- Center for Urban Science and Engineering, Indian Institute of Technology Bombay, Mumbai, India
Intermittent Water Supply (IWS) is prevalent in most developing countries. Specifically, in India, IWS is existent throughout the country. Many studies focus on documenting the effects of IWS, and rarely the drivers of the IWS regime are studied. In this study, a systematic literature review was conducted on IWS studies around the globe. The various causes for IWS were documented. Then, by studying India's typical water supply system (WSS) configuration, the vicious cycle of IWS in India is discussed. Further, the drivers of IWS were identified and elaborated with the causing mechanisms. This knowledge will help devise strategies and solutions for improving the IWS in India and other developing countries with similar socio-economic conditions.
Introduction
Piped water supply is the safest way to provide potable water to consumers in adequate quantity and recommended quality. The planning, design, construction, operation, and maintenance of a Water Supply System (WSS) requires comprehensive efforts from multiple stakeholders. Due to reliable design and operation in developed countries as per the standards, Continuous Water Supply (CWS) prevails. However, the situation is diametrically opposite in developing countries. Although designs of WSSs are as per stipulated standards and manuals, the operation of the WSS is not as expected in design (Abu-Madi and Trifunovic, 2013). Especially in the South Asian countries, 100% Intermittent Water Supply (IWS) exists (Charalambous and Laspidou, 2017). Typically, in India, the design is based on the CWS guidelines, and the system operates as the IWS. A piped water supply distributing water to consumers for less than 24 h a day is termed as IWS (Mokssit et al., 2018).
In India, all the WSSs are operated as an IWS, with supply duration varying from 1 to 6 h/day (Ahluwalia et al., 2011). The IWS results in inequitable supply, increased Non-Revenue Water (NRW), and deteriorated water quality. Most of the WSS in India fail to comply with the service level benchmark (Table 1). The standard is based on Central Public Health and Environmental Engineering Organization (CPHEEO) norms, an advisory board to the Ministry of Urban Development, Government of India.
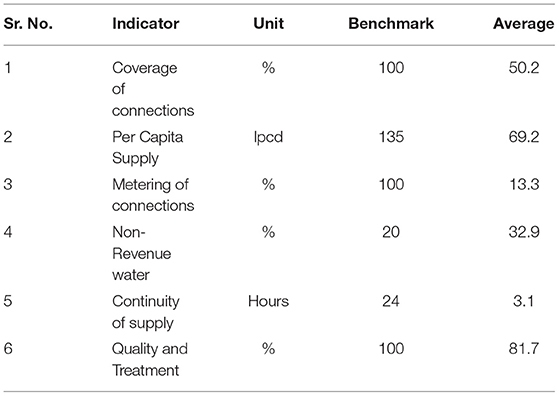
Table 1. Service level benchmarks for water supply in India (MoUD, 2012).
Many past studies (Klingel, 2012; Galaitsi et al., 2016; Kumpel and Nelson, 2016; Mokssit et al., 2018; Simukonda et al., 2018) have focused on the causes and the consequences of IWS worldwide. Table 2 illustrates the past review studies on IWS, which depicts the studies' objective and significant findings. Additionally, few studies (Andey and Kelkar, 2009; Kumpel and Nelson, 2013) reported the comparison of CWS and IWS with the study area as India. Another set of studies (Elala et al., 2011; Mellor et al., 2016) reported the IWSs impact on water quality in India. Apart from reporting the causes and consequences of the IWS, studies reported the methodology for the direct transition of IWS to the CWS (Jayaramu et al., 2015; Hastak et al., 2017; Burt et al., 2018). However, these studies were based on the pilot scale level, and achieving CWS throughout the WSS is a distant dream in developing countries (Mokssit et al., 2018; Kalbar and Gokhale, 2019).
Limited literature is available on the improvement of existing IWS. To solve the IWS issues, understanding the root cause of the intermittency is of prime importance. The causes and consequences of IWS enlisted in the past literature are primarily generic. A detailed understanding of the interaction between various drivers responsible for IWS is necessary to propose interventions for improving existing IWS. To the best of our knowledge, a comprehensive description of various drivers responsible for IWS in India is missing in the literature. The present study captures the different design, operation, economic, and institutional arrangement issues of WSS responsible for IWS in India. Based on the field condition and operational hydraulics, various drivers of IWS are discussed. It is hoped this study will bring more clarity toward understanding the drivers responsible for IWS in India.
The paper includes five sections. After this introduction, the second section is about the review methodology adopted in the study. The third section is focused on the analysis of various studies on IWS. The drivers for IWS in India are discussed in section four. Finally, the conclusion of the study is reported in section Vicious cycle of WSS failure in India.
Review Methodology
In this study, only peer-reviewed journal articles were selected as the novelty of work is given priority in the peer-reviewed journal articles (Owens et al., 2020). The journal articles were retrieved from the Scopus database. The search string used was “Intermittent Water Supply,” and the search was conducted for “Article title, abstract, and keywords.” A total of 1080 articles were reported from 1873 to 2020, and all the articles were screened in detail. The first screening level was based on reading the title, abstract, keywords, and excluding the duplication, which resulted in 201 publications. A detailed reading of the title, abstract, introduction, and conclusion was done in the second level of screening, and 91 articles were selected for the detailed review.
The articles were classified based on three criteria: design and analysis, operation, and Economic aspects (revenue). The main aim of these studies was used as criteria while classifying the articles. It was observed that studies reported before 2002 (and in the years 2004 and 2005) were not entirely focusing on IWS, hence excluded from the review process. As shown in Figure 1, a substantial amount of work is done on the IWS operation aspect. As expected, a significant number of studies have considered WSS in developing countries, especially India. Additionally, based on knowledge gained through numerous field visits to WSSs across India, the existing layout, design and operational practices are discussed, followed by the drivers for IWS in India.
Studies on IWS
The past studies (91 screened articles) on IWS are discussed based on three criteria Design and Analysis, Operation, and Revenue. The bibliographic information of the articles included in the survey is provided in the Supplementary Information (Supplementary Table SI-1). The category wise summary of each study is compiled in Supplementary Tables SI-2.1–2.3 of the Supplementary Information.
Design and Analysis of IWS
Design and analysis are an essential part of WSS irrespective of the supply regime. Out of 91 articles screened, only three studies focused on a new set of design guidelines for IWS (Table 3). However, the guidelines are not available in the public domain. From the analysis point of view, most of the studies (almost 50%) focused on simulating the process of partial pipe filling in IWS. Although Pressure Driven Analysis (PDA) was used to simulate the pipeline's partial flow condition, Demand Driven Analysis (DDA) is a standard practice to simulate the WDN with IWS. Simulating partial flow conditions is one of the steps for identifying the problematic areas in the network. Once the problems are identified, the next step is to improve the existing network.
The studies (Table 3) on rehabilitation of existing networks with IWS were based on the theoretical model. Validation of the proposed theoretical models using various WDN is missing. Also, the proposed models failed to capture the actual ground reality of the IWS. Instead of studies focusing on simulating the actual ground reality of IWS, studies on direct conversion of IWS to CWS were encountered. While modeling IWS, emphasis should be given to analyzing household storage's impact on the overall network performance. Overall, a holistic design and analysis approach covering all the elements of the actual operation of WSS in developing countries is required to minimize the system's failure.
Operation of IWS
The operation of a WSS based on IWS results in various problems like increased Non-Revenue Water (NRW), inequitable supply, deterioration of water quality, etc. In relation to this, a significant amount of studies (Table 4) are reported to either quantify the problems or ways to improve the efficiency of IWS. The studies conducted regarding the operational aspect of the IWS focused on enlisting impacts of IWS, reducing the leakages, reasons for deterioration of water quality, improving the supply equity, and converting to the CWS. Among the impacts of IWS, leakage is the most widely studied area. The different components vulnerable to the leakage in the WSS and various leakage quantification techniques suitable for IWS are reported in the literature (Supplementary Table SI-2.2). However, the proposed methodologies are labor-intensive, costly, and tested on a pilot scale, resulting in replicability issues. Hence, the exact quantification of water loss in IWS remains a tedious task.
From the water quality aspect in IWS, most of the studies reported the household storage tank is the main component responsible for the deterioration of water quality. Limited studies (Supplementary Table SI-2.2) highlighted the pathway for improving the water quality in IWS.
