potential (Hulscher and Cornelissen, 1996).
The SMBR evident the decrease in biodegradation percentage of pharmaceuticals at low operating temperature. The fate of pharmaceuticals in SMBR at an operating temperature of 15 and 10°C was reported in Tables 2–4, respectively. At an operating temperature of 15°C, the biodegradation percentage of diclofenac, hydroxy diclofenac, sulfamethoxazole, clarithromycin, venlafaxine, and desvenlafaxine were reduced significantly (Table 4). However, only a slight decrease in the biodegradation percentage of estrone and caffeine was observed. The high biodegradation of estrone at low temperatures confirms the previous assumption that heterotrophic bacteria are involved in the degradation of these pharmaceuticals (Tiwari et al., 2021). Heterotrophs had a faster growth rate, and they are relatively less sensitive to temperature variation compared to nitrifying bacteria. Among the studied pharmaceuticals, a massive reduction in the biodegradation percentage of acebutolol from 92.94% at 20°C to 41.06 at 15°C was observed. A 51.88% reduction in biodegradation percentage of acebutolol indicates that the microorganism involved in acebutolol degradation was greatly affected by temperature variation.
Discussion
Removal Mechanism of Pharmaceuticals in SMBR
The removal mechanisms of pharmaceuticals in WWTP are photo-degradation, biodegradation, volatilization, sorption, and physical retention. The physicochemical properties of studied pharmaceuticals are provided in Table 5. The factors limiting pharmaceutical removal in biological treatment include low Henry's constant of pharmaceutical (for volatilization), high turbidity, which prevents photo-degradation, and small molecular weight of drug residue, which facilitate its diffusion from an ultrafiltration membrane. Thus, biodegradation and sorption to biomass are the only processes responsible for pharmaceutical removal (Cirja et al., 2007; Tiwari et al., 2016). The study investigated the removal mechanism of pharmaceuticals in SMBR. The percentage of pharmaceutical degradation, sorption to sludge solid were estimated according to Kim et al. (2014). Since the study could not analyze ibuprofen and hydroxy-ibuprofen in sludge, the fate of these two compounds was not discussed.
The mechanism of caffeine degradation in bacteria involves oxidative demethylation of caffeine, resulting in the formation of dimethyl uric acid and xanthine (Korekar et al., 2019). The catabolic pathway of microbial degradation of caffeine was studied in bacteria like Pseudomonas, Rhodococcus, Klebsiella, and Serratia (Korekar et al., 2019). Lin et al. (2010) demonstrated that the sorption of acebutolol was a major removal mechanism in the batch study, and biodegradation was insignificant. However, the high biodegradation of acebutolol might be due to the presence of amine and amide functional groups, which act as a strong electron donor and electron acceptor. The presence of a strong electron donor group facilitates electrophilic attack by oxygenase enzymes (Tiwari et al., 2021). To our knowledge, this is one of the first studies which analyses the fate of acebutolol in SMBR and its high biodegradation requires further in-depth investigation.
Gaulke et al. (2009) studied the biodegradation kinetic of estrone and reported that the biodegradation rate coefficient (kb) of estrone was higher than the ethinylestradiol. The kb of estrone and ethinylestradiol was 17.5 and 0.522 L/gVSS-day, respectively. The study showed that the ammonia-oxidizing bacteria (AOB) aid in the abiotic transformation of estrone to nitro estrone. The nitro estrone has lower estrogenic activity than its parent compound (Gaulke et al., 2009). However, the study further stated that the estrogen degradation was not limited to nitrifying bacteria. The high kb of estrone, i.e., 17.5 L}gVSS-day, indicated that the heterotrophic bacteria play an essential role in estrogen degradation (Gaulke et al., 2009).
