- Department of Psychology, Clinical Psychology and Psychotherapy, University of Regensburg, Regensburg, Germany
Background: Driving simulators are established tools used for different research purposes. Either head-mounted displays (HMDs) or monitors are applied. However, the extent to which the setting (HMD vs monitors) or the interactivity in driving (active behaviour as a driver or passive behaviour as a co-driver) influences the experience of presence, realism or simulator sickness is not yet entirely clear.
Objective: We aimed to compare different conditions to assess the influence of interactivity and presentation setting on the experience of presence, realism, and simulator sickness. Further, different driving performance parameters (e.g., speed perception or sign detection) were aimed to be assessed between the two settings.
Methods: Ninety participants were recruited. This was an experimental study (2 × 2 within-between-subject design) with interactivity as within-subject factor (2 levels: active vs passive) and setting as between-subject factor (2 levels: HMD vs monitors). As driving scenarios, we selected four specific driving maneuvers that we assume would affect driving behaviour differently depending on the setting. Differences in the experience of presence as well as in the development of simulator sickness were assessed using standardised questionnaires.
Results: There was no difference between the two settings regarding presence, realism or simulator sickness. Regarding interactivity, presence was significantly higher in the active compared to the passive condition. The most prominent difference between the two settings was found in the sign detection task where participants wearing an HMD performed worse.
Conclusion: The choice of interactivity appears to have a stronger effect on the experience of presence than the setting. Sign detection was worse in the HMD setting probably due to the lower display resolution. These results are relevant for the interpretation of driving simulator studies and the implementation of future studies.
Background
The use of simulators in experimental studies offers many advantages compared to real objects or situations. For example, the simulated environment can be kept consistent for each participant, allowing only the independent variable to be varied. In addition, scenarios that could be dangerous in real life (such as driving situations) can be recreated in virtual reality (Blissing et al., 2022; Ryan et al., 2019). It is aimed to provide the user with a realistic impression of the displayed scenario. Driving simulators are used in a wide range of applications, from driver education and training to entertainment and research. The visual environment is presented with head-mounted displays (HMDs), screens/monitors, projectors or even with LED walls (Aykent et al., 2015; Blissing et al., 2022; Himmels et al., 2023).
At a first look, HMDs seem to have many advantages compared to displays, projectors or screens: being more compact, having a lower hardware cost or providing a user-friendly installation. Moreover, only a steering wheel and pedals (and no complete car dummies as often used when monitors or projectors are applied) are required since all parts of the vehicle can be displayed virtually (Himmels et al., 2023). Speed perception is probably very similar to real conditions and due to additional depth cues with an HMD, the position and orientation of the vehicle should be better estimated with an HMD than projectors or monitors (Blissing et al., 2022; Renner et al., 2013). Nevertheless, there are also some challenges that should be reported. First of all, a certain level of technological skills is required (Blissing and Bruzelius, 2018). Second, HMDs are more susceptible to latency during rapid head turns (Brooks et al., 2012). Similar to head turns, rapid sideways movements of the vehicle also cause the entire scene to move on the retina. Discrepancies between the visual feedback and the motion feedback can increase simulator sickness (Blissing et al., 2022). Visual acuity appears to be better in projector- or screen-based simulators (Blissing et al., 2022; Zöller et al., 2019). A further disadvantage of HMDs cited in the literature is that they hide their own body. For instance, participants cannot see their hands on the steering wheel. They can only feel it. For this reason, the participants have to rely solely on their proprioception, which can lead to sensory errors (Blissing et al., 2022). Mismatches between visual and proprioceptive inputs can negatively affect user performance and the sense of immersion in virtual reality environments (Deligiannidis et al., 2009). Finally, participants are subjected to additional weight and often extra cables, which can result in an unnatural feeling and reduced head movement (Himmels et al., 2023). A limitation of screens is the fact that boundaries are usually visible when multiple monitors are combined, which may lead to reduced immersion.
Presence refers to the feeling that the participants subjectively perceive themselves as existing in the environment (even if they know objectively that this environment is not real and that they are physically in a different environment) (Kim and Park, 2020; Witmer and Singer, 1998). It is described as a human reaction to immersion. While immersion refers to the objective, sensory depth of the virtual reality experience, presence describes the participants subjective feeling of actually being in the virtual world. In HMDs, participants are fully surrounded by the virtual environment meaning that immersion is greater compared to screen-based settings. This fact can be associated with a higher experience of presence as a result (Slater, 2003). In the Presence Questionnaire (PQ) or rather the IGroup Presence Questionnaire (IPQ), for instance, presence is divided in four categories: general presence, spatial presence, involvement and experienced realism (Schubert et al., 2001; Witmer and Singer, 1998). Realism is considered as a sub-domain of presence, even if it is sometimes recorded separately (Blissing et al., 2022). Perceived realism is defined by the subjective degree of reality of the depicted virtual environment and its overall plausibility and credibility. Thus, the experience of presence in a virtual world is conceived as a composite of being there and perceived realism (Weber et al., 2021). In the literature, there is evidence that the presentation setting did not influence the experience of presence (Aykent et al., 2015; Himmels et al., 2023) or realism. However, in a flight simulation study, spatial presence and the experience of realism were improved with HMDs compared to screens (Kim and Park, 2020).
One challenge with simulators is the fact that some participants are more susceptible to physical symptoms such as simulator sickness when there are conflicting sensory impressions from the visual and vestibular systems (Reason and Brand, 1975). The extent to which the type of presentation (HMD vs projectors, screens, displays or LED walls) influences simulator sickness is not yet entirely clear. While there are studies reporting a higher simulator sickness when using HMDs (Aykent et al., 2015; Benz et al., 2019; Cao et al., 2020; Suwarno et al., 2019), there are also references where the presentation setting had no influence on simulator sickness (Blissing et al., 2022; Himmels et al., 2023).