Besides the leakage and water quality, inequitable water supply is a significant drawback in IWS. However, studies on replicable methodology to achieve equity in IWS are scarce (SI-2.2). The limited literature (Table 4) on methods to achieve equitable water supply in the IWS suggests a lack of willingness to improve the IWS's performance.
The focus is on direct conversion of IWS to CWS (Klingel and Nestmann, 2014; Jayaramu et al., 2015; Hastak et al., 2017). However, the studies focus on a city or town pilot area for conversion to CWS. The scaling up of direct conversion to the CWS throughout the city is difficult without completely understanding the ground reality and gradually improving the existing system (Kalbar and Gokhale, 2019). Moreover, the lack of consistency in defining benchmarking parameters and reliability in the data collection framework makes it challenging to utilize the benchmarking data to directly implement the interventions (Rawas et al., 2020).
Revenue in IWS
Like all other infrastructure projects, revenue generation in WSS is the driving force for successful operation of a scheme. However, the failure of WSS with IWS leads to a lack of consumer satisfaction, which minimizes willingness to pay and ultimately reduces revenue generation.
Due to the reduction in efficiency of WSS with IWS, the consumers water demand is not fulfilled. Hence, consumers spend on household storage, pumps, underground tanks to store water in IWS. The additional expenditure on the solution mentioned above is termed as coping cost to consumers (McIntosh, 2003). In relation to coping costs, most of the studies (Supplementary Table SI-2.3) classified the various expenditure consumer have to incur on various components to satisfy the demand in IWS. Moreover, factors responsible for the willingness to pay from the consumer end were discussed in the literature. From service providers point of view, exact quantification of water consumption is vital to generate appropriate water bills. In IWS, accurate determination of water consumption is difficult to achieve (Jayaramu et al., 2016; Kumpel et al., 2017). A methodology to accurately quantify the household's actual consumption with IWS was proposed by Guragai et al. (2018) using data loggers in Kathmandu, Nepal. However, the scaling up of the proposed methodology is difficult, as a considerable investment is required to install and maintain the data loggers at a larger scale.
IWS in India
This section describes the configuration, design norms, and the actual operation of WSSs in India. The actual ground reality will help understand prevailing IWS both in urban and rural settings in India.
Configuration of WSSs in India
A typical layout of WSS with a surface water source in India is illustrated in Figure 2A. Raw water from the source is transported to the Water Treatment Plant (WTP) through gravity or using pumps based on the terrain. The pipe carrying the water from the source to WTP is typically called the Rising(pumped)/Gravity main. The treated water from WTP is then stored in a Master Balancing Reservoir (MBR). From the MBR, water is transported to different Elevated Storage Reservoir (ESR) through a pipe network known as a transmission network. Ideally, MBR is situated at a high altitude such that water is distributed to all the ESRs under gravity. Preferably, the ESR is supposed to be at the center of the serving area. Water from ESR to the consumer is delivered through a network of pipelines termed distribution network. ESR is provided with a separate inlet and outlet pipe. Different valves are provided at appropriate locations to maintain the efficiency of the system. The configuration is such that water is transported in a single direction from source to consumer.
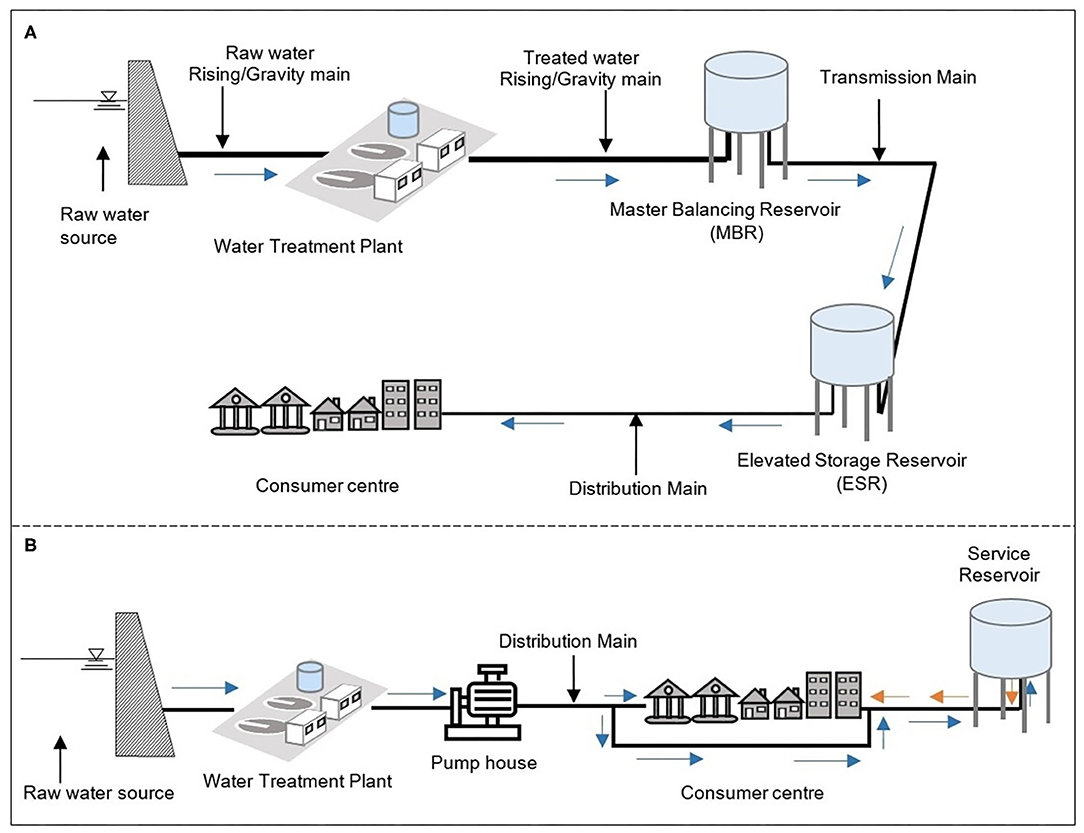
Figure 2. (A) Typical layout of WSS with a surface water source in India, (B) Recommended WSS layout by AWWA manual M31.
The difference between the WSS in developed countries and India was studied based on technical norms for water supply laid by the America Water Works Association (AWWA). As per AWWA manual M31 (AWWA American Water Works Association, 2008), the most efficient WSS configuration is one with a floating Service Reservoir (SR) situated at the end of the consumer center (Figure 2B). In other words, the consumer center should be between the pump station and SR. Water from the pumping station is pumped to both the consumer center and the SR. Unlike the ESRs in India, SR has only a single pipe to fill and withdraw water. At the time of emergency like fire demand, water is provided to the consumer center from both pump station and SR. Whereas, at the time of the off-peak period, water is pumped in SR and stored. Thus, the SR is floating on the network, with the flexibility to store and deliver water based on the consumer center's demand. As shown in Figure 2B, the WSS configuration is one of the primary reasons for its high reliability in developed countries. In addition to the layout, the design norms are an essential factor responsible for WSS's performance. The subsequent section will highlight the design norms of WSS in India and developed countries.
WSS Design Norms in India
In India, the WSSs are designed as per the CPHEEO norms. The guidelines include the per capita demand, minimum residual pressure, minimum pipe diameter, the design period of various components, peak factor, and demand pattern. Table 5 enlists the different norms as per CPHEEO and AWWA Manual M31. Even though both the standards are based on CWS, there is a gap between standards laid by two agencies. Apart from the minimum residual criteria and per capita norm, the significant difference is in fire flow demand. In the USA, the pipe diameter is designed based on the maximum day demand and fire flow demand (Walski, 2014). The pipe diameter should satisfy the combined demand with the minimum residual pressure shown in Table 5. Whereas in India, additional fire demand is considered for calculating the storage capacity of ESR. Fire demand is not included in the design of the distribution network. Moreover, Smith and Liu (2020) enlisted the minimum pressure standards around the world. The dissimilarity in the design norms between developed and developing nations makes the WSS in the developed countries less vulnerable to failure than the developing countries.
Apart from the design parameters mentioned in Table 5, the transmission network between MBR and ESR is designed for a 1.2 to 1.5 peak factor in India. On the distribution side, the consumer demand pattern is considered a diurnal pattern, peaking in the morning and evening. Population forecast is done for the design period of 30 years (CPHEEO, 1999). The various methods mentioned in the CPHEEO manual are used to arrive at the forecasted population, and per capita demand (Table 5) is multiplied to meet the future demands. MBR and ESR's capacity is finalized based on the mass curve method, based on the supply and demand pattern throughout the day. Furthermore, Demand Driven Approach (DDA) is used to design transmission and distribution networks.
