- 1Civil Engineering School, Griffith University, Southport, QLD, Australia
- 2School of Civil Engineering, University of Sydney, Sydney, NSW, Australia
- 3BMT, Brisbane, QLD, Australia
- 4Department of Civil, Environmental and Construction Engineering, University of Central Florida, Orlando, FL, United States
- 5Griffith Centre for Coastal Management, Griffith University, Southport, QLD, Australia
Sea-level rise, storm surges, and floods in coastal cities have already threatened large population and infrastructure with potential to increase significantly in future as climate changes. Therefore, increasing disaster resilience has become a major priority for coastal cities. At the same time, recent development in information and communication technology, ubiquitous sensors, and advanced data science allow us to generate insights that were unimaginable before and can assist in better managing coastal disaster risks. In this paper, using an infrastructure resilience lens, we critically review a set of academic literature that focus on the new development of smart systems in coastal disaster management and a set of use cases that focus on their practical application in different coastal cities. We find that smart city technologies such as internet of things (IoT) and crisis informatics have significant potential and have been increasingly used in academic studies but their city-scale applications in coastal disaster management have been limited. We discuss the challenges and opportunities of using smart city frameworks for increasing disaster resilience of coastal communities.
Introduction
Natural hazard events have been an ongoing element in the coastal regions around the world. Flooding and erosion episodes in the coastal margins during storms, hurricanes or cyclones are putting a significant amount of assets, infrastructures, and communities at risk. Seventy-two percentage of the 63 most populated cities (with 5 million or more inhabitants in 2011) are located on or near the coast (United Nations, 2019). In one hand, population density in the hazard-prone coastal areas and megacities is expected to grow by 25% by 2050 (Hallegatte et al., 2013). On the other hand, a rise in sea level is likely to increase the frequency and impacts of these episodic coastal hazard events. In 2005, average global flood losses in the world's largest 131 coastal cities was approximately US$6 billion per year with the potential to increase to US$52 billion per year by 2050 due to sea level rise (Hallegatte et al., 2013). As a result, global investment and maintenance costs of protecting the coast from sea level rise estimated to be US$ 12–71 billion per year in 2100 (Hinkel et al., 2014). Therefore, increasing resilience of our coastal cities, their infrastructure systems and community in general to these episodic natural disasters is very critical.
Cities are inherently complex with interactions among multiple systems (e.g., supply chains and transportation networks, water and energy networks, housing and business infrastructures, social networks), agents (individuals, businesses etc.) and institutes that manage or influence these systems and agents (Tyler and Moench, 2012). The impacts of natural disasters in a coastal city therefore stems from complex interactions among a potentially damaging physical event (e.g., flood, erosion, storm) and the vulnerability of these systems, agents, and institutes (Birkmann, 2006; Tonmoy et al., 2014). Therefore, increasing disaster resilience of a coastal city needs an understanding of inherent stresses and vulnerabilities of these multiple systems. Specifically disruption in vulnerable critical infrastructure systems such as water supply network, transportation network, electricity production and distribution systems, health care facilities etc. that are located in the hazard prone areas of a coastal city can result in significant social and economic disruption. Therefore major coastal cities around the world are putting emphasis on increasing disaster resilience of their critical infrastructure systems (Aerts et al., 2014). Here critical infrastructure refers to any infrastructure that provides a service to the maintenance of the well-being of the population and something that if disrupted might cause serious harm to the well-being of the community (Attwood et al., 2011).
On the other hand, information and communications technology (ICT) has seen significant advancements in recent years with different types of smart and connected technologies capable to generate real-time information at an unprecedented scale. Alongside there has been significant improvement in computational power to manage and analyse big datasets. Specifically, rapid advancements in artificial intelligence, ubiquitous sensing technology, smart city/infrastructure, availability of big data sources such as social media, mobile devices, infrastructure management systems (e.g., SCADA) allow us to collect and analyse data with details and coverage unimaginable before (Kitchin, 2014; Gupta and Gupta, 2016; Murayama et al., 2017). These advancements offer opportunities to develop data-driven decision support tools for a better understanding and management of coastal disasters in cities with a substantial potential to increase the resilience of coastal communities. Deploying these advanced ICT infrastructures within a city context to improve citizen services is often referred as “smart city” and has become the latest trend in urbanization (Hollands, 2008; Batty et al., 2012; Kitchin, 2015; Wiig, 2015).
The definition of a “smart city” is diverse and so are its applications. Two distinct understandings of smart cities are prevalent in the literature. First, smart cities are viewed as urban places with ubiquitous computing and digitally instrumented devices built into the very fabric of urban environments. Examples include wireless telecom networks, digitally controlled utility services and transport infrastructure, sensor and camera networks, building management systems, smart phones producing data about resident's location, and activity etc. (Kitchin, 2014). These can make a city “knowable and controllable” and ultimately improve the performance and delivery of public services. A second view of smart cities is broader: this is seen as the development of knowledge economy within a city driven by ICT as a central platform. This notion of smart cities is based on the fact that embedding ICT in urban infrastructure on its own does not make a city smart; rather its entire ecosystem (economy, community, infrastructure, environment) should be managed having ICT as one of the central platform (Hollands, 2008). A similar view is presented by Batty et al. (2012) where authors sketched the fundamentals of what constitutes a smart city and argued that smart cities should include smart economy (competitiveness and entrepreneurship), smart people (social and human capital), smart governance (participation in decision making), smart mobility (transport and ICT), smart environment (sustainable resource management and smart living (quality of life). In both schools of thought, however, a common theme is the need to enhance the sustainability and resilience of the city as a whole. This paper adopts the former definition in order to investigate to what extent smart city frameworks have been used around the world toward increasing coastal disaster resilience of critical infrastructure systems.
Disaster resilience of a city is often characterized in three distinct stages of a hazard event namely preparedness of multiple systems of the city to reduce potential impact of the hazard, ability to manage and respond during the event to minimize loss, and finally the ability to manage recovery of the affected systems from the hazard impacts (i.e., bringing the system to its normal state). A review of the use of communications technology during disasters in recent years shows that while it has played a positive role, its full potential has not yet been realized (Diane and Meier, 2009; Dunaway et al., 2017). Different smart city features have been implemented around the world for better management of all these three phases of disasters. City specific case studies have been released highlighting how cities have implemented smart features in their urban margins [e.g., (Caragliu et al., 2011; Bakici et al., 2013; Scuotto et al., 2016)] but not all of them attempted to tackle coastal disasters. Furthermore, some of these case studies do not appear in the peer reviewed literature as they are often published as project reports. On the other hand, there has been a number of academic peer reviewed research publications reporting on the innovative development and implementation of smart city features for disaster management of cities from a range of natural and man-made hazards (e.g., Alazawi et al., 2011, 2012, 2014; Ancona et al., 2015; Choi and Bae, 2015; Kumar et al., 2015; Lo et al., 2015; Hernández-Nolasco et al., 2016; Shalini et al., 2016). This peer reviewed literature often reports a specific application or innovation of using smart features in disaster management. Therefore, it is difficult to understand from this body of literature to what extent new innovations are infiltrating in practical applications and what are the major challenges and opportunities of implementing smart city features for increasing coastal disaster resilience. There are several review papers that highlight the broader application of smart city features, but mainly focusing on different aspects of the discipline e.g., review of definitions and terminologies of smart cities used around the globe, review of smart city governance, review of enabling technologies, review of IBM smart city projects etc. (Caragliu et al., 2011; Cocchia, 2014; Anthopoulos, 2015; Yin et al., 2015; Meijer and Bolívar, 2016). However, to the best of our knowledge, none of the review papers investigated both gray literature application case studies and academic peer reviewed literature to analyse the variety of development and application of smart city features in coastal disaster management. This knowledge gap makes it difficult to anticipate future research directions and major development trends within the field of coastal disaster management.
