- 1Graduate School of Life and Environmental Sciences, Kyoto Prefectural University, Kyoto, Japan
- 2Department of Aquaculture and Fisheries, Faculty of Livestock, Fisheries and Nutrition, Wayamba University of Sri Lanka, Makandura, Sri Lanka
- 3Department of Civil Engineering, University of Peradeniya, Peradeniya, Sri Lanka
- 4College of Fisheries and Aquatic Sciences, Western Philippines University Puerto Princesa Campus, Puerto Princesa, Philippines
- 5Graduate School of Agriculture, Meijo University, Nagoya, Japan
- 6National Institute of Animal Health, National Agriculture and Food Research Organization, Ibaraki, Japan
- 7Graduate School of Life and Environmental Sciences, Osaka Prefecture University, Osaka, Japan
- 8National Institute of Fundamental Studies, Kandy, Sri Lanka
In this study, the composition and richness of bacterial communities in treated and untreated wastewater from hospitals, commercial, and non-commercial fish farming sites, sewage effluents, and surface waters, which included seawater and fresh water in Sri Lanka and the Philippines, were investigated through 16S rRNA gene amplicon sequence analysis. Firmicutes were found predominantly in Sri Lankan hospital wastewaters, while Cyanobacteria and Acidobacteria were typically detected in fish culture sites and the waste canal in Sri Lanka, respectively. The Shannon–Weaver index (SW) and number of Operational Taxonomic Units (OTUs) were higher in the Philippines than in Sri Lanka. The bacterial richness in the university non-commercial fish pond and sewage effluent displayed greater than that in hospital wastewaters. In addition, the bacterial richness was higher in the untreated wastewater compared to that in the treated wastewater in hospitals. These results indicate the differences among water types in terms of bacterial community, especially influenced by their source.
Introduction
Water is one of the most important and essential resources for living organisms in the world. However, uncontaminated water is a basic necessity for humans. Contamination of water resources due to anthropogenic activities is common throughout the world (Khatri and Tyagi, 2015), particularly by chemicals and microorganisms. Integrating knowledge from multiple fields such as hydrology, microbiology, and ecology would increase the understanding of pollution levels and potential causes of pollution (Pandey et al., 2014). Surface, groundwater, and chlorinated urban water in some cities are contaminated with bacteria levels regarded as unsafe as per the standards for potable water (Onyango et al., 2018). There is a growing need to develop a strategy for recognizing potential emerging waterborne pathogens. An understanding of disinfectant action and microbial resistance to treatment processes is required to better identify those pathogens likely to be of greatest concern (Ashbolt, 2015). Likewise, it is important to have a more relevant and faster indicator for microbial contamination detection in water (Jung et al., 2014).
In a typical tropical country like Sri Lanka, bacterial diseases are frequently linked to the consumption of drinking water contaminated with Shigella spp., Salmonella spp., and Campylobacter spp. A recent study revealed that water in one of the major river basins in Sri Lanka (Kelani river basin) is not suitable for direct consumption as drinking water without proper treatment (Mahagamage et al., 2016). The Kelani river water in Sri Lanka is not only contaminated with Escherichia coli but also other species that are resistant to more than one antibiotic. Antibiotic resistance is probably due to the significant seasonal variations and environmental changes (Kumar et al., 2020). The majority (90%) of public water sources (well-water) in the Northern Province of Sri Lanka are microbiologically unsuitable for consumption due to microbial contamination (Arulnesan et al., 2015). The coastal areas of the Ampara district of the eastern area of the country face a lot of challenges induced by water-borne diseases due to pathogenic contamination (Ameer, 2017). Recently, Guruge et al. (2021) reported a wide range of antimicrobials with high concentrations of clarithromycin, sulfamethoxazole, and sulfapyridine in the hospital wastewaters in Sri Lanka. Approximately 61% of the examined E. coli isolates in those samples were categorized as multidrug-resistant bacteria.
Practices of open defecation, unhygienic practices, livestock feces, and latrine sources have a significant correlation to contaminated water sources (Gwimbi et al., 2019). Pathogens that are present in the aquatic environment may cause various diseases to people by ingestion of contaminated drinking water. The concentration of selected antimicrobials, the occurrence of resistant E. coli, and resistance genes in hospital wastewater and adjacent surface water were previously reported (Guruge et al., 2021). However, details regarding the bacterial community in such wastewaters in Sri Lanka are not well-documented. Therefore, the main objective of this study was to obtain preliminary information on the microbial community structure in wastewaters, urban waterways, lakes, and aquaculture facilities in Sri Lanka. Several wastewaters and freshwater samples previously studied for antimicrobials were included in the present study. For comparison, few samples from similar environmental settings from the Philippines were also investigated.