The antibiotic sulfamethoxazole has a low octanol-water coefficient (log Kow <1), which prevents its sorption on the sludge solids. Moreover, the presence of an ionizable amino group in sulfamethoxazole makes it negatively charged at neutral pH, which causes electrostatic repulsion between the drug residue and sludge. The removal of sulfamethoxazole during the biological treatment is governed by co-metabolic degradation by bacteria (Kassotaki et al., 2016). Kassotaki et al. (2016) reported that the inhibition of AOB by the addition of allylthiourea reduces the elimination of sulfamethoxazole. However, Pseudomonas, Bacillus, Rhodococcus, and Mycobacterium were reported to degrade sulfamethoxazole via oxidative catabolism (Larcher and Yargeau, 2012). The antibiotic clarithromycin has a high log Kow of 3.16; the compounds with Kow ≥ 3 are considered as bioaccumulative (Sahar et al., 2011). Abegglen et al. (2009) reported that the biodegradation of clarithromycin was insignificant in batch degradation tests using activated sludge and only sorption constitutes in its removal from wastewater. However, the study examined a relatively high concentration of clarithromycin (0.25 to 1 mg L−1) (Abegglen et al., 2009). In the present study, the average clarithromycin concentration in HWW was 21 ng L−1; thus the high biodegradation percentage of clarithromycin in SMBR could be due to its low concentration and co-metabolism (Terada et al., 2017).
Gasser et al. (2012) demonstrated that the microbial biotransformation of venlafaxine requires stereoisomeric selectivity. In the human body, the enzyme cytochrome P450 (CYP) aid in the stereo-selective metabolism of venlafaxine. The biodegradation of venlafaxine and desvenlafaxine in SMBR has been attributed to the presence of microbial communities which produce CYP enzymes. Several fungal strains reported to produce CYP enzyme were identified in the SMBR and discussed elsewhere (Tiwari et al., 2021).
The anticonvulsant drug carbamazepine elimination was insignificant in the SMBR during the entire study period. The investigation on the fate of carbamazepine during the biological treatment process reveals that the carbamazepine are poorly removed during the aerobic treatment of wastewater due to their complex chemical structure and low adsorption coefficient (Cirja et al., 2007; Zhang et al., 2008; Tadkaew et al., 2011).
The sorption of diclofenac on primary and secondary sludge varies between 5 and 15%, and the highest adsorption coefficient (Kd) of diclofenac was reported for primary sludge, i.e., 1.3–2.3 (Vieno and Sillanpaa, 2014). The Kd of diclofenac on MBR sludge were ranged between 2.3 and 2.5, which is slightly higher than primary sludge (Vieno and Sillanpaa, 2014). Previous investigations revealed that MBR exhibits smaller flocs sizes compared to conventional activated sludge processes (Munz et al., 2008). Thus, the high adsorption potential of diclofenac in MBR could be due to the small floc size, which provides a higher surface area for sorption. The low sorption percentage of diclofenac in SMBR might be due to the long SRT (100 d), which might enhance the biodegradation potential of the microbial community. The acidic pharmaceutical diclofenac was reported to alter the bacterial community structure of WWTP and resulted in a decreased diversity of nitrifiers population (Vieno and Sillanpaa, 2014; Cherik et al., 2015). The biodegradation constant (Kbiol) of diclofenac in MBR was < 0.1 (Vieno and Sillanpaa, 2014). According to a study, the biodegradation of pollutants with Kbiol < 0.1 was <20% (Suárez et al., 2012). However, the SMBR exhibits ~37% of biodegradation of diclofenac. The slight high removal of diclofenac in SMBR could result from the long SRT and acclimatizing microbial population. The long SRT favors the growth of slow-growing nitrifying bacteria, which are assumed to be involved in diclofenac degradation (Tiwari et al., 2021). A study reported an increase in diclofenac degradation while enriching the sludge with the nitrifying bacterial population (Suárez et al., 2012).
Removal Mechanism of Pharmaceuticals at Varying Temperature
The decrease in temperature from 20 to 15°C resulted in a slight increase in the sorption percentage of all the studied pharmaceuticals except carbamazepine. A negative removal of carbamazepine was observed in SMBR. The negative removal of carbamazepine was either due to the reconversion of carbamazepine metabolites into the parent compound or due to the desorption of carbamazepine residue from sludge at low temperature (Duran-Alvarez et al., 2012; Tiwari et al., 2019). The increased sorption of pharmaceuticals like diclofenac, clarithromycin, venlafaxine, desvenlafaxine, estrone, and caffeine at low operating temperature (15°C) indicates that the sorption of these pharmaceuticals could be exothermic and reversible process (Ren et al., 2007). For instance, the thermodynamic analysis of adsorption of estrone on sludge showed that the estrone tended to adsorb more at low temperature and can be considered an exothermic adsorption process (Ren et al., 2007; Qingling et al., 2009). Although the sorption of most of the studied pharmaceuticals was increased at 15°C, the overall removal of pharmaceuticals in SMBR was reduced due to a decrease in biodegradation percentage.