To this end, the objectives of this study are: (1) to describe the broad characteristics of coastal disasters and their potential impacts on the resilience of city infrastructures; (2) to investigate a sample of case studies around the world to identify how coastal disaster resilience has been tackled using smart city features in those cities; (3) to analyse a sample of peer reviewed literature on disaster management and smart city to identify new innovation and development trends; (4) discuss current challenges, opportunities, and potential future research directions for this sector.
Characteristics of Coastal Disaster Resilience in Cities
A number of coastal hazards can trigger a natural disaster in the coastal zone. Here it is important to make a distinction between “Coastal Hazards” and “Hazards in the coastal zone.” Where the first one is about hazards that are introduced by the action of sea and its interaction with the coast, the second one includes all hazards that are relevant for the coastal zone regardless whether they are due to action of sea or not (e.g., heatwave, landslide etc.). The scope of his paper is limited to the former. Different coastal hazards such as coastal flooding, erosion, sea level rise etc. propagate and have impact on coastal systems at a different time scale. As an example, while coastal flooding during a storm or cyclone is a rapid event with immediate impacts (in the order of hours and days), sea level rise has a slow onset with longer-term impacts (in the order of decades to centuries). Similarly, coastal erosion can have a rapid onset when a coastal storm or cyclone swept away significant part of the sand of a beach (in the order of days), but erosion can also have a moderate-term onset as a result of long-shore sediment transport where sediments are transported by waves from one beach to the other resulting in sediment deficit and erosion (in the order of seasons) and a longer-term onset where erosion of the coast increases as sea level gradually rises (in the order of decades and century) (Table 1). These time scales of coastal hazard govern the hazard impacts on coastal infrastructure. On top of temporal variation, these hazards also vary spatially as intensity of these hazards are amplified with specific geographic and geomorphic formation of the coastal area (e.g., low lying areas, erodible shoreline etc.).
In order to make coastal infrastructure systems robust against hazards that vary temporally and spatially, the concept of “resilience” is becoming increasingly popular among disaster management professionals and researchers. The definitions of resilience emanate from multiple disciplines (e.g., ecology, disaster management, engineering) and therefore are quite diverse in the literature (Cimellaro et al., 2010). In general, resilience is defined as the ability of a system to resist and/or to recover from a shock. Scholars use other terminologies as a measure of resilience such as flexibility and the ability to maintain the status quo or to reorganize after stress or shock (Manyena, 2006; Bhamra et al., 2011). Resilience is also considered as an emergent property of a system to manage high variability and uncertainty in order to continuously pursue successful performance of a system (Cimellaro et al., 2010; Francis and Bekera, 2014; Kong and Simonovic, 2019; Kong et al., 2019). Tyler and Moench (2012) argued that the application of the concept of resilience to urban climate adaptation and hazard mitigation practice would help to address some of the “predict and prevent” approach and allow preparing infrastructure systems for climate change even under high uncertainty.
Disaster Risk Management (DRM) includes all activities, programmes, and measures which can be taken up before, during and after a disaster with the purpose to avoid a disaster, reduce its impact or recover from its losses (Khan et al., 2008). Figure 1 shows the characteristics of a resilient infrastructure system in these three distinct phases of DRM. To better prepare for any future hazard, in the preparedness stage, several steps can be conducted such as understanding the characteristics of coastal hazards though modeling and engineering analysis, developing early warning systems, installing hazard mitigation measures, educating and informing communities about hazards etc. When a disaster is unfolding (e.g., crossing of a cyclone/hurricane over a coastal area) a resilient infrastructure system should be able to absorb physical stresses from the hazard and maintain serviceability even with reduced capacity and should have the capacity to restore the system to full operation mode once the stress from the hazard is over (e.g., providing backup, restore function etc.). Finally, a resilient system uses its learnings from an event, policies are implemented and capacity increased so that in the face to future disaster stress, the system can better cope and fight back quickly. For any critical infrastructure operator it is crucial to reduce the time the system spend between t1 and t2.
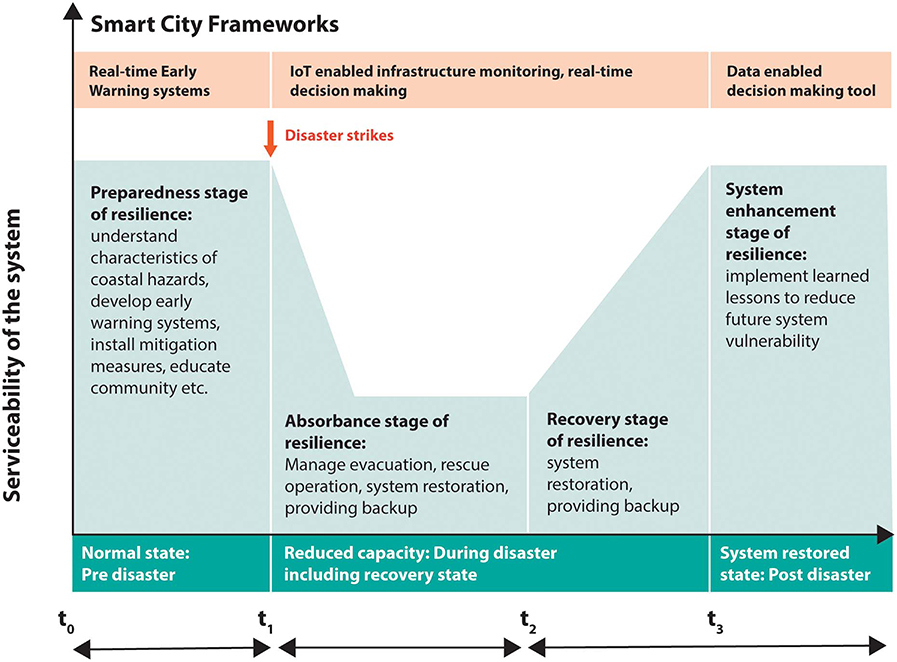
Figure 1. Characteristics of a resilient infrastructure system in three distinct phases of disaster risk management.