Materials and Methods
Water Samples
Several types of water samples were obtained from Sri Lanka such as untreated and treated wastewaters from three hospitals, five commercial ornamental fish culture sites, one non-commercial university fishpond, one lake, and an urban waste canal receiving treated effluents from the above hospitals. The samples from the Philippines were included with three sewage effluents and seawater from a bay that receives those effluents. Grab water samples were collected in clean 500-ml polypropylene bottles between 2018 and 2019 (Guruge et al., 2021). Details about the sampling are described in Table 1. Unfiltered samples were maintained in a freezer (−18°C) before the DNA extraction. A total of 17 samples were analyzed.
Amplicon Gene Analysis
A 0.25-ml aliquot was taken from each sample to extract the DNA by the extraction buffer method (Kageyama et al., 2003) and purified using the Promega PCR purification Kit (Promega Co., USA). The DNA concentration was verified by nanodrop, where the minimum of 5 ng/μl of DNA concentration was established to proceed with the amplicon PCR. The 16S rRNA gene sequences were analyzed by amplicon PCR using a primer pair V3/V4 (Klindworth et al., 2013). The forward primer sequence was V3 (5′-CCTACGGGNGGCWGCAG-3′) and the reverse was V4 (5′-GACTACHVGGGTATCTAATCC-3′) with the overhang adapter added (Fluidigm Co., USA) followed by library construction with Fluidigm barcodes. Then, the library was sequenced at Genome Quebec, Co., Canada using Illumina MiSeq 250 bp, and the data on amplicon sequencing analysis were performed using the Qiime2-2019.10 pipeline (https://qiime2.org/) (Bolyen et al., 2018). This was used to assess the relative abundance of bacterial phyla, richness, and principal coordinate analysis (PCoA) of water samples. For the metataxonomic classification, bacterial DNA was assessed using the classifier gg-13-8-99-515-806-nb-classifier.qza from the Green genes database, whose assignment was carried out by the Basic Local Alignment Search Tool (BLAST). All the metagenome sequences were registered in the DNA Data Bank of Japan (DDBJ) (http://www.ddbj.nig.ac.jp), accession number DRA012530.
Statistical Analysis
One-way ANOSIM (analysis of similarities based on number of OTUs) was performed based on non-parametrical tests, to evaluate the effects of treatments and location on bacterial community structure in the PCoA. The correlation between the concentration of antimicrobials and the prevalence of the bacterial community was determined by Pearson analysis. The antimicrobial concentrations in the samples from Sri Lanka (SL-1 to SL-8) were retrieved from our previous study (Guruge et al., 2021).
Results and Discussion
Bacterial Community Analysis
The total number of sequences obtained from all water samples was 2,391,573 with 3,466 features and 780,768 of total frequency, and the overall quality trim length was standardized at 230 bp, using the training process of SKLEARN based on k-mers, value 7, as it is the default balanced QIIME 2 parameter. The results among the bacterial community indicated on a scale from highest to lowest that Proteobacteria and Actinobacteria were the most common phyla among the samples based on relative abundance (RA). Lower RA of Proteobacteria was found in the Sri Lanka lake, whereas Actinobacteria was detected in a wide range of sources. Actinobacteria showed the lowest RA in samples from the three Sri Lanka hospitals and in the Philippine waste effluents. Furthermore, Firmicutes were found mostly in the Sri Lanka hospital sample while Cyanobacteria and Acidobacteria were typically present in the Sri Lanka fish farming sites and waste canals, respectively. Chloroflexi was found in almost all sources except for the hospitals. Planctomycetes were strongly detected in the Sri Lanka lake sample as well (Figure 1).