The further decrease in SMBR operating temperature from 15 to 10°C reduces the sorption and biodegradation and thus deteriorates pharmaceutical removal. The comparative analysis of the sorption percentage of pharmaceuticals at 15 and 10°C indicates a decrease in sorption of all the studied pharmaceuticals except caffeine. An investigation on sorption kinetics of caffeine demonstrates that caffeine has a fast initial sorption phase which dominates the biodegradation process (Martinez-Hernandez et al., 2016). However, the effect of temperature on the sorption kinetics of caffeine required further investigation.
Although the sorption of pharmaceuticals is less sensitive to low temperature, the overall decrease in pharmaceutical removal indicates that bio-degradation is the major removal mechanism of all the studied pharmaceuticals. The reduction in biodegradation percentage shows that the degrading microbial community are highly susceptible to temperature variation. In order to enhance the pharmaceutical removal in SMBR, the investigation on pharmaceutical removal at warmer temperatures (25, 30, and 35°C) is required. However, the operation and maintenance of warmer temperatures in WWTP in colder countries like Canada or America are challenging; thus, tertiary treatment of pharmaceutical containing wastewater may provide complete neutralization.
Conclusion
The study investigated the removal mechanism of pharmaceutical pollutants during the treatment of hospital wastewater in SMBR. The obtained result showed that biodegradation was the major removal mechanism of pharmaceuticals in SMBR at optimized treatment conditions. The change in treatment conditions affects the removal efficiency and removal mechanism of pharmaceutical pollutants in SMBR. The investigation on the fate of pharmaceuticals in SMBR at varying temperatures leads to a decline in the biodegradation potential of microorganisms for pharmaceutical removal. The partial bio-degradation of certain pharmaceuticals in SMBR such as diclofenac, venlafaxine, sulfamethoxazole, and clarithromycin, indicates that the microbial community of SMBR has the capability to degrade these recalcitrant pollutants. However, the complete degradation requires thorough investigation on other factors which influence their biodegradability. The study extended our knowledge on the fate of pharmaceutical pollutants in SMBR and suggested that the influence of other operating parameters on the removal mechanism of pharmaceutical pollutants should be investigated.
Data Availability Statement
The original contributions presented in the study are included in the article/supplementary material, further inquiries can be directed to the corresponding author/s.
Author Contributions
BT: conceptualization, methodology, formal analysis, investigation, and writing original draft. YO: conceptualization and investigation. PD, SS, GB, RD, and RT: supervision and review. MV: methodology, resource, and software. All authors contributed to the article and approved the submitted version.
Conflict of Interest
The authors declare that the research was conducted in the absence of any commercial or financial relationships that could be construed as a potential conflict of interest.
The authors declare that this study received funding from Natural Sciences and Engineering Research Council of Canada (NSERC-STPGP-479160 Strategic grant). The funder provides financial support for conducting the study. The funder was not involved in the study design, collection, analysis, interpretation of data, the writing of this article or the decision to submit it for publication.
Publisher's Note
All claims expressed in this article are solely those of the authors and do not necessarily represent those of their affiliated organizations, or those of the publisher, the editors and the reviewers. Any product that may be evaluated in this article, or claim that may be made by its manufacturer, is not guaranteed or endorsed by the publisher.
Acknowledgments
Authors would like to thank Natural Sciences and Engineering Research Council of Canada, Canada (NSERC-STPGP-479160 Strategic grant, Premier Tech Ltée, and Canada Research Chair) for financial support.
