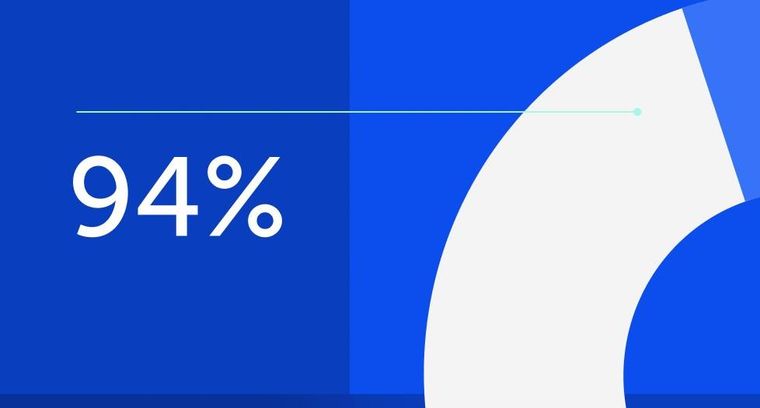
94% of researchers rate our articles as excellent or good
Learn more about the work of our research integrity team to safeguard the quality of each article we publish.
Find out more
ORIGINAL RESEARCH article
Front. Virtual Real., 04 September 2024
Sec. Virtual Reality in Medicine
Volume 5 - 2024 | https://doi.org/10.3389/frvir.2024.1452486
Introduction: Researchers frequently speculate that Immersive Virtual Reality (VR) diminishes pain by reducing how much attention is available to process nociceptive signals, but attention has rarely been measured in VR analgesia studies.
Methods: The current study measured how much attention VR uses. Using a repeated measures crossover design, 72 college students (mean = 19 year old) gave pain ratings (0–10 GRS scale) during brief painful but safe and tolerable heat stimulations during No VR, vs. immersive VR vs. semi-immersive VR (treatment order randomized).
Results: Compared to semi-immersive VR, during immersive VR, participants reported a significant 25% drop in pain intensity, and a significant 23% increase in fun during the pain stimulus, (p < .001 for each measure).
Discussion: As predicted by an attention mechanism for how VR reduces pain (the distraction hypothesis), participants made significantly more mistakes on an attention-demanding odd-number divided-attention task during the immersive VR condition than during the less immersive VR condition. Secondary analyses also showed that immersive VR was still effective at higher pain intensity levels, and was widely effective regardless of gender, race, or participant’s tendency to catastrophize.
Excessive acute pain during medical procedures can have significant long term medical and psychological consequences. Repeated exposure to uncontrollable pain can create aversion to medical care, anxiety disorders, can pathologically alter the patient’s processing of pain (chronic pain) and/or lead to PostTraumatic Stress Disorder (Rosenberg et al., 2018; Rosenberg et al., 2015). Opioid analgesics have long been the cornerstone of acute pain management, and often help control pain during medical procedures, but opioid side effects restrict dose levels. Due to the current crisis of opioid-related overdose deaths (Baumann et al., 2023; CDC, 2013) and new Federal government restrictions on prescribing opioids, finding effective new opioid sparing pain management innovations has become an urgent national priority (Keefe et al., 2018; Keefe et al., 2012). Although VR is also increasingly being used to treat chronic pain (Keefe et al., 2012) via a number of mechanisms (Gupta et al., 2018), the current study explores the mechanism of simple VR distraction for acute pain.
Traditional (non-VR) distraction (e.g., listening to music) is a non-pharmacologic technique widely used in clinical practice (e.g., in dentist offices) to help reduce acute procedural pain, but with mixed results (Rohilla et al., 2018; van der Heijden et al., 2018). According to (McCaul and Malott, 1984) traditional distraction works via an attentional mechanism. “Pain interrupts and demands attention” (Eccleston and Crombez, 1999) p 356. And people can only attend to a limited amount of information from the outside world (Kahneman, 1973; Anderson et al., 1996). The goal of pain distraction is to focus the participant’s attention on something other than their pain.