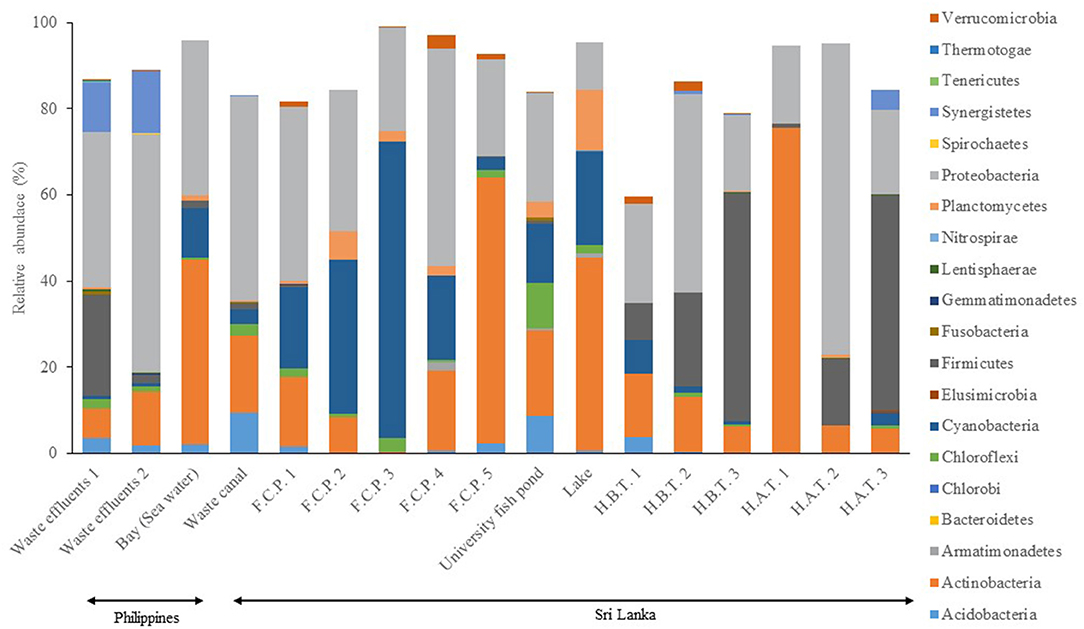
Figure 1. Distribution (relative abundance) of bacterial phylum in water samples. FCP, Commercial Fish Culture Pond; HAT, Hospital Wastewater (After Treatment); HBT, Hospital Wastewater (Before Treatment). The information of sample type is available in Table 1.
Proteobacteria, Actinobacteria, and Acidobacteria are ubiquitous in several environments, such as soils, food, and animal feces; thus, it is expected to occur in wastewaters, too. The lakes, fish culture ponds, and hospitals in Sri Lanka have low Proteobacteria, and this may be due to the low biochemical oxygen demand (BOD) of these environments. In addition, the high abundance of Cyanobacteria in the Sri Lanka fish culture pond could indicate the strong photosynthetic activity in these waters, which could later lead to a healthy and thriving fish community. Each environment could be characterized by a “fingerprint”; i.e., specific bacteria phyla is unique to that particular location. For example, Verrucomicrobia was unique to hospital waste effluents in Sri Lanka and the Philippines, while Gemmatimonadetes was unique to the waste canal and university fish pond samples in Sri Lanka. The abundance of the Methylotenera genus in wastewater is usually associated with the presence of methanol and nitrate from industrial sources (Kalyuhznaya et al., 2009).
Nitrospirae and Chloroflexi are the main bacteria indicators for nitrite oxidation and denitrification (Qin et al., 2018). The increase in salt concentration of water bodies may lead to lower bacterial richness because of the selection pressure of this element. A similar effect may be attributed to heavy metals such as mercury, arsenic, and cadmium in soil and marine sediments (Li et al., 2017; Ou et al., 2018). The differences in RA could be clearly noted on Alphaproteobacteria and Betaproteobacteria phyla. These phyla could accumulate polyhydroxyalkanoate, in wastewater, which could have a strong effect on niche speciation (Oshiki et al., 2013). This chemical has been detected in other environments as well such as compost, river biofilm, and freshwater; thus, similar effect patterns could be extended to other environments, including those in the current research. Hospital wastewater could have distinguished bacterial species more as compared to domestic ones (Ahn and Choi, 2016), with particular regard to Bacterioidetes and Proteobacteria.
Usually, the source of wastewater determines the bacterial community, and the presence of antibiotics can have a strong influence in this regard (Guruge et al., 2021). In the case of hospital samples from Sri Lanka, the orders Bifidobacteriales and Coriobacteriales had a positive correlation with chloramphenicol. Additionally, Lactobacillales, Clortridiales, and Victivalles had correlations with the fluoroquinolone antibiotics such as norfloxacin, levofloxacin, and ciprofloxacin (Table 2). This could suggest the strong effect of these bacterial groups on water chemical composition. The bacterial community could be distinct in the influent and effluent of wastewater treatment systems. Some harmful ones may be reduced during treatment, but treatment might still release other highly resistant pathogenic bacteria into the environment (Numberger et al., 2019). Moreover, other species such as Cyanobacteria are controlled by pH and nitrate (Wei et al., 2014) while Microcystis is controlled by temperature (Ji et al., 2018). Therefore, monitoring the nutrient input ccould provide a way to control the bacterial community in lakes (Zhang et al., 2019). Such factors could explain the highest abundance of Cyanobacteria in lakes, fish cultures, and bays as compared to hospital wastes.
