- 1Civil and Environmental Engineering, Tufts University, Medford, MA, United States
- 2School of Public and International Affairs, Wright State University, Dayton, OH, United States
- 3Water Diplomacy, Civil and Environmental Engineering and The Fletcher School of Law and Diplomacy, Tufts University, Medford, MA, United States
Some of the most persistent challenges facing society and the environment arise from an intricate coupling of natural and human systems (CNHS). These challenges resist traditional expert-driven problem-solving approaches and require a careful synthesis of both “explanation” and “understanding” to achieve equity and sustainability. Whereas, explanations tend to be the domain of scientific experts who seek generalizable solutions through theory building, modeling, and testing, understandings represent the wisdom of practitioners that enables real-world problem solving to proceed by accounting for contextual values, capacities, and constraints. Using a case study from Bangladesh as an illustrative case of CNHS, we take an explanatory approach in using the extended case study method to show why and how an expert-led response to remediation of arsenic-contaminated wells led to unintended outcomes, which could have been accounted for if a complexity science informed framework of the problem was in place. The complexity frame keeps one alert to emergent patterns that otherwise remain unanticipated, and thereby, form the basis of adaptive actions. For a path forward in addressing complex CNHS problems, we introduce a novel problem-solving approach that combines pragmatic explanations and interpretive understandings with attention to emergent patterns. We argue that this problem-solving approach – which we term principled pragmatism – can effectively synthesize and apply scientific knowledge and local practical knowledge to develop and implement adaptive, actionable, and sustainable interventions.
Introduction
The lines that demarcate environmental problems from societal problems are getting thinner. Processes such as industrialization, urbanization, and agricultural intensification muddle our ability to partition phenomena into neat categories such as natural or human-made. Indeed, a growing body of literature suggests that many natural and human systems have become coupled in ways that are intrinsically complex. The resulting coupled natural and human systems (CNHS) often exhibit behaviors dominated by non-linear interactions and feedback that cross multiple scales (Maguire et al., 2006; Ramalingam et al., 2008; Jeffrey, 2011; Li, 2012; Binder et al., 2013; Allen, 2014; Cooper and Islam, 2015). The inherent irreducibility, unpredictability, and non-controllability of complex systems stymie many traditional problem-solving techniques, which often assume that the elements of a system (e.g., variables, processes, actors, and institutions) can be studied in isolation to arrive at conclusions about the system as a whole. Such approaches are not appropriate for complex systems, where the high degree of interconnectedness and interactions between system elements leads to emergent behaviors that cannot be predicted from their constituent parts (Holland, 2002; Fromm, 2005; Palash et al., 2019).
Certainly, interest in the interactions between natural and human systems, and especially human-water systems, is not new. For decades, researchers in natural and social sciences as well as in engineering have been studying this interface and exploring the question of how to mediate these coupled dynamics to improve both social and environmental outcomes. The last decade, however, has seen a tremendous increase in the interest of researchers and funding agencies to bring CNHS into the water domain through the lenses of social-ecological systems thinking, socio-hydrology, the hydro-social cycle, adaptive water management, etc. A recently published paper aptly summarizes the current debates in the literature: “The socio-hydrology community has been very successful in promoting the need for taking the human factor into account in the mainstream hydrology literature since 2012. However, the interest in studying and modeling human-water systems is not new and pre-existed the post-2012 socio-hydrology. So, it is critical to ask what socio-hydrology has been able to offer that would have been unachievable using the existing methods, tools, and analysis frameworks” (Madani and Shafiee-Jood, 2020).
Therefore, while there are several competing frameworks for exploring the dynamics of CNHS, there remains doubt about their ability to address real-world CNHS problems. Moreover, while the terms “complexity” and “contingency” are now in frequent use in discussions about how to address CNHS problems (e.g., Bazilian et al., 2011; RAND Corporation, 2016; Islam and Susskind, 2018), a generally accepted framework for problem-solving in these contexts remains elusive (Lloyd, 2001; Bonabeau, 2008; Cilliers et al., 2013). Contentious debates surround attempts to understand, explain, and manage CNHS problems by employing commonly available deterministic or statistical characterizations. After all, how do we move forward if the answer is always contingent?
While complex systems do not offer Newtonian certainty or generalizable predictability (e.g., Bar-Yam, 1997; Holland, 1998; Pahl-Wostl, 2004; Islam and Susskind, 2018), they produce emergent patterns that are not entirely random either. By examining these patterns, one can identify appropriate technical and societal interventions in light of the capacities and constraints imposed by the problem context. These patterns are neither deterministic nor random, neither perfectly predictable nor totally unpredictable, neither reducible from the macroscale nor scalable from the microscale. We suggest that an insistence on causality-based explanations and a lack of adequate appreciation for emergent phenomena have contributed to inadequacies in previous attempts to address complex CNHS challenges.
Consequently, we argue that equitable and sustainable responses to problems arising from the complexity of CNHS will require a careful synthesis of both “explanation” and “understanding” that are operant in a given context. To support our claim, we first explore a well-documented arsenic pollution case from Bangladesh to serve both as our context as well as an illustrative example of a complex CNHS. Using this case study, we show why and how an expert-led response to remediation of arsenic-contaminated wells was inadequate. Then, we suggest how the inadequacies of this approach led to unintended outcomes, which could have been accounted for if a complexity science informed problem-solving framework was in place. Such an approach pairs pragmatic explanations with interpretive understandings and pays attention to emergent patterns. We refer to this problem-solving approach as a principled pragmatic framework; the principles constitute the rigor of scientific methods and attention to value considerations (based on the understanding and interpretations of the stakeholders in a given context), while the pragmatism constitutes the factual considerations in a given context (based on explanations provided by the stakeholders using the rigor of scientific methods). On the basis of effective synthesis and application of rigorous scientific methods and nuanced local knowledge, our proposed principled pragmatic framework can lead to adaptive, actionable, and sustainable interventions in complex CNHS contexts.
Complexity, Emergence, and the Need for Both Explanation and Understanding to Address CNHS Challenges
Complexity and Emergence
The term complexity has been applied to water systems in myriad ways, creating a “lack of clarity between theory and practice as well as assertion and outcome” (Islam and Choudhury, 2018, p. 5). It finds pervasive use in the water literature as an adjective to describe systems that are exceedingly intricate, high-dimensional, or difficult to control. In this sense of the word, complexity is a challenge to be overcome through advances in data acquisition (e.g., Chen et al., 2018; Zounemat-Kermani et al., 2018), computational modeling (e.g., Berger et al., 2007; Hadka and Reed, 2015), and technologies that improve the efficiency of resource production, distribution, and consumption (e.g., Fereres et al., 2011; Stokes-Draut et al., 2017). In addition to this colloquial usage, complexity is found in multiple distinct and domain-specific senses within the water literature. For example, hydrologists have recently started to use complexity as a quantifiable statistical property of hydrologic systems (e.g., Mihailović et al., 2019; Wang et al., 2020). In this sense of the word, complexity is a measure of entropy and apparent randomness within the system or a quantification of the non-predictability inherent in deterministic chaos. Here quantifying complexity is “motivated by the fact that anthropogenically impacted basins … are coupled natural-human systems” and that complexity in CNHS is “assumed but rarely quantified” (Jovanovic et al., 2017).
In this paper, complexity is not used in a colloquial sense or to describe a quantifiable measure. Rather, our use of complexity refers to a particular condition of a system that arises when elements (i.e., variables, processes, actors, and institutions) are coupled in non-linear, ambiguous, and non-prescriptive ways and “emergent” surprises often dominate system dynamics and responses (Cilliers, 1998; Holland, 2002; Fromm, 2005; Cilliers et al., 2013). Complex systems often cross multiple domains (e.g., natural, societal, political) and scales (e.g., space, time, jurisdictional, institutional) (Islam and Repella, 2015). One popular taxonomy of complexity science refers to this as “aggregate complexity” (i.e., emergent patterns from interactions) in contrast with “algorithmic complexity” (i.e., the subject of information theory) or “deterministic complexity” (i.e., the subject of chaos theory) (Manson, 2001). We don't object to such a classification, but one misleading aspect of the phrase “aggregate complexity” is that it implies the possibility for system simplification through disaggregation, while our usage of complexity refers to systems that are not amenable to disaggregation.
Indeed, one of the most important ideas about our usage of complexity is that it lies between order (deterministic) and randomness (e.g., Bar-Yam, 1997; Holland, 1998; Pahl-Wostl, 2004; Islam and Susskind, 2018). In this sense, neither entirely ordered nor entirely random systems qualify as complex. Ordered and random systems are amenable to description in an algorithmic and predictable sense using the notion of cause and effect. However, this is not a reliable method for complex system characterization, which exhibit emergent phenomena that cannot be pre-specified or forced to reoccur. Therefore, it is nearly impossible to predict the behavior of a complex system by reducing it to its constituent elements and studying them in isolation. This inadequacy of reductionist approaches to describe system complexity is at the heart of the distinction we make between complex systems and those that are simple or complicated (Islam and Repella, 2015; Smith and Islam, 2019).
Categorizing systems as either simple, complicated, or complex provides a useful taxonomy for problem-solving (Snowden and Boone, 2007; Islam and Repella, 2015; Smith and Islam, 2019). While simple and complicated systems can be intricate, their behavior can be ascertained from the properties of their constituent parts. Such an assessment is not possible for complex systems which exhibit “emergence” – a key feature that distinguishes a system as complex rather than simple or complicated (see Table 1 for details). Emergence challenges the traditional notion of deterministic causality because the outcomes of some interactions within a system are inherently unpredictable. As a result, some macroscale phenomena emerge in complex systems that cannot be traced back to any particular element at the microscale, a condition known as equifinality. This suggests the conventional notion of cause-effect relationships may not be an appropriate framework for problem-solving in complex contexts because cause-effect relationships are often too ambiguous to be useful. At the same time, it is not practically possible to prespecify a particular response from a complex system or force such a response to occur.