Regarding the interactivity of driving, meaning being the driver in comparison of being just the co-driver or passenger, there is some literature that motion sickness is less apparent in drivers. One possible reason is the feeling of control since drivers are having a direct connection between their actions and the reactions of the car (Rolnick and Lubow, 1991). The lack of active motor control can often lead to a sensory conflict between the sense of balance and what the eyes perceive. Active vs passive exposure to sensory conflicts in different contexts has been thoroughly described in the literature over the last decades (Cressman and Henriques, 2010).
The main question to be investigated in this study is how an HMD affects the experience of presence compared to monitors in two interactivity conditions (active driving vs passive driving as a co-driver). This means, that in the active condition, the participant is the driver of the car, whereas in the passive condition, the participant sits in the passenger seat. We assume that the experience of presence, realism, and simulator sickness are significantly more pronounced with the HMD than with monitors in both interactivity conditions. Furthermore, we expect the active condition to lead to increased experiences of presence and realism, and decreased simulator sickness, compared to the passive condition. Regarding simulator sickness, it is hypothesized that this occurs significantly more frequently and is more pronounced in the passive condition, as the participants may be more distracted and concentrated and experience a higher feeling of control in the active condition, regardless of the setting. Additionally, we hypothesise that the difference in presence between HMD and monitor setting is significantly smaller in the active condition than in the passive condition. As an additional part of this study, we aimed to replicate the findings of Blissing et al. (2022) who compared different driving tasks between two different display types (HMD vs projectors). In our study, the performance in the same four driving tasks, namely, speed perception, lane change, sign detection and a narrow curve maneuver, will be compared between our two settings (HMD vs monitors). We hypothesize that speed perception is more accurate with HMD than monitors due to additional depth cues from the binocular display system. Therefore, the speed deviation compared to the instructed reference speed is smaller in the HMD setting. We further assume that at low speed, the average deviation from the reference lane is smaller with HMD than with monitors (since the HMD introduces added depth cues). At medium speed, the performance with HMD is worse compared to monitors (since the fast lateral movement may cause the entire scene to move across the retina). We also have the hypothesis that the visual acuity is lower with HMD than with monitors. Therefore, the signs are detected later and thus, the button is pressed closer to the sign in the HMD setting compared to the monitor setting. Regarding the narrow curve maneuver, we assume that due to additional depth cues using an HMD and the ability to turn around the head, participants in the HMD setting have smaller deviations from the reference lane than participants from the monitor setting when driving the curve.
Methods
Participants and recruitment
Ninety-one participants (42 men and 49 women, mean age 25.0 years (SD = 8.3), range: 18–59 years) were recruited. Inclusion criteria were health (self-report) and age between 18 and 65 years of age, valid driving license as well as providing written consent for study participation. Exclusion criteria were non-compensated visual impairment, physical impairments (e.g., coordination problems) and lack of German language skills if this impairs task comprehension. Individuals who reported intake of specific medication (recorded on a list, e.g., strong painkillers or sedating psychotropic drugs) or who indicated a high sensitivity to motion sickness during the study information phase (nausea when driving or flying), as well as women who reported an existing pregnancy, were also excluded. Recruitment was carried out via flyers, the university-specific recruitment platform SONA, social media posts and personal contacts. Data acquisition was conducted from 13th of March to 29th of November 2023 at the University of Regensburg, Germany. All participants received 10 € as a compensation for their participation.
Power analysis
The sample size required to identify a small effect of f = 0.15 with regard to the main question is 90 participants with a power of .80 to be achieved (G*Power; F-tests, ANOVA: repeated-measures, within-between interaction, f = 0.15, α = .05, 1-β = .80, number of groups = 2, number of measurements = 2). A small effect size was assumed since no relevant effect size has been reported in the literature so far.
Study design
This was an experimental study (2 × 2 within-between-subject design) with two conditions regarding interactivity (active behaviour as a driver vs passive behaviour as a co-driver) as within-subject factors and two settings (HMD vs monitor) as between-subject factor.
Procedure
Participants read a written information about the study and gave their written informed consent to participate. After that, the examine administered the tablet-based pre-assessment including demographics, questions about driving experience and competence, fear of driving, simulator sickness, arousal, pleasure and control, and emotions. The participants were then accompanied to the driving simulator and shown how to adjust the seat, etc. When the participants used a HMD, they were instructed how to adjust the device for a firm and comfortable fit. The trial started with three test drives (where the participants could try steering, accelerating and braking) to familiarize with the simulator followed by a quick questionnaire to assess simulator sickness (paper-pencil). After that, participants started either with the active or the passive condition and solved different tasks. In the active condition, participants sat in the driver seat, in the passive condition, participants sat in the passenger seat. Each experimental run was followed by a tablet-based assessment including questions on simulator sickness, presence, realism, arousal, valence, control, and emotions. At the end, two closing questions were presented where participants could indicate whether they enjoyed taking part in the experiment and whether they had any comments on the experiment (see Figure 1). All tablet-based questions were presented with soscisurvey. de.
The route contained four different scenarios (see Figure 3).
1. Speed perception: In the active condition, participants were instructed to drive the prescribed speed (two times 30 km/h, two times 50 km/h and once 70 km/h) according to their feeling while the speedometer was switched off. They were asked to press a button at the steering wheel as soon as they thought they were driving at the corresponding speed. The difference between the target speed and the actual speed was of interest here. In the passive condition, participants were instructed to estimate by pressing buttons whether the car drives too fast (left button), too slow (right button) or just the right speed (middle button) while the speedometer switched off.
2. Lane change (low-speed and medium-speed): Participants were instructed to overtake parked cars at a certain speed (two times 30 km/h and two times 50 km/h) by switching completely to the left lane and back. The deviation from the reference lane was measured as the mean deviation from the centerline of the left lane while overtaking the parking car. Since this was a pure driving task, participants in the passive condition passed this scenario without any instruction, meaning they just watched while an autonomously diving car passed the scenario.