The WSS design norms in India cover all the fundamental aspects. A Detailed Project Report (DPR) is formed based on the design norms and submitted to concerned authorities for design approval. The WSS construction is carried out based on the DPR, followed by its commissioning and operation. The following section will highlight the actual operation of WSS in India.
Actual Operation of WSS in India
The operation of WSS in India is intermittent, both in transmission and distribution networks. The operation is different from the design guidelines used. In the following subsection, the operation of the transmission and distribution network is discussed separately.
Operation of the Transmission System
The transmission network is designed based on CWS, assuming that all the downstream ESRs will be filled simultaneously. Conversely, the ESRs are filled in a staggered manner with the help of control valves (ON/OFF valves). A peak factor between 1.2 and 1.5 is used to design the transmission network CPHEEO, 1999. However, the transmission network's staggering operation increases the peak factor, deterioration of the pipeline, increasing the water loss.
The design life of a transmission network is 30 years CPHEEO, 1999. Accordingly, the demand is calculated, and pipe diameter is determined and commissioned at the site. The buffer capacity available at the initial phase of the design period is utilized to expand the network unscientifically. Such a scenario results in over withdrawal in the transmission network to fulfill the increased demand. The uncontrolled withdrawal causes partially filling of the ESRs especially, at the tail end ESRs.
Furthermore, to counter the ground undulations, Break Pressure Tanks (BPT) are used at a higher elevation to reduce the energy consumption by converting the pumped flow into gravity flow. However, due to the inappropriate location of BPT, the actual required discharge through the pipe is not achieved, aggravating the issue of partial filling of ESRs. Finally, illegal tapings on the main transmission line are commonly found in India. The unauthorized connections reduce the system pressure, which already fails to deliver the desired flow.
Operation of the Distribution System
In India, a large-scale centralized approach is used to design WSS (Figure 2A). Large ESRs with a capacity between 5–10 ML and 10–25 ML (Kalbar and Gokhale, 2019) are designed and constructed to cater to fluctuations between demand and supply. As the operation is based on IWS, the large storage tanks develop a perception of the operators that an infinite volume of water is available, which results in the expansion of the distribution network beyond the hydraulic capacity (influence) of the tank. Furthermore, due to land constraints in urban areas, ESRs are generally located far away from the serving area, resulting in an inefficient distribution network. The consumers at the end of network are deprived of water, and the consumers near the tank receive ample water.
The general practice for the operation of WDN is to divide the service area with the help of control valves. The supply time is bifurcated with the use of control valves. The division is based on operator experience, and the aim is to supply water to consumers irrespective of the residual pressure, resulting in inequitable distribution. Sometimes, the WDN from different storage tanks is interconnected, and it becomes difficult to demarcate the exact location of the pipelines. In other words, the concept of isolated District Metered Area (DMA) is failed at the ground level.
Moreover, as discussed in the previous section, the distribution network is designed based on the peak factor as per the diurnal demand pattern. As water is supplied to a particular area with the help of a valve for a limited time, the peak factor exerted is higher than the one considered in the design. For example, if the water is supplied to an area for 2 h a day, the actual peak factor exerted in the respective pipe network will be 12. The high peak factor results in the deterioration of pipes and increased NRW.
Vicious Cycle Of WSS Failure in India
In the previous section, the actual design and operation of WSS in India were discussed. Further, it is necessary to understand the relationship between the various aspects of WSS in India causing the existence of current IWS. As shown in Figure 3, a vicious cycle is formed between the operation and maintenance, infrastructure condition, service delivery, consumer satisfaction, and revenue aspect of a WSS. Lack of proper operation and maintenance deteriorates the infrastructure condition, which reduces the service quality in terms of quantity and quality. The decreased service quality negatively impacts consumer satisfaction; reduced consumer satisfaction results drop in revenue generation. In turn, the depleted revenues affect the operation and maintenance of the WSS, which again initiates the vicious cycle of failure.
Various factors are responsible for the formation of the vicious cycle (Figure 3). The operation and maintenance of a WSS is dependent on the aspects like asset management, DMA, network expansion, and so on. Failure of one of the factors results in the WSS's degraded operation and forms the vicious cycle. Similarly, the infrastructure condition is governed by the planning, design, and analysis practices along with the operation and maintenance of the scheme. The infrastructure condition and reliability decide the service quality of WSS and ultimately dictates consumer satisfaction.
Additionally, the coping costs govern consumer satisfaction. The revenue generation aspect relies on the tariff policy, which is influenced by social and political willingness. Furthermore, illegal connections, physical losses, and consumer satisfaction impact the revenue generation of a WSS. Finally, the shared point of all the factors is the institutional capacity of the water supply sector in India. The institutional capacity affects significant aspects like planning, design, analysis, operation, and tariff policy of a WSS. The vicious cycle (Figure 3) can be converted to a virtuous cycle if all the factors are maintained as per standards. However, understanding the different drivers of the formation of a vicious cycle is vital. The following section will discuss the drivers responsible for the failure of WSS in India.
Drivers for Vicious Cycle of WSS Failure in India
As discussed in section IWS in India, there is a gap between WSS's design and actual operation in India. The design is based on CWS, whereas the operation on IWS. The difference in the design and operation results in the failure of WSS in India. Section Vicious cycle of WSS failure in India illustrated the vicious cycle of WSS failure in India. In this section, the drivers responsible for forming a vicious cycle are discussed based on the field conditions and operational hydraulics.
Design and Analysis
In India, a highly centralized approach is adopted for planning a WSS (section Configuration of WSSs in India). The concept of the ideal location of ESR (center of the serving area) fails at the ground level due to land constraint, resulting in hydraulic failure of the WDN. Additionally, population forecast by standard methods fails to capture the uncertainties, leads to the faulty demand forecast. The design norms of WSS in India are discussed in section WSS design norms in India. The gap between the design and actual operation of WSS makes the design standard unrealistic. For example, there might be a difference between the required diameter at the tail end of the network based on intermittent operation and the minimum pipe diameter criteria as per the standards (Table 5).
Furthermore, as discussed in sections WSS design norms in India and Actual operation of WSS in India, WSS's design is based on the peak factor as per CWS. In contrast, the actual peak exerted on the network is higher due to the intermittent operation. Moreover, the per capita demand norm does not account for the changed lifestyle, especially in rural areas. The penetration of new technologies and instruments in rural areas has changed the lifestyle, increasing the per capita water demand.
The next step after the design of WSS is the analysis of the designed network. DDA is predominantly used to simulate the network. In DDA, demand at nodes is assumed to be completely satisfied irrespective of the node's residual pressure (Berardia et al., 2014). However, due to IWS in India, wide variation in the residual pressure is observed, and accordingly, the disparity in the nodal outflow is recorded. To simulate the variation in nodal flow regarding residual pressure, Pressure Driven Analysis (PDA) is preferred and used in recent times (Sayyed et al., 2015; Mahmoud et al., 2017). Even though PDA gives satisfactory results in terms of variation in nodal outflow with respect to residual pressure, it is not easy to estimate the actual withdrawal from the node. The service connection pipe in the Indian WSS is a crucial component to calculate the actual withdrawal from the system. Also, household storage is a significant component of IWS. The analysis methods adopted for WSSs in India ignore the impact of household storage and the service connection pipe. The exclusion of these two vital components fails to capture the ground reality. Hence, it is not easy to simulate the actual scenario of WSSs in India.
The factors mentioned above related to planning, design, and analysis directly impact the infrastructure condition (Figure 3). Additionally, the operation and maintenance affect the infrastructure condition; the subsequent section will discuss the drivers for unsuccessful operation and maintenance of WSS in India.
Operation and Maintenance
In India, the intermittent operation of WSS has become standard practice. As discussed in section Operation of the distribution system, the concept of DMA is failed at the actual operation of a scheme. The maintenance activity of the pipelines becomes challenging with the lack of DMA in the network (Diao et al., 2013). In addition to the maintenance activities, conducting a water audit to quantify the actual water loss becomes tedious. As discussed in section Operation of the distribution system, the transmission network's partial flow condition results in incomplete filling of the ESRs. In India, the ESR is bypassed at many places, and the transmission network is directly connected to the distribution network.