References
Abegglen, C., Joss, A., McArdell, C. S., Fink, G., Schlusener, M. P., Ternes, T. A., and Siegrist, H. (2009). The fate of selected micropollutants in a single-house MBR. Water Res. 43, 2036–2046. doi: 10.1016/j.watres.2009.02.005
Casas, M. E., Chhetri, R. K., Ooi, G., Hansen, K. M., Litty, K., Christensson, M., et al. (2015). Biodegradation of pharmaceuticals in hospital wastewater by staged Moving Bed Biofilm Reactors (MBBR). Water Res. 83, 293–302. doi: 10.1016/j.watres.2015.06.042
Cherik, D., Benali, M., and Louhab, K. (2015). Occurrence, ecotoxicology, removal of diclofenac by adsorption on activated carbon and biodegradation and its effect on bacterial community: a review. World Scientific News 10, 116–144.
Cirja, M., Ivashechkin, P., Schäffer, A., and Corvini, P. F. X. (2007). Factors affecting the removal of organic micropollutants from wastewater in conventional treatment plants (CTP) and membrane bioreactors (MBR). Rev. Environ. Sci. Bio/Technol. 7, 61–78. doi: 10.1007/s11157-007-9121-8
Cleuvers, M. (2003). Aquatic ecotoxicity of pharmaceuticals including the assessment of combination effects. Toxicol. Lett. 142, 185–194. doi: 10.1016/S0378-4274(03)00068-7
Das, S., Ray, N. M., Wan, J., Khan, A., Chakraborty, T., and Ray, M. B. (2017). Micropollutants in wastewater: fate and removal processes. Physico-chem. Wastewater Treat. Resour. Recov. 75:65644. doi: 10.5772/65644
Duran-Alvarez, J. C., Prado-Pano, B., and Jimenez-Cisneros, B. (2012). Sorption and desorption of carbamazepine, naproxen and triclosan in a soil irrigated with raw wastewater: estimation of the sorption parameters by considering the initial mass of the compounds in the soil. Chemosphere 88, 84–90. doi: 10.1016/j.chemosphere.2012.02.067
Gasser, G., Pankratov, I., Elhanany, S., Werner, P., Gun, J., Gelman, F., et al. (2012). Field and laboratory studies of the fate and enantiomeric enrichment of venlafaxine and O-desmethylvenlafaxine under aerobic and anaerobic conditions. Chemosphere 88, 98–105. doi: 10.1016/j.chemosphere.2012.02.074
Gaulke, L. S., Strand, S. E., Kalhorn, T. F., and Stensel, H. D. (2009). Estrogen biodegradation kinetics and estrogenic activity reduction for two biological wastewater treatment methods. Environ. Sci. Technol. 43, 7111–7116. doi: 10.1021/es901194c
Hai, F. I., Li, X., Price, W. E., and Nghiem, L. D. (2011). Removal of carbamazepine and sulfamethoxazole by MBR under anoxic and aerobic conditions. Bioresour. Technol. 102, 10386–10390. doi: 10.1016/j.biortech.2011.09.019
Hoeger, B., Köllner, B., Dietrich, D. R., and Hitzfeld, B. (2005). Water-borne diclofenac affects kidney and gill integrity and selected immune parameters in brown trout (Salmo trutta f. fario). Aquatic Toxicol. 75, 53–64. doi: 10.1016/j.aquatox.2005.07.006
Hulscher, T. E., and Cornelissen, G. (1996). Effect of temperature on sorption equilibrium and sorption kinetics of organic micropollutants-a review. Chemosphere 32, 609–626. doi: 10.1016/0045-6535(95)00345-2