3. Sign detection: For this task, a sign was customized (see Figure 2). In both interactivity conditions, participants were instructed to press a right or left button as soon as they could distinctly identify if the symbol on the sign had its opening on the right or left side. The sign was presented six times along the highway. Distance between the signs was 1 km. The sign was quite large (4.44 m × 4.46 m) to ensure that it was not overlooked by the participants, and thus, to prevent missings. The distance to the sign was measured at the time the button was pressed.
4. Narrow curve maneuver: Participants were instructed to drive two identical hairpin curves as accurately as possible. In order to ensure that the participants stayed in their lane, oncoming traffic approached them as they entered the curve. The mean deviation from the reference lane, i.e., the centerline of the right lane was of interest here. Since this was a pure driving task, participants in the passive condition passed this scenario without any instruction (similar to the lane change task).
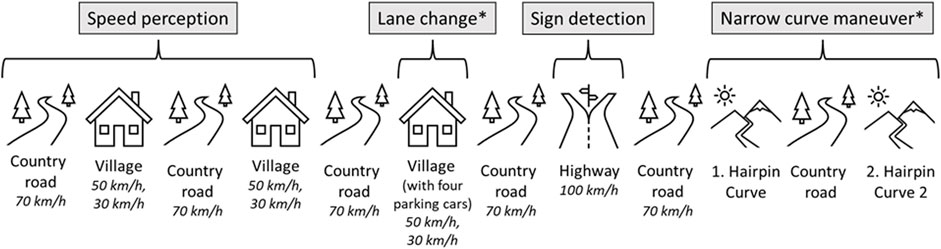
Figure 3. Outline of the route with speed limits. *no task in the passive condition. Abbreviations: km/h = kilometers per hour.
The instruction for the participants was always text-to-speech. The first attempt of each task was not respected in the analysis to control novelty issues of the corresponding tasks. It is important to mention that due to technical reasons the used car models differed between the two settings. While in both settings the car was red, in the monitor setting, a car with closed passenger cabin and analogue speedometer and rev counter was used, while in the HMD setting the car was a convertible with a digital display (see Figure 4). Since the monitor condition has already been running before the HMD condition was finalized, the car from the HMD condition had to be matched as closely as possible to that of the monitor condition which was only partially successful due to technical limitations.
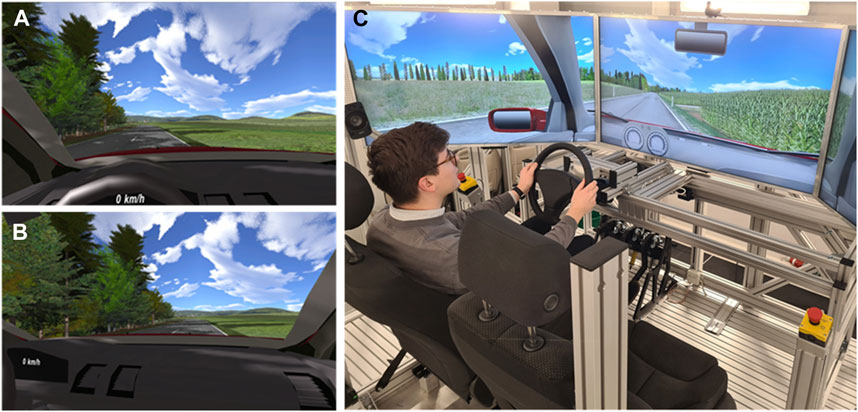
Figure 4. The setup and virtual environments of the HMD-based condition (A): perspective from the driver, (B) perspective from the co-driver) and the setup, here for the monitor-based condition (C).
Questionnaires
Several questionnaires were used before, during, and after the virtual driving experiences.
- Fear of driving was assessed with the German version of the 5-item Instrument for Fear of Driving (IFD) (Fischer et al., 2021) and the 20-item Driving Cognitions Questionnaire (DCQ) (Heider et al., 2018).
- Simulator sickness was assessed using the 16-item Simulator Sickness Questionnaire (SSQ) (Kennedy et al., 1993).
- Arousal, valence and control/dominance were assessed with the three Self-Assessment Manikin (SAM) scales (Bradley and Lang, 1994).
- Positive and negative affect or rather emotions were assessed using the 20-item Positive and Negative Affect Schedule (PANAS) (Breyer and Bluemke, 2016).
- Presence was assessed using two scales à 5 items of the Multimodal Presence Scale (MPS), Physical Presence and Self-Presence (Volkmann et al., 2018).
- Realism was assessed using three self-generated items with a 7-point Likert scale in relation to different aspects (visual and acoustic environment and movements, see Supplementary A1).
Technique
The driving simulator is located in the motion lab at the Department of Psychologie, University of Regensburg. The motion lab is built on a motion system from the company E2M (https://www.e2mtechnologies.eu/). The aluminum construction is a special design with emergency stop buttons on the test person’s seat and on the control table. The motion platform can only be started when the test person is strapped in, the door to the platform is closed and the barrier tape has been tightened. The motion lab has been tested by TÜV. The SILAB driving simulation software was used for the driving simulation (WIVW GmbH, Germany), which was also used to create the individual scenarios. The used HMD was the HTC Vive (HTC Corporation, Taiwan) with 90 Hz. The three screens are ultra-HD monitors with a resolution of 3,840*2,160 pixels and a playback of 60 Hz. For audio, there is a 5.1 system (Logitech, Schweiz) on the motion platform that can be used to play directional sounds.
Randomization
It was planned to randomly assign the participants to one of the two settings (HMD or monitors), separately according to gender, however, the randomization could not be adhered to due to technical problems with the HMD setting. Most of the data of the monitor setting (except for two participants) were collected first, followed by the HMD setting. The interactivity conditions were balanced between the participants. Half of the participants started as a driver (active condition), and the other half started as a co-driver (passive condition).