Additionally, the refilling of pipelines requires a large volume of water in every cycle of supply time. Water loss in the network is intensified due to the filling and refilling of the pipelines. The Hydraulic Grade Line (HGL) at the storage tanks is one of the governing factors for calculating the residual pressure at nodes. At the design phase, the starting HGL at the storage tank is assumed to be above the Low Supply Level (LSL). In the actual operation, after sometime, the outlet of the storage tank is partially filled due to uncontrolled withdrawal from the tanks. Hence, the HGL sets below the LSL of the tank. The difference between the actual and design HGL value negatively impacts the performance of the distribution network.
For effective operation and maintenance of infrastructure, the fundamental prerequisite is maintaining the infrastructure database. The date of the laying of the pipeline is of vital importance in WSS. In urban areas of India, most of the functional pipelines are very old. In a deteriorated condition, the lack of a proper database regarding the pipe age and rehabilitation activity exacerbates the deterioration of the network. The application of Geographical Information System (GIS) in the database management of WSS is yet to be realized by most of the authorities. The operation practice like short duration supply, lack of DMA, unplanned expansion, uncontrolled withdrawal, and lack of asset management deteriorate WSS's infrastructure condition in India.
Consumer Satisfaction
Consumer satisfaction depends upon the service quality of the WSS. The service quality is decided based on the infrastructure condition and reliability of the WSS. As discussed in the previous sections, the shortcomings in the planning, design, analysis, and operation of WSS in India decline the infrastructure condition. Even after overcoming the issues mentioned above, the improvement in service quality is not assured. The reliability of the WSS is another aspect that governs the service quality. Interrupted electricity supply, uneven population growth, seasonal variation, drought conditions, and unexpected surge in water demand (e.g., large social gatherings) hampers the reliability of the WSS.
In India's rural areas, pumps used in lifting the water from the source often fail within the design period due to interrupted electricity supply. The pump failure disrupts the operation of the scheme, aggravating existing problems of operation and maintenance. Additionally, haphazard growth, especially in urban areas, increases the load on essential utilities like water supply. However, the WSS is not designed to cater to such an increase in demand. Lack of sustainable raw water source to fulfill the increased demand is another vital issue related to WSS's reliability.
As a result of IWS, consumers have to invest in household storage tanks to fulfill the water demand until the next supply cycle. Consumers adopt an underground storage tank, overhead storage tank, large vessels, etc., as per the financial capabilities (Cook et al., 2016). In addition to the storage facility, consumers spend on the pumps to fetch water from the system due to inadequate residual pressures. In India, to counter the health issues caused by IWS, it has become common to install a household water filtration unit (Ercumen et al., 2015). Consumers with unfulfilled water demand like rural areas or slum populations in the urban area adopt alternate sources like groundwater, tanker feed water, bottled water, community water filters and fulfill their water demand. Different alternatives adopted by consumers to accomplish the water demand are known as coping strategies. The investment made by the consumers on different coping strategies is termed coping cost. In addition to the payment of water bills, consumers spend a substantial amount of their earnings on different coping strategies (Yepes et al., 2001; Cook et al., 2016; Gurung et al., 2017). Apart from the coping costs, consumers have to modify the daily schedule as per the supply hours. In most households, one of the members has to stay at home to store water in the respective supply hours, reducing the job prospect for one household member. Hence, to overcome the ill effects of IWS, consumers spend extra time and money.
The quantification of service quality can be done by monitoring the water quantity and quality received by consumers. In addition to it, the quantification of coping cost will lead to determining the service quality, in turn, consumer satisfaction. Consumer satisfaction is directly proportional to the willingness to pay toward the water bill. Further, studies (Burt and Ray, 2014) have shown that willingness to pay is more to reliable water supply than the continuous water supply.
Revenue
Revenue generation plays a vital role in the success of any infrastructure project. Water is considered the basic need for the survival of human beings. Hence, people's general perspective is that the water tariff should be as minimal as possible, or water should be provided free of cost (Aggarwal et al., 2013). However, as a significant investment is required to design, construct, operate, and maintain a WSS, revenue generation is of prime importance for the service providers. In India, the coping costs and the working hours spent on satisfying the water demand dictate the willingness to pay for water bills in a household. As discussed in section Consumer satisfaction, consumer satisfaction is not achieved in most of the WSS in India, resulting in reduced willingness to pay.
From the tariff on water supply point of view, the tariff structure varies among different cities. The tariff policy is finalized by the state government, making it a politically driven decision (Aggarwal et al., 2013). In India, the average tariff rate is below the production cost (McKenzie and Ray, 2009). For example, the Municipal Council of Greater Mumbai (MCGM) spends Rs. 20 (0.27 USD1) per thousand liters for treatment of water, whereas only Rs. 5 (0.06 USD) per thousand liters is charged to the consumers (Singh, 2021).
Furthermore, some service providers opt for flat rate tariffs others follow telescopic rates for tariff collection. A flat rate policy is adopted for unmetered connection, block tariff for metered connection. The block's price and bifurcation vary among the cities; McKenzie and Ray (2009) have enlisted the various tariff structure in major Indian cities. In a flat rate policy, the tariff is based on the size of the service connection. In contrast, the block tariff requires household water meters. However, mechanical water meters in IWS fail to quantify the exact water consumption, and after a while, the meters become defective (Walter et al., 2018). Also, the Electromagnetic and Ultrasonic water meters require high operation and maintenance costs (Research Design Standards Organization, 2015; Li and Chong, 2019). Additionally, the insufficient coverage, unequal distribution, low pressure in the WDN escalate the illegal connections, theft of water from the system. Such malpractices increase the burden of revenue loss on the utilities and the lack of exact quantification of water consumption.
Finally, automation is considered the most relevant solution for increasing the efficiency of WSSs. Different automation techniques like Programmable Logic Control (PLC), Supervisory Control and Data Acquisition (SCADA), valve actuators, level sensors, etc., are adopted at a different scale in India. However, the operation and maintenance of such high-end solutions are costly. As discussed above, the willingness to pay is directly proportional to consumer satisfaction. Hence, generating financial returns of the considerable investment in automation becomes an uphill task.
Institutional capacity
In India, as per the 73rd Amendment to the constitution supplying clean drinking water is the state's responsibility (Ministry of Jal Shakti Government of India, 2019). WSS's institutional structure in India is fragmented; different states have a varied institutional framework (Ahmed and Araral, 2019). Most of the states have the Urban Local Body (ULB) as the responsible authority for managing WSS. In contrast, others have a centralized board to manage the water supply (Government of India, 2009). An excellent institutional system has well-trained staff, a financially sustainable tariff structure, an improved revenue collection system, accountability, transparency, and public participation (McIntosh, 2003; Bakker et al., 2008). A weak institutional framework leads to the failure of WSS (Government of India, 2009). In most ULBs or water supply boards, technical and managerial skills are scarce (Klingel, 2012; Mathur, 2017). Instead of suitable capacity building at the institute level, the planning, design, and construction of WSS are outsourced to private vendors. Only the operation and maintenance of the scheme are done by ULBs or water supply boards.
The central government of India allocates funds to states for the construction of WSS. At the state level, money from various national level schemes is used to increase the number of tanks and pipelines; there is no cognizance of WSS expenditure. Separate funds are also allocated for capacity building, used for various training programs for the engineer. However, the actual issues and appropriate solutions are rarely discussed in the training programs.
Furthermore, the dynamics between two different institutions also impact the operation of WSS. In Maharashtra, India, the institutional arrangement for water supply is complex (SI-2.4). Maharashtra Jeevan Pradhikaran (MJP), a parastatal body, is responsible for planning, designing, and constructing WSS. Post construction, MJP handover the scheme to respective local bodies. ULBs are accountable for the scheme's operation and maintenance in urban areas; Zilha Parishad and Gram Panchayat in the rural area are responsible for the scheme's operation and maintenance. The relation between different institutions defines the fate of the WSS. Moreover, the dynamics of a civil servant and the engineers at various institutes also impact the water distribution (Bawa, 2011).
In addition to it, the water tariff structure fixed by the authority is unrealistic, institutions fail to recover the operating cost of the scheme (Golin et al., 2015). The application of GIS for maintaining the revenue collection database is yet to be incorporated by all the institutions. As discussed in the earlier section, lack of proper database management escalates the problem of cost recovery. Furthermore, the actual issues and appropriate solutions are rarely discussed in the training programs used for capacity building. In the wake of such a scenario, the consultant's unrealistic design gets approved, and the scheme enters the vicious cycle of failure (Figure 3).