(McCaul and Malott, 1984) suggested that traditional distraction (e.g., listening to music or watching cartoons or movies) would be most effective for treating mild pain, but they predicted that at higher pain intensity levels, distraction would be much less effective, (e.g., would not reduce pain) because pain would be given priority access to limited attentional resources. Their predictions were made before Virtual Reality (VR) became available. VR is proving effective at reducing pain during medical procedures, and is often used as an opioid sparing adjunct (Trost et al., 2021; Smith et al., 2022; Gold and Mahrer, 2018; Gold et al., 2021; Caruso et al., 2020; Eccleston et al., 2022; Colloca et al., 2020; Hemphill et al., 2021; Hemphill et al., 2022; Hitching et al., 2023; Peterson et al., 2021; Jeffs et al., 2023; Kanad et al., 2023; Lind et al., 2023; Matamala-Gomez et al., 2019; Jeffs et al., 2014; McSherry et al., 2018). Immersive VR is a powerful multisensory distraction that may be able to compete with pain for an individual’s limited attentional resources, even at higher pain intensity levels (Maani et al., 2011b; Maani et al., 2011a).
According to fMRI brain scans using custom-made magnet-friendly fiberoptic VR goggles, participants report large reductions in perceived pain intensity during VR, and in one study participants’ brains showed a 50% drop in pain-related brain activity during VR, in each of the five brain regions of interest (identified apriori), the primary and secondary somatosensory cortex, the anterior cingulate, the insula, and the thalamus (Hoffman et al., 2004b). In a second fMRI study, VR reduced pain as effectively as a moderate dose of opioids (Hoffman et al., 2007).
In the current laboratory study, we predict that immersive Virtual Reality systems custom tailored for pain control will be able to significantly reduce pain intensity levels higher than 5 on a zero to 10 scale. Humans are visually dominant. “Human visual cortex includes the entire occipital lobe and extends significantly into the temporal and parietal lobes … spanning about 20% of cortex” (Wandell et al., 2007) p 366, and scientists estimate that 50% of the human cortex (including visual cortex and other cortical areas) is involved in processing visual information coming into the brain via the eyes (Hagen, 2012). We thus predict that flooding the brain with multisensory information (unusually strong visual illusions, with converging evidence from motor activity from interacting with the virtual objects, and proprioceptive information from head movements as the participant looks around the virtual world) will enable VR to reduce higher intensity pain (e.g., above five on a zero to ten pain scale during no distraction). Whether immersive VR can reduce such higher intensity pain (moderate pain and higher) is tested in the current laboratory study.
The following definitions may help understand the distinction between immersion and presence, as these terms are used in the present paper. According to Slater and Wilbur (1997), p 4, “Immersion can be an objective and quantifiable description of what any particular system does provide. Presence is a state of consciousness, the (psychological) sense of being in the virtual environment.” Put another way, Gupta et al., 2018 (p 152) define immersion as “an objective term that describes the amount of sensory input the VR system creates.” According to Slater et al. (Slater, 2018) the immersiveness of the VR system is measurable, and involves such factors as the field of view (the angular extent of the observable virtual world seen in the VR goggles), head tracking, interactivity, resolution (the number of pixels in the VR goggles) and other objectively measurable factors. In general, more immersive VR systems often increase the participant’s illusion of “being there” in the computer-generated world (Hoffman et al., 2004c). Put another way, “an increase in immersion often leads to an increase in presence felt by the user” (Gupta et al., 2018; p. 152). Improving the immersiveness of a given VR system via the hardware and software of the VR system can lead to increased presence, which is the psychological experience of the user (Slater and Wilbur, 1997). Wide field of view VR (Hoffman et al., 2006), interactivity (Wender et al., 2009), and tactile feedback (Hoffman et al., 2023) have each been shown to significantly increase VR analgesia. Several studies from a number of laboratories have found that increasing the immersiveness of a VR system increases analgesic effectiveness (Jeffs et al., 2014; Law et al., 2011).