Table 1. Comparison and contrasts of different aspects of simple, complicated, and complex systems [adapted from the Cynefin framework initially proposed by Snowden and Boone (2007)].
We view complexity as the condition underlying these “emergent surprises,” but not their cause. As such, one cannot hope to control the response of a complex system by simply controlling its inputs. Therefore, causal reasoning in complex systems needs to be framed with the expectation of and appreciation for emergence rather than determinism. Indeed, such an appreciation of complexity and the conditions for emergence is critical to explain, understand, and resolve complex CNHS problems (Islam and Choudhury, 2018). Keeping such an emergence in mind, recent work addressing problems in complex water systems has highlighted the importance of adaptive governance (e.g., Pahl-Wostl et al., 2012), as well as the need to blend “hard” infrastructure-based approaches with “soft” knowledge-based approaches (Kumar, 2015). However, operationalizing these ideas within complex CNHS requires us to also confront a disconnect between the observation-based technical facts (what is) from the values (what ought to be) dimensions of emergent problems. To bridge this gap, we build on this recent work by introducing “understanding” and “explanation” as mutually reinforcing concepts essential to addressing CNHS challenges. We then describe a problem-solving framework that combines pragmatic explanations and interpretive understandings with attention to emergent patterns – a framework we call “principled pragmatism.”
The Function of Understanding and Explanation in Addressing CNHS Challenges
Historically, it has been common to draw conceptual boundaries between “natural” systems and “human” systems. Roughly speaking, this distinction has been made between systems governed by invariant physical laws (natural systems) and those involving norms and values (human systems). Natural systems are often taken to be describable in objective ways, while descriptions of human systems are woven with subjective meanings. While we think it is important that readers are aware that such distinctions remain prevalent in theory and practice today, we will not examine the problematics of these disciplinary “border zones” (Brown, 1963) in detail here as the precision of such distinctions between natural and human systems is not critical to our argument at this moment. Nor do we require that readers accept the designations of natural and human as the appropriate system signifiers. Rather, we adopt these terms as a colloquial shorthand that allows us to distinguish between the physical laws that suggest that water flows downhill (natural) and the societal facts that may create conditions for water to flow uphill to money and power (human).
Addressing problems that arise from a complex coupling between natural and human systems (i.e., CNHS) requires both the ability to explain natural phenomena (water flows downhill) and to develop a value-based understanding of societal facts (water may flow uphill toward money). Here “understanding” and “explanation” serve distinct but mutually reinforcing roles. Understanding enables actors in a given context to address the problems they face in terms of the values they hold and the tools or knowledge they have. Both values and tools together constitute the local capacities and constraints that determine the feasibility of particular actions in addressing the problems they face. Such an understanding is different from explanation, which is the domain of scientific experts who seek theory building, modeling, and testing. These practices also provide knowledge of capacity and constraints that are qualitatively different from those based on understanding. Experts usually consider a given context to be an instance or a variation of a general phenomenon. The challenge here lies in translating general models to local settings, as this cognitive process tends to overlook distinct patterns that emerge locally. Yet, relying on local understanding alone may artificially restrict the availability and applicability of potentially transferable knowledge. To address the complexity of CNHS problems, we suggest that both understanding and explanation are needed to develop actionable outcomes based on the constraints and capacities that operate in a given context.
As an illustrative pedagogical example, we will make a distinction between floods as a natural phenomenon and flooding as a coupled natural and human process. Here, the word “flood” is used to describe a natural process involving a large number of variables and processes from hydrology, meteorology, river morphology, terrestrial ecology, and hydraulics. We use the word “flooding,” on the other hand, to describe the consequences of a CNHS involving socio-ecological processes, interactions, and responses that influence and are influenced by floods (i.e., the natural process).
Two important conditions dictate a flood's space-time scale and magnitude as a natural process: a river basin condition that controls the state of the basin (e.g., soil, land use, and vegetation, etc.) and an atmospheric condition that provides the key forcing mechanism (e.g., precipitation) (Palash et al., 2018). However, as soon as rising waters begin to overtop embankments, the natural process of a flood may turn into the coupled phenomena of flooding, as dikes begin to erode, populated floodplains are washed out, and nearby communities are inundated. Through interactions with human settlements and involvement of actors and institutions, it is, therefore, possible that a natural process (e.g., a flood) can become inseparably coupled to societal elements and turn into a CNHS problem (e.g., flooding).
When dealing with flood as a natural phenomenon, understanding and explanation go hand in hand. In these situations, the conventional logic of cause and effect prevails, and objective measures and metrics can be used to design, track, and measure outcomes for a given intervention (e.g., building floodwalls, reservoirs, retention ponds, etc.). On the other hand, when managing a response to complex CNHS problems, such as flooding, the relationships between explanation and understanding become ambiguous because of non-prospective cause-effect relationships and emergent surprises for a chosen intervention (e.g., waterlogging, community displacement, ecological degradation, people's resistance, etc.). Such surprises can occur at different scales, from a local community mobilizing to create temporary flood mitigation barriers to international campaigns to raise aid money for victims. In both of these examples, the surprises may manifest with emergent outcomes resulting from human compassion, which is not a variable that can be easily plugged into a predictive model. Thus, both objective explanations (e.g., of floods as natural phenomena) and subjective interpretation and understanding (e.g., societal responses to flooding) are needed to address the system complexity of a CNHS.
The difference between understanding and explanation forms the basis of distinguishing the purpose and practice of social science from natural science. Social science is primarily interested in describing the understanding that people show in their actions, while natural science is primarily characterized by the search for and validation of explanation of how nature functions. Max Weber pioneered the effort to align the two in practice. Weber did so by keeping the subjective understanding of the researcher in check while trying to explain social actions and events in terms of the subjective understanding of actors and the patterns of history. Thus, understanding becomes the “social facts” which functions as causal conditions in the explanation of social action or event (Kim, 2017). In elaborating this coupling of understanding and explanation, Peter Winch reinforced the Weberian argument with a non-reductive and contextually embedded understanding of social behavior that is simultaneously rule-based, practical, and participatory (Winch, 1990).
Thus, addressing problems arising from complex systems requires the joint consideration of understanding and explanation as mutually reinforcing. This is particularly critical in addressing CNHS problems where emergent patterns often dominate system behavior. Causality-based explanatory reasoning is usually based on past patterns; hence, most interventions derived from this form of reasoning simply seek to overcome the constraints based on capacities that were embedded in those past patterns. Consequently, an explanatory approach at most may help to address the strengths and shortcomings of those capacities and constraints that were based on past patterns and derived from causal explanations. As complex CNHS problems are likely to create emergent patterns, our aim in addressing CNHS problems is not to provide a causal explanation based on past patterns, but to take action based on the present capacity and constraints in light of emergent patterns. An arsenic case study from Bangladesh further underscores and clarifies the importance of explanation and understanding as mutually reinforcing factors in addressing CNHS problems.
A Methodological Framework for this Study
The choice and justification of a methodology rest on the nature of the research question or problem (Siggelkow, 2007; Yin, 2014, 2015; Ragin, 2015). The research question pursued in this paper is to explore the reasons for unanticipated and unintended outcomes often associated with purely technical reasoning in CNHS contexts and identify the nature of decision-making that can minimize such outcomes. To address these two questions, we will first examine the introduction of tube well technology for solving rural water problems in Bangladesh, and then subsequently explore the discovery of arsenic contamination and the approach of color-coding the wells that was used as a mitigation. Both stand as examples of expert-led technical approaches that created unanticipated social consequences. We hold that such unanticipated consequences are rooted in a lack of appreciation of the coupling of natural and human systems and related complexity. To minimize such consequences, we suggest a complexity science informed framework. The complexity frame keeps one alert to emergent patterns that otherwise remain unanticipated, and thereby, form the basis of adaptive actions. For a path forward in addressing complex CNHS problems, we introduce a principled pragmatic framework to address the complexity of CNHS problems.
The coupling of natural and human systems means that their interaction is what gives rise to knowledge and learning that can be adaptively used in a given context to find appropriate interventions. This is why we framed knowledge and learning as a synthesis of explanation (scientific and technical reason) and understanding (value laden fact and contextual meaning). Operating from this frame we argue that neither expert knowledge nor local understanding operating alone can resolve a complex problem like arsenic contamination in wells.
No established methodology has yet emerged for studying the research questions that arise in a CNHS. This is because, such questions can neither be fully explored by the positivist (e.g., hypothesis testing or survey) nor the interpretivist (ethnography or interview) methods. Therefore, a key goal for this study is not to seek generalizable theory but to explore the efficacy of our principled pragmatic framework to explain and understand the dynamics of arsenic contamination. This led us to use the case study method of research. In this case-based research methodology, our approach falls under the illustrative/explanatory (Kuiken, 2010; Tulodziecki, 2011; Yin, 2014) and extended (Anderson et al., 2005; Schritt, 2019) case research methods. A classic example of such an approach is Graham Allison's study of the Cuban Missile Crisis (Allison and Zelikow, 1999). In this classic study, Allison extended the same events to examine three different theoretical frameworks of executive decision making.