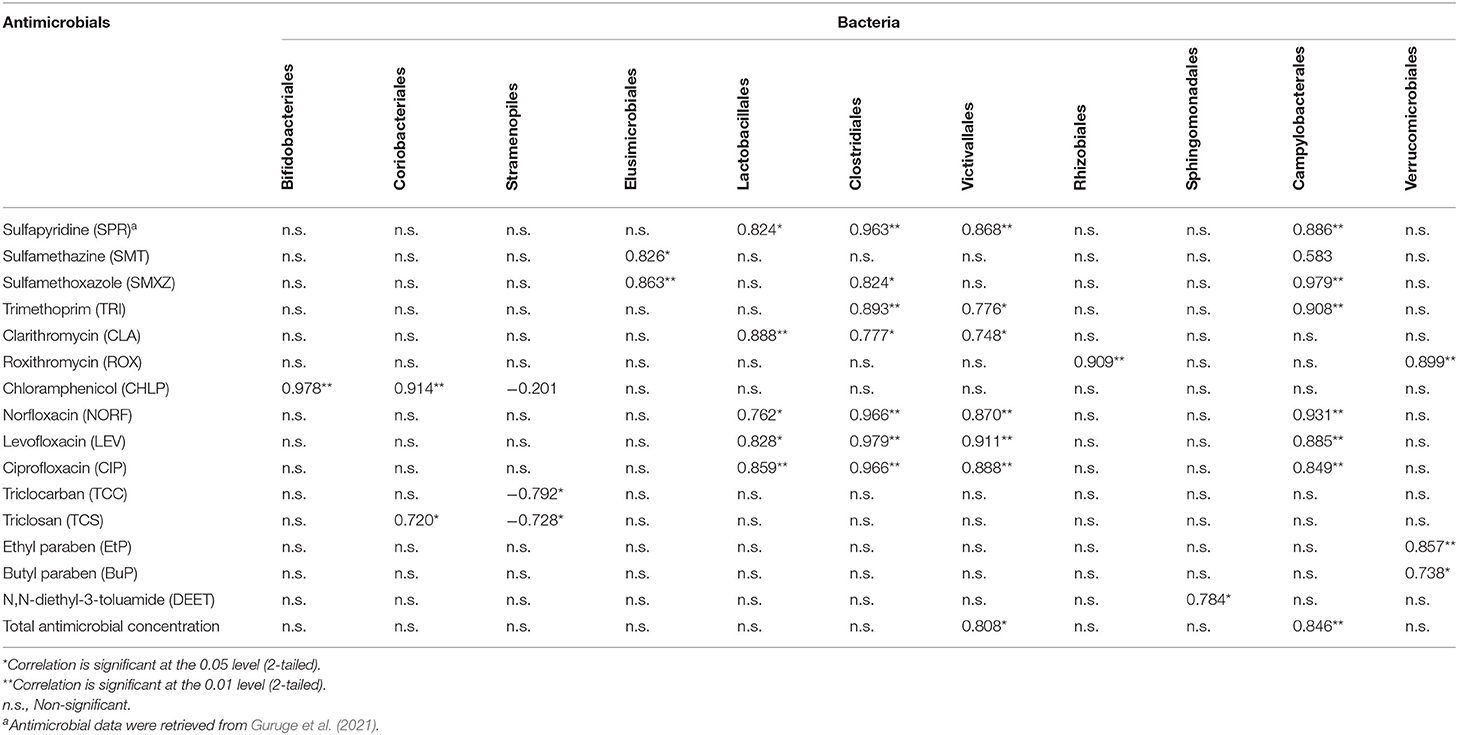
Table 2. Correlations between relative abundance of bacteria (Order level) and concentrations (ng/L) of antimicrobialsa in SL1–SL 8 samples.
Previous studies have shown the influence of environmental parameters such as pH, temperature, salinity, and nutrient status on the microbial community in these waters (Swan et al., 2010; Ganzert et al., 2014). The bacterial community could be responsive to environmental changes in Lake Chaohu, China, especially with regard to the differences between water and sediments (Zhang et al., 2019). Microorganisms have potential roles in nutrient biodynamics, pollutant degradation, and transformation in organic matter; hence, they can be used as indicators of watershed quality in the ecosystems (Wei et al., 2008; Chen et al., 2018).