To summarize, a robust institutional capacity will improve all aspects of WSS, including planning, design, analysis, operation, and tariff policy. The recently launched Jal Jeevan Mission (JJM) by the Government of India has focused on strengthening the institutional capacity with a four tier institutional setup at the National, State, District, and Village level. Also, strong database management and community involvement are made mandatory for states (Ministry of Jal Shakti Government of India, 2019). However, the provision for appointment of the Project Management Unit (PMU) defies the purpose. In PMU, the institutions are free to appoint consultants for the design and operation of WSS. As the focus will be on outsourcing, vendors will provide high-end solutions which are generally suitable for developed nations. The issue of the lack of appropriate interventions to improve WSS in India will remain unresolved.
Learnings and Way Forward
In the present study, a detailed analysis of drivers for IWS in India is presented. It is clear that the drivers for IWS are not independent and reinforce each other. One of the crucial aspects of IWS is how to operate the WSS considering the field conditions. As water is supplied intermittently, household storage is a common phenomenon in IWS. Additionally, over withdrawal from the network is also predominant in IWS. However, such scenarios are hardly considered during the design and planning phase, resulting in the WSS's failure. Bhave and Gupta (2000) proposed hydraulic devices such as orifice plate to restrict the over withdrawal from the WSS.
The ultimate goal of a WSS is to provide water to consumers in adequate quantity and prescribed quality in CWS mode. However, with the present scenario of operating the WSS, directly converting the IWS to CWS will result in more problems, as such approach will not tackle the drivers to IWS. This will not effectively use the investment made for achieving CWS as the WSS will again move back to IWS with such an approach of direct transition. There should be an intermediate step of improving the performance of existing IWS such that the vicious cycle of failure (Figure 3) is broken. To improve the existing IWS, the application of appropriate design and operation interventions is required. As discussed in the present study, limited studies proposed the alternative design and operation methodology for IWS. Kalbar and Gokhale (2019) described few design and operation interventions, viz. shaft, manifold, and multi-outlet tanks, for improving the efficiency of existing IWS. Furthermore, Ghorpade et al. (2021) reported multi-outlet tanks' superiority over conventional single outlet tanks in terms of hydraulic and operational aspects.
Once the performance of IWS is improved and consumer satisfaction is achieved, a gradual transition to CWS is possible. Such a transition will result in sustainable CWS. To summarize, the approach of directly converting IWS to CWS will lead to reinforcing the vicious cycle of failure. To overcome such situations, an intermediate step is necessary to bridge the gap between IWS to CWS.
Conclusions
IWS is the most common operational regime of WSSs in India. In this study, the drivers for the IWS in India were identified based on studies on field conditions and operational hydraulics. Initially, an SLR was conducted to understand the various aspects of IWS and the variety of work done to improve IWS. This was followed by a detailed study of WSS in India, including the layout, planning, design, analysis, and actual operation. It was found that the WSSs of India are trapped in the vicious cycle, wherein failure of one factor initiates the decline in the performance of another factor, and ultimately the system fails to deliver a satisfactory water supply to the consumers. Application of solutions for improving the performance of WSSs in India without understanding the vicious cycle and drivers of IWS will lead to the continuation of the vicious cycle of failure. Also, direct conversion of WSS operating in IWS mode to CWS is not recommended as it will not address the drivers for IWS and WSS will again convert back to IWS. The present study will help understand the interrelation between various drivers responsible for the vicious cycle of IWS. Once a thorough understanding of the various drivers is developed in the practitioners, they can develop strategies to overcome the vicious cycle of IWS. The success of national level missions like JJM (Rural and Urban) and any future programmes depend upon overcoming the vicious cycle of IWS.
Author Contributions
AG with significant contributions from PK designed the research idea and the basis of the content for the manuscript. AG wrote the first draft of the manuscript. AS provided inputs about the comparison of WSS between developed and developing nations. PK, AG, and AS revised and edited the manuscript. All authors contributed to the article and approved the submitted version.
Funding
The funding received under the sponsored research project from the Department of Science and Technology (DST), India—Water Technology Initiative (WTI) −2017 DST/TM/WTI/2K17/39.
Conflict of Interest
The authors declare that the research was conducted in the absence of any commercial or financial relationships that could be construed as a potential conflict of interest.
Publisher's Note
All claims expressed in this article are solely those of the authors and do not necessarily represent those of their affiliated organizations, or those of the publisher, the editors and the reviewers. Any product that may be evaluated in this article, or claim that may be made by its manufacturer, is not guaranteed or endorsed by the publisher.
Acknowledgments
The first author acknowledges the scholarship received under the Teaching Assistant Category at Indian Institute of Technology Bombay by the Ministry of Human Resource and Development (MHRD), Government of India. Technical discussions with Mr. Pradeep Gokhale, Retired Superintendent Engineer, Maharashtra Jeevan Pradhikaran, Maharashtra, India, helped to bring clarity to this work.
Supplementary Material
The Supplementary Material for this article can be found online at: https://www.frontiersin.org/articles/10.3389/frwa.2021.696630/full#supplementary-material
Footnotes
1. ^Exchange rate of currency 1 INR=0.013 USD 16th March 2021.
References
Aboelnga, H., Saidan, M., Al-Weshah, R., Sturm, M., Ribbe, L., and Frechen, F. B. (2018). Component analysis for optimal leakage management in Madaba, Jordan. J. Water Suppl. Res. Technol. 67, 384–396. doi: 10.2166/aqua.2018.180
Abu-Madi, M., and Trifunovic, N. (2013). Impacts of supply duration on the design and performance of intermittent water distribution systems in the West Bank. Water Int. 38, 263–282. doi: 10.1080/02508060.2013.794404
Agathokleous, A., Christodoulou, C., and Christodoulou, S. E. (2017). Influence of intermittent water supply operations on the vulnerability of water distribution networks. J. Hydroinform. 19, 838–852. doi: 10.2166/hydro.2017.133
Agathokleous, A., and Christodoulou, S. (2016). Vulnerability of urban water distribution networks under intermittent water supply operations. Water Resourc. Manage. 30, 4731–4750. doi: 10.1007/s11269-016-1450-3
Aggarwal, V., Maurya, N., and Jain, G. (2013). Pricing urban water supply. Environ. Urbaniz. Asia 4, 221–241. doi: 10.1177/0975425313477768
Ahluwalia, I. J., Munjee, N., Mor, N., Vijayanunni, M., Mankad, S., Lall, R., and Sankaran, H. (2011). Report on Indian urban Infrastructure and Services. Ministry of Urban Development, New Delhi, India.
Ahmed, M., and Araral, E. (2019). Water Governance in India: evidence on Water Law, policy, and administration from eight Indian states. Water 11:2071. doi: 10.3390/w11102071
Alazzeh, S., Galaitsi, S. E., Bishara, A., Al-Azraq, N., and Durant, J. L. (2019). Impacts of intermittent water supply on water quality in two Palestinian refugee camps. Water 11:670. doi: 10.3390/w11040670
Al-Ghamdi, A. S. (2011). Leakage–pressure relationship and leakage detection in intermittent water distribution systems. J. Water Supply Res. Technol. 60, 178–183. doi: 10.2166/aqua.2011.003
Al-Ghamdi, A. S., and Gutub, S. A. (2002). Estimation of leakage in the water distribution network of the Holy City of Makkah. J. Water Supply Res. Technol. 51, 343–349. doi: 10.2166/aqua.2002.0031
Al-Washali, T., Sharma, S., Al-Nozaily, F., Haidera, M., and Kennedy, M. (2019). Monitoring nonrevenue water performance in intermittent supply. Water. 11:1220. doi: 10.3390/w11061220
Al-Washali, T., Sharma, S., Lupoja, R., Fadhl, A. N., Haidera, M., and Kennedy, M. (2020). Assessment of water losses in distribution networks: Methods, applications, uncertainties, and implications in intermittent supply. Resources, Conserv. Recycl. 152:104515. doi: 10.1016/j.resconrec.2019.104515
Ameyaw, E. E., Memon, F. A., and Bicik, J. (2013). Improving equity in intermittent water supply systems. J. Water Supply Res. Technol. 62, 552–562. doi: 10.2166/aqua.2013.065
Andey, S. P., and Kelkar, P. S. (2007). Performance of water distribution systems during intermittent versus continuous water supply. J. Am. Water Works Assoc. 99, 99–106. doi: 10.1002/j.1551-8833.2007.tb08011.x
Andey, S. P., and Kelkar, P. S. (2009). Influence of intermittent and continuous modes of water supply on domestic water consumption. Water Resourc. Manage. 23, 2555–2566. doi: 10.1007/s,11269-008-9396-8
AWWA American Water Works Association (2008). Distribution System Requirements for Fire Protection, Vol. 31. Denver, CO: American Water Works Association.