Kassotaki, E., Buttiglieri, G., Ferrando-Climent, L., Rodriguez-Roda, I., and Pijuan, M. (2016). Enhanced sulfamethoxazole degradation through ammonia oxidizing bacteria co-metabolism and fate of transformation products. Water Res. 94, 111–119. doi: 10.1016/j.watres.2016.02.022
Kim, M., Guerra, P., Shah, A., Parsa, M., Alaee, M., and Smyth, S. A. (2014). Removal of pharmaceuticals and personal care products in a membrane bioreactor wastewater treatment plant. Water Sci. Technol. 69, 2221–2229. doi: 10.2166/wst.2014.145
Korekar, G., Kumar, A., and Ugale, C. (2019). Occurrence, fate, persistence and remediation of caffeine: a review. Environ. Sci. Pollut. Res. Int. 19:7. doi: 10.1007/s11356-019-06998-8
Larcher, S., and Yargeau, V. (2012). Biodegradation of sulfamethoxazole: current knowledge and perspectives. Appl. Microbiol. Biotechnol. 96, 309–318. doi: 10.1007/s00253-012-4326-3
Letsinger, S., Kay, P., Rodríguez-Mozaz, S., Villagrassa, M., Barceló, D., and Rotchell, J. M. (2019). Spatial and temporal occurrence of pharmaceuticals in UK estuaries. Sci. Total Environ. 678, 74–84. doi: 10.1016/j.scitotenv.2019.04.182
Li, Z., Gomez, E., Fenet, H., and Chiron, S. (2012). Chiral signature of venlafaxine as a marker of biological attenuation processes. Chemosphere 90, 1933–1938. doi: 10.1016/j.chemosphere.2012.10.033
Lin, A. Y., Lin, C. A., Tung, H. H., and Chary, N. S. (2010). Potential for biodegradation and sorption of acetaminophen, caffeine, propranolol and acebutolol in lab-scale aqueous environments. J. Hazard Mater. 183, 242–250. doi: 10.1016/j.jhazmat.2010.07.017
Lishman, L., Smyth, S. A., Sarafin, K., Kleywegt, S., Toito, J., Peart, T., et al. (2006). Occurrence and reductions of pharmaceuticals and personal care products and estrogens by municipal wastewater treatment plants in Ontario, Canada. Sci. Total Environ. 367, 544–558. doi: 10.1016/j.scitotenv.2006.03.021
Martinez-Hernandez, V., Meffe, R., Herrera Lopez, S., and Bustamante, I.d. e. (2016). The role of sorption and biodegradation in the removal of acetaminophen, carbamazepine, caffeine, naproxen and sulfamethoxazole during soil contact: A kinetics study. Sci. Total Environ. 559, 232–241. doi: 10.1016/j.scitotenv.2016.03.131
Maurer, L., Villette, C., Zumsteg, J., Wanko, A., and Heintz, D. (2020). Large scale micropollutants and lipids screening in the sludge layers and the ecosystem of a vertical flow constructed wetland. Sci. Total Environ. 746:141196. doi: 10.1016/j.scitotenv.2020.141196
Munz, G., Gualtiero, M., Salvadori, L., Claudia, B., and Claudio, L. (2008). Process efficiency and microbial monitoring in MBR (membrane bioreactor) and CASP (conventional activated sludge process) treatment of tannery wastewater. Bioresour. Technol. 99, 8559–8564. doi: 10.1016/j.biortech.2008.04.006
National Library of Medicine (2014). Fact Sheet: Hazardous Substances Data Bank (HSDB). Available online at: http://www.nlm.nih.gov/pubs/factsheets/hsdbfs.html (accessed 24, 09.14).