Richness Estimation
The diversity estimation was according to the Shannon–Weaver index (SW), which compared two countries and types of water. The SW was greater in the Philippines than in Sri Lanka (Figure 2), whereas such values were found higher in the University fish pond and Waste Effluents and lower at Hospital 1 After treatment and Fish culture 1. In general, the SW of water from the hospital is greater than from fish farming sites; additionally, within the hospital samples, those before treatment have greater SW. However, some variations between these two treatments were apparent. The order of SW from highest to lowest follows the sequence: University fishpond > Waste effluents > H3AT > H3BT > Waste canal > Lake > H2BT > FC4 > FC1 > H2BT > Bay > H1BT > FC5 > FC2 > FC3 = H1AT (AT—After Treatment; BT—Before Treatment; FC—Fish Culture) (Figure 2). A similar pattern for the number of Operational Taxonomic Units (OTUs), standardized at 6,000 sequences, was found with a greater number in the Philippines than in Sri Lanka (Figure 2). In addition, the comparison between the types of water indicated the highest number of OTUs in fish ponds and waste effluents and lowest in H1AT and FC2. The number of OTUs followed this sequence: University fish pond > Waste effluents > H3BT > Waste canal > H3AT > Lake > Bay > FC1 = FC3 > H2BT > FC5 > FC4 > H2AT > H1BT > H1AT = FC2 (Figure 2). The PCoA graph clearly indicated a significant separation between Philippine and Sri Lanka samples. The fish culture samples could also be distinguished from the three samples from hospitals (Figure 3). Such differences were confirmed by the ANOSIM data.
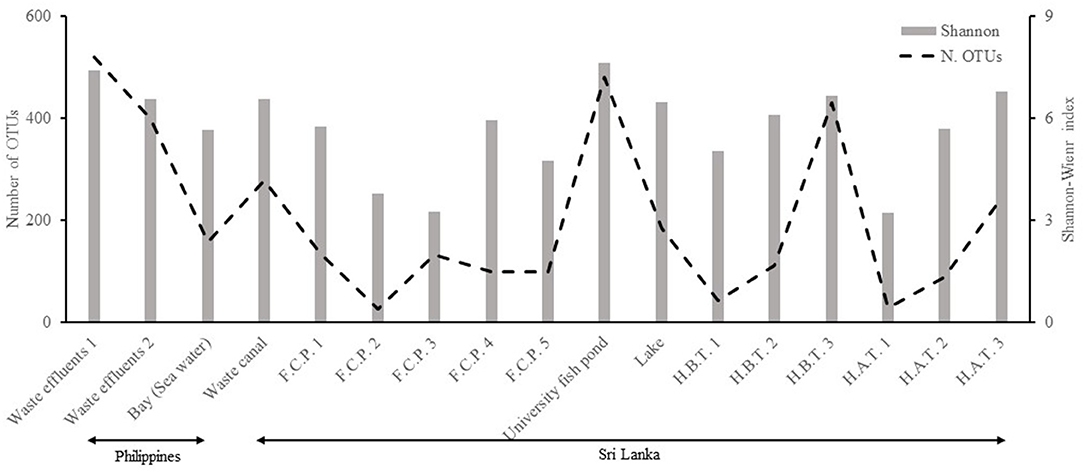
Figure 2. Bacterial richness (Shannon–Weaver index and Observed number of operational taxonomic units—OTUs) of water samples. FCP, Commercial Fish Culture Pond; HAT, Hospital Wastewater (After Treatment); HBT, Hospital Wastewater (Before Treatment). The information of sample type is available in Table 1.
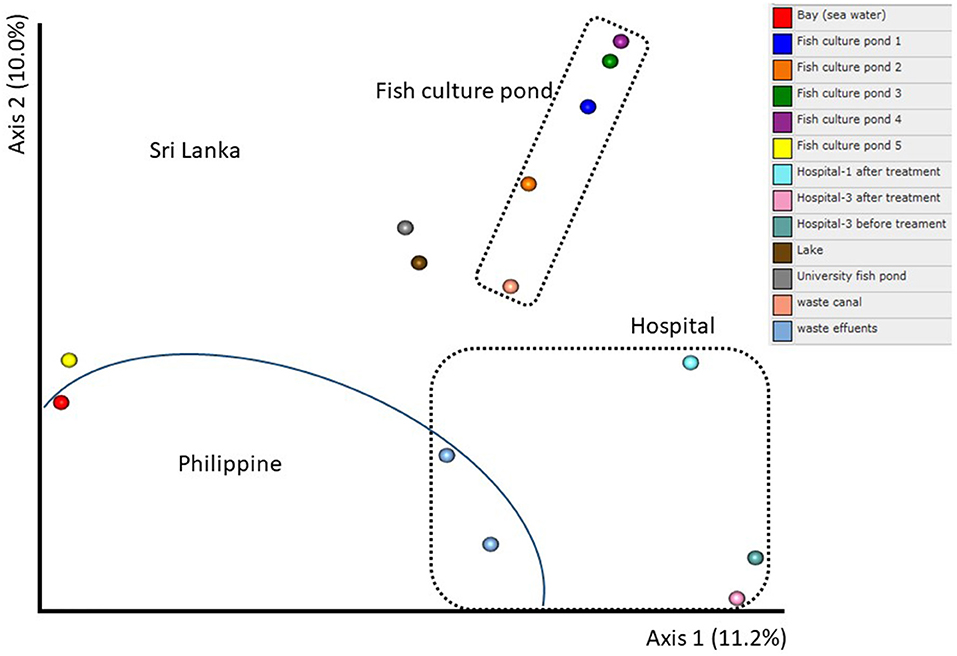
Figure 3. Principal coordinate analysis (PCoA) of 16S rRNA genes in water samples according to the Bray–Curtis method. The information of sample type is available in Table 1.