Ayoub, G. M., and Malaeb, L. (2006). Impact of intermittent water supply on water quality in Lebanon. Int. J. Environ. Pollut., 26(4), 379–397. doi: 10.1504/IJEP.2006.009328
Bakker, K., Kooy, M., Shofiani, N. E., and Martijn, E. J. (2008). Governance failure: rethinking the institutional dimensions of urban water supply to poor households. World Develop. 36, 1891–1915. doi: 10.1016/j.worlddev.2007.09.015
Bautista-de los Santos, Q. M., Chavarria, K. A., and Nelson, K. L. (2019). Understanding the impacts of intermittent supply on the drinking water microbiome. Curr. Opin. Biotechnol. 57, 167–174. doi: 10.1016/j.copbio.2019.04.003
Bawa, Z. (2011). Where is the state? how is the state? Accessing water and the state in Mumbai and Johannesburg. J. Asian Afr. Stud. 46, 491–503. doi: 10.1177/0021909611403707
Berardia, L., Laucellia, D., and Giustolisia, O. (2014). Accounting for local water storages in assessing WDN supply capacity. Proc. Engi. 70, 142–151. doi: 10.1016/j.proeng.2014.02.017
Bhave, P. R., and Gupta, R. (2000). Design, performance and operation of regional rural water supply systems. J. Indian Water Works Assoc. 32, 273–278.
Bivins, A. W., Sumner, T., Kumpel, E., Howard, G., Cumming, O., Ross, I., and Brown, J. (2017). Estimating infection risks and the global burden of diarrheal disease attributable to intermittent water supply using QMRA. Environ. Sci. Technol. 51, 7542–7551. doi: 10.1021/acs.est.7b01014
Burt, Z., Ercümen, A., Billava, N., and Ray, I. (2018). From intermittent to continuous service: Costs, benefits, equity and sustainability of water system reforms in Hubli-Dharwad, India. World Develop. 109, 121–133. doi: 10.1016/j.worlddev.2018.04.011
Burt, Z., and Ray, I. (2014). Storage and non-payment: persistent informalities within the formal water supply of Hubli-Dharwad, India. Water Alternat. 7:183.
Campisano, A., Gullotta, A., and Modica, C. (2018). Using EPA-SWMM to simulate intermittent water distribution systems. Urban Water J. 15, 925–933. doi: 10.1080/1573062X.2019.1597379
Charalambous, B., and Laspidou, C. (2017). Dealing with the Complex Interrelation of Intermittent Supply and Water Losses. London: IWA Publishing.
Christodoulou, S., and Agathokleous, A. (2012). A study on the effects of intermittent water supply on the vulnerability of urban water distribution networks. Water Sci. Technol. Water Supply 12, 523–530. doi: 10.2166/ws.2012.025
Coelho, S. T., James, S., Sunna, N., Abu Jaish, A., and Chatila, J. (2003). Controlling water quality in intermittent supply systems. Water Sci. Technol. Water Supply 3, 119–125. doi: 10.2166/ws.2003.0094
Cook, J., Kimuyu, P., and Whittington, D. (2016). The costs of coping with poor water supply in rural Kenya. Water Resourc. Res. 52, 841–859. doi: 10.1002/2015WR017468
CPHEEO (1999). Manual on Water supply and Treatment. 3rd Edn. New Delhi: Ministry of Urban Development, Government of India.
Criminisi, A., Fontanazza, C. M., Freni, G., and Loggia, G. L. (2009). Evaluation of the apparent losses caused by water meter under-registration in intermittent water supply. Water Sci. Technol. 60, 2373–2382. doi: 10.2166/wst.2009.423
Cronk, R., and Bartram, J. (2018). Identifying opportunities to improve piped water continuity and water system monitoring in Honduras, Nicaragua, and Panama: evidence from Bayesian networks and regression analysis. J. Clean. Product. 196, 1–10. doi: 10.1016/j.jclepro.2018.06.017
David, C., García, M., and Navarro Gómez, C. J. (2020). Analysis of hybrid demand pattern on a water distribution network with transition from intermittent to continuous water supply in Riberas de Sacramento, Chihuahua. Water Pract. Technol. 15, 213–224. doi: 10.2166/wpt.2020.013
De Marchis, M., Fontanazza, C. M., Freni, G., La Loggia, G., Napoli, E., and Notaro, V. (2010). A model of the filling process of an intermittent distribution network. Urban Water J. 7, 321–333. doi: 10.1080/1573062X.2010.519776
De Marchis, M., Fontanazza, C. M., Freni, G., La Loggia, G., Notaro, V., and Puleo, V. (2013). A mathematical model to evaluate apparent losses due to meter under-registration in intermittent water distribution networks. Water Sci. Technol. Water Supply 13, 914–923. doi: 10.2166/ws.2013.076
Diao, K., Zhou, Y., and Rauch, W. (2013). Automated creation of district metered area boundaries in water distribution systems. J. Water Resourc. Plann. Manage. 139, 184–190. doi: 10.1061/(ASCE)WR.1943-5452.0000247
El Achi, N., and Rouse, M. J. (2020). A hybrid hydraulic model for gradual transition from intermittent to continuous water supply in Amman, Jordan: a theoretical study. Water Supply 20, 118–129. doi: 10.2166/ws.2019.142
Elala, D., Labhasetwar, P., and Tyrrel, S. F. (2011). Deterioration in water quality from supply chain to household and appropriate storage in the context of intermittent water supplies. Water Sci. Technol. Water Supply 11, 400–408. doi: 10.2166/ws.2011.064
Ercumen, A., Arnold, B. F., Kumpel, E., Burt, Z., Ray, I., Nelson, K., and Colford, J. M. Jr (2015). Upgrading a piped water supply from intermittent to continuous delivery and association with waterborne illness: a matched cohort study in urban India. PLoS Med 12:e1001892. doi: 10.1371/journal.pmed.1001892
Erickson, J. J., Smith, C. D., Goodridge, A., and Nelson, K. L. (2017). Water quality effects of intermittent water supply in Arraiján, Panama. Water Res. 114, 338–350. doi: 10.1016/j.watres.2017.02.009
Fan, L., Liu, G., Wang, F., Ritsema, C. J., and Geissen, V. (2014). Domestic water consumption under intermittent and continuous modes of water supply. Water Resourc. Manage. 28, 853–865. doi: 10.1007/s11269-014-0520-7
Fontanazza, C. M., Freni, G., and La Loggia, G. (2007). Analysis of intermittent supply systems in water scarcity conditions and evaluation of the resource distribution equity indices. WIT Trans. Ecol. Environ. 103:70591. doi: 10.2495/WRM070591
Fontanazza, C. M., Freni, G., Loggia, G. L., Notaro, V., and Puleo, V. (2013). Evaluation of the water scarcity energy cost for users. Energies, 6, 220–234. doi: 10.3390/en6010220
Freni, G., De Marchis, M., and Napoli, E. (2014). Implementation of pressure reduction valves in a dynamic water distribution numerical model to control the inequality in water supply. J. Hydroinform. 16, 207–217. doi: 10.2166/hydro.2013.032
Galaitsi, S. E., Russell, R., Bishara, A., Durant, J. L., Bogle, J., and Huber-Lee, A. (2016). Intermittent domestic water supply: a critical review and analysis of causal-consequential pathways. Water 8:274. doi: 10.3390/w8070274
Ghorpade, A., Sinha, A. K., and Kalbar, P. (2021). Multi-outlet storage tanks to improve water distribution networks in India. Urban Water J. 19, 1-9. doi: 10.1080/1573062X.2021.1914117
Golin, C., Cox, M., Brown, M., and Thomas, V. (2015). The water efficiency gap. Sustain. Water Resourc. Manage. 1, 315–324. doi: 10.1007/s40899-015-0025-4
Gottipati, P. V., and Nanduri, U. V. (2014). Equity in water supply in intermittent water distribution networks. Water Environ. J. 28, 509–515. doi: 10.1111/wej.12065
Government of India (2009). Guidance Notes for Continuous Water Supply (24-7 Supply). A Guide to Project Preparation, Implementation and Appraisal. New Delhi: Ministry of Urban Development.