Increasing Coastal Disaster Resilience in Cities Using ICT
Due to technological advancements as well as increased trend in natural and man-made disasters, the importance and scope of information and communication technology (ICT) in increasing disaster resilience have increased in recent years. Different phases of disaster management of infrastructure systems that are discussed in the earlier section (Figure 1), are benefitting from the recent advancement of ICT. Examples can be drawn from the use of early warning systems (in the preparedness phase), collection and analysis of real-time hazard information for effective coordination of disaster management and recovery operation (during the disaster), setting up of long-term monitoring systems for understanding trend of the coastal or climatic variable that are responsible for creating the hazard (during the post disaster state). At a global, regional, and national scale advanced information and communication technologies have been implemented for the generation and distribution of disaster alerts and warnings (be it coastal or not) (see Table 2). Among these, Common Alerting Protocol (CAP) and Emergency Data Exchange Language (EDEL) messaging standards have been adopted by developed nations such as in Canada, Australia, Japan and Taiwan etc. These systems allow these countries to facilitate automatic notification of certain natural hazards by sensor systems, analyse, and exchange results between emergency information systems and services. CAP and EDEL are international standards for exchanging emergency alerts in a digital format that allows a consistent alert message to be disseminated simultaneously over many different communications systems. These consistent formats allowed development of critical services such as Google Alert, European public warning systems etc.
These global and regional alert systems act as early warning systems for coastal disasters and assist national, regional authorities to communicate the risk of the cyclones, hurricanes, flooding etc. to coastal communities so that they can take necessary measures to reduce their loss from the disaster. Owner and operators of critical infrastructure systems also use these early warning systems to inform their disaster management activities. However, these global and regional alerts alone are not sufficient for managing disaster risks of critical infrastructure systems within a city. These critical systems often have complex and interdependent networks and increasing disaster resilience of such networks requires finer scale real-time information that goes beyond just the disaster alert systems.
To this end, recent development of sensors, their web of networks with ability to communicate via internet (i.e., internet of things) provide a tremendous opportunity to make better and real-time disaster management decisions for critical interdependent infrastructure systems. Specific opportunities of using ICT at a local scale coastal disaster management includes:
• Supplementing early warning systems with near-real-time to real-time analysis of hazard information. This will allow more pro-active decision making due to any disruption and recovery effort of a critical infrastructure failure.
• Opportunity to know more about how different infrastructure systems perform under stress during disasters (e.g., maintain physical integrity, cope with increased demand, cope with reduced capacity as result of disruption of connected services etc.), so that system vulnerabilities can be identified and initiatives can be taken to make them more resilient.
• Opportunity to use of data enabled decision support systems to prepare, manage, and recover from coastal disasters. Visualization and analysis of near-real-time information from sensors about the extent of the hazard, performance of the infrastructure etc. provides an opportunity to make informed decision about where and how resources should be allocated to recover lost infrastructure services
Emergence of the Smart City Concept and Its Use in Coastal Disaster Management at a City Scale
The term “smart cities” has gained significant attention in academia, businesses and government in the last decade, especially in cities where ICT has been embraced as a development strategy. Cities that are embedding digital infrastructure in the urban fabric for providing better service to its citizens through web access services, to better manage facilities and to promote entrepreneurship are often termed as “smart cities,” “intelligent cities,” “connected cities” etc. (Kitchin, 2014).
What Does a Smart City Framework Look Like?
There are four main layers in a smart city framework (Figure 2). A perception layer which includes range of sensors that can collect real-time or “near real time” data. This also includes a sensor or device management component to handle registration of new devices, assignment of unique identifiers, format data, etc. A network of sensors provides interfaces to interconnect heterogeneous information sources in a secure way and a data storage services to persistently store collected data. Finally this includes a layer which analyses and visualizes stored and real-time data stream. Visualization services include different formats like visual diagrams, reports, graphs, etc. Some of the specialized type of application also include analysis of georeferenced data and dissemination of alarms and notifications. This is beyond the scope of this paper to present detailed smart city frameworks and for more information readers are directed to following references (Alazawi et al., 2014; Sanchez et al., 2014; Scuotto et al., 2016). Rather, we investigate how this generic framework has been used for the coastal disaster management in cities.
Development Trend in Peer Reviewed Academic Literature
Selection of Peer Reviewed Academic Literature
In order to identify relevant academic papers we conducted an extensive search in the “Web of Science” database in early 2018. Initial search was conducted using key words “smart” AND “disaster management” within topics for the entire database and it yielded 223 papers (Type-1). This included papers related to disaster planning and management of all hazards which goes beyond the scope of the paper. We then refined the search by adding “coast” in the search term and it yielded only 6 papers. It was quite clear that these search terms are very narrow therefore we then expanded the scope of the search by making it “smart” AND “disaster management” AND “flood” which yielded 33 papers (type-2). Often flooding in the coastal city is a big issue and used as a topic in the academic papers. However, by reading through the abstracts of this list it was evident that this list includes studies that are non-coastal in nature. In order to get a representative sample of coastal studies we replaced “flood” with “coast OR flood” and also included “IoT” (internet of things) along with our initial search term “smart.” Type 3 search criteria was “smart OR IoT” AND “disaster management” AND “coast OR flood” which yielded 18 papers. IoT has been one of the main driver of smart city developments and its inclusion in our search criteria allowed capturing technical papers that not necessarily use the term “smart” rather report their development using a more technical terms. A clear trend is visible in all these three sets that research on smart and innovative disaster management systems have been growing exponentially since 2010 (Figure 3). It is possible that we may have missed few papers, but our aim was not to find every paper that deals with smart city and coastal disaster, rather to find a representative sample of papers to understand major trend within this emerging body of work.
Analysis of Peer Reviewed Literature
Analysis of this final type-3 set of papers revealed two clear branches. First, a set of papers developed innovative IoT based smart systems for better identification and communication of hazard information (flooding, Tsunami etc.). Table 3 shows key feature of these studies. Table 3 maps seven studies from the type 3 sample (which specifically used IoT based smart systems) across their application, type of hazards they addressed, resilience stage which they aimed to cover and technologies used across four layers of smart city framework that are described in Figure 2. It shows that application of IoT based major innovations in our study samples mainly focused on managing two types of coastal hazards, flood and Tsunami. While investigating the technologies that are used across different layers the smart city framework, it was found that in the “perception layer” along with different types of sensors, CCTV camera and smart phones are used for collection of real-time information. Collected information of the perception layer is transmitted by various mediums e.g., Wi-Fi, cellular, internet. Studies which conducted further analysis to generate insights often stored the data either in cloud based systems of local servers. Choice of storage was primarily guided by the size of data generated by the perception layer and analysis method that were used by the authors.