Qingling, Z., Yongmei, L., and Guowei, G. (2009). Effect of Temperature on the Sorption of 17α-ethinylestradiol to Aerobic and Anaerobic Sludges. Int. Conferen. Energy Environ. Technol. 9, 294–297. doi: 10.1109/ICEET.2009.536
Reis, E. O., Foureaux, A. F. S., Rodrigues, J. S., Moreira, V. R., Lebron, Y. A., Santos, L. V., et al. (2019). Occurrence, removal and seasonal variation of pharmaceuticals in Brasilian drinking water treatment plants. Environ. Pollut. 250, 773–781. doi: 10.1016/j.envpol.2019.04.102
Ren, Y. X., Nakano, K., Nomura, M., Chiba, N., and Nishimura, O. (2007). A thermodynamic analysis on adsorption of estrogens in activated sludge process. Water Res. 41, 2341–2348. doi: 10.1016/j.watres.2007.01.058
Rua-Gomez, P. C., and Puttmann, W. (2012). Occurrence and removal of lidocaine, tramadol, venlafaxine, and their metabolites in German wastewater treatment plants. Environ. Sci. Pollut. Res. Int. 19, 689–699. doi: 10.1007/s11356-011-0614-1
Sahar, E., Messalem, R., Cikurel, H., Aharoni, A., Brenner, A., Godehardt, M., et al. (2011). Fate of antibiotics in activated sludge followed by ultrafiltration (CAS-UF) and in a membrane bioreactor (MBR). Water Res. 45, 4827–4836. doi: 10.1016/j.watres.2011.06.023
Sipma, J., Osuna, B., Collado, N., Monclús, H., Ferrero, G., Comas, J., et al. (2010). Comparison of removal of pharmaceuticals in MBR and activated sludge systems. Desalination 250, 653–659. doi: 10.1016/j.desal.2009.06.073
Suárez, S., Reif, R., Lema, J. M., and Omil, F. (2012). Mass balance of pharmaceutical and personal care products in a pilot-scale single-sludge system: influence of T, SRT and recirculation ratio. Chemosphere 89, 164–171. doi: 10.1016/j.chemosphere.2012.05.094
Tadkaew, N., Hai, F. I., McDonald, J. A., Khan, S. J., and Nghiem, L. D. (2011). Removal of trace organics by MBR treatment: the role of molecular properties. Water Res. 45, 2439–2451. doi: 10.1016/j.watres.2011.01.023
Terada, A., Usui, H., Bao, Q., Nakai, S., and Hosomi, M. (2017). Feasibility of biodegradation of clarithromycin by Nitrosomonas europaea. Kagaku Kogaku Ronbunshu 43, 264–270. doi: 10.1252/kakoronbunshu.43.264
Tiwari, B., Sellamuthu, B., Ouarda, Y., Drogui, P., Tyagi, R. D., and Buelna, G. (2016). Review on fate and mechanism of removal of pharmaceutical pollutants from wastewater using biological approach. Bioresourc. Technol. 224, 1–12. doi: 10.1016/j.biortech.2016.11.042
Tiwari, B., Sellamuthu, B., Piché-Choquette, S., Drogui, P., Tyagi, R. D., Buelna, G., et al. (2021). Compositional microbial-community shift of submerged membrane bioreactor treating hospital wastewater at varying temperatures. J. Environ. Eng. 147:04020152. doi: 10.1061/(ASCE)EE.1943-7870.0001842
Tiwari, B., Sellamuthu, B., Piche-Choquette, S., Drogui, P., Tyagi, R. D., Vaudreuil, M. A., Sauve, S., Buelna, G., and Dube, R. (2019). The bacterial community structure of submerged membrane bioreactor treating synthetic hospital wastewater. Bioresourc. Technol. 286:121362. doi: 10.1016/j.biortech.2019.121362
Vieno, N., and Sillanpaa, M. (2014). Fate of diclofenac in municipal wastewater treatment plant—a review. Environ Int. 69, 28–39. doi: 10.1016/j.envint.2014.03.021
Keywords: removal mechanism, biodegradation, sorption, hospital wastewater, submerged membrane bioreactor
Citation: Tiwari B, Ouarda Y, Drogui P, Tyagi RD, Vaudreuil MA, Sauvé S, Buelna G and Dubé R (2021) Fate of Pharmaceuticals in a Submerged Membrane Bioreactor Treating Hospital Wastewater. Front. Water 3:730479. doi: 10.3389/frwa.2021.730479
Received: 25 June 2021; Accepted: 29 November 2021;
Published: 23 December 2021.
Edited by:
Yizi Shang, China Institute of Water Resources and Hydropower Research, ChinaReviewed by:
Xuan-Thanh Bui, Ho Chi Minh City University of Technology, VietnamYijing Shi, South China Normal University, China
Copyright © 2021 Tiwari, Ouarda, Drogui, Tyagi, Vaudreuil, Sauvé, Buelna and Dubé. This is an open-access article distributed under the terms of the Creative Commons Attribution License (CC BY). The use, distribution or reproduction in other forums is permitted, provided the original author(s) and the copyright owner(s) are credited and that the original publication in this journal is cited, in accordance with accepted academic practice. No use, distribution or reproduction is permitted which does not comply with these terms.
*Correspondence: Patrick Drogui, patrick.drogui@ete.inrs.ca