Guragai, B., Hashimoto, T., Oguma, K., and Takizawa, S. (2018). Data logger-based measurement of household water consumption and micro-component analysis of an intermittent water supply system. J. Clean. Product. 197, 1159–1168. doi: 10.1016/j.jclepro.2018.06.198
Gurung, Y., Zhao, J., Kumar, K. C. B, Wu, X., Suwal, B., and Whittington, D. (2017). The costs of delay in infrastructure investments: a comparison of 2001 and 2014 household water supply coping costs in the Kathmandu Valley, Nepal. Water Resourc. Res. 53, 7078–7102. doi: 10.1002/2016WR019529
Haddad, M., Mcneil, L., and Omar, N. (2014). Model for predicting disinfection by-product (DBP) formation and occurrence in intermittent water supply systems: Palestine as a case study. Arab. J. Sci. Eng. 39, 5883–5893. doi: 10.1007/s13369-014-1200-x
Haddad, O., Hoseini-Ghafari, S., Solgi, M., and Loáiciga, H. A. (2016). Intermittent urban water supply with protection of consumers' welfare. J. Pipeline Syst. Eng. Pract. 7:04016002. doi: 10.1061/(ASCE)PS.1949-1204.0000231
Haider, H., Al-Salamah, I. S., Ghazaw, Y. M., Abdel-Maguid, R. H., Shafiquzzaman, M., and Ghumman, A. R. (2019). Framework to establish economic level of leakage for intermittent water supplies in arid environments. J. Water Resourc. Plann. Manage. 145:05018018. doi: 10.1061/(ASCE)WR.1943-5452.0001027
Hastak, S., Labhasetwar, P., Kundley, P., and Gupta, R. (2017). Changing from intermittent to continuous water supply and its influence on service level benchmarks: a case study in the demonstration zone of Nagpur, India. Urban Water J. 14, 768–772. doi: 10.1080/1573062X.2016.1240808
Hernandez-Lopez, R. D., Tzatchkov, V. G., Martin-Dominguez, A., and Alcocer-Yamanaka, V. H. (2016). Study of hydraulics and mixing in roof tanks used in intermittent water supply. J. Water, Sanit. Hygiene Develop. 6, 547–554. doi: 10.2166/washdev.2016.147
Ilaya-Ayza, A. E., Campbell, E., Pérez-García, R., and Izquierdo, J. (2016). Network capacity assessment and increase in systems with intermittent water supply. Water 8:126. doi: 10.3390/w8040126
Ilaya-Ayza, A. E., Martins, C., Campbell, E., and Izquierdo, J. (2017). Implementation of DMAs in intermittent water supply networks based on equity criteria. Water 9:851. doi: 10.3390/w9110851
Ilaya-Ayza, A. E., Martins, C., Campbell, E., and Izquierdo, J. (2018). Gradual transition from intermittent to continuous water supply based on multi-criteria optimization for network sector selection. J. Comput. Appl. Mathemat. 330, 1016–1029. doi: 10.1016/j.cam.2017.04.025
Jayaramu, K. P., Burt, Z., and Manoj Kumar, B. (2015). A study of the consumption pattern in a continuous water service demonstration zone and bulk water demand forecasting for Hubli-Dharwad, India. J. Water Sanit. Hygiene Develop. 5, 201–212. doi: 10.2166/washdev.2015.096
Jayaramu, K. P., Manoj Kumar, B., and Prasanna Rashmi, K. K. (2016). Improving cost recovery in urban water supply service: an experience from India. Water Policy 18, 685–707. doi: 10.2166/wp.2015.134
Kalbar, P., and Gokhale, P. (2019). Decentralized infrastructure approach for successful water supply systems in India: use of multi-outlet tanks, shafts and manifolds. J. Water Supply Res. Technol. Aqua 68, 295–301. doi: 10.2166/aqua.2019.158
Kaminsky, J., and Kumpel, E. (2018). Dry pipes: Associations between utility performance and intermittent piped water supply in low and middle income countries. Water 10:1032. doi: 10.3390/w10081032
Khadse, G. K., Patni, P. M., Talkhande, A. V., and Labhasetwar, P. K. (2016). Surveillance of the chemical and microbial quality of drinking water for safe water supply in an urban area. J. Water Supply Res. Technol. 65, 220–233. doi: 10.2166/aqua.2016.062
Klingel, P. (2012). Technical causes and impacts of intermittent water distribution. Water Sci. Technol. Water Supply 12, 504–512. doi: 10.2166/ws.2012.023
Klingel, P., and Nestmann, F. (2014). From intermittent to continuous water distribution: a proposed conceptual approach and a case study of Béni Abbès (Algeria). Urban Water J. 11, 240–251. doi: 10.1080/1573062X.2013.765493
Kumar, T., Post, A. E., and Ray, I. (2018). Flows, leaks and blockages in informational interventions: a field experimental study of Bangalore's water sector. World Develop. 106, 149–160. doi: 10.1016/j.worlddev.2018.01.022
Kumpel, E., and Nelson, K. L. (2013). Comparing microbial water quality in an intermittent and continuous piped water supply. Water Res. 47, 5176–5188. doi: 10.1016/j.watres.2013.05.058
Kumpel, E., and Nelson, K. L. (2014). Mechanisms affecting water quality in an intermittent piped water supply. Environ. Sci. Technol. 48, 2766–2775. doi: 10.1021/es405054u
Kumpel, E., and Nelson, K. L. (2016). Intermittent water supply: prevalence, practice, and microbial water quality. Environ. Sci. Technol. 50, 542–553. doi: 10.1021/acs.est.5b03973
Kumpel, E., Woelfle-Erskine, C., Ray, I., and Nelson, K. L. (2017). Measuring household consumption and waste in unmetered, intermittent piped water systems. Water Resourc. Res. 53, 302–315. doi: 10.1002/2016WR019702
Li, H., Cohen, A., Li, Z., Lv, S., He, Z., Wang, L., and Zhang, X. (2020). Intermittent water supply management, household adaptation, and drinking water quality: a comparative study in Two Chinese Provinces. Water 12:1361. doi: 10.3390/w12051361
Li, X. J., and Chong, P. H. J. (2019). Design and implementation of a self-powered smart water meter. Sensors 19:4177. doi: 10.3390/s19194177
Lieb, A. M., Rycroft, C. H., and Wilkening, J. (2016). Optimizing intermittent water supply in urban pipe distribution networks. SIAM J. Appl. Math. 76, 1492–1514. doi: 10.1137/15M1038979
Mahmoud, H. A., Savi,ć, D., and Kapelan, Z. (2017). New pressure-driven approach for modeling water distribution networks. J. Water Resourc. Plann. Manage. 143:04017031. doi: 10.1061/(ASCE)WR.1943-5452.0000781
Manohar, U., and Mohan Kumar, M. S. (2014). Modeling equitable distribution of water: dynamic inversion-based controller approach. J. Water Resourc. Plann. Manage. 140, 607–619. doi: 10.1061/(ASCE)WR.1943-5452.0000368
Mastaller, M., and Klingel, P. (2017). Adapting the IWA water balance to intermittent water supply and flat-rate tariffs without customer metering. J. Water Sanitat. Hyg. Dev. 7, 396–406. doi: 10.2166/washdev.2017.116
Mathur, S. (2017). Public-private partnership for municipal water supply in developing countries: Lessons from Karnataka, India, urban water supply improvement project. Cities 68, 56–62. doi: 10.1016/j.cities.2017.05.007
McIntosh, A. (2003). Asian Water Supplies: Reaching the Urban Poor. Mandaluyong: Asian Development Bank.
McKenzie, D., and Ray, I. (2009). Urban water supply in India: status, reform options and possible lessons. Water Policy 11, 442–460. doi: 10.2166/wp.2009.056
Mellor, J., Kumpel, E., Ercumen, A., and Zimmerman, J. (2016). Systems approach to climate, water, and diarrhea in Hubli-Dharwad, India. Environ. Sci. Technol. 50, 13042–13051. doi: 10.1021/acs.est.6b02092
Ministry of Jal Shakti and Government of India New Delhi. (2019). “Operational guidelines for the implementation of Jal Jeevan Mission (Har Ghar Jal).”