The second group of papers, often categorized as “crisis informatics,” reported use of different advanced analysis techniques (optimization, social media or crowd sourced information etc.) or advanced technologies such as virtual reality for better preparing and managing coastal disasters. As an example, Basu et al. (2016), collected situational information through interactive crowd-sourcing using SMS from the “crowd” present at the disaster site, and analyzed them to develop situational awareness to support appropriate decision-making regarding damage or need assessment during coastal disasters in India. Ai et al. (2016) combined a geographical information system and social media to develop a dynamic decision support system (GIS-SM-DDSS) that integrates geographical information with Twitter technology to enable self-organized information networks to support decision making and collective actions in emergency situations. Ogie et al. (2017) used network analysis to determine optimal sensor locations in developing countries, so that a low cost early warning system of coastal flooding can be implemented. Anbalagan and Valliyammai (2016) used real time social media contents such as micro blogs, tweets, posts and multimedia content along with geographical location tags (geo-tags) to map the severity of the disaster. Kawai et al. (2015) developed a smart Tsunami drill system using virtual reality technologies to educate and increase awareness of Tsunami disaster among school children.
Implementation Trend at a City Scale
After investigating the development trends in the academic literature, we investigated, how cities are embracing some of these smart city features for managing their coastal disasters. Smart city projects are often reported as case studies to showcase city's progress in embracing new technology to serve its citizens. Among many other information, these case studies generally highlight key technical features of the implemented smart city projects. Analyzing a sample of such case studies can provide a sense about how new developments in the academic literature are translated into practical project implementation.
Selection of the Case Study Sample
A google search was conducted using key words “smart city” AND “Case study” to generate a sample of city specific case studies from the unpublished gray literature that document how city's smart city projects are implemented. Initial screening of the sample documents revealed that not all smart city case studies have disaster management related content. This is mainly due to the fact that some cities do not include disaster management as their focus while implementing their smart city, be it coastal or not, or the case study document that we selected may not have included the disaster management component of the city. Whichever is the case, our analysis discarded those case studies and retain only those that report any disaster management related features. In the next step some of the non-coastal city specific case studies were eliminated to ensure that our final sample only consists of case studies that include coastal disaster management.
Table 4 shows the final list of the sample case studies. It should be noted that this list is not exhaustive and it is likely to miss some. However, the aim of this paper is not to develop an exhaustive list of case studies rather analyzing a sample in order to understand major trends.
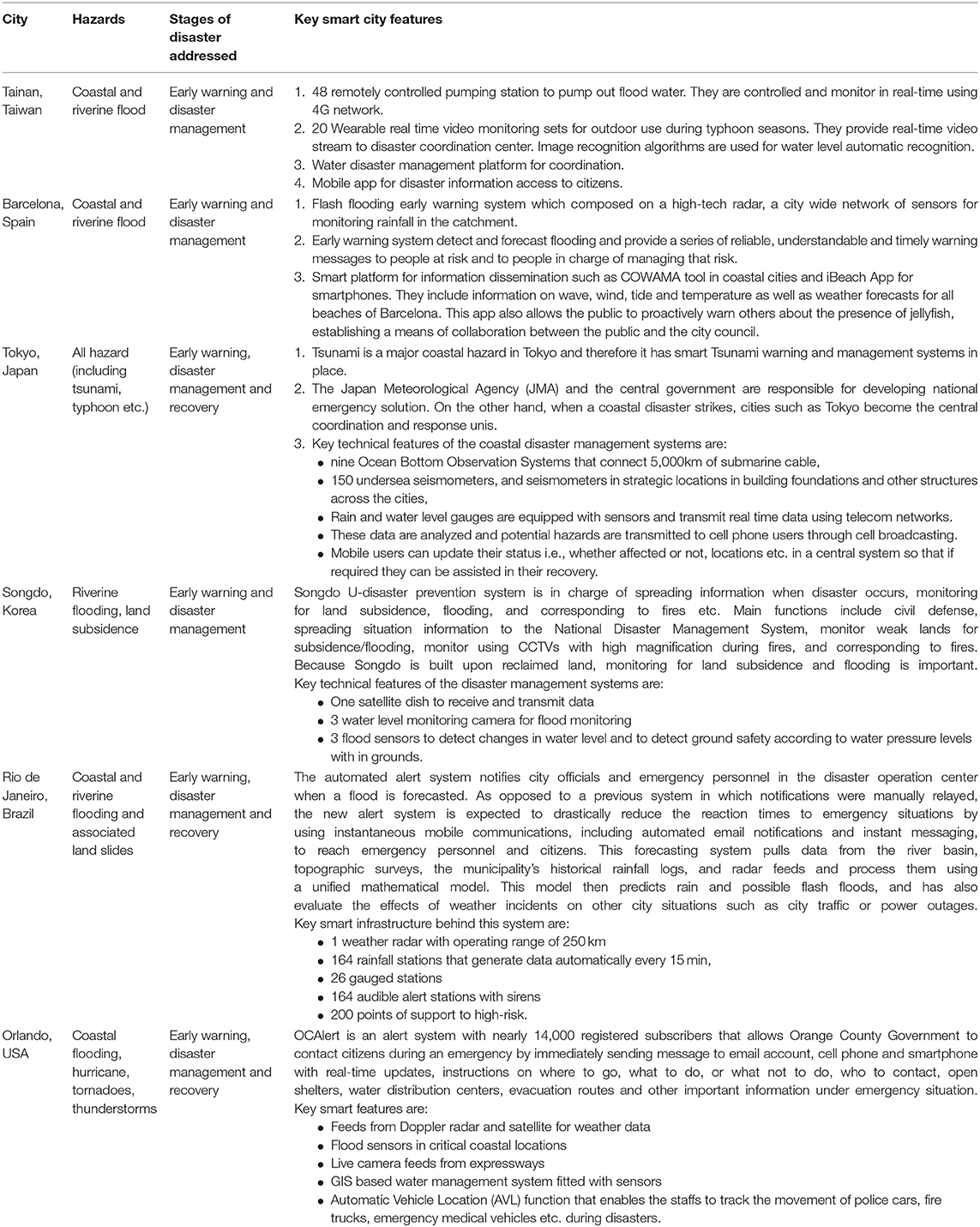
Table 4. Analysis of Smart city case studies for identifying key features of coastal disaster management.
Analysis of Selected Case Studies
Six sample case studies were analyzed to identify what types of coastal disasters were tackled by cities using smart systems, at what stages of the disaster were they used (e.g., early warning, during disaster, disaster recovery etc.), and what smart city features and systems were implemented in the city (Table 4). Flooding (coastal and riverine), cyclone (typhoon, hurricane, tornado, and thunderstorm), tsunami, and landslide have been tacked by these cities. Early warning systems of these hazards were most benefited as information about these hazards were collected, analyzed and communicated to citizens for better preparation. As an example, Tokyo's smart disaster early warning system covers a range of hazards, not just coastal, including earthquake. Technology vendor NEC has developed Tokyo's disaster resilience solution which includes observation systems, information gathering capabilities, data analysis and decision-making aids, together with an intelligent warning system, all linked together in an interoperable manner.