Researchers have long speculated (Hoffman et al., 2000; Hoffman, 1998) that immersive VR reduces acute pain by diverting attention away from the brain’s processing of nociceptive signals, the distraction hypothesis. But unlike the current study, previous VR analgesia studies have almost never measured how much attention VR uses up. The current study will test our hypothesis that VR reduces pain by capturing/diverting attentional resources that would otherwise be used to process nociceptive signals. We predict that the stronger the distraction, the more effectively pain is reduced.
In the current study, we predict that using the more immersive, interactive, wider field of view, head tracked VR system (hitherto referred to as “immersive VR” for short) will increase the participants’ illusion of feeling present in a specific VR environment called “SnowCanyon,” and will make VR capture more attention, as measured by an increase in errors on a divided attention task. We predict that immersive VR will thus be more effective than semi-immersive VR (narrow field of view, no head tracking and no interactivity) at reducing how much pain participants report during brief thermal stimuli.
The measurement of how much attention is drawn into VR is important for understanding the mechanisms of VR analgesia for acute pain, as well as its optimization. However, measures of attention have been missing from nearly all VR analgesia studies to date. To test the distraction hypothesis for VR analgesia (Hoffman, 2021), used a divided attention task to quantify the amount of attention used up by VR. Immersive VR was significantly more effective at reducing pain than the semi-immersive VR condition, and immersive VR measurably diverted more attentional resources than the semi-immersive VR, see also (Hoffman et al., 2003). The current study further explores whether immersive VR is more attention demanding (and is a more powerful analgesic) than a less immersive VR condition, as predicted by the distraction hypothesis. Replicability is a hallmark of good science, … “replicability involves new data collection to test for consistency with previous results of a similar study” (National Academies of Sciences, 2009) p. 7. Using different VR hardware and different VR worlds, but a similar study design and measures, the current study attempts to conceptually replicate the findings of (Hoffman, 2021), to further test the distraction hypothesis explanation for the mechanism of VR analgesia.
As mentioned above, some researchers have speculated that distraction would be less effective for higher intensity pain stimuli (McCaul and Malott, 1984). The current study will analyze the subset of participants who report pain intensity greater than five, to measure if VR can reduce higher pain intensities (when VR is needed most). Similarly, catastrophizing can exert a powerful influence on how much pain and emotional distress is experienced by the individual (Fabian et al., 2011), and there is recent evidence that distraction is less effective for high catastrophizers (Asefi Rad and Wippert, 2024). The current study will analyze the subset of participants prone to catastrophizing, to see if VR can reduce the pain of high (clinical level) catastrophizers. Further, preliminary evidence has shown that “meaningful differences among various pain relevant outcomes (e.g., pain ratings, endogenous pain inhibitory processes) can be attributed to individuals' ethnic backgrounds” (Fabian et al., 2011) p, 314. And females are typically more sensitive than males in their perception of noxious heat stimuli (Feine et al., 1991; Bartley and Fillingim, 2013) and other painful stimuli (Bartley and Fillingim, 2013). Whether VR reduces pain of ethnic minorities (Asians) and females, is analyzed in the current study.
In sum, in addition to measuring how much attention is used by VR, the current study also measures whether immersive VR is still effective when used to treat higher pain intensity levels (the subset of patients with pain higher than 5 on a zero to ten scale), and whether VR analgesia is widely effective regardless of gender, race, or participants’ tendency to catastrophize.
“Collectively, greater understanding of the factors that commonly and differentially affect the disparity in pain perception, as well as analgesic response, are beginning to illuminate research targets and promising areas of therapeutic intervention for improved pain management” (Paller et al., 2009) page 289.
This randomized cross-over study was conducted at the University of Washington, Seattle WA, United States, in agreement with the Declaration of the World Medical Association. Each participant was fully informed about the risks, benefits, and alternatives of participating and gave written informed consent in accordance with the Declaration of Helsinki in its latest version, http://www.wma.net/ and in compliance with the University of Washington Human Research Review Committee, Study number 0007801.
