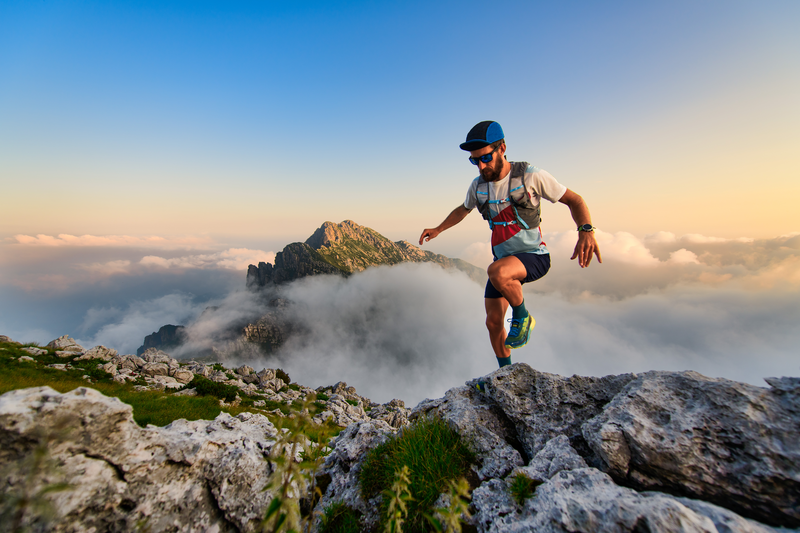
94% of researchers rate our articles as excellent or good
Learn more about the work of our research integrity team to safeguard the quality of each article we publish.
Find out more
ORIGINAL RESEARCH article
Front. Virtual Real.
Sec. Virtual Reality and Human Behaviour
Volume 6 - 2025 | doi: 10.3389/frvir.2025.1469409
The final, formatted version of the article will be published soon.
You have multiple emails registered with Frontiers:
Please enter your email address:
If you already have an account, please login
You don't have a Frontiers account ? You can register here
This paper presents a Google Cardboard VR simulation to raise awareness among participants aged 11 to 16 about marine ecosystem protection. Focusing on the impact of everyday pollutants on sea turtles, a user study (N=12) gauged the VR sessions' impact on student motivation. Mixed-effects modeling was conducted on Likert scale ratings on both the pre-and post-session surveys, finding scores for motivation to learn about nature-and VR-related topics to be significantly higher than 3 ("Neither agree or disagree") after the VR simulation, while also showing that the simulation had some impact on increasing participant interest across both environmental and VR topics. Mixed-effects modeling conducted on Likert scale ratings from the pre-and post-session surveys found significant evidence of above average motivation to learn about nature related topics after the VR simulation. Qualitative feedback emphasized the desire for more interactivity, improved graphics, and enhanced authenticity. Despite these suggestions, participants effectively grasped the educational message, highlighting VR's potential in environmental conservation education. This study's findings underscore the potential of VR-based educational tools in fostering a deeper understanding and commitment among young students towards environmental stewardship, suggesting a promising avenue for integrating technology into environmental education curricula..
Keywords: Educational game-based learning, environmental awareness, virtual reality, Mobile VR, user study
Received: 23 Jul 2024; Accepted: 21 Feb 2025.
Copyright: © 2025 Roy, Sparra, Skripnikov and Gilchrist. This is an open-access article distributed under the terms of the Creative Commons Attribution License (CC BY). The use, distribution or reproduction in other forums is permitted, provided the original author(s) or licensor are credited and that the original publication in this journal is cited, in accordance with accepted academic practice. No use, distribution or reproduction is permitted which does not comply with these terms.
* Correspondence:
Tania Roy, New College of Florida, Sarasota, United States
Disclaimer: All claims expressed in this article are solely those of the authors and do not necessarily represent those of their affiliated organizations, or those of the publisher, the editors and the reviewers. Any product that may be evaluated in this article or claim that may be made by its manufacturer is not guaranteed or endorsed by the publisher.
Research integrity at Frontiers
Learn more about the work of our research integrity team to safeguard the quality of each article we publish.