Mohan, S., and Abhijith, G. R. (2020). Hydraulic analysis of intermittent water-distribution networks considering partial-flow regimes. J. Water Resourc. Plann. Manage. 146:04020071. doi: 10.1061/(ASCE)WR.1943-5452.0001246
Mohapatra, S., Sargaonkar, A., and Labhasetwar, P. K. (2014). Distribution network assessment using EPANET for intermittent and continuous water supply. Water Resourc. Manage. 28, 3745–3759. doi: 10.1007/s11269-014-0707-y
Mokssit, A., De Gouvello, B., Chazerain, A., Figuères, F., and Tassin, B. (2018). Building a methodology for assessing service quality under intermittent domestic water supply. Water 10:1164. doi: 10.3390/w10091164
MoUD (2012). Service levels in Urban Water and Sanitation Sector. 1st edn. New Delhi: Ministry of Urban Development, Government of India.
Neelakantan, T. R., Rammurthy, D., Smith, S. T., and Suribabu, C. R. (2014). Expansion and upgradation of intermittent water supply system. Asian J. Appl. Sci. 7, 470–485. doi: 10.3923/ajaps.2014.470.485
Nyende-Byakika, S., Ndambuki, J. M., and Ngirane-Katashaya, G. (2013). Modelling of pressurised water supply networks that may exhibit transient low pressure–open channel flow conditions. Water Pract. Technol. 8, 503–514. doi: 10.2166/wpt.2013.054
Nyende-Byakika, S., Ngirane-Katashaya, G., and Ndambuki, J. M. (2012). Modeling flow regime transition in intermittent water supply networks using the interface tracking method. Int. J. Physic. Sci. 7, 327–337. doi: 10.5897/IJPS11.873
Owens, C. E., Angles, M. L., Cox, P. T., Byleveld, P. M., Osborne, N. J., and Rahman, M. B. (2020). Implementation of quantitative microbial risk assessment (QMRA) for public drinking water supplies: Systematic review. Water Res. 174:115614. doi: 10.1016/j.watres.2020.115614
Rawas, F., Bain, R., and Kumpel, E. (2020). Comparing utility-reported hours of piped water supply to households' experiences. npj Clean Water 3, 1–9. doi: 10.1038/s41545-020-0053-y
Research Design and Standards Organization (2015). Ministry of Railways, Government of India. Guidelines on water meters.
Rosenberg, D. E., Talozi, S., and Lund, J. R. (2008). Intermittent water supplies: challenges and opportunities for residential water users in Jordan. Water Int. 33, 488–504. doi: 10.1080/02508060802474574
Rubino, F., Corona, Y., Jiménez Pérez, J. G., and Smith, C. (2019). Bacterial contamination of drinking water in Guadalajara, Mexico. Int. J. Environ. Res. Public Health 16:67. doi: 10.3390/ijerph16010067
Sakomoto, T., Lutaaya, M., and Abraham, E. (2020). Managing water quality in intermittent supply systems: the case of Mukono Town, Uganda. Water 12:806. doi: 10.3390/w12030806
Sayyed, M. A. H. A., Gupta, R., and Tanyimboh, T. T. (2015). Noniterative application of EPANET for pressure dependent modelling of water distribution systems. Water Resourc. Manage. 29, 3227–3242. doi: 10.1007/s11269-015-0992-0
Simukonda, K., Farmani, R., and Butler, D. (2018). Intermittent water supply systems: causal factors, problems and solution options. Urban Water J. 15, 488–500. doi: 10.1080/1573062X.2018.1483522
Singh, L. (2021). BMC Needs Sustainable Revenue Sources like Independent Fee Revision Authority as Long Term Plan. New Delhi: The Indian Express. Available online at: https://indianexpress.com/article/cities/mumbai/bmc-needs-sustainable-revenue-sources-like-independent-fee-revision-authority-as-long-term-plan-7228292/lite/
Smith, K., and Liu, S. (2020). Methodology for evaluating city-level energy footprint for water distribution systems. J. Clean. Product. 2020:125463. doi: 10.1016/j.jclepro.2020.125463
Solgi, M., Bozorg Haddad, O., Seifollahi-Aghmiuni, S., and Loáiciga, H. A. (2015). Intermittent operation of water distribution networks considering equanimity and justice principles. J. Pipeline Syst. Eng. Pract. 6:04015004. doi: 10.1061/(ASCE)PS.1949-1204.0000198
Strijdom, L., Speight, V., and Jacobs, H. E. (2017). An assessment of sub-standard water pressure in South African potable distribution systems. J. Water Sanit. Hygiene Develop. 7, 557–567. doi: 10.2166/washdev.2017.227
Tamari, S., and Ploquet, J. (2012). Determination of leakage inside buildings with a roof tank. Urban Water J. 9, 287–303. doi: 10.1080/1573062X.2012.660959
Taylor, D. D., Slocum, A. H., and Whittle, A. J. (2018). Analytical scaling relations to evaluate leakage and intrusion in intermittent water supply systems. PloS ONE 13:e0196887. doi: 10.1371/journal.pone.0196887
Taylor, D. D., Slocum, A. H., and Whittle, A. J. (2019). Demand satisfaction as a framework for understanding intermittent water supply systems. Water Resour. Res. 55, 5217–5237. doi: 10.1029/2018WR024124
Tokajian, S., and Hashwa, F. (2003). Water quality problems associated with intermittent water supply. Water Sci. Technol. 47, 229–234. doi: 10.2166/wst.2003.0200
Vairavamoorthy, K., and Elango, K. (2002). Guidelines for the design and control of intermittent water distribution systems. Waterlines 21, 19–21. doi: 10.3362/0262-8104.2002.041
Vairavamoorthy, K., Gorantiwar, S. D., and Mohan, S. (2007a). Intermittent water supply under water scarcity situations. Water Int. 32, 121–132. doi: 10.1080/02508060708691969
Vairavamoorthy, K., Yan, J., Galgale, H. M., and Gorantiwar, S. D. (2007b). IRA-WDS: A GIS-based risk analysis tool for water distribution systems. Environ. Model. Softw. 22, 951–965. doi: 10.1016/j.envsoft.2006.05.027
Walski, T. (2014). “How does water distribution design really work?,” in World Environmental and Water Resources Congress 2014, 375–382.
Walter, D., Mastaller, M., and Klingel, P. (2018). Accuracy of single-jet and multi-jet water meters under the influence of the filling process in intermittently operated pipe networks. Water Sci. Technol. Water Supply 18, 679–687. doi: 10.2166/ws.2017.149
Yepes, G., Ringskog, K., and Sarkar, S. (2001). The high costs of intermittent water service. J. Indian Water Works Assoc. 33, 99–115.
Zyoud, S. H., and Fuchs-Hanusch, D. (2019). Comparison of several decision-making techniques: a case of water losses management in developing countries. Int. J. Inform. Technol. Decis. Mak. 18, 1551–1578. doi: 10.1142/S0219622019500275
Keywords: continuous water supply, India, intermittent water supply, vicious cycle, water supply system design, water supply system operation
Citation: Ghorpade A, Sinha AK and Kalbar PP (2021) Drivers for Intermittent Water Supply in India: Critical Review and Perspectives. Front. Water 3:696630. doi: 10.3389/frwa.2021.696630
Received: 17 April 2021; Accepted: 31 August 2021;
Published: 29 September 2021.
Edited by:
Auroop Ratan Ganguly, Northeastern University, United StatesReviewed by:
Katarzyna Pietrucha-Urbanik, Rzeszów University of Technology, PolandRamakrishna Tipireddy, Pacific Northwest National Laboratory (DOE), United States
Copyright © 2021 Ghorpade, Sinha and Kalbar. This is an open-access article distributed under the terms of the Creative Commons Attribution License (CC BY). The use, distribution or reproduction in other forums is permitted, provided the original author(s) and the copyright owner(s) are credited and that the original publication in this journal is cited, in accordance with accepted academic practice. No use, distribution or reproduction is permitted which does not comply with these terms.
*Correspondence: Pradip P. Kalbar, a2FsYmFyQGlpdGIuYWMuaW4=