The richness data indicated some low variation among environments (hospital, fish culture). The higher diversity in fish farming sites as compared to hospital wastewater could be explained in terms of the contribution of the fish community in maintaining the local microflora, although the biochemical oxygen demand might be higher in these areas. Fish culture demands a significant oxygen requirement, but they could improve the bacterial diversity in these environments.
Normally, the industrial wastewater exhibits lower bacterial richness than other sources, with particular concern to Nitrospira populations. This suggests the effect of these influents on nitrification and denitrification (Yang et al., 2020).
Bacterial richness is considered a crucial factor to monitor the quality of water since the diversity is thought to improve the defense and recovery ability of the ecosystem against disturbances (Zinger et al., 2012). Hospital wastewaters are full of antibiotic-resistant bacteria as compared to others, suggesting that the presence of pharmaceuticals could affect and determine the bacterial community in these waters (Akiba et al., 2015; Guruge et al., 2021). Wastewater can affect the sediment bacterial community by the nutrient and organic loading they carry, consequently altering the local habitat atmosphere (Saarenheimo et al., 2017) by driving the increase in carbon availability (Garnier et al., 1992). The characterization of the bacterial community in water as a response to change the environment would provide a valuable assessment of the aquatic microbial ecology and their risks (Wang et al., 2016). The distribution and composition of the bacterial community in water were greatly affected by time and space with special regard to seasonal effects (Zhang et al., 2019).
Data Availability Statement
The original contributions presented in the study are included in the article/supplementary material, further inquiries can be directed to the corresponding authors. And I detected the following words and expressions: Global list: DNA Data Bank of Japan, Illumina, 16s rRNA, Library construction.
Author Contributions
AC: data analysis. RW: text review. KJ: data supplier. BG: literature review. TO: data discussion and conclusions. KG: experimental design. All authors contributed to the article and approved the submitted version.
Funding
This research was partially supported by the Grant-in-Aid for Scientific Research (B) [Grant no. 17H01865].
Conflict of Interest
The authors declare that the research was conducted in the absence of any commercial or financial relationships that could be construed as a potential conflict of interest.
Publisher's Note
All claims expressed in this article are solely those of the authors and do not necessarily represent those of their affiliated organizations, or those of the publisher, the editors and the reviewers. Any product that may be evaluated in this article, or claim that may be made by its manufacturer, is not guaranteed or endorsed by the publisher.
References
Ahn, Y., and Choi, J. (2016). Bacterial communities and antibiotic resistance communities in a full-scale hospital wastewater treatment plant by high-throughput pyrosequencing. Water 8:580. doi: 10.3390/w8120580
Akiba, M., Senba, H., Otagiri, H., Prabhasankar, V. P., Taniyasu, S., Yamashita, N., et al. (2015). Impact of wastewater from different sources on the prevalence of antimicrobial-resistant Escherichia coli in sewage treatment plants in South India. Ecotoxicol. Environ. Saf. 115, 203–208. doi: 10.1016/j.ecoenv.2015.02.018
Ameer, M. L. (2017). Water-Borne diseases and the their challenges in the coastal of Ampara District in Sri Lanka. World News Nat. Sci. 9, 7–18.
Arulnesan, C. A., Kamsan, S., Rajeshkannan, N., Sivapalan, K., and Murugananthan, K. (2015). Microbial Contamination of Public Drinking Water Sources and Associated Factors in Kopay Medical Officer of Health area Jaffna. Sri Lanka.
Ashbolt, N. J. (2015). Microbial contamination of drinking water and human health from community water systems. Curr. Environ. Heal. Rep. 2, 95–106. doi: 10.1007/s40572-014-0037-5
Bolyen, E., Rideout, J. R., Dillon, M. R., Bokulich, N. A., Abnet, C., Al-Ghalith, G. A., et al. (2018). QIIME 2: Reproducible, Interactive, Scalable, and Extensible Microbiome Data Science, PeerJ Preprints.