Another example is from Rio De Janeiro, which has a central disaster coordination and operation center. Since opening, the Rio Operations Center has integrated information and processes from across 30 different city agencies into a single operations center that provides a holistic view of how the city is functioning on a 24 h basis. The Operations Center serves as the nerve center for the city, applying analytical models developed by IBM to more effectively predict and coordinate disaster management.
Discussion
Increased potential of natural disaster events as a result of climate change along with rapid development and population growth in coastal cities have created significant risks and challenges for disaster management authorities. Rapid improvement in IT systems has fuelled growing interest in designing and implementing disaster management systems which can predict climatic conditions based on near real-time information, analyse them and communicate with citizens so that disaster impacts can be minimized. To this end a range of innovative systems, tools etc. are developed by the research and academic sector and some of them are making their way to practical city scale implementation. Among these, “smart-city” concept or framework is growing in interest among disaster management professionals, specifically in developed countries. A “smart-city” concept uses embedded sensors, live camera, radar to collect near real-time information and further analyse them to generate valuable insights for disaster management professionals. In the earlier part of the paper, our analysis of a selected sample of peer reviewed academic papers as well as gray unpublished literature revealed some interesting facts about using ICT based smart city frameworks in disaster management and resilience.
Trends and Gaps
The present review highlights the lack of scalable smart city technologies for improving coastal disaster resilience, and as a result, the lack of at scale implementation of smart city frameworks from a coastal disaster resilience perspective. Our findings are summarized below:
• Various smart city technologies, platforms, analysis techniques and applications that are relevant to coastal disaster resilience have been identified. It is clear that the choice and appropriateness of the technology should be judged under the decision-making contexts for which it is developed (i.e., early warning or pre-disaster stage, disaster management when the disaster is unfolding or post disaster recovery etc.). Future applications and/or further development of a smart city tool for coastal disaster resilience depend on the specific decision-making context. Thus, the lens of decision-making contexts is absolutely necessary to assess the appropriateness of a smart city technology.
• While comparing the analysis findings of both peer reviewed literature and city scale case studies, it is clear that while peer reviewed literature is testing and trialing new approaches and techniques, city scale applications are leaning toward more matured approaches and technologies. As an example, in our study sample of the academic literature an innovative CCTV enabled and machine learning based visual monitoring system was used for automating and process real time flood monitoring using image processing. Whereas, this approach did not proliferate in our city scale case studies as cities mostly used conventional sensors. While, conventional sensing networks can only offer one-dimensional physical parameters measured by gauge sensors, visual sensors can acquire dynamic image information of monitored sites and provide disaster prevention agencies with actual field information for decision-making to relieve flood hazards (Lo et al., 2015).
• At present, there is a scarcity of studies investigating and applying smart city technologies for managing coastal hazards at a local government scale. However, coastal local governments commonly face such issues related to the management and planning for potential coastal hazards. As sea-level rises, this issue is likely to become more critical for coastal local governments (Torabi et al., 2017; Tonmoy et al., 2018).
• In peer reviewed literature “Crisis informatics” has become an emerging smart city framework that develops analytics approaches to extract, analyze, and predict online activities (e.g., tweets and Facebook posts) to address challenges in disaster warning, response, and recovery operations (Palen and Anderson, 2016). For instance, during a disaster, disseminating information effectively and timely plays a critical role in spreading awareness in a community. It requires a range of delivery techniques to reach the target audience using different media and communication means. Online social media (such as Facebook, Twitter) can serve as alternative channels to disseminate information to a wider audience. Applications of such crisis informatics can be found in many recent studies using social media data during disasters (Kryvasheyeu et al., 2015; Thom et al., 2016; Sadri et al., 2018). Since crisis informatics is a relatively new field investigating the role of social media during disasters, most studies commonly focus on high-level correlations among the variables of interest when analyzing large-scale data sets (Palen and Anderson, 2016). However, many other questions (Gladwin et al., 2007; Murray-Tuite and Wolshon, 2013) about behavioral and social phenomena, critical to achieving disaster resilience, still remain open. These questions include: how to use social media communications to rapidly identify infrastructure disruption issues and monitor disaster responses and recovery efforts; or how to measure the effectiveness of the available information sources in warning message propagation using the topological properties of the social network observed?
• In city scale implementation case studies:
a) different types of early warning systems are developed for communicating detected coastal hazard (e.g., flood, cyclone) warnings to public through variety of mechanisms such as SMS alerts, social media, sirens etc.
b) different types of remote sensing technologies such as water sensors, radar imagery etc. are used to monitor water levels in flood, tide and rain gauges or to continuously measure the water level variation within a large area (e.g., flood monitoring systems). As an example, flash flood alarming systems require a dense network of rain gauges for monitoring intense local rain storms both to ensure its survival in case of extreme weather and to have a more accurate collection of data. However, those data have to be interpreted using empirical models by correlating in real time the river level and the flow intensity for early flood forecasting and consequent anticipated alarming (Ancona et al., 2015).
c) application of other types of sensors such as temperature sensors, pressure sensors in this domain remain limited.
• One common theme emerged as IoT based software platforms are used in both academic literature and city specific case studies for deploying sensors, communicating collected near-real time data, their storage and analysis.
Lack of Use in Erosion Management
Although coastal erosion has been a major coastal hazard, but it did not appear neither in academic literature nor in city scale case studies. Long-term monitoring of shoreline changes is of significant importance for coastal erosion prediction and coastal planning. Using drones or fixed CCTV camera in combination with image analysis techniques can provide opportunity to implement coastal erosion monitoring of city's most erosion prone beaches (Turner et al., 2016). However, this lack of application of smart city frameworks in coastal erosion management brought up a critical point that time scale of coastal hazard governs the choice of ICT in disaster management. Coastal hazards not only vary in space (e.g., lower areas get inundated in coastal flooding) but also vary in time (e.g., while cyclones can cause inundation of coastal areas within days, increase in sea level rise may cause inundation gradually over decades). The time scale of coastal hazards often determines the choice and characteristics of the ICT feature that are to be used for risk management. As an example, for managing longer-term coastal hazards such as sea level rise, it is important to understand trends and therefore monitoring tides, sea levels, sediment transport etc. are critical with relevant ICT features such as implementation of monitoring systems with sensors being used (Harley et al., 2015). On the other hand, ICT features for managing coastal storms and cyclones require real-time information to feed early warning systems therefore relevant ICT features and sensors with capability to transmit real time information are used.
Not All Smart City Projects Include Smart Disaster Management
ICT have been used for providing early warning to coastal hazards at both global and local scale (Application of local scale mostly in developed countries). Analysis of a sample of smart city gray literature case studies suggest that not all smart city projects around the world have incorporated smart ICT features for managing coastal hazards. There is a chance that not all features get reported in case studies therefore this needs to be further investigated.