Data recording, data processing and statistics
Several driving parameters (e.g., measurement time point, speed, steering angle, lateral distance, etc.) were continuously recorded with a frequency of 50 Hz. Data analysis was conducted via IBM SPSS Statistics 29. Alpha level was set to 5%.
Ethics declaration
The study was reviewed and approved by the ethics committee of the University of Regensburg (file number: 22–3,200-101). The experimental procedure was in line with the Declaration of Helsinki. Participants provided written informed consent to participate in this study. The study was conducted according to the approved procedure.
Results
Participants
The demographic characteristics of the study participants are presented in Table 1.
On average, the participants had held their driving license for 7.8 years (range: 1–41 years). None of the participants rated their driving skills as insufficient, 14 participants (15.4%) rated their driving competence as moderate, 64 (70.3%) as fairly competent and 13 (14.3%) as very competent. Sixteen participants (17.6%) reported to drive every day, 38 participants (41.8%) stated to drive several times a week. 8 (8.8%) once a week, 10 (11.0%) several times a month, 9 (9.9%) once a month and 10 participants (11.0%) indicated to drive rarely to never.
Three participants (all women, twice HMD) had to abort the study early due to simulator sickness. Another three participants aborted the study for any reason. Due to technical problems with the HMD, the study had to be aborted twice. In another eight participants the HMD failed a few times during the experimental condition, which led to gaps in the recording. Consequently, the analysis does not contain data from these 16 individuals. For one participant, the first main-assessment was not done, however this participant was not excluded. When analyzing the driving parameters in the active conditions, further four participants had to be excluded for task 1, five participants for task 3 and one participant for task 4. One participant wrongly classified one sign. This value was excluded from the analysis.
Data from 75 participants entered the analysis. The sample size for the monitor setting contained 43 participants (female: 23 (53.5%), mean age: 25.1 (SD = 8.6) years, age range: 18–56 years) and for the HMD setting 32 participants (female: 17 (53.1%), mean age: 24.1 (SD = 7.0) years, age range: 19–59 years).
Both sub-samples were analyzed for significant differences using Mann-Whitney-U-tests. We could not find any significant difference between the two setting conditions for age, gender, mother tongue, education, professional qualification, years of holding a driving license, frequency of driving, subjective diving competence, fear of driving measured by the IFD and DCQ, arousal, valence and control assessed by SAM scales at baseline, positive and negative affect measured by PANAS at baseline and simulator sickness at baseline assessed by the SSQ (all ps ≥ .077). For this reason, it can be assumed that there are no relevant differences between groups in these variables.
Using Shapiro-Wilk-tests, we tested for normal distribution separately for setting and interactivity. The normal distribution assumption was fulfilled for the variable presence (except for physical presence in the active monitor-condition, p = .003) and was partly fulfilled for the realism-variables (HMD setting for movements, p ≥ .103, passive monitor setting for visual environment, p ≥ .085) and some SSQ variables (SSQ total for the HMD setting, p ≤ .076. SSQ oculomotor for the active HMD setting, p ≤ .054). However, ANOVAs with repeated measures are relatively robust against the violation of this assumption (Vasey and Thayer, 1987), a 2 × 2 ANOVA with repeated measures was conducted to test the main hypothesis that the experience of presence is significantly more pronounced in the HMD setting than in the monitor setting.
Main findings
Presence
MPS means for both settings and interactivity conditions are presented in Table 2. For physical presence, the 2 × 2 ANOVA with repeated measures revealed a main effect of interactivity, F (1,74) = 16.75, p < .001, ηp2 = 0.189, and no significant main effect of setting, F (1,74) = 0.25, p = .619, ηp2 = 0.003, or interaction between interactivity and setting, F (1,74) = 0.34, p = .561, ηp2 = 0.005. For self-presence, the results were similar. We found a significant main effect of interactivity, F (1,74) = 18.58, p < .001, ηp2 = 0.205, however no significant main effect of setting, F (1,74) = 1.74, p = .191, ηp2 = 0.024, and no interaction between interactivity and setting, F (1,74) = 0.39, p = .537, ηp2 = 0.005 (see Figure 5).
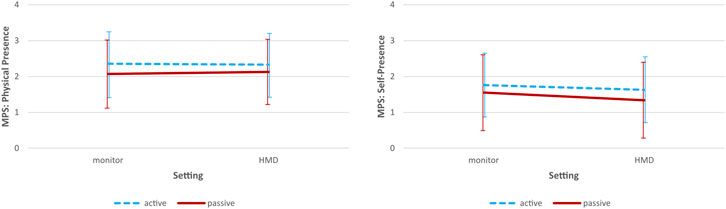
Figure 5. Presence scores for both setting and interactivity conditions (including standard deviations) Abbreviations: HMD = head-mounted display, MPS = Multimodal Presence Scale.
Realism
Means for the three realism sub-scales are presented in Table 2. For the visual environment, there were neither significant main effects for interactivity, F (1,74) = 1.19, p = .278, ηp2 = 0.016, and setting, F (1,74) = 0.36, p = .548, ηp2 = 005, nor a significant interaction between interactivity and setting, F (1,74) = 0.66, p = .418, ηp2 = 0.009. Same for the acoustic environment (interactivity: F (1,74) = 0.67, p = .417, ηp2 = 0.009, setting: F (1,74) = 0.80, p = .374, ηp2 = 0.011, interactivity by setting: F (1,74) = 0.01, p = .912, ηp2 < 0.001) and movements (interactivity: F (1,74) = 1.50, p = .225, ηp2 = 0.020, setting: F (1,74) = 0.02, p = .877, ηp2 < 0.001, interactivity by setting: F (1,74) = 0.57, p = .455, ηp2 = 0.008).
Simulator sickness
Means for the SSQ and its sub-scales are presented in Table 2. For the total scale and its sub-scales we did not find any significant main effects or interaction effects (SSQ total, interactivity: F (1,74) = 2.05, p = .157, ηp2 = 0.028, setting: F (1,74) = 0.27, p = .607, ηp2 = 0.004, interactivity by setting: F (1,74) = 0.12, p = .728, ηp2 = 0.002, oculomotor, interactivity: F (1,74) = 2.08, p = .154, ηp2 = 0.028, setting: F (1,74) = 0.00, p = .966, ηp2 < 0.001, interactivity by setting: F (1,74) = 2.48, p = .120, ηp2 = 0.033, disorientation, interactivity: F (1,74) = 0.00, p = .962, ηp2 < 0.001, setting: F (1,74) = 0.74, p = .393, ηp2 = 0.010, interactivity by setting: F (1,74) = 0.13, p = .722, ηp2 = 0.002, nausea, setting: F (1,74) = 0.54, p = .466, ηp2 = 0.007, interactivity by setting: F (1,74) = 0.27, p = .603, ηp2 = 0.004) except for the nausea subscale, where we found a significant main effect of interactivity (F (1,74) = 5.22, p = .025, ηp2 = 0.068). Exploratorily, we assessed simulator sickness over time to evaluate how strong simulator sickness was induced. Since interactivity was balanced over time, this factor was not considered within this analysis. Simulator sickness was higher after the first and second experimental condition than after baseline. A repeated measures ANOVA with the between-subject factor setting and the within-subject factor time revealed a significant main effect of time, F (1,83) = 33.16, p < .001, ηp2 = 0.290, and no significant main effect of setting, F (1,83) = 0.09, p = .767, ηp2 = 0.001 and no significant interaction, F (1,83) = 2.53, p = .083, ηp2 = 0.030 (see Figure 6). The two experimental conditions did not differ significantly from each other (p ≥ .797), meaning the short break between the active and passive condition did not improve simulator sickness. “Descriptively, the increase in simulator sickness is higher in the HMD setting than in the monitor setting (see in Figure 6). Change scores between T1 and baseline and T2 and baseline, respectively, have been calculated. However, a 2 × 2 ANOVA on the chance scores of the SSQ showed no significant effects of setting, F (1,83) = 3.48, p < .066, ηp2 = 0.041, of time, F (1,83) = 0.09, p = .760, ηp2 = 0.001, or of the interaction of setting and time, F (1,83) < 0.01, p < .997, ηp2 < 0.001.”
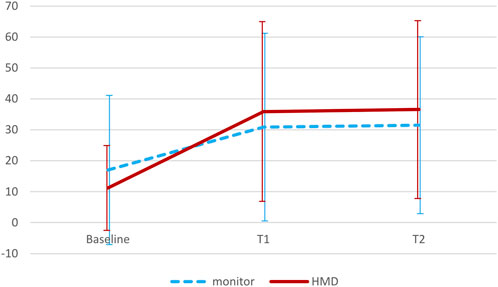
Figure 6. Motion sickness over time (including standard deviations) Abbreviations: T1 = after the first experimental condition, T2 = after the second experimental condition, SSQ = Simulator Sickness Questionnaire, HMD = head-mounted display.
Analysis of driving behavior
Speed perception
The mean speed deviation from the reference speed is presented in Table 3. The lowest deviation was descriptively for both settings at 50 km/h and highest at 70 km/h.
A 2 × 3 mixed ANOVA with the between-subject factor setting (monitor, HMD) and the within-subject factor target speed (50 km/h, 30 km/h, 70 km/h) and the dependent variable deviation from target speed revealed a significant main effect of target speed (F (1,66) = 32.82, p ≤ .001, ηp2 = .339), however no significant main effect of setting (F (1,66) = 0.55, p = .461, ηp2 = .009) and no significant interaction effect (F (1,66) = 1.08, p = .343, ηp2 = .017). Therefore, the extent of deviation was influenced by the target speed, but not by the presentation setting.
Lane change
A baseline deviation from the reference lane for a straight stretch of road without changing lanes was calculated for both target speeds of task 2, respectively. Two ANOVAs with repeated measures had been calculated to show a significant difference in deviation between baseline and lane change. For the medium-speed maneuver, we found a significant main effect of deviation (F (1,75) = 13.99, p < .001, ηp2 = 0.161), for the low-speed maneuver, this main effect of deviation was not significant, however descriptively visible (see Figure 7).
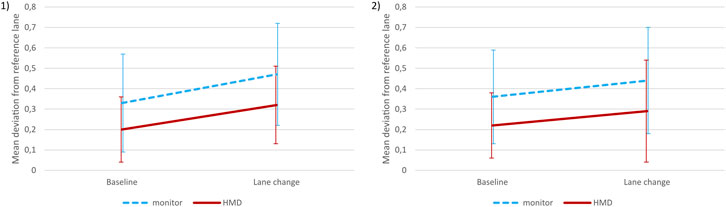
Figure 7. Mean deviation from reference lane for the medium-speed (1) and the low-speed (2) lane change (including standard deviations) Abbreviations: HMD = head-mounted display.
Both, the medium-speed (50 km/h) and the low-speed (30 km/h) maneuvers, were performed at a comparable speed in both settings. The medium-speed maneuver was performed at a mean speed of 52.5 km/h (SD = 3.3) in the monitor setting and at a mean speed of 51.7 km/h (SD = 8.8) in the HMD setting. There were no significant differences between the two settings regarding mean speed (p ≥ .631). For the low-speed maneuver, there was a mean speed of 33.5 km/h (SD = 4.0) in the monitor setting and a mean speed of 34.1 km/h (SD = 6.0) in the HMD setting.
A 2 × 2 mixed ANOVA with the between-subject factor setting and the within-subject factor speed (30 km/h, 50 km/h) and the dependent variable average deviation from the centerline revealed a significant main effect of setting (F (1,75) = 9.29, p = .003, ηp2 = 0.113), however no significant main effect of speed (F (1,75) = 1.09, p = .300, ηp2 = 0.015) or the interaction of setting and speed (F (1,75) = 0.00, p = .969, ηp2 < 0.001). Participants in the monitor condition deviated significantly stronger from the reference lane than people in the HMD condition.
Sign detection
We did not expect any differences between the five tries of sign detection. The mean sign detection distance over all tries was 765.45 m (±188.98) for the monitor setting and 181.69 m (±46.43) for the HMD setting. A t-test over all sign detection tries in order to compare both settings regarding the dependent variable distance to sign when pressing the button to classify the opening direction reached statistical significance (t (66) = 19.28, p < .001, d = 3.81).
Narrow curve maneuver
This maneuver was performed at a mean speed of 17.4 km/h (SD = 4.5) in the monitor setting and at a mean speed of 19.6 km/h (SD = 4.8) in the HMD setting. This difference in mean speed during the maneuver was significant (t (72) = −2.03, p = .046, d = −.48). Mean deviation from thereference lane was 0.36 m (SD = 0.28) in the monitor setting and 0.49 m (SD = 0.39) in the HMD setting. This difference was not significant (t (72) = −1.57, p = .061, d = −.39).
Exploratory analyses
Fear of driving
In this sample, 89.4% of the participants were below the IFD cut-off score of 3.5 (Fischer et al., 2021), indicating no relevant fear of driving. A small part of the sample, 10.6. %, had an IFD sum scores >3.5, with even one participant achieving the highest score of 15 and one participant achieving the second highest score of 14. Therefore, the sample included also participants with fear of driving. However, participants with relevant fear of driving did not significantly differ from participants with no fear of driving regarding the experience of presence, realism, or simulator sickness (p ≥. 117).
Participants’ feedback to the study
Participants were asked at the end of the study whether they enjoyed taking part of the study: 84.1% in the monitor setting and 64.1% in the HMD setting responded with a definite yes, 14.0% in the monitor setting and 31.0% in the HMD setting said rather yes and 4.7% in the monitor setting and 2.4% in the HMD setting responded with rather no. No one of the participants answered with a definite no which means that this study was highly accepted by the participants. Participants also had the opportunity to give feedback and suggestions for improvement in open questions. Examples were: Autonomous driving of the passive condition could be even smoother, more details in the scenery would make it even more realistic (e.g., guard rails), indicators were missing, mirrors did not work, and the acuity of the HMD could be better.
Discussion
There is still a lack of clarity in the literature how the application of monitors differs from the application of HMDs in motion platform-based driving simulators regarding the experience of presence, realism and simulator sickness. The present findings show that there is no significant difference between HMD and monitors regarding these three aspects. Taking a look at the interactivity within a driving simulation, meaning being the driver (active) or just the co-driver (passive), there were also no differences between the two settings regarding the three outcomes. Disregarding the setting, we found only for presence significantly higher values in the active condition meaning that being the driver of a car increases the feeling of being in the virtual environment than lower engagement as a co-driver. For the nausea subscale of the SSQ, there were significantly higher values in the passive condition which is not surprising. The driver is usually keeping his eyes constantly on the road ahead, which means he or she is less affected by nausea. We further focused on the active condition to replicate the findings of Blissing et al. (2022). Within the active condition, there was no significant difference in speed perception between the two settings. Regarding the lane change, participants in the monitor condition deviated significantly stronger from the reference lane than people in the HMD condition, regardless of the target speed. The findings of the sign detection tasks are totally in line with our hypothesis. Participants in the monitor setting could detect the signs much further away than those from the HMD setting. The performance of the narrow curve maneuver did not significantly differ between the two settings.
Overall, our data suggest that monitors and HMDs work the same way with respect to presence, realism and simulator sickness which is consistent with other studies (Aykent et al., 2015; Blissing et al., 2022; Himmels et al., 2023), although there are also some studies reporting a higher simulator sickness (Aykent et al., 2015; Cao et al., 2020; Malone and Brünken, 2021; Suwarno et al., 2019) or higher values of presence or realism (Kim and Park, 2020; Malone and Brünken, 2021) using an HMD than monitors or similar. While the monitor setting performed worse during the lane change maneuver, the HMD setting was clearly at a disadvantage during the sign detection task. This substantial difference between the two setting might be due to the low angular resolution in the current generation HMD. However, there could be also other reasons, e.g., a reduced visual scanning to prevent simulator sickness. Contrary to our expectations regarding the narrow curve maneuver, the descriptive deviation from the reference lane was even higher in the HMD setting compared to monitors which is consistent with the results of Blissing et al. (2022).
Strengths and limitations
First of all, as described in the methods section, the randomization could not be adhered to due to technical problems with the HMD setting. This could have led to sequence effects since the experimenters got more routine in implementing the study. We assume that this fact did not significantly influence the results since there were no systematic differences between the two setting (regarding age, gender, fear of driving, etc.). In addition, the routine that the examiners gained when implementing the monitor condition could not be fully transferred to the HMD setting since the application of the HMD required further knowledge (e.g., alignment of the HMD). Further, the used car models differed between the two settings. In the monitor setting, as described above, a different car with a different dashboard was used than in the HMD setting. It is assumed that these differences had no effect on the performance of the participants, as it was not expected that the vehicle models differ in their functionality. Future research should endeavor to use identical vehicle models. Additionally, the car had no indicators and no working side mirrors or rear-view mirrors in both settings what might have influenced the experience of presence and realism. The steering wheel was not height-adjustable and the driver’s seat could not be raised very high which might have influenced the performance of smaller participants. We furthermore had some technical problems with the HMD that led to many exclusions in this condition. Moreover, we did not track the hands of the participants, thus, they could not see their own hands while driving the simulator in the HMD setting. This lack of self-embodiment might have influenced the ratings of presence and realism. Another suggestion for improvement is the size of sign used for the sign detection task. Future studies may use smaller signs (e.g., 0.84 m × 0.84 m like a normal zone 30 sign) to make the scenario even more realistic. Another limitation is the fact, that three participants (two from the HMD and one from the monitor setting) aborted the study due to severe simulator sickness what might have led to an underestimation of simulator sickness. However, the sample size with n = 75 was still big enough assuming that these exclusions do not have a substantial impact on the results. Finally, we did not use a validated scale to measure the construct of realism what might have influenced our results. The three realism items used in this study were generated in accordance with those used by Blissing et al. (2022). However, this scale has not been validated so far. There are only few more or less validated scales available, such as one sub-scale of the IPS (Schubert et al., 2001) or the German Simulation Realism Scale (Poeschl and Doering, 2013). In our study, a moderate correlation of ρ = .495 was found between realism and the MPS, indicating that realism is something similar to presence, but different. The accurate measurement of realism should be therefore promoted.
Future studies should differ between clinically conspicuous and inconspicuous participants. In this study, there were few participants with clinically relevant fear of driving. Those might be emotionally more involved and experience a higher level of arousal what might influence the experience of presence (Diemer et al., 2015) and simulator sickness (Cao et al., 2020). Moreover, this study consisted of specific driving tasks. It would be of interest how presence, realism and simulator sickness are rated in a more naturalistic driving scenario.
Conclusion
There is no difference regarding presence, realism or simulator sickness regarding the two settings, monitors and HMD, indicating that use of monitors is sufficient for diving simulations. It is even better regarding visual acuity aspects as demonstrated by the sign detection task. The choice of interactivity, in contrast, appears to have a stronger effect on the experience of presence than the setting. Future studies should investigate whether this finding can be transferred to other simulators and virtual reality situations.
Data availability statement
The raw data supporting the conclusions of this article will be made available by the authors, without undue reservation.
Ethics statement
The studies involving humans were approved by Ethics Board of the University of Regensburg (file number: 22-3200-101). The studies were conducted in accordance with the local legislation and institutional requirements. The participants provided their written informed consent to participate in this study.
Author contributions
MG: Conceptualization, Data curation, Formal Analysis, Methodology, Project administration, Writing–original draft, Writing–review and editing. AM: Conceptualization, Methodology, Project administration, Supervision, Writing–original draft, Writing–review and editing.
Funding
The author(s) declare that financial support was received for the research, authorship, and/or publication of this article. The purchase of the driving simulation system was supported by the DFG by the grant INST 89/519-1 FUGG (Forschungsgroßgeräte).
Acknowledgments
We thank Thilo Hohl for his help in programming the driving scenarios. Furthermore, we are grateful to Finja Kossira and Katrin Schulze for their help in data collection.
Conflict of interest
The authors declare that the research was conducted in the absence of any commercial or financial relationships that could be construed as a potential conflict of interest.
Publisher’s note
All claims expressed in this article are solely those of the authors and do not necessarily represent those of their affiliated organizations, or those of the publisher, the editors and the reviewers. Any product that may be evaluated in this article, or claim that may be made by its manufacturer, is not guaranteed or endorsed by the publisher.
Supplementary material
The Supplementary Material for this article can be found online at: https://www.frontiersin.org/articles/10.3389/frvir.2024.1484739/full#supplementary-material
References
Aykent, B., Mérienne, F., and Kemeny, A. (2015). “Effect of VR device – HMD and screen display – on the sickness for driving simulation,” in Driving simulation conference, allemagne.
Benz, T. M., Riedl, B., and Chuang, L. L. (2019). “Projection displays induce less simulator sickness than head-mounted displays in a real vehicle driving simulator,” in Acm. doi:10.1145/3342197.3344515
Blissing, B., and Bruzelius, F. (2018). “Exploring the suitability of virtual reality for driving simulation,” in Driving Simulation Conference 2018, 163–166.
Blissing, B., Bruzelius, F., and Eriksson, O. (2022). The effects on driving behavior when using a head-mounted display in a dynamic driving simulator. ACM Trans. Appl. Percept. 19 (1), 1–18. doi:10.1145/3483793
Bradley, M. M., and Lang, P. J. (1994). Measuring emotion: the self-assessment Manikin and the semantic differential. J. Behav. Ther. Exp. Psychiatry 25 (1), 49–59. doi:10.1016/0005-7916(94)90063-9
Breyer, B., and Bluemke, M. (2016). Deutsche Version der Positive and Negative Affect Schedule PANAS. Mannheim: GESIS Panel. doi:10.6102/ZIS242
Brooks, F. P., Whitton, M., and Jerald, J. (2012). Scene-motion thresholds during head yaw for immersive virtual environments. Vorab-Onlinepublikation 9, 1–23. doi:10.1145/2134203.2134207
Cao, S., Nandakumar, K., Babu, R., and Thompson, B. (2020). Game play in virtual reality driving simulation involving head-mounted display and comparison to desktop display. Virtual Real. 24 (3), 503–513. doi:10.1007/s10055-019-00412-x
Cressman, E. K., and Henriques, D. Y. P. (2010). Reach adaptation and proprioceptive recalibration following exposure to misaligned sensory input. J. neurophysiology 103 (4), 1888–1895. doi:10.1152/jn.01002.2009
Deligiannidis, L., McConnell, D. S., and Vallee, C. (2009). “Visual and proprioceptive integration of the virtual and real fingertips,” in Human System Interactions, 2009. HSI '09. 2nd Conference on (S. 190–195) (IEEE/Institute of Electrical and Electronics Engineers Incorporated). doi:10.1109/HSI.2009.5090977
Diemer, J., Alpers, G. W., Peperkorn, H. M., Shiban, Y., and Mühlberger, A. (2015). The impact of perception and presence on emotional reactions: a review of research in virtual reality. Front. Psychol. 6, 26. doi:10.3389/fpsyg.2015.00026
Fischer, C., Schröder, A., Taylor, J. E., and Heider, J. (2021). Measuring driving fear. Eur. J. Psychol. Assess. 39, 49–60. Artikel 1015-5759/a000683. Vorab-Onlinepublikation. doi:10.1027/1015-5759/a000683
Heider, J., Fischer, C., and Schröder, A. (2018). Die deutsche Version des “Driving Cognitions Questionnaire” (DCQ). Z. für Klin. Psychol. Psychother. 47 (1), 36–47. doi:10.1026/1616-3443/a000459
Himmels, C., Andreev, V., Syed, A. A., Lindner, J., Denk, F., and Riener, A. (2023). “Are head-mounted displays really not suitable for driving simulation? A comparison with a screen-based simulator,” in 2023 IEEE intelligent vehicles symposium (IV) (IEEE). doi:10.1109/iv55152.2023.10186592
Kennedy, R. S., Lane, N. E., Berbaum, K. S., and Lilienthal, M. G. (1993). Simulator sickness questionnaire: an enhanced method for quantifying simulator sickness. Int. J. Aviat. Psychol. 3 (3), 203–220. doi:10.1207/s15327108ijap0303_3
Kim, J., and Park, T. (2020). Investigation of factors influencing simulator sickness and the sense of presence in flight simulator. ICIC Express Lett. 11 (5), 463–470. Part B: Applications. doi:10.24507/icicelb.11.05.463
Malone, S., and Brünken, R. (2021). Hazard perception, presence, and simulation sickness-A comparison of desktop and head-mounted display for driving simulation. Front. Psychol. 12, 647723. doi:10.3389/fpsyg.2021.647723
Poeschl, S., and Doering, N. (2013). The German VR simulation realism scale – psychometric construction for virtual reality applications with virtual humans. Annu. Rev. Cybertherapy Telemedicine 11, 33–37. doi:10.3233/978-1-61499-282-0-33
Renner, R. S., Velichkovsky, B. M., and Helmert, J. R. (2013). The perception of egocentric distances in virtual environments - a review. ACM Comput. Surv. 46 (2), 1–40. doi:10.1145/2543581.2543590
Rolnick, A., and Lubow, R. E. (1991). Why is the driver rarely motion sick? The role of controllability in motion sickness. Ergonomics 34 (7), 867–879. doi:10.1080/00140139108964831
Ryan, W. S., Cornick, J., Blascovich, J., and Bailenson, J. N. (2019). Virtual reality: whence, How and what for (virtual reality for psychological and neurocognitive interventions). New York, S: Springer, 15–46.
Schubert, T., Friedmann, F., and Regenbrecht, H. (2001). The experience of presence: factor analytic insights. Presence Teleoperators Virtual Environ. 10 (3), 266–281. doi:10.1162/105474601300343603
Slater, M. (2003). A note on presence terminology. Available at: https://api.semanticscholar.org/CorpusID:60395352.
Suwarno, D. C. D., Wijayanto, T., and Trapsilawati, F. (2019). “Cybersickness evaluation while using driving simulator in a head-mounted display environment,” in International Conference on Mechatronics, Robotics and Systems Engineering (MoRSE), 103–106. doi:10.1109/morse48060.2019.8998692
Vasey, M. W., and Thayer, J. F. (1987). The continuing problem of false positives in repeated measures ANOVA in psychophysiology: a multivariate solution. Psychophysiology 24 (4), 479–486. doi:10.1111/j.1469-8986.1987.tb00324.x
Volkmann, T., Wessel, D., Jochems, N., and Franke, T. (2018). Ger. Transl. Multimodal Presence Scale. doi:10.18420/muc2018-mci-0428
Weber, S., Weibel, D., and Mast, F. W. (2021). How to get there when you are there already? Defining presence in virtual reality and the importance of perceived realism. Front. Psychol. 12, 628298. doi:10.3389/fpsyg.2021.628298
Witmer, B. G., and Singer, M. F. (1998). Measuring presence in virtual environments: a presence questionnaire. Presence 7 (3), 225–240. doi:10.1162/105474698565686
Zöller, C., Müller, A., Eggert, L., Winner, H., and Abendroth, B. (2019). “Applicability of head-mounted displays in driving simulation,” in Proceedings of the Driving Simulation Conference 2019 Europe VR. Symposium im Rahmen der Tagung von Driving Simulation Association. Editors A. Kemeny, F. Colombet, F. Merienne, and S. Espie Strasbourg: GmbH.
Keywords: driving simulation, monitors, HMD, presence, realism, simulator sickness
Citation: Gabes M and Mühlberger A (2024) Driving simulation: the effects of interactivity and presentation setting. Front. Virtual Real. 5:1484739. doi: 10.3389/frvir.2024.1484739
Received: 22 August 2024; Accepted: 12 November 2024;
Published: 28 November 2024.
Edited by:
Maria Limniou, University of Liverpool, United KingdomReviewed by:
Daniel S. McConnell, University of Central Florida, United StatesOtto Lappi, University of Helsinki, Finland
Copyright © 2024 Gabes and Mühlberger. This is an open-access article distributed under the terms of the Creative Commons Attribution License (CC BY). The use, distribution or reproduction in other forums is permitted, provided the original author(s) and the copyright owner(s) are credited and that the original publication in this journal is cited, in accordance with accepted academic practice. No use, distribution or reproduction is permitted which does not comply with these terms.
*Correspondence: Michaela Gabes, bWljaGFlbGEuZ2FiZXNAdXIuZGU=