Chen, J., Wang, P., Wang, C., Wang, X., Miao, L., Liu, S., et al. (2018). Bacterial communities in riparian sediments: a large-scale longitudinal distribution pattern and response to dam construction. Front. Microbiol. 9:999. doi: 10.3389/fmicb.2018.00999
Ganzert, L., Bajerski, F., and Wagner, D. (2014). Bacterial community composition and diversity of five different permafrost-affected soils of Northeast Greenland. FEMS Microbiol. Ecol. 89, 426–441. doi: 10.1111/1574-6941.12352
Garnier, J., Servais, P., and Billen, G. (1992). Bacterioplankton in the Seine River (France): impact of the Parisian urban effluent. Can. J. Microbiol. 38, 56–64. doi: 10.1139/m92-009
Guruge, K. S., Tamamura, Y. A., Goswami, P., Tanoue, R., Jinadasa, K. B. S. N., Nomiyama, K., et al. (2021). The association between antimicrobials and the antimicrobial-resistant phenotypes and resistance genes of Escherichia coli isolated from hospital wastewaters and adjacent surface waters in Sri Lanka. Chemosp. 279:130591. doi: 10.1016/j.chemosphere.2021.130591
Gwimbi, P., George, M., and Ramphalile, M. (2019). Bacterial contamination of drinking water sources in rural villages of Mohale Basin, Lesotho: exposures through neighbourhood sanitation and hygiene practices. Environ. Health Prev. Med. 24:33. doi: 10.1186/s12199-019-0790-z
Ji, B., Qin, H., Guo, S., Chen, W., Zhang, X., and Liang, J. (2018). Bacterial communities of four adjacent fresh lakes at different trophic status. Ecotoxicol. Environ. Saf. 157, 388–394. doi: 10.1016/j.ecoenv.2018.03.086
Jung, A.-V., Le Cann, P., Roig, B., Thomas, O., Baurès, E., and Thomas, M. F. (2014). Microbial contamination detection in water resources: interest of current optical methods, trends and needs in the context of climate change. Int. J. Environ. Res. Public Health 11, 4292–4310. doi: 10.3390/ijerph110404292
Kageyama, K., Komatsu, T., and Suga, H. (2003). Refined PCR protocol for detection of plant pathogens in soil. J. Gen. Plant Pathol. 69, 153–160. doi: 10.1007/s10327-002-0037-4
Kalyuhznaya, M. G., Martens-Habbena, W., Wang, T., Hackett, M., Stolyar, S. M., Stahl, D. A., et al. (2009). Methylophilaceae link methanol oxidation to denitrification in freshwater lake sediment as suggested by stable isotope probing and pure culture analysis. Environ. Microbiol. Rep. 1, 385–392. doi: 10.1111/j.1758-2229.2009.00046.x
Khatri, N., and Tyagi, S. (2015). Influences of natural and anthropogenic factors on surface and groundwater quality in rural and urban areas. Front. Life Sci. 8, 23–39. doi: 10.1080/21553769.2014.933716
Klindworth, A., Pruesse, E., Schweer, T., Peplies, J., Quast, C., Horn, M., et al. (2013). Evaluation of general 16S ribosomal RNA gene PCR primers for classical and next-generation sequencing-based diversity studies. Nucleic Acids Res. 41:e1. doi: 10.1093/nar/gks808
Kumar, M., Chaminda, G. G. T., and Honda, R. (2020). Seasonality impels the antibiotic resistance in Kelani River of the emerging economy of Sri Lanka. NPJ Clean Water 3:12. doi: 10.1038/s41545-020-0058-6
Li, X., Meng, D., Li, J., Yin, H., Liu, H., Liu, X., et al. (2017). Response of soil microbial communities and microbial interactions to long-term heavy metal contamination. Environ. Pollut. 231, 908–917. doi: 10.1016/j.envpol.2017.08.057
Mahagamage, M., Pathirage, M., and Manage, P. M. (2016). Microbiological Quality in Ground Water of the Kelani River Basin, Sri Lanka.