Future Directions and Challenges
Major Research Questions
Major Research Questions That Need to Be Addressed Include.
• How can we design and implement a smart city decision support system for coastal hazards which includes the vast range of stakeholder concerns and decision-making contexts related to disaster management?
• How can we develop models to better understand infrastructure interdependency at a local government or city scale and integrate them with smart city platforms?
• Will it be possible or effective to develop one smart city tool that can satisfactorily address all the key disaster management issues in coastal areas, or will it be more effective to create an ensemble of smart city platforms with properly designed interfaces allowing information and decision exchange among various platforms?
• How can we create a smart city platform with linked models and data interoperability for modeling coastal hazards and use them for the development of smart decision making under different types of disaster management contexts (pre disaster, during disaster, and post disaster) in coastal areas?
Opportunities for Implementing Smart City Framework for Coastal Disaster Management
Use of a smart city framework provides a basis for monitoring coastal activities and there are significant opportunities to integrate them with computational models of floods (coastal, riverine, Tsunami) and erosion. These models are developed and validated and used for identifying hazard prone areas. Combining these models with real-time information using smart city frameworks can provide opportunity to disaster managers' to dynamically characterize coastal hazards as they unfold. This is especially critical for cyclone or hurricane as their track changes dynamically and so does areas that are likely to get affected by the cyclone or hurricane. Combining validated numerical hydrodynamic model of coastal inundation with real-time information of cyclone track can assist disaster managers to prioritize evacuation dynamically as cyclone changes its track. Not only cyclones, flood models can also be integrated with smart systems to understand concurrent events of catchment flooding and coastal surge. All of this knowledge can then be integrated with socioeconomic and demographic information (i.e., location of vulnerable populations, critical infrastructure, old structure etc.) to better inform disaster management.
Challenges for Implementing Smart City Framework for Coastal Disaster Management
One of the main driving factors of this framework is power and communication systems which are vulnerable to any man-made or natural disasters including coastal ones. If we lose either we will lose the effectiveness of the whole system. Use of these smart systems will increase in future in managing different infrastructure layers and the connectivity will only increase. Smart city technologies will make different city functions more interdependent. Emergency services depending on data analytics or artificial intelligence will have to depend on the availability of the sensors. However, these interdependencies will be a major challenge in future as there are gaps in our understanding about infrastructure interdependency at a local scale that are arising from these smart infrastructures (Tonmoy and El-Zein, 2013; Hasan et al., 2015; Ersoy, 2017).
Although academic literature is developing innovative new smart systems and analysis techniques, they are often designed and tested in isolation. Integration of these systems to serve a common goal such as disaster management at a city scale across different city systems still remains an unresolved challenge. Transferring a newly developed prototype of smart technology for disaster management into a real-life city scale implementation to inform existing disaster management (DM) decision-making protocol is difficult. DM sector is often very hierarchical and often can be resistant toward getting information from different sources that might affect central command under emergency situation. At the same time, multi-asset integration of disaster management is challenged by data sharing although some progress has been made. As an example, safe data sharing initiative has been initiated and implemented across multiple infrastructure operators in Australia to support managing critical infrastructure during disaster events (Australian Government: Department of Infrastructure, 2018).
Because of the complexity of the disaster management issues in the coastal region and a diverse range of stakeholders and available smart systems, it will be a nearly impossible task to build a super-smart city platform to support a wide range of disaster management related decision-makers. Instead, a more pragmatic approach will be to build interfaces amongst different aspects of the problem linking models, platforms and their outputs. Such an interoperable approach of decision support system will be able to fit different smart technologies, analysis techniques in the decision support platform depending on the disaster management decision context. Also, there is a need for a consistent benchmark of relevant data sets to be able to integrate them within smart city compatible decision support systems.
Conclusions
A disaster resilience framework has been presented to assess current state of research and application of smart city frameworks in disaster management within coastal cities. A set of academic literature and city specific application case studies were reviewed. We find that smart city technologies such as internet of things (IoT) and crisis informatics have significant potential and have been increasingly used in academic studies but their city-scale applications in coastal disaster management have been limited. We have identified critical gaps, RandD needs and practical challenges to foster development of smart city oriented decision support systems for coastal disaster management.
Author Contributions
FT research idea and manuscript preparation. SH and RT research idea enhancement and review of draft.
Conflict of Interest
The authors declare that the research was conducted in the absence of any commercial or financial relationships that could be construed as a potential conflict of interest.
References
Aerts, J. C., Botzen, W. W., Emanuel, K., Lin, N., de Moel, H., and Michel-Kerjan, E. O. (2014). Evaluating flood resilience strategies for coastal megacities. Science 344, 473–475. doi: 10.1126/science.1248222
Ai, F., Comfort, L. K., Dong, Y., and Znati, T. (2016). A dynamic decision support system based on geographical information and mobile social networks: a model for tsunami risk mitigation in Padang, Indonesia. Safety Sci. 90, 62–74. doi: 10.1016/j.ssci.2015.09.022
Aimmanee, S., Ekkawatpanit, C., and Asanuma, H. (2016). Smart disaster mitigation in Thailand. Paper Presented at the Sensors and Smart Structures Technologies for Civil, Mechanical, and Aerospace Systems. doi: 10.1117/12.2219206
Alazawi, Z., Abdljabar, M. B., Altowaijri, S., Vegni, A. M., Mehmood, R. (2012). “ICDMS: an intelligent cloud based disaster management system for vehicular networks,” in Communication Technologies for Vehicles. Nets4Cars/Nets4Trains 2012. Lecture Notes in Computer Science, Vol. 7266, eds A. Vinel, R. Mehmood, M. Berbineau, C. R. Garcia, C. M. Huang, and N. Chilamkurti (Berlin: Springer).
Alazawi, Z., Alani, O., Abdljabar, M. B., Altowaijri, S., and Mehmood, R. (2014). A smart disaster management system for future cities. Paper Presented at the Proceedings of the 2014 ACM International Workshop on Wireless and Mobile Technologies for Smart Cities. doi: 10.1145/2633661.2633670
Alazawi, Z., Altowaijri, S., Mehmood, R., and Abdljabar, M. B. (2011). Intelligent disaster management system based on cloud-enabled vehicular networks. Paper Presented at the ITS Telecommunications (ITST), 2011 11th International Conference on. doi: 10.1109/ITST.2011.6060083
Anbalagan, B., and Valliyammai, C. (2016). “ChennaiFloods: leveraging human and machine learning for crisis mapping during disasters using social media,” in 2016 IEEE 23rd International Conference on High Performance Computing Workshops (HiPCW) (Hyderabad), 50–59.