In the case study method, an illustrative case is used to describe in details the actors' experience of events that is typical of a given context. The point of using such a case is to provide an example of what occurs or tends to occur in similar contexts. In the literature, such an illustrative case is also labeled as an exemplary case. Such use of case is comparable to the use of a sample in quantitative research, of which there may be several explanations that may be inferred from a sample. The illustrative case functions as supporting a theory in specific contexts, but it can't validate a theory. The extended case method, on the other hand, does more than illustrate, it incorporates facts from the case in terms of the logic of a theory. When the illustration provided by the case is extended to explain a theory (that is independent of the case), then, an illustrative case becomes an extended case.
Our study utilized the features of both illustrative and extended case analysis. The expert-driven intervention to provide drinking water in villages constituted a typical illustrative case of solving a natural resource problem. Later, when wells were found to be contaminated, experts labeled the wells according to a binary categorization of safe or unsafe, and the problem was considered resolved from a technical perspective. However, soon a new problem emerged in an unexpected way, when it was found that people who drank the water became socially shunned. The illustrative case of expert-led technical intervention neither anticipates nor accounts for these unexpected social outcomes. Furthermore, while these social problems originated from a technical intervention, there is no technical remedy that can resolve them. One way to address such unanticipated outcomes is to be aware of the interlinkages of facts and values in the coupling of natural and human systems. We use an extended case methodology to show how a complexity informed principled pragmatic framework - that integrates social and cultural elements in designing appropriate technical strategies - may address CNHS problems independent of this particular case study of arsenic contamination.
The literature cited reflects: (1) a theoretical account of understanding complexity in CNHS; (2) a historical account of both the original intent in drilling tube wells and the delay recognition and response to arsenic contamination; (3) a factual account of the measured improvements in agricultural yields and infant mortality (intended outcome of original intervention) as well as the rules set forth for designating wells safe or unsafe (mitigation of unintended outcome of original intervention); (4) an illustrative account of the unintended outcomes arising from an expert-led mitigation of a complex problem.
A Closer Look at A CNHS Problem From Bangladesh
To make the abstract notion of CNHS clear, let us have a closer look at a CNHS problem from southwest Bangladesh. The geography, hydrology, and climate of this region (Figure 1) are highly diverse and often described as a dynamic co-evolutionary system within the Ganges-Brahmaputra-Meghna (GBM) basin (e.g., Brammer, 2014; Nicholls et al., 2016; Roy et al., 2017) with numerous socio-environmental challenges. A range of problems exists, including: arsenic contamination and related effects (Paul and De, 2000; Clarke, 2001; Harvey et al., 2002; Chakraborti et al., 2010; Flanagan et al., 2012; Edmunds et al., 2015; Naser et al., 2017); upstream water withdrawal (Mirza, 1998; Gain and Giuponni, 2014); management of coastal polders (Dewan et al., 2015; Gain et al., 2017a,b); impacts related to increasing shrimp aquaculture (Swapan and Gavin, 2011; Shameem et al., 2014; Islam et al., 2018); climate change induced sea level rise and salinity (Brammer, 2014; Nicholls et al., 2016); cyclonic storm surges and natural disasters (Akter and Mallick, 2013); preserving critical Sundarbans ecosystems (Akber et al., 2018); and an increasing number of non-eco-friendly developmental initiatives including the mega Rampal power plant construction (Chowdhury, 2017; Mahmud et al., 2020). In Figure 2, we present a hypothetical and conceptual network diagram that describes various interconnections and interactions among system elements critical to some water issues in southwest Bangladesh. Past studies (e.g., Szabo et al., 2015; Hossain et al., 2016; and Borgomeo et al., 2017) used a similar looped network for household food security and social-ecological system problems in southwest Bangladesh.
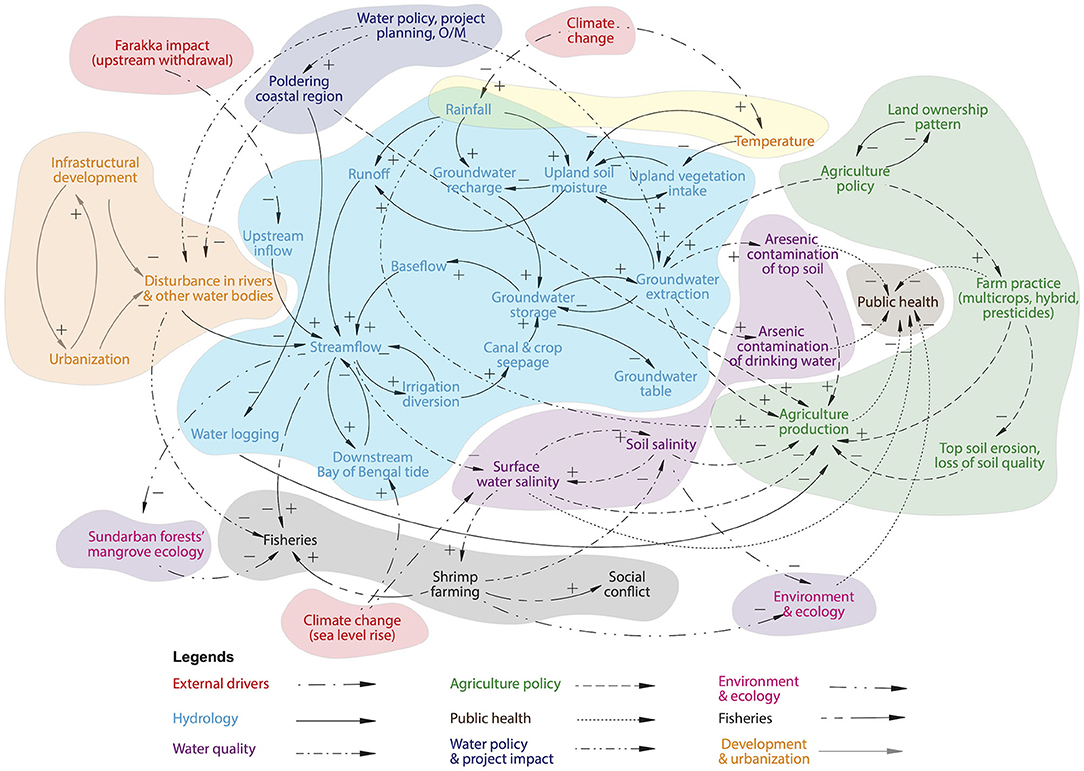
Figure 2. A scenario of interconnectedness and interactions of different elements of a CNHS from the southwest Bangladesh delta. The positive and negative signs indicate positive and negative contributions, respectively, not the positive and negative feedback [after Palash et al. (2019)].
There is a general consensus that these interlinked challenges threaten equitable access to natural resources as well as their long-term sustainable management. Yet, there is no general consensus on how to proceed, as highlighted by several problems from the southwest Bangladesh delta identified and discussed in existing literature (Harvey et al., 2002; Chakraborti et al., 2010; Hossain et al., 2013; Gain and Giuponni, 2014; Palash et al., 2014, 2019; Sala and Bocchi, 2014; Auerbach et al., 2015; Kirby et al., 2015; Szabo et al., 2015; Borgomeo et al., 2017; Parvin et al., 2017). These experiences suggest the traditional approach of generating causality based “reliable scientific knowledge” is likely to have limited success unless we incorporate “socially acceptable robust knowledge” that reflects a local understanding of the problem and work to identify interventions that are sensitive to local capacities and constraints. We will use a case study of an expert-led response to remediation of arsenic-contaminated wells in Bangladesh to highlight the risks of treating a complex CNHS as a simple system with generalizable interventions. This case study will also illustrate the need for an operational framework to define and refine CNHS interventions that are technically robust as well as socially and politically feasible.
Case Study: Promotion of Groundwater Use and Arsenic Contamination
We have argued that both explanations and understandings are required to address complexity and emergence in a CNHS. To contextualize this further, let us examine a policy intervention in the 1960s in Bangladesh: the promotion of groundwater usage to increase agricultural productivity and reduce infant mortality. During the era of the green revolution, the then East Pakistan government encouraged people to use more and more groundwater to increase crop production and cropping intensity. During the same period, there was also a massive campaign to encourage people to drink tube well water to reduce exposure to microbiologically contaminated surface water (Naser et al., 2017). While both of these initiatives succeeded in fulfilling their respective policy objectives (i.e., increasing crop production and reducing infant mortality), extensive use of groundwater created a massive public health tragedy that started emerging from the mid-1980s. No one could have “predicted” the emergence of arsenic contamination when the promotion of groundwater usage was prescribed as a scientifically reasonable policy in the 1960s.
The increased extraction of groundwater created environmental conditions that exposed a large number of people to arsenic-contaminated water and food (Paul and De, 2000; Edmunds et al., 2015; Naser et al., 2017). Over 20 million people in 59 out of 64 districts of the country were affected by arsenic contamination, and the southwest delta and southeast region are the two most affected areas in Bangladesh (Figure 3) (Chakraborti et al., 2010; Flanagan et al., 2012). The dynamics of arsenic contamination in Bangladesh is a prototypical example of emergence in a complex CNHS. A key lesson: it was nearly impossible to predict the emergence of widespread arsenic contamination when the use of groundwater was initiated and promoted with two reasonable and attainable objectives in the 1960s.