Numberger, D., Ganzert, L., Zoccarato, L., Mühldorfer, K., Sauer, S., Grossart, H. P., et al. (2019). Characterization of bacterial communities in wastewater with enhanced taxonomic resolution by full-length 16S rRNA sequencing. Sci. Rep. 9:9673. doi: 10.1038/s41598-019-46015-z
Onyango, A. E., Okoth, M. W., Kunyanga, C. N., and Aliwa, B. O. (2018). Microbiological quality and contamination level of water sources in Isiolo County in Kenya. J. Environ. Public Health 2018:2139867. doi: 10.1155/2018/2139867
Oshiki, M., Onuki, M., Satoh, H., and Mino, T. (2013). Microbial community composition of polyhydroxyalkanoate-accumulating organisms in full-scale wastewater treatment plants operated in fully aerobic mode. Microbes Environ. 28, 96–104. doi: 10.1264/jsme2.ME12141
Ou, D., Li, H., Li, W., Wu, X., and Wang, Y. (2018). Salt-tolerance aerobic granular sludge: formation and microbial community characteristics. Bioresour. Technol. 249, 132–138. doi: 10.1016/j.biortech.2017.07.154
Pandey, P. K., Kass, P. H., Soupir, M. L., Biswas, S., and Singh, V. P. (2014). Contamination of water resources by pathogenic bacteria. AMB Express 4:51. doi: 10.1186/s13568-014-0051-x
Qin, H., Ji, B., Zhang, S., and Kong, Z. (2018). Study on the bacterial and archaeal community structure and diversity of activated sludge from three wastewater treatment plants. Mar. Pollut. Bull. 135, 801–807. doi: 10.1016/j.marpolbul.2018.08.010
Saarenheimo, J., Aalto, S. L., Rissanen, A. J., and Tiirola, M. (2017). Microbial community response on wastewater discharge in boreal lake sediments. Front. Microbiol. 8:750. doi: 10.3389/fmicb.2017.00750
Swan, B. K., Ehrhardt, C. J., Reifel, K. M., Moreno, L. I., and Valentine, D. L. (2010). Archaeal and bacterial communities respond differently to environmental gradients in anoxic sediments of a California hypersaline lake, the Salton Sea. Appl. Environ. Microbiol. 76, 757–768. doi: 10.1128/AEM.02409-09
Wang, W., Wang, H., Feng, Y., Wang, L., Xiao, X., Xi, Y., et al. (2016). Consistent responses of the microbial community structure to organic farming along the middle and lower reaches of the Yangtze River. Sci. Rep. 6:35046. doi: 10.1038/srep35046
Wei, C., Bao, S., Zhu, X., and Huang, X. (2008). Spatio-temporal variations of the bacterioplankton community composition in Chaohu Lake, China. Prog. Nat. Sci. 18, 1115–1122. doi: 10.1016/j.pnsc.2008.04.005
Wei, G., Li, J., Wang, N., and Gao, Z. (2014). Spatial abundance and diversity of bacterioplankton in a typical stream-forming ecosystem, Huangqian reservoir, China. J. Microbiol. Biotechnol. 24, 1308–1318. doi: 10.4014/jmb.1403.03067
Yang, Y., Wang, L., Xiang, F., Zhao, L., and Qiao, Z. (2020). Activated sludge microbial community and treatment performance of wastewater treatment plants in industrial and municipal zones. Int. J. Environ. Res. Public Health 17:436. doi: 10.3390/ijerph17020436
Zhang, L., Zhao, T., Wang, Q., Li, L., Shen, T., and Gao, G. (2019). Bacterial community composition in aquatic and sediment samples with spatiotemporal dynamics in large, shallow, eutrophic Lake Chaohu, China. J. Freshw. Ecol. 34, 575–589. doi: 10.1080/02705060.2019.1635536
Keywords: bacterial community, hospital wastewater, fish culture sites, surface water, 16S rRNA (16S rDNA)
Citation: Cruz AF, Wijesekara RGS, Jinadasa KBSN, Gonzales BJ, Ohura T and Guruge KS (2021) Preliminary Investigation of Microbial Community in Wastewater and Surface Waters in Sri Lanka and the Philippines. Front. Water 3:730124. doi: 10.3389/frwa.2021.730124
Received: 24 June 2021; Accepted: 26 October 2021;
Published: 03 December 2021.
Edited by:
Jingqiu Liao, Columbia University, United StatesReviewed by:
Aeriel Belk, Colorado State University, United StatesJiazhang Chen, Chinese Academy of Fishery Sciences, China
Copyright © 2021 Cruz, Wijesekara, Jinadasa, Gonzales, Ohura and Guruge. This is an open-access article distributed under the terms of the Creative Commons Attribution License (CC BY). The use, distribution or reproduction in other forums is permitted, provided the original author(s) and the copyright owner(s) are credited and that the original publication in this journal is cited, in accordance with accepted academic practice. No use, distribution or reproduction is permitted which does not comply with these terms.
*Correspondence: Andre Freire Cruz, andre@kpu.ac.jp; Keerthi S. Guruge, guruge@affrc.go.jp