Ancona, M., Dellacasa, A., Delzanno, G., Camera, A., and Rellini, I. (2015). An “Internet of Things” vision of the flood monitoring problem. Paper Presented at the Performance: The Fifth International Conference on Ambient Computing, Applications, Services and Technologies (Nice).
Anthopoulos, L. G. (2015). “Understanding the smart city domain: a literature review,” in Transforming City Governments for Successful Smart Cities. Public Administration and Information Technology, Vol. 8, ed M. Rodríguez-Bolívar (Cham: Springer).
Attwood, A., Merabti, M., Fergus, P., and Abuelmaatti, O. (2011). Sccir: Smart cities critical infrastructure response framework. Paper Presented at the Developments in E-systems Engineering (DeSE). doi: 10.1109/DeSE.2011.112
Australian Government: Department of Infrastructure, R. D. a. C. (2018). National Infrastructure Data Collection and Dissemination Plan. Available online at: https://bitre.gov.au/data_dissemination/files/National_Infrastructure_Data_Collection_and_Dissemination_Plan.pdf (accessed January 11, 2019).
Bakici, T., Almirall, E., and Wareham, J. (2013). A smart city initiative: the case of Barcelona. J. Knowl. Econ. 4, 135–148. doi: 10.1007/s13132-012-0084-9
Basu, M., Bandyopadhyay, S., and Ghosh, S. (2016). Post disaster situation awareness and decision support through interactive crowdsourcing. Proc. Eng. 159, 167–173. doi: 10.1016/j.proeng.2016.08.151
Batty, M., Axhausen, K. W., Giannotti, F., Pozdnoukhov, A., Bazzani, A., Wachowicz, M., et al. (2012). Smart cities of the future. Eur. Phys. J. Spec. Top. 214, 481–518. doi: 10.1140/epjst/e2012-01703-3
Bhamra, R., Dani, S., and Burnard, K. (2011). Resilience: the concept, a literature review and future directions. Int. J. Prod. Res. 49, 5375–5393. doi: 10.1080/00207543.2011.563826
Birkmann, J. (2006). “Measuring vulnerability to promote disaster-resilient societies: conceptual frameworks and definitions,” in Measuring Vulnerability to Natural Hazards: Towards Disaster Resilient Societies. (Tokyo: United Nations university press) 1, 7–54.
Caragliu, A., Del Bo, C., and Nijkamp, P. (2011). Smart cities in Europe. J Urban Technol. 18, 65–82. doi: 10.1080/10630732.2011.601117
Choi, S., and Bae, B. (2015). “The real-time monitoring system of social big data for disaster management,” in Computer Science and its Applications (Springer), 809–815. doi: 10.1007/978-3-662-45402-2_115
Cimellaro, G. P., Reinhorn, A. M., and Bruneau, M. (2010). Framework for analytical quantification of disaster resilience. Eng. Struct. 32, 3639–3649. doi: 10.1016/j.engstruct.2010.08.008
Cocchia, A. (2014). “Smart and digital city: a systematic literature review,” in Smart City (Springer), 13–43. doi: 10.1007/978-3-319-06160-3_2
Diane, C., and Meier, P. (2009). New Technologies in Emergencies and Conflicts: The Role of Information and Social Networks. Washington, DC; London: UN Foundation-Vodafone Foundation Partnership.
Dunaway, M., Murphy, R., Venkatasubramanian, N., Palen, L., and Lopresti, D. (2017). Research agenda in intelligent infrastructure to enhance disaster management, community resilience and public safety. arXiv [preprint] arXiv:1705.01985.
Ersoy, A. (2017). Smart cities as a mechanism towards a broader understanding of infrastructure interdependencies. Reg. Stud. Reg. Sci. 4, 26–31. doi: 10.1080/21681376.2017.1281154
Francis, R., and Bekera, B. (2014). A metric and frameworks for resilience analysis of engineered and infrastructure systems. Reliab. Eng. Syst. Safe. 121(Suppl. C), 90–103. doi: 10.1016/j.ress.2013.07.004
Gladwin, H., Lazo, J. K., Morrow, B. H., Peacock, W. G., and Willoughby, H. E. (2007). Social science research needs for the hurricane forecast and warning system. Nat. Hazards Rev. 8, 87–95. doi: 10.1061/(ASCE)1527-6988(2007)8:3(87)
Gupta, R., and Gupta, R. (2016). ABC of Internet of Things: advancements, benefits, challenges, enablers and facilities of IoT. Paper Presented at the Colossal Data Analysis and Networking (CDAN), Symposium. doi: 10.1109/CDAN.2016.7570875
Hallegatte, S., Green, C., Nicholls, R. J., and Corfee-Morlot, J. (2013). Future flood losses in major coastal cities. Nat. Clim. Change 3, 802–806. doi: 10.1038/nclimate1979
Harley, M. D., Turner, I. L., Short, A. D., Bracs, M. A., Phillips, M. S., Simmons, J. A., et al. (2015). Four decades of coastal monitoring at Narrabeen-Collaroy Beach: the past, present and future of this unique dataset. Paper presented at the Australasian Coasts & Ports Conference 2015: 22nd Australasian Coastal and Ocean Engineering Conference and the 15th Australasian Port and Harbour Conference.
Hasan, S., Tonmoy, F., Foliente, G., and El-Zein, A. (2015). Modelling infrastructure interdependency at a local scale: value, methodologies and challenges. Paper presented at the 21st International Congress on Modelling and Simulation (Gold Coast, QLD).
Hernández-Nolasco, J. A., Ovando, M. A. W., Acosta, F. D., and Pancardo, P. (2016). Water level meter for alerting population about floods. Paper presented at the Advanced Information Networking and Applications (AINA), 2016 IEEE 30th International Conference on. doi: 10.1109/AINA.2016.76
Hinkel, J., Lincke, D., Vafeidis, A. T., Perrette, M., Nicholls, R. J., Tol, R. S., et al. (2014). Coastal flood damage and adaptation costs under 21st century sea-level rise. Proc. Natl. Acad. Sci. U.S.A. 111, 3292–3297. doi: 10.1073/pnas.1222469111
Hollands, R. G. (2008). Will the real smart city please stand up? Intelligent, progressive or entrepreneurial? City 12, 303–320. doi: 10.1080/13604810802479126
Kawai, J., Mitsuhara, H., and Shishibori, M. (2015). Tsunami evacuation drill system using smart glasses. Proc. Comput. Sci. 72, 329–336. doi: 10.1016/j.procs.2015.12.147
Khan, H., Vasilescu, L. G., and Khan, A. (2008). Disaster management cycle-a theoretical approach. J. Manag. Mark. 6, 43–50.