Seventy-two undergraduates from the University of Washington (UW) Department of Psychology (72 students aged 18–39, mean age = 19.06 years old, SD = 2.52) participated. There were no drop-outs and no pre-screening questionnaires were used. Participants received extra credit in their Psychology class for participating in this hands-on educational experience. The method used was based in part on the methodology used by (Hoffman, 2021), as shown in quotation marks below, using the collective commons copyright. The inclusion and exclusion criteria are the same as (Hoffman, 2021), shown verbatim in italics below. Note that all italics in this manuscript are verbatim from (Hoffman, 2021) and used with permission via the creative commons copyright agreement.
“Currently enrolled in a course at the University of Washington Psychology Department, participating in the UW Psychology research pool
Able to read, write and comprehend English
Able to complete study measures
Willing to follow our UW approved instructions
18 years of age or older
Exclusion Criteria: listed in the recruitment information and confirmed during a brief in person eligibility screening interview
Extreme susceptibility to motion sickness
Seizure history
Unusual sensitivity or lack of sensitivity to pain
Sensitive skin
Sensitive feet
Migraines, Diabetes”
According to the 2020 IASP definition (Raja et al., 2020), p. 1976, pain is “An unpleasant sensory and emotional experience associated with, or resembling that associated with, actual or potential tissue damage.” The current study measures the effect of VR on both the sensory (pain intensity) and emotional (unpleasantness) components of pain. Participants’ subjective rating of worst pain intensity during the thermal stimulus was the primary study outcome. Pain unpleasantness and Time Spent Thinking About Pain, the cognitive component of pain (Hoffman et al., 2000), served as secondary outcomes. Graphic Rating Scales (GRS) (Jensen, 2003) were used to assess each of these outcome domains, as shown in Supplementary Appendix SE. “Fun during the most recent stimulus” introduced by (Hoffman et al., 2004a) was also included as a surrogate measure of positive affect. Finally, the Pain Catastrophizing Scale measures a person’s tendency to imagine the worst possible outcome of a pain-related action or event (Sullivan et al., 1995).
In the current study, using a within-subject repeated measure design, we powerfully manipulated the immersiveness of the VR system by simultaneously changing three immersion factors; the field of view, head tracking, and interactivity of SnowCanyon. The Head Mounted Display, HTC VIVE Pro VR helmet, with 1,440 × 1,600 pixels per eye (2,880 × 1,600 pixels combined), refresh rate = 90 hz, field of view = 110°. SnowCanyon is a research VR world built for our research team by BigEnvironments.com.
During both the immersive and semi-immersive VR treatment conditions, each participant floated along a pre-defined spline path in SnowCanyon (BigEnvironments.com). The participants did not have any control over the navigation, which was all pre-programmed. Participants saw themselves moving slowly through a 3-D computer generated snowy canyon (see Figure 1, left). In the semi-immersive VR condition, instead of seeing SnowCanyon all around them, the “Virtual Desktop” app (https://www.vrdesktop.net/) was used to reduce the field of view of SnowCanyon (see Figure 1). Looking into the HTC VIVE Pro VR goggles, the participant only saw SnowCanyon on a 60 inch 2D Virtual Desktop screen appearing to float in a stationary location, approximately 6 feet in front of them with an outer space (dark nebulous) background in the semi-immersive VR condition (immobilized head, no head tracking, and a narrower, approximately 45° field of view of SnowCanyon, hitherto referred to as semi-immersive VR for short). And, during semi-immersive VR, participants could not interact with virtual objects they saw in the virtual world (no Snowballs). In the immersive VR condition (Figure 1, right), participants received a much wider 110° field of view SnowCanyon with head tracking via an HTC VIVE Pro VR helmet, using the HTC VIVE base stations to track participants 360° head movements in the immersive VR condition. For example, if participants pointed their head orientation toward the floor in the laboratory room, they saw the computer generated river in the virtual world in the goggles, etc. As they moved slowly through the immersive snow laden 3D canyon, they interacted with objects in VR (e.g., Snowmen) by using head movements/gaze direction to aim, and they pushed a button to direct Snowballs at Snowmen and virtual penguins.