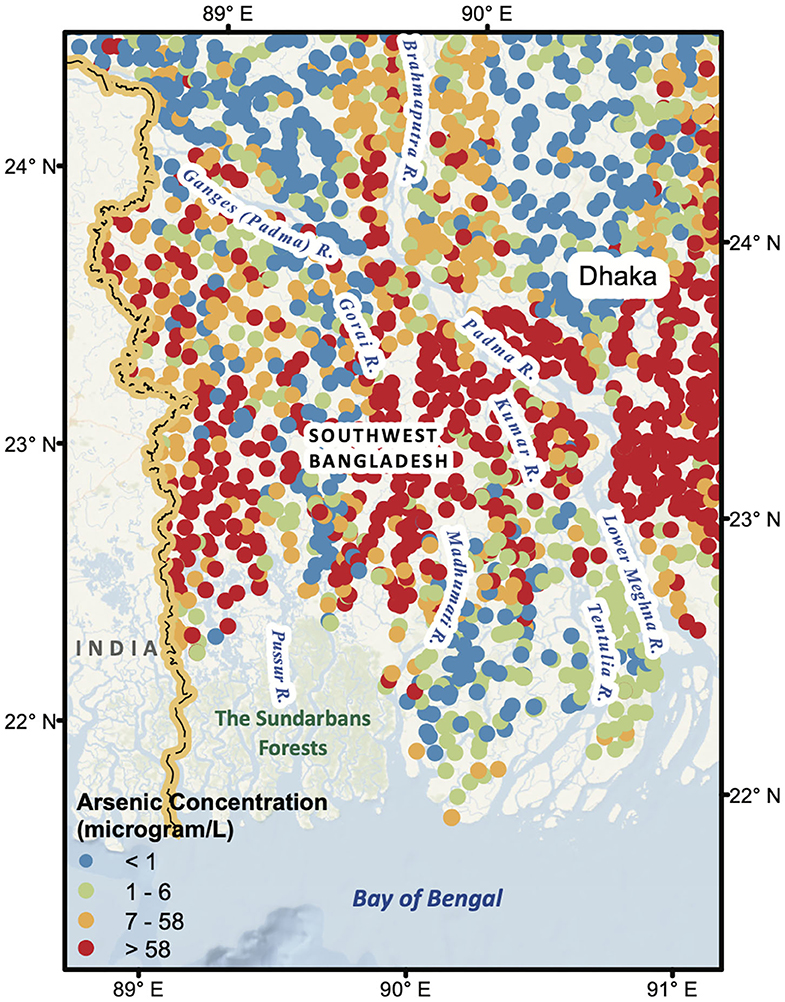
Figure 3. Arsenic contamination in tube well water during the late 1990s [data source: BGS and DPHE (2001)].
The evolution of unanticipated emergence continues with new interventions to address the problem. In 1999, UNICEF initiated a multimillion-dollar campaign to screen wells for arsenic contamination and educate communities about the dangers of consuming arsenic-contaminated water (Chappell et al., 2001). Within 5 years, the majority of shallow tube wells in rural Bangladesh were painted either red or green; red rims indicated high levels of arsenic contamination, while wells with green rims were considered safe for use (Hanchett et al., 2002; Sultana, 2007). More than 20 percent of the shallow tube wells were painted red (Chappell et al., 2001). Suddenly, the primary water source for roughly 1 in 5 households was no longer considered safe (Field et al., 2011).
However, limited access to clean water was not the only consequence that emerged from the 1999 UNICEF campaign. With the red-rimmed tube wells came tremendous stigmatization, and to this day, individuals with arsenicosis face discrimination both financially and socially (Ahmad et al., 2007; Brinkel et al., 2009). Many Bangladeshi women suffer disproportionately (Ahmad et al., 2007; Sultana, 2007; Brinkel et al., 2009); for example, women from households with red-rimmed wells are faced with diminished prospects for marriage because of suspected arsenicosis (Majumder, 2017). Some communities with red wells switched to more distant green wells facing higher levels of microbial contamination both at point-of-source and point-of-use which in some cases led to an increase in diarrheal disease. For example, it has been found that arsenic concentration in shallow tube wells is inversely related to microbial contamination which increases pathogen exposure at point-of-source (Leber et al., 2011; van Geen et al., 2011). On the other hand, less arsenic-contaminated water from distant tube wells may lead to higher microbial contamination at the point-of-use due to poor transportation and storage facilities, and water storage time (Wu et al., 2011; Ercumen et al., 2015; Buchmann et al., 2019).
To sum, the policy promoting increased groundwater use was successful in creating greater agricultural productivity and reducing infant mortality, but it also exposed millions to arsenic contamination. Later, another expert-led response to this crisis created new forms of social stigma and socioeconomic division. While we would not suggest these detrimental “side effects” could have been predicted or entirely avoided, there is evidence to suggest that their impacts were intensified by a lack of appreciation of emergence and system complexity. Indeed, arsenic exposure was largely treated as a purely natural phenomenon, and the chosen interventions reflect an assumption of a simple, rather than a complex system. Limiting exposure – the experts explained – was simply a matter of locating and identifying contaminated wells. This led to a generalized response with little room for localized understandings or adaptive responses to failed interventions. Of course, recognizing the need for both explanations and understandings is only the first step; we also need a framework for operationalizing these ideas to identify adaptive, actionable, and sustainable interventions.
Actionable Recommendations
Operationalizing Explanations and Understandings to Adaptively Manage CNHS
The debate related to differences between understanding and explanation and their relationship with one another continues to be contentious. We recognize the importance of such debates from philosophical perspectives but suggest that both understanding and explanation are needed to deal with emergent CNHS responses. As discussed earlier, in our view, both explaining observed phenomena (water flows downhill based on physical laws) and developing a system-level understanding (water may flow uphill based on societal norms and values) are integral to effective problem-solving for any complex CNHS.
Whichever operational technique is used for CNHS problem solving, we need to recognize and account for the coupling of understanding with explanation. A key attribute of such a recognition is a dialogical process that involves all affected stakeholders in a given context. Such a context-driven dialogical process is essential to explore and implement strategies that are relevant and adaptive to changing circumstances. We recognize that the resolution of complex CNHS issues usually involves interactions of many parties with conflicting values and interests operating across multiple boundaries and scales to make decisions. The interdependence and feedback among interacting variables, processes, actors, and institutions are hard to model and difficult to forecast, as we have documented in this case study of arsenic contamination.
It follows that any decision-making related to complex CNHS problems needs to be contingent and adaptive. Drawing on a number of ideas from complexity science and negotiation theory, following Islam and Susskind (2018), we suggest using three key ideas from complexity science (interdependence and interconnectedness; uncertainty and feedback; emergence and adaptation) and three from negotiation theory (stakeholder identification and engagement; joint fact-finding; and value creation through option generation) to explain and understand emergent CNHS responses and show how to operationalize these ideas to enhance the effectiveness of CNHS management.
Currently, the dominant mode of addressing CNHS problems is what we would call primarily theory driven. It starts from the premise that the problem under study is systemic in nature. In turn, it is taken for granted that solving the problem requires a theory that can predict, or at the very least, explain the behavior of the system with causality-based reasoning. For example, attributing widespread arsenic exposure to a lack of knowledge about which wells are contaminated suggests a simple and generalizable intervention: locating and identifying contaminated wells. In our assessment, such explanation-based approaches work well for uncoupled natural systems where prediction and control are possible because system behavior is governed by fundamental laws of nature. We would categorize most of these systems as simple or complicated, but not complex [for details of these distinctions, please see Smith and Islam (2019)].
If reliance on underlying laws of nature or society is a prerequisite for theory-driven problem solving, one must question whether such a premise can serve us well in addressing our most pressing social, political, and economic challenges where natural and human systems are coupled. There are no generalizable laws that can govern the dynamics of a CNHS. Attribution of causality in such systems is fraught with practical and philosophical difficulties. We challenge the dominant premise that any amount of synthesis and incremental tweaking of disparate subject matter is likely to improve this condition. Rather, in the case of complex CNHS issues, we heed Karl Popper's advice to be “not students of some subject matter, but students of problems” (Popper, 2002, p. 88).
Therefore, our proposed approach to operationalizing explanation and understanding begins by pairing a problem-driven question (understanding) with a dynamic hypothesis of observed signals (explanation). Leading with a specific problem-driven question rather than a general theory allows us to better contextualize the problem, evaluate feasible interventions, and identify locally-relevant outcome metrics. A dynamic hypothesis is developed to explain the behavior of the outcome metrics over time; a framework can then be put to use to guide and communicate the development, testing, and refinement of ideas into actionable outcomes.
Another reason for starting with a problem-driven question and hypothesis is that there are inherent trade-offs among generality, realism, and precision (Levins, 1966). Levins argued that to maximize any one of the three requires sacrifices to the other two. For example, an approach designed to explain an observed behavior may have poor predictive performance compared to an approach explicitly designed for prediction (Bokulich, 2013). Sterman (2000) goes one step further, arguing that, in the context of socio-technical systems, useful models must represent a problem and not a system. He notes that the selected explanation and understanding of observed response need to be justified by a problem to be solved or the intervention to be tested. We argue that operationalizing a problem-driven approach that incorporates both understanding and explanation requires attention to both principles and pragmatism.
A Principled and Pragmatic Approach
Many contemporary CNHS problems will require professionals to engage in inclusive conversations on the contextual relationship between facts and values, and on collective decision-making processes involving experts from other disciplines as well as stakeholders with local knowledge. We argue that effective synthesis and application of these domains of knowledge requires principled pragmatism, where the principles constitute value considerations (which shapes understanding), and pragmatism constitutes the factual considerations (explanation based on objective facts in a given action context). The meaning of principled pragmatism we offer here seeks to move away from the dichotomy of being either “pragmatic” or “normative” and to focus on their interaction.
In methodological terms, this means respecting both the need to (slowly) cultivate understandings (i.e., to identify shared values, establish “social facts,” agree on acceptable risks, and pinpoint areas of irreconcilable disagreement) and the importance of (quickly) arriving at actionable “experiments” to test and revise possible explanations (i.e., identify physical system constraints, establish “scientific facts,” and elucidate “knowable unknowns” through joint fact-finding). In practical terms, this involves a focus on the most effective means of generating scientifically defensible ideas and transforming them into action, given the constraints and capacities available or applicable in the problem context. It recognizes that strict adherence to principles without pragmatism is often not actionable and that pure pragmatism exercised without guiding principles is not likely to be sustainable and equitable.
Thus, for a pattern of action to be considered as principled and pragmatic, it needs to be value-based, that is, purposeful or intentional. The efficacy of such purposive action arises jointly from the understanding of the actors involved and expert explanations that are based on scientifically defensible facts and metrics. With expert knowledge, one can quantify certain measures of sustainability, the limits of resource use, or the limits of knowledge for a given problem. Yet such knowledge may not be actionable without the value-based understanding of the social actors involved. For example, the primacy of sustainability and equity as guiding principles need to enter into the meaning of purpose in order for any scientific explanations of the observed natural processes to provide a principled and pragmatic solution.
A principled approach is essential when taking future action when outcomes are uncertain and mostly unknowable. In these cases, an assessment of only past actions may not be very helpful in guiding actions in light of emergent patterns. This is because we do not and cannot have any facts (as facts are yet to happen) about the emergent future. Hence, principled pragmatism, as conceived here, rests on focused experimentation and exploration as modes of action. The use of contextual principles provides the focus for such experiments. Thus, trial and error and tacit understanding are likely to be more useful to address CNHS problems than relying exclusively on causality-based explanation, top-down expert guidelines (plans), and standard operating procedures (precedents).
For a principled pragmatic action to be effective, experimentation and adaptation to changing situations are more important than compliance and expert direction. Best practices have to be adapted to local conditions and capacities. Developing the capacity of actors to affect this mode of action also requires addressing their understanding rather than simply imposing an explanation on them based on authority or resource enticement. The response to arsenic-contaminated groundwater in Bangladesh presents a cautionary tale of imposed explanations, generalized interventions, and a lack of understanding of local capacities and constraints.
Revisiting the Response to Arsenic Contamination Through the Lens of Principled Pragmatism
When the rapid growth in drilling first started in the 1960s, and for the two decades that followed, the promotion of groundwater for both agricultural and potable household use was seen as non-controversial. With the widespread availability of fertilizers and pesticides, obtaining the required quantities of water was the last hurdle of the impending “green revolution.” At the same time, the intensification of agriculture and the densification of population led to increasingly contaminated surface waters, a source of disease and a leading cause of infant mortality. Groundwater was seen as a vast source of water that was protected from the abundant sources of pollution at the surface. The system was taken to be simple, separable, and repeatably addressable with an off-the-shelf solution: the tube well. The results were impressive: agricultural yields increased, and infant mortality was reduced. The best available knowledge in the hydrogeological literature in the 1960s did not suggest there was a risk of arsenic presence in riverine or deltaic plains, so it simply wasn't tested for (Clarke, 2001).
If one were to drill a well in the southwest Bangladesh delta today and failed to test it for arsenic – we would call that an act of professional negligence. However, it is unfair to make such accusations today with regard to what happened in the 1960s when there was no evidence of neglect. There will always be “unknown unknowns,” and it is impossible to eliminate the risk of overlooking something one didn't know to look for. Where we can be critical of the situation, and provide possible suggestions, is in how the response to the recognition of arsenic contamination was handled. That is to say, while the surprise of arsenic contamination could not have been foreseen, the subsequent surprises that came out of the well-painting campaign may have been anticipated, or at the very least, adaptively managed through a synthesis of principles and pragmatism.
The first published indications of arsenic groundwater contamination in the region were available in 1984 (Garat et al., 1984), and by 1988 there were six journal publications on the topic, including one in the WHO Bulletin (Chakraborti et al., 2015). In 1995, an international conference on arsenic was held in West Bengal, India, and “representatives of international agencies in Bangladesh and Bangladesh government attended the conference but they denied the groundwater arsenic contamination in Bangladesh” (Chakraborti et al., 2015, p. 237). It was not until 1998 when the international arsenic conference was held in Dhaka that the problem was widely acknowledged (ibid). It is hard to describe exactly why it took nearly 15 years after the first alarms were sounded for the problem to be acknowledged (nearly doubling the exposure time for many users). There was, of course, political resistance to acknowledging a mistake, and this should not be discounted. However, there was also the initial assumption that the system was simple and well-understood. In such cases, there are few provisions made upfront for assimilating and responding to new information; that is, there was no capacity for adaptive management, a situation that continues to this day.
Once the signal of an emerging crisis was too strong to ignore, the assumption that the system was simple was carried forward into the response, that is, the repetition of a past pattern. A simple, generalizable, and causal solution was sought. A bureaucratic decision-making process involving government officials and international NGOs came to a conclusion: a static threshold of 50 ppb arsenic would be used to assess the safety of tube wells; wells found to be above this concentration would be painted red and wells below this concentration would be painted green. This formula was simple enough that it could be deployed rapidly on a massive scale. And why shouldn't it work? Didn't John Snow manage to stop a cholera epidemic in London simply by removing the handle of the Broad Street pump in 1854? Why wouldn't warning users of arsenic-contaminated wells have the same effect?
However, simple approaches to complex problems are bound to be inadequate. For complex problems, interventions in one context are not immediately transferable to another. In particular, John Snow's approach was effective because it eliminated one water source among many safe alternatives. However, the well-painting campaign in Bangladesh was carried out without considering that the availability of safe alternatives is a local, site-specific condition. The lack of nuance meant it was possible for all wells in a community to be painted red, leaving little practical guidance about the safest possible sources of available water.
If the system had been recognized as complex, the response to the discovery of arsenic contamination could have been approached with both principles and pragmatism. A principled approach would have avoided a top-down form of bureaucratic management, on the understanding that, while such forms of management may be well-equipped for simple and complicated contexts, they are inadequate in the complex contexts presented by this CNHS. The assumption of simplicity led to an intervention “designed for rural Bangladeshi communities instead of with them” (Majumder, 2017, p. xiv). A principled approach would have opted for a more horizontal and inclusive process, where impacted communities could be consulted, and shared understandings of acceptable risk could have been developed.
In areas where most or all of the wells were above the strict 50 ppb limit, experts and stakeholders could have worked together to establish a rating system that identified the safest possible sources for drinking water, rather than strictly marking them all as red. While a bureaucratic process is unable to compromise in this way (no allowable deviation from the 50 ppb rule), a consultative and collaborative process can come to a shared understanding and make a pragmatic compromise (e.g., a well-contaminated with 60 ppb arsenic may be deemed preferable with a minimal consumption schedule than relying on surface water that is likely to be contaminated with water-borne pathogens). Such a compromise does not entail a compromise of the community's values (e.g., maximizing the safety of drinking water available and accessible to all), but rather represents a pragmatic compromise between alternatives that acknowledges contextual constraints.
Moreover, through an open and inclusive consultative process, the most vulnerable communities would have had a more direct channel to aid organizations regarding the acquisition of expensive but safe technological alternatives, such as deep tube wells. However, in the absence of such a forum, the distribution of publicly financed deep tube wells appears to have suffered from “elite capture,” where the siting decisions for deep tube wells were made largely at the discretion of the political elite. One study found that a more equitable distribution of the same number of wells “would have brought almost three times as many exposed households within walking distance of a [low arsenic] source” (van Geen et al., 2016, p. 148).
Acknowledging system complexity also means accepting that the situation is dynamic, and any choice of action is likely to be contingent. That is, there are no static “solutions” in complex systems; rather, there is a need for ongoing observations and collaborative adaptive management. In a very simple sense, the concentration of arsenic in groundwater is not a static feature of an aquifer, but the wells were painted so as to suggest they were permanently safe or unsafe. In one follow-up survey, “17% of wells marked green in 2001 were marked red in 2005” and “12% of wells marked red in 2001 were marked green in 2005” (Johnston and Sarker, 2007, p. 1894). However, such sampling has been piecewise, and today there are no formal institutions driving any systematic effort for long-term well monitoring or maintaining up-to-date records about which wells are above the 50 ppb limit and which are not. In contrast to this, an essential part of any collaborative and adaptive management process is routine monitoring, assessment, and provisional response managed by local stakeholders in a local context.
As mentioned earlier, principled pragmatism requires that we acknowledge that understanding and explanation are mutually reinforcing and that both are critical aspects of managing complex sustainability issues. In addition to providing spaces for arriving at a shared understanding of values, inclusive processes that engage experts and stakeholders also provide mechanisms for identifying and correcting commonly held factual misunderstandings. For example, an expert-informed explanation of the biochemical mechanisms of arsenicosis is an essential step in reducing social stigma around the disease, which is widely misunderstood to be contagious (Hassan et al., 2005). Of course, the explanations of experts are not enough. Expert-provided explanations must be coupled with a shared understanding of contextually effective ways to communicate the relevant facts so that they are received as both trustworthy and important. In this way, a principled and pragmatic approach appreciates the mutually reinforcing mechanisms of understanding and explanation in a CNHS and acknowledges that it is their interaction that leads to actionable and equitable interventions, rather than relying on one approach isolated from the other.
Discussion
Some of the most persistent challenges facing society and the environment arise from an intricate coupling of natural and human systems. We have argued that this complex coupling creates emergent problems that resist traditional expert-driven problem-solving approaches, which rely primarily on causality-based reasoning about previously observed patterns. Effective responses to the complexity and emergence of CNHS requires a careful synthesis of both “explanations” and “understandings” to achieve equitable and sustainable outcomes in a concrete problem context.
In making our arguments, we've attempted to avoid unnecessarily describing the attributes of complex systems purely in the abstract (e.g., as systems that possess non-linear boundary-crossing feedbacks at multiple scales, etc.). Too often, these abstract descriptions lead to semantic debates about what is and isn't complex. While valuable in some academic contexts, within the scope of the present work these debates serve as a distraction from the more important question: why do such distinctions matter?
Our key takeaway is this: the way that we characterize a system shapes our response to its problems. As summarized in Table 1, if we see the system as simple, we expect that we can rely on experts (or the marketplace) to develop repeatable solutions that treat root causes and adopt widely available and tested “best practices.” If we identify the system as complicated, we might seek diverse expertise to create locally tailored interventions that meet a required level of service. However, if we identify the system as complex, we recognize that expert informed explanations must be paired with understandings derived from inclusive, early, and ongoing stakeholder engagement. Achieving sustainable and equitable outcomes requires mobilizing both explanation and understanding in a way that that is responsive to emergent patterns and reflective of local capacities and constraints.
To this end, we have offered problem-driven principled pragmatism as a framework for operationalizing the application of explanation and understanding in complex CNHS contexts. The vision of principled pragmatism we offer seeks to move away from the dichotomy of “being pragmatic” or “being ideological” by focusing on how to transform ideas to action given the constraints and capacities of the problem context. It recognizes that strict adherence to principles without pragmatism is often not actionable and that pure pragmatism exercised without guiding principles is not sustainable and unlikely to be equitable. Sustainability in complex problem contexts requires adaptation, and equity demands that this adaptation be consultative. Through a consultative process, experts and stakeholders can arrive at a shared vision of the problem, identify areas for experimentation and joint fact-finding, and ultimately build a portfolio of actionable interventions with measurable outcomes.
Our case study on arsenic poisoning highlights just one of the many ongoing CNHS issues impacting the southwest Bangladesh delta. Our aim in reflecting on these issues is not to place blame or to suggest that it was possible to foretell all of the unintended and emergent detrimental outcomes. Rather, our goal is to understand how failures to recognize system complexity contributed to interventions and forms of management that were ill-suited for the dynamic and adaptive contexts posed by the CNHS. We use the evolution of arsenic contamination and associated interventions to show how the recognition of system complexity and a commitment to both principles and pragmatism could have improved – and still could improve – the management of this complex and emergent CNHS crisis of arsenic contamination.
Author Contributions
WP wrote the first draft, developed the background material on Bangladesh as a CNHS, and created all figures. KS lead the development of subsequent drafts, refined and extended the case study, and managed the revision and interactive review process. EC and SI developed the arguments regarding principled pragmatism and the distinction between explaining and understanding. EC developed the methodology section. All authors contributed sections to the manuscript. All authors contributed to manuscript revision, read, and approved the submitted version.
Funding
This research was supported, in part, by US National Science Foundation grants (NSF-IGERT 0966093; NSF-RCN 1140163; and NSF-NRT 2021874).
Conflict of Interest
The authors declare that the research was conducted in the absence of any commercial or financial relationships that could be construed as a potential conflict of interest.
References
Ahmad, S. A., Sayed, M. H. S., Khan, M. H., Karim, M. N., Haque, M. A., Bhuiyan, M. S. A., et al. (2007). Sociocultural aspects of arsenicosis in Bangladesh: community perspective. J. Environ. Sci. Health A 42, 1945–1958. doi: 10.1080/10934520701567247
Akber, M. A., Patwary, M. M., Islam, M. A., and Rahman, M. R. (2018). Storm protection service of the Sundarbans mangrove forest, Bangladesh. Nat. Hazards 94, 405–418. doi: 10.1007/s11069-018-3395-8
Akter, S., and Mallick, B. (2013). The poverty–vulnerability–resilience nexus: evidence from Bangladesh. Ecol. Econ. 96, 114–124. doi: 10.1016/j.ecolecon.2013.10.008
Allen, P. M. (2014). Evolution: complexity, uncertainty and innovation. J. Evol. Econ. 24, 256–289. doi: 10.1007/s00191-014-0340-1
Allison, G. T., and Zelikow, P. (1999). Essence of Decision: Explaining the Cuban Missile Crisis. 2nd Edn. Boston, MA: Addison Wesley Longman.
Anderson, R. A., Crabtree, B. F., Steele, D. J., and McDaniel, R. R. Jr. (2005). Case study research: the view from complexity science. Qual. Health Res. 15, 669–685. doi: 10.1177/1049732305275208
Auerbach, L. W. Jr., Goodbred, S. L., Mondal, D. R., Wilson, C. A., Ahmed, K. R., Roy, K., et al. (2015). Flood risk of natural and embanked landscapes on the Ganges: Brahmaputra tidal delta plain. Nat. Clim. Change 5, 153–157. doi: 10.1038/nclimate2472
Bar-Yam, Y. (1997). Dynamics of Complex Systems (Studies in Nonlinearity). 1st Edn. Reading, MA: Perseus Books.
Bazilian, M., Rogner, H., Howells, M., Hermann, S., Arent, D., Gielen, D., et al. (2011). Considering the energy, water and food nexus: towards an integrated modelling approach. Energy Policy 39, 7896–7906. doi: 10.1016/j.enpol.2011.09.039
Berger, T., Birner, R., Mccarthy, N., Díaz, J., and Wittmer, H. (2007). Capturing the complexity of water uses and water users within a multi-agent framework. Water Resour. Manag. 21, 129–148. doi: 10.1007/s11269-006-9045-z
BGS and DPHE (2001). “Arsenic contamination of groundwater in Bangladesh,” in British Geological Survey Technical Report WC/00/19, eds D. G. Kinniburgh and P. L. Smedley (Keyworth: British Geological Survey). p. A1–A149.
Binder, C. R., Hinkel, J., Bots, P. W. G., and Pahl-Wostl, C. (2013). Comparison of frameworks for analyzing social-ecological systems. Ecol. Soc. 18:26. doi: 10.5751/ES-05551-180426
Bokulich, A. (2013). “Explanatory Models versus predictive models: reduced complexity modeling in geomorphology,” in EPSA11 Perspectives and Foundational Problems in Philosophy of Science. The European Philosophy of Science Association Proceedings, Vol 2, eds V. Karakostas and D. Dieks (Cham: Springer). doi: 10.1007/978-3-319-01306-0_10
Borgomeo, E., Hall, J. W., and Salehin, M. (2017). Avoiding the water-poverty trap: insights from a conceptual human-water dynamical model for coastal Bangladesh. Int. J. Water Resources Dev. 34, 900–922. doi: 10.1080/07900627.2017.1331842
Brammer, H. (2014). Bangladesh's dynamic coastal regions and sea-level rise. Climate Risk Manage. 1, 51–62. doi: 10.1016/j.crm.2013.10.001
Brinkel, J., Khan, M. H., and Kraemer, A. (2009). A systematic review of arsenic exposure and its social and mental health effects with special reference to Bangladesh. Int. J. Environ. Res. Public Health 6, 1609–1619. doi: 10.3390/ijerph6051609
Buchmann, N., Erica, F., Rachel, G., and Reshmaan, H. (2019). Throwing the Baby Out with the Drinking Water: Unintended Consequences of Arsenic Mitigation Efforts in Bangladesh. NBER Working Paper Series, No. 25729, National Bureau of Economic Research, Cambridge, MA, United States. doi: 10.3386/w25729
Chakraborti, D., Rahman, M. M., Das, B., Murrill, M., Dey, S., Mukherjee, S. C., et al. (2010). Status of groundwater arsenic contamination in Bangladesh: a 14-year study report. Water Resources 44, 5789–5802. doi: 10.1016/j.watres.2010.06.051
Chakraborti, D., Rahman, M. M., Mukherjee, A., Alauddin, M., Hassan, M., Dutta, R. N., et al. (2015). Groundwater arsenic contamination in Bangladesh – 21 years of research. J. Trace. Elem. Med. Biol. 31, 237–248. doi: 10.1016/j.jtemb.2015.01.003
Chappell, W. R., Abernathy, C. O., and Calderon, R. L. (2001). Arsenic Exposure and Health Effects, IV. Oxford: Elsevier.
Chen, Y., Fan, R., Yang, X., Wang, J., and Latif, A. (2018). Extraction of urban water bodies from high-resolution remote-sensing imagery using deep learning. Water 10:585. doi: 10.3390/w10050585
Chowdhury, A. H. (2017). Environmental impact of coal based power plant of rampal on the sundarbans (world largest mangrove forest) and surrounding areas. MOJ Ecol. Environ. Sci. 2:00022. doi: 10.15406/mojes.2017.02.00022
Cilliers, P., Biggs, H. C., Blignaut, S., Choles, A. G., Hofmeyr, J. S., Jewitt, G. P. W., et al. (2013). Complexity, modeling, and natural resource management. Ecol. Soc. 18:1. doi: 10.5751/ES-05382-180301
Clarke, T. (2001). Bangladeshis to sue over arsenic poisoning. Nature 413:556. doi: 10.1038/news011011-14
Cooper, E., and Islam, S. (2015). Exploring the interconnections and interdependencies in California's water problem. Available online at: http://blog.waterdiplomacy.org/2015/09/exploring-the-interconnections-and-interdependencies-at-play-in-californias-water-problem/ (accessed October 10, 2020).
Dewan, C., Mukherji, A., and Buisson, M. C. (2015). Evolution of water management in coastal Bangladesh: from temporary earthen embankments to depoliticized community-managed polders. Water Int. 40, 401–416. doi: 10.1080/02508060.2015.1025196
Edmunds, W. M., Ahmed, K. M., and Whitehead, P. G. (2015). Environmental science processes and impacts a review of arsenic and its impacts in groundwater of the Ganges: Brahmaputra – Meghna delta. Environ. Sci. 17, 1032–1046. doi: 10.1039/C4EM00673A
Ercumen, A., Naser, A. M., Unicomb, L., Arnold, B. F., Colford, J. M. Jr, and Luby, S. P. (2015). Effects of source-versus household contamination of tubewell water on child diarrhea in rural Bangladesh: a randomized controlled trial. PLoS ONE 10:e0121907. doi: 10.1371/journal.pone.0121907
Fereres, E., Orgaz, F., and Gonzalez-Dugo, V. (2011). Reflections on food security under water scarcity. J. Exp. Bot. 62, 4079–4086. doi: 10.1093/jxb/err165
Field, E., Glennerster, R., and Hussam, R. (2011). Throwing the Baby Out With the Drinking Water: Unintended Consequences of Arsenic Mitigation Efforts in Bangladesh. Work. Pap., Dep. Econ, Harvard Univ., Cambridge, MA, United States.
Flanagan, S. V., Johnston, B., and Zheng, Y. (2012). Arsenic in tube well water in Bangladesh: health and economic impacts and implications for arsenic mitigation. Bull. World Health Organ. 90, 839–846. doi: 10.2471/BLT.11.101253
Fromm, J. (2005). Ten Questions About Emergence, Technical Report. Available online at: http://arxiv.org/abs/nlin.AO/0509049 (accessed October 10, 2020).
Gain, A. K., Benson, D., Rahman, R., Datta, D. K., and Rouillard, J. J. (2017a). Tidal river management in the south west Ganges-Brahmaputra delta in Bangladesh: moving towards a transdisciplinary approach? Environ. Sci. Policy 75, 111–120. doi: 10.1016/j.envsci.2017.05.020
Gain, A. K., and Giuponni, C. (2014). Impact of the Farakka dam on thresholds of the hydrologic flow regime in the lower Ganges River basin (Bangladesh). Water 6, 2501–2518. doi: 10.3390/w6082501
Gain, K. A., Mondal, S. M., and Rahman, R. (2017b). From flood control to water management: a journey of Bangladesh towards integrated water resources management. Water 9:55. doi: 10.3390/w9010055
Garat, R., Chakraborty, A. K., Dey, S. B., and Saha, K. C. (1984). Chronic arsenic poisoning from tube-well water. J. Indian Med. Assoc. 82, 34–35.
Hadka, D., and Reed, P. (2015). Large-scale parallelization of the Borg multiobjective evolutionary algorithm to enhance the management of complex environmental systems. Environ. Model. Softw. 69, 353–369. doi: 10.1016/j.envsoft.2014.10.014
Hanchett, S., Nahar, Q., Agthoven, A. V., Geers, C., and Rezvi, F. J. (2002). Increasing awareness of arsenic in Bangladesh: lessons from a public education programme. Health Policy Plann. 17, 393–401. doi: 10.1093/heapol/17.4.393
Harvey, C. F., Swartz, C. H., Badruzzaman, A. B. M., Keon-Blute, N., Yu, W., Ali, M. A., et al. (2002). Arsenic mobility and groundwater extraction in Bangladesh. Science 298 1602–1606. doi: 10.1126/science.1076978
Hassan, M. M., Atkins, P. J., and Dunn, C. E. (2005). Social implications of arsenic poisoning in Bangladesh. Social Sci. Med. 61, 2201–2211. doi: 10.1016/j.socscimed.2005.04.021
Holland, J. H. (2002). “Complex adaptive systems and spontaneous emergence,” in Complexity and Industrial Clusters. Contributions to Economics, eds A. Q. Curzio and M. Fortis (Heidelberg: Physica-Verlag HD), 25–34. doi: 10.1007/978-3-642-50007-7_3
Hossain, M. S., Eigenbrod, F., Johnson, F. A., and Dearing, J. A. (2016). Unravelling the interrelationships between ecosystem services and human wellbeing in the Bangladesh delta. Int. J. Sustain. Dev. World Ecol. 24, 120–134. doi: 10.1080/13504509.2016.1182087
Hossain, M. S., Uddin, M. J., and Fakhruddin, A. N. M. (2013). Impacts of shrimp farming on the coastal environment of Bangladesh and approach for management. Rev. Environ. Sci. Biotechnol. 12, 313–332. doi: 10.1007/s11157-013-9311-5
Islam, M. A., Akber, M. A., Ahmed, M., Rahman, M. M., and Rahman, M. R. (2018). Climate change adaptations of shrimp farmers: a case study from southwest coastal Bangladesh. Clim. Dev. 11, 1–10. doi: 10.1080/17565529.2018.1442807
Islam, S., and Choudhury, E. (2018). “Complexity and contingency: understanding transboundary water issues,” in Complexity of Transboundary Water Conflicts: Enabling Conditions for Negotiating Contingent Resolutions, eds E. Choudhury and S. Islam (London: Anthem Press), 3–26. doi: 10.2307/j.ctv8xngkk.6
Islam, S., and Repella, A. C. (2015). Water diplomacy: a negotiated approach to manage complex water problems: University council on water resources. J. Contemp. Water Res. Educ. 155, 1–10. doi: 10.1111/j.1936-704X.2015.03190.x
Islam, S., and Susskind, L. (2018). Using complexity science and negotiation theory to resolve boundary-crossing water issues. J. Hydrol. 562, 589–598. doi: 10.1016/j.jhydrol.2018.04.020
Jeffrey, G. (2011). “Emergence in complex systems,” in The SAGE Handbook of Complexity and Management, eds P. Allen, S. Maguire, and B. McKelvey (London: SAGE Publications), 65–78. doi: 10.4135/9781446201084.n4
Johnston, R. B., and Sarker, M. H. (2007). Arsenic mitigation in Bangladesh: national screening data and case studies in three upazilas. J. Environ. Sci. Health A 42, 1889–1896. doi: 10.1080/10934520701567155
Jovanovic, T., García, S., Gall, H., and Mejía, A. (2017). Complexity as a streamflow metric of hydrologic alteration. Stoch. Environ. Res. Risk Assess. 31, 2107–2119. doi: 10.1007/s00477-016-1315-6
Kirby, J. M., Ahmad, M. D., Mainuddin, M., Palash, W., Quadir, M. E., Shah-Newaz, S. M., et al. (2015). The impact of irrigation development on regional groundwater resources in Bangladesh. Agric. Water Manage. 159, 264–276. doi: 10.1016/j.agwat.2015.05.026
Kuiken, D. (2010). “Exemplary case design,” in Encyclopedia of Case Study Research, eds A. J. Mills, E. Wiebe, and G. Durepos (Thousand Oaks, CA: Sage Publications). p. 359–362.
Kumar, P. (2015). Hydrocomplexity: addressing water security and emergent environmental risks. Water Resour. Res. 51, 5827–5838. doi: 10.1002/2015WR017342
Leber, J., Rahman, M. M., Ahmed, K. M., Mailloux, B., and Geen, A. (2011). Contrasting influence of geology on E. coli and arsenic in aquifers of Bangladesh. Ground Water 49, 111–123. doi: 10.1111/j.1745-6584.2010.00689.x
Li, B. (2012). “From a micro: macro framework to a micro – Meso – Macro framework,” in Engineering, Development and Philosophy, eds S. Christensen, C. Mitcham, B. Li, and Y. An (Dordrecht: Springer), 23–36. doi: 10.1007/978-94-007-5282-5_2
Lloyd, S. (2001). Measures of complexity: a nonexhaustive list. IEEE Control Syst. Magazine 21, 7–8. doi: 10.1109/MCS.2001.939938
Madani, K., and Shafiee-Jood, M. (2020). Socio-hydrology: a new understanding to unite or a new science to divide? Water 12:1941. doi: 10.3390/w12071941
Maguire, S., McKelvey, B., Mirabeau, L., and Ötzas, N. (2006). “Complexity science and organization studies,” in The SAGE Handbook of Organization Studies, 2nd Edn, eds S. R. Clegg, C. Hardy, T. B. Lawrence, and W. R. Nord (London: SAGE Publications), 165–214. doi: 10.4135/9781848608030.n6
Mahmud, M. S., Roth, D., and Warner, J. (2020). Rethinking “development”: land dispossession for the Rampal power plant in Bangladesh. Land Use Policy 94:104492. doi: 10.1016/j.landusepol.2020.104492
Majumder, M. (2017). “The blind men, the elephant and the well: A parable for complexity and contingency,” in Water Diplomacy in Action: Contingent Approaches to Managing Complex Water Problems, eds S. Islam and K. Madani (London; New York, NY: Anthem Press), xiii-xvi.
Manson, S. M. (2001). Simplifying complexity: a review of complexity theory. Geoforum 32, 405–414. doi: 10.1016/S0016-7185(00)00035-X
Mihailović, D. T., Nikolić-Ðorić, E., Arsenić, I., Malinović-Milićević, S., Singh, V. P., Stošić, T., et al. (2019). Analysis of daily streamflow complexity by Kolmogorov measures and Lyapunov exponent. Physica A 525, 290–303. doi: 10.1016/j.physa.2019.03.041
Mirza, M. M. Q. (1998). Diversion of the Ganges Water at Farakka and its effects on salinity in Bangladesh. Environ. Manage. 22, 711–722. doi: 10.1007/s002679900141
Naser, A. M., Martorell, R., Narayan, K. M. V., and Clasen, T. F. (2017). First do no harm: the need to explore potential adverse health implications of drinking rainwater. Environ. Sci. Technol. 51, 5865–5866. doi: 10.1021/acs.est.7b01886
Nicholls, R. J., Hutton, C. W., Lázár, A. N., Allan, A., Adger, W. N., Adams, H., et al. (2016). Integrated assessment of social and environmental sustainability dynamics in the Ganges-Brahmaputra-Meghna delta, Bangladesh. Estuar. Coast. Shelf Sci. 183, 370–381. doi: 10.1016/j.ecss.2016.08.017
Pahl-Wostl, C. (2004). “The implications of complexity for integrated resources management,” in Keynote Paper in iEMSs International Congress: Complexity and Integrated Resources Management, eds C. Pahl-Wostl, S. Schmidt, and T. Jakeman (Osnabrück: International Environmental Modelling and Software Society), 561–569. doi: 10.1016/j.envsoft.2005.12.024
Pahl-Wostl, C., Lebel, L., Knieper, C., and Nikitina, E. (2012). From applying panaceas to mastering complexity: toward adaptive water governance in river basins. Environ. Sci. Policy 23, 24–34. doi: 10.1016/j.envsci.2012.07.014
Palash, W., Jiang, Y., Akanda, A. S., Small, D. L., Nozari, A., and Islam, S. (2018). A streamflow and water level forecasting model for the Ganges, Brahmaputra and Meghna rivers with requisite simplicity. J. Hydrometeorol. 19, 201–225. doi: 10.1175/JHM-D-16-0202.1
Palash, W., Quadir, M. E., Shah-Newaz, S. M., Kirby, M. D., Mainuddin, M., Khan, A. S., et al. (2014). Surface Water Assessment of Bangladesh and Impact of Climate Change, Bangladesh Integrated Water Resources Assessment Report. Dhaka: Institute of Water Modeling.
Palash, W., Smith, K. M., and Islam, S. (2019). “Coupling of natural and human systems: a case study of a complex system from southwest Bangladesh delta,” in Interdisciplinary Collaboration for Water Diplomacy: A Principled and Pragmatic Approach, eds S. Islam and K. Smith (New York, NY: Routledge), 224–239. doi: 10.4324/9780429428760-12
Parvin, G. A., Ali, M. H., Fujita, K., Abedin, M. A., Habiba, U., and Shaw, R. (2017). “Land use change in southwestern coastal Bangladesh: consequence to food and water supply,” in Land Use Management in Disaster Risk Reduction: Disaster Risk Reduction (Methods, Approaches and Practices), eds M. Banba and R. Shaw (Tokyo: Springer), 381–401. doi: 10.1007/978-4-431-56442-3_20
Paul, B. K., and De, S. (2000). Arsenic poisoning in Bangladesh: a geographic analysis. J. Am. Water Work. Assoc. 36, 799–809. doi: 10.1111/j.1752-1688.2000.tb04307.x
Popper, K. (2002). Conjectures and Refutations: The Growth of Scientific Knowledge. 2nd Edn. New York, NY: Routledge.
Ragin, C. (2015). “Case-oriented research,” in International Encyclopedia of the Social and Behavioral Sciences, 2nd Edn, Vol 3, ed J. D. Wright (Berkeley, CA: Elsevier), 187–193. doi: 10.1016/B978-0-08-097086-8.44004-3
Ramalingam, B., Jones, H., Reba, T., and Young, J. (2008). Exploring the science of complexity: Ideas and implications for development and humanitarian efforts. Development 16:89. Available online at: www.odi.org.uk/rapid/publications/RAPID_WP_285.html
RAND Corporation (2016). Developing the Pardee Rand Food-energy-water Security Index: toward a Global Standardized, Quantitative, and Transparent Resource Assessment. Santa Monica, CA: RAND Corporation.
Roy, K., Gain, A. K., Mallick, B., and Vogt, J. (2017). Social, hydro-ecological and climatic change in the southwest coastal region of Bangladesh. Reg. Environ. Change 17, 1895–1906. doi: 10.1007/s10113-017-1158-9
Sala, S., and Bocchi, S. (2014). Green revolution impacts in Bangladesh: exploring adaptation pathways for enhancing national food security. Clim. Dev. 6, 238–255. doi: 10.1080/17565529.2014.886988
Schritt, J. (2019). An ethnography of public events: reformulating the extended case method in contemporary social theory. Ethnography. doi: 10.1177/1466138119891446. [Epub ahead of print].
Shameem, M. I. M., Momtaz, S., and Rauscher, R. (2014). Vulnerability of rural livelihoods to multiple stressors: a case study from the southwest coastal region of Bangladesh. Ocean Coast Manag. 102, 79–87. doi: 10.1016/j.ocecoaman.2014.09.002
Siggelkow, N. (2007). Persuasion with case studies. Acad. Manage. J. 50, 20–24. doi: 10.5465/amj.2007.24160882
Smith, K. M., and Islam, S. (2019). “Making distinctions: the importance of recognizing complexity in coupled natural and human systems,” in Interdisciplinary Collaboration for Water Diplomacy: A Principled and Pragmatic Approach, eds S. Islam and K. M. Smith (New York, NY: Routledge), 22–40. doi: 10.4324/9780429428760-2
Snowden, D. J., and Boone, M. E. (2007). A leader's framework for decision making. Harv. Bus. Rev. 85, 69–76.
Sterman, J. D. (2000). Business Dynamics: Systems Thinking and Modeling for a Complex World. New York, NY: McGraw-Hill.
Stokes-Draut, J., Taptich, M., Kavvada, O., and Horvath, A. (2017). Evaluating the electricity intensity of evolving water supply mixes: the case of California's water network. Environ. Res. Lett. 12:114005. doi: 10.1088/1748-9326/aa8c86
Sultana, F. (2007). Water, water everywhere, but not a drop to drink: pani politics (water politics) in Rural Bangladesh. Int. Femin. J. Polit. 9, 494–502. doi: 10.1080/14616740701607994
Swapan, M. S. H., and Gavin, M. (2011). A desert in the delta participatory assessment of changing livelihoods induced by commercial shrimp farming in southwest Bangladesh. Ocean Coast. Manage. 54, 45–54. doi: 10.1016/j.ocecoaman.2010.10.011
Szabo, S., Hossain, M. S., Adger, W. N., and Matthews, Z. (2015). Soil salinity, household wealth and food insecurity in tropical deltas: evidence from south-west coast of Bangladesh. Sustain. Sci. 11, 411–421. doi: 10.1007/s11625-015-0337-1
Tulodziecki, D. (2011). A case study in explanatory power: John Snow's conclusions about the pathology and transmission of cholera. Stud. Hist. Philos. Biol. Biomed. Sci. 42, 306–316. doi: 10.1016/j.shpsc.2011.02.001
van Geen, A., Ahmed, K. M., Ahmed, E. B., Choudhury, I., Mozumder, M. R., Bostick, B. C., et al. (2016). Inequitable allocation of deep community wells for reducing arsenic exposure in Bangladesh. J. Water Sanit. Hyg. Dev. 6, 142–150. doi: 10.2166/washdev.2015.115
van Geen, A., Ahmed, K. M., Akita, Y., Alam, M. J., Culligan, P. J., Emch, M., et al. (2011). Fecal contamination of shallow tubewells in Bangladesh inversely related to arsenic. Environ. Sci. Technol. 45, 1199–1205. doi: 10.1021/es103192b
Wang, Y., Tao, Y., Sheng, D., Zhou, Y., Wang, D., Shi, X., et al. (2020). Quantifying the change in streamflow complexity in the Yangtze River. Environ. Res. 180:108833. doi: 10.1016/j.envres.2019.108833
Winch, P. (1990). The Idea of a Social Science and its Relation to Philosophy, 2nd Edn. London: Routledge.
Wu, J., van Geen, A., Ahmed, K. M., Alam, Y. A. J., Culligan, P. J., et al. (2011). Increase in diarrheal disease associated with arsenic mitigation in Bangladesh. PLoS ONE 6:e29593. doi: 10.1371/journal.pone.0029593
Yin, R. K. (2014). Case Study Research: Design and Methods, 5th Edn. Thousand Oaks, CA: SAGE Publications.
Yin, R. K. (2015). “Case studies,” in International Encyclopedia of the Social and Behavioral Sciences, 2nd Edn, Vol 3, ed J. D. Wright (Berkeley, CA: Elsevier), 194–201. doi: 10.1016/B978-0-08-097086-8.10507-0
Keywords: complexity, emergence, principled pragmatism, coupled natural and human systems, explanation and understanding, arsenic contaminated drinking water
Citation: Smith KM, Palash W, Choudhury E and Islam S (2021) Addressing Complex Challenges in Coupled Natural and Human Systems Through Principled Pragmatism: A Case Study From Bangladesh. Front. Water 3:617255. doi: 10.3389/frwa.2021.617255
Received: 14 October 2020; Accepted: 05 January 2021;
Published: 28 January 2021.
Edited by:
Saket Pande, Delft University of Technology, NetherlandsReviewed by:
Timos Karpouzoglou, Royal Institute of Technology, SwedenRiveraine Walters, University of Idaho, United States
Copyright © 2021 Smith, Palash, Choudhury and Islam. This is an open-access article distributed under the terms of the Creative Commons Attribution License (CC BY). The use, distribution or reproduction in other forums is permitted, provided the original author(s) and the copyright owner(s) are credited and that the original publication in this journal is cited, in accordance with accepted academic practice. No use, distribution or reproduction is permitted which does not comply with these terms.
*Correspondence: Shafiqul Islam, c2hhZmlxdWwuaXNsYW1AdHVmdHMuZWR1