Kitada, S., Sato, G., and Shibata, Y. (2017). “Performance evaluation of a DTN based multi-hop network for disaster information transmission by smart devices,” in International Conference on Broadband and Wireless Computing, Communication and Applications (Cham: Springer). doi: 10.1016/B978-0-12-809859-2.00004-8
Kitchin, R. (2014). The real-time city? Big data and smart urbanism. GeoJournal 79, 1–14. doi: 10.1007/s10708-013-9516-8
Kitchin, R. (2015). Making sense of smart cities: addressing present shortcomings. Camb. J. Regions, Econ. Soc. 8, 131–136. doi: 10.1093/cjres/rsu027
Kong, J., and Simonovic, S. P. (2019). Probabilistic multiple hazard resilience model of an interdependent infrastructure system. Risk Anal. 39, 1843–1863. doi: 10.1111/risa.13305
Kong, J., Simonovic, S. P., and Zhang, C. (2019). Resilience assessment of interdependent infrastructure systems: a case study based on different response strategies. Sustainability 11:6552. doi: 10.3390/su11236552
Kryvasheyeu, Y., Chen, H., Moro, E., Van Hentenryck, P., and Cebrian, M. (2015). Performance of social network sensors during hurricane sandy. PLoS ONE 10:e0117288. doi: 10.1371/journal.pone.0117288
Kumar, A. V., Girish, B., and Rajesh, K. R. (2015). Integrated weather & flood alerting system. Int. Adv. Res. J. Sci. Eng. Technol. 2, 21–24.
Lo, S.-W., Wu, J.-H., Lin, F.-P., and Hsu, C.-H. (2015). Visual sensing for urban flood monitoring. Sensors 15, 20006–20029. doi: 10.3390/s150820006
Manyena, S. B. (2006). The concept of resilience revisited. Disasters 30, 434–450. doi: 10.1111/j.0361-3666.2006.00331.x
Meijer, A., and Bolívar, M. P. R. (2016). Governing the smart city: a review of the literature on smart urban governance. Int. Rev. Admin. Sci. 82, 392–408. doi: 10.1177/0020852314564308
Murayama, Y., Velev, D., Zlateva, P., and Gonzalez, J. J. (2017). Information Technology in Disaster Risk Reduction: First IFIP TC 5 DCDRR International Conference, ITDRR 2016. Sofia: Springer.
Murray-Tuite, P., and Wolshon, B. (2013). Evacuation transportation modeling: an overview of research, development, and practice. Transp. Res. C 27, 25–45. doi: 10.1016/j.trc.2012.11.005
Ogie, R. I., Shukla, N., Sedlar, F., and Holderness, T. (2017). Optimal placement of water-level sensors to facilitate data-driven management of hydrological infrastructure assets in coastal mega-cities of developing nations. Sustain. Cities Soc. 35, 385–395. doi: 10.1016/j.scs.2017.08.019
Palen, L., and Anderson, K. M. (2016). Crisis informatics—New data for extraordinary times. Science 353, 224–225. doi: 10.1126/science.aag2579
Sadri, A. M., Hasan, S., Ukkusuri, S. V., and Cebrian, M. (2018). Crisis communication patterns in social media during Hurricane Sandy. Transp. Res. Rec. 2672, 125–137. doi: 10.1177/0361198118773896
Sanchez, L., Muñoz, L., Galache, J. A., Sotres, P., Santana, J. R., Gutierrez, V., et al. (2014). SmartSantander: IoT experimentation over a smart city testbed. Comput. Networks 61, 217–238. doi: 10.1016/j.bjp.2013.12.020
Scuotto, V., Ferraris, A., and Bresciani, S. (2016). Internet of Things: applications and challenges in smart cities: a case study of IBM smart city projects. Bus. Process Manag. J. 22, 357–367. doi: 10.1108/BPMJ-05-2015-0074
Shalini, E., Surya, P., Thirumurugan, R., and Subbulakshmi, S. (2016). Cooperative flood detection using SMS through IoT. Int. J. Adv. Res. Elect. Electron. Instrum. Eng. 5, 3410–3414.
Thom, D., Krüger, R., and Ertl, T. (2016). Can Twitter save lives? A broad-scale study on visual social media analytics for public safety. IEEE Trans. Vis. Comput. Graph. 22, 1816–1829. doi: 10.1109/TVCG.2015.2511733
Tonmoy, F., and El-Zein, A. (2013). Vulnerability of infrastructure to Sea Level Rise: a combined outranking and system-dynamics approach. Paper presented at the European Safety and Reliability (ESREL-2013) (Amsterdam). doi: 10.1201/b15938-359
Tonmoy, F. N., El-Zein, A., and Hinkel, J. (2014). Assessment of vulnerability to climate change using indicators: a meta-analysis of the literature. Wiley Interdiscip. Rev. Clim. Change 5, 775–792. doi: 10.1002/wcc.314
Tonmoy, F. N., Wainwright, D., Verdon-Kidd, D. C., and Rissik, D. (2018). An investigation of coastal climate change risk assessment practice in Australia. Environ. Sci. Policy, 80, 9–20. doi: 10.1016/j.envsci.2017.11.003
Torabi, E., Dedekorkut-Howes, A., and Howes, M. (2017). Not waving, drowning: can local government policies on climate change adaptation and disaster resilience make a difference? Urban Policy Res. 35, 312–332. doi: 10.1080/08111146.2017.1294538
Turner, I. L., Harley, M. D., and Drummond, C. D. (2016). UAVs for coastal surveying. Coast. Eng. 114, 19–24. doi: 10.1016/j.coastaleng.2016.03.011
Tyler, S., and Moench, M. (2012). A framework for urban climate resilience. Clim. Dev. 4, 311–326. doi: 10.1080/17565529.2012.745389
United Nations, Department of Economic and Social Affairs, and Population Division (2019). World Urbanization Prospects: The 2018 Revision (ST/ESA/SER.A/420). New York, NY: United Nations.
Wiig, A. (2015). IBM's smart city as techno-utopian policy mobility. City 19, 258–273. doi: 10.1080/13604813.2015.1016275
Keywords: coastal disaster, smart city, resilience, vulnerability, internet of things, crisis informatics
Citation: Tonmoy FN, Hasan S and Tomlinson R (2020) Increasing Coastal Disaster Resilience Using Smart City Frameworks: Current State, Challenges, and Opportunities. Front. Water 2:3. doi: 10.3389/frwa.2020.00003
Received: 30 June 2019; Accepted: 17 February 2020;
Published: 10 March 2020.
Edited by:
Ronita Bardhan, University of Cambridge, United KingdomReviewed by:
Subimal Ghosh, Indian Institute of Technology Bombay, IndiaAndre Schardong, University of Western Ontario, Canada
Copyright © 2020 Tonmoy, Hasan and Tomlinson. This is an open-access article distributed under the terms of the Creative Commons Attribution License (CC BY). The use, distribution or reproduction in other forums is permitted, provided the original author(s) and the copyright owner(s) are credited and that the original publication in this journal is cited, in accordance with accepted academic practice. No use, distribution or reproduction is permitted which does not comply with these terms.
*Correspondence: Fahim Nawroz Tonmoy, fahim.tonmoy@hotmail.com