Figure 1. SnowCanyon. As shown on the left, during semi-immersive VR, participants moved slowly through SnowCanyon via a less immersive display (45 degree Field of View) with no head tracking and no interactivity. During Immersive VR (shown on the right side above), participants saw a much wider field of view display (110 degree Field of View), with 360° head tracking and interactivity (throwing snowballs at virtual snowmen). Image by Bigenvironments.com, copyright Hunter Hoffman, U.W., vrpain.com. Used with permission.
As shown in Figure 1, in SnowCanyon participants float slowly through a virtual glacial canyon, with a river below, flowing downhill, cloudy sky, and ledges on the sides of the canyon containing animated cartoonish snowmen, and virtual penguins.
Quantitative Sensory Testing (medoc_Ltd, 2024) was used. Thermal heat stimuli were delivered via a new Q-Sense CPM thermal stimulator from Medoc LTD, Israel. As described by (Hoffman et al., 2004b; Hoffman et al., 2007), using the Medoc Advanced Medical Systems Q-sense Ramp and Hold program, https://www. medoc-web.com/q-sense each participant received brief 10 s stimuli to the top of their left foot, and selected a temperature they found “painful but tolerable” that they were willing to receive two more times, later in the study during the VR analgesia test. The mean stimulus temperature selected by participants in the current study was 45.72°C (SD = 1.10, range = 44°C–48.0°C).
After entering the research room, participants sat down and completed informed consent. They were then shown the Quantitative Sensory Testing thermode, (the white cube in Figure 2), which was briefly explained to them, and they were asked to take off their left shoe and sock. The participant helped the researcher attach the thermode to the top surface of their foot, using a Velcro strap (shown in Figure 2).
Figure 2. Thermal stimulator attached to foot. Photo and copyright by Hunter Hoffman, http://www.vrpain.com.
Participants rated their pain during the “no VR” (baseline) thermal heat stimulus. After a brief approximately 5 min inter-stimulus washout period between conditions, they also rated their pain during the semi-immersive VR and during Immersive VR.
Each participant received all three conditions (No VR, semi-immersive VR and immersive VR). Using an AB/BA design and block randomization (blocks n = 2), some students received immersive VR first and semi-immersive VR second, and others received semi-immersive VR first and immersive VR second, (see Figure 3). Having each person act as their own control avoids variance from individual differences in sensitivity to pain, thus increasing statistical power.
As illustrated in Figure 4, each participant selected a brief and safe thermal stimulus they found “painful but tolerable” (Hoffman, 2021), p 4, (baseline pain during no VR), that they were willing to receive two more times, shortly thereafter. During the VR analgesia test, each participant experienced an immersive VR treatment during one brief pain stimulus, and they experienced a semi-immersive VR condition during another brief stimulus (VR treatment order randomized). The instructions, taken verbatim from (Hoffman, 2021), p. 4, were are shown in Supplementary Appendix SA.
Figure 4. Each participant was randomly assigned to either Treatment Order 1 or Treatment Order 2. Treatment Order 1 received semi-immersive VR before immersive VR. Treatment Order 2 received immersive VR before semi-immersive VR. The fire symbol indicates brief painful Quantitative Sensory Testing stimuli at safe and tolerable temperatures individualized to each participant.
As previously used verbatim by (Hoffman, 2021) page 4, the instructions for the pain ratings are shown in Supplementary Appendix SB.
“After each brief thermal stimulus, participants indicated how painful they found the stimulus using Graphic Rating Scales (GRS), validated by the measures’ strong associations with other measures of pain intensity, as well as through the measure’s ability to detect treatment effects (Jensen, 2003; Williamson and Hoggart, 2005). GRS ratings were used to measure worst pain, pain unpleasantness, and time spent thinking about pain that correspond to three separable components of the pain experience; sensory pain, affective pain, and cognitive pain, respectively. The question regarding ‘to what extent did you feel like you ‘went into’ the virtual world,’ was adapted from (Slater et al., 1994; Slater and Wilbur, 1997). Similar presence measures have been shown to be reliable and able to detect treatment effects” (Hoffman, 2021) p. 4.
For the semi-immersive VR condition, participants received the following instructions, also based on (Hoffman, 2021), p 4 (verbatim is shown in italics), as shown in Supplementary Appendix SC “Note that all text shown in italics in this manuscript are verbatim from Hoffman (2021), with permission, via the collective commons copyrights.
For the immersive VR condition, participants were given the following instructions. “For this next part of the study, please put on the VR helmet, you will see SnowCanyon. You can move your head to look around and throw snowballs at anything you want in SnowCanyon. Use your gaze direction to aim and click the mouse button to throw snowballs. While you are in VR doing this, you will receive another 10 s pain stimulus at one of the temperatures you have approved. In other words, we will not go any higher than that last stimulus you approved.” After receiving a brief thermal stimulus while in the Immersive VR condition, they took off the VR helmet and answered the pain ratings, using the pen and paper GRS pain and presence ratings. See Supplementary Appendix SE Text in italics above are verbatim from (Hoffman, 2021), p. 4.
The odd number task is a divided attention task previously used to study human memory (Craik, 1983; Iidaka et al., 2000; Jacoby et al., 1989). The traditional odd number task was recently repurposed/modified by our team to measure how much attention is drawn into a given VR analgesia system (Hoffman et al., 2023; Hoffman, 2021), see also (Hoffman et al., 2003).
In the current study, participants performed this brief psychological test designed to quantify how effectively VR captures a person’s attentional resources by measuring the number of errors the participant commits during the auditory “odd number” task during No VR, during semi-immersive VR, and during Immersive VR (see Figure 5). In this divided attention task, the patient’s attention is divided between attending to auditory information coming into their brains from the real world via their ears (i.e., hearing a long string of numbers between one and ten), vs. attending to visual information coming into their brain from the computer-generated VR world, primarily via their eyes in the current study. The more of their attention captured by the computer-generated virtual world, the more errors they will likely make on the attention demanding odd number task in the real world.
Figure 5. participants listen to a list of numbers between one and ten, and said “now” any time they heard three odd numbers in a row.
As described by (Hoffman, 2021) p. 5, verbatim shown in italics in Supplementary Appendix SD.
“The researcher then played the pre-recorded auditory string of numbers (see below) via a digital audio file on a laptop with high quality Dolby Sound laptop speakers. The traditional odd number divided attention task (Craik, 1983; Iidaka et al., 2000; Jacoby et al., 1989) was adapted for use in the current VR study, using our own new number set (shown below) customized for the present study (with 10 odd number triads during a 2 min session)” (Hoffman, 2021, p. 5).
“The auditory string of numbers (i.e., the odd number task) lasted 2 min. The same identical two-minute audio file was played a total of three times. The first time, it was played with No VR (baseline). The second time the odd number audio task was played during semi-immersive VR and the third time, it was played in VR again (e.g., immersive VR). The VR treatment order used for the odd number divided attention task was the opposite of the VR treatment order used in thermal pain VR analgesia stimuli, and VR treatment order was thus also randomized” (Hoffman, 2021) page 6.
Note that participants did not receive any pain stimuli during the Odd Number Task. “To summarize, the Odd Number Task is a divided attention task that involves monitoring auditory numbers during No VR (for 2 min) vs. semi-immersive VR (for 2 min) vs. during immersive VR (for 2 minutes). During the “dual task,” participants must perform the two tasks at the same time, being in VR was one task, and monitoring the odd number task was a competing task. In each condition, participants listened to an auditory string of numbers from 1 to 10, and said “now” every time they heard three odd numbers in a row. They were told that the researcher would be monitoring their accuracy on the odd number task. During the odd number task, the researcher had a printout of the number sequence, and the researcher made a mark to indicate every time the participant said “now” in each of the following conditions” (Hoffman, 2021) p. 5.
Participants did not wear a VR helmet during the No VR condition. They performed the odd number task with No VR.
During the semi-immersive VR condition, each participant passively watched themselves float slowly through SnowCanyon displayed on an approximately 45° field of view 2D screen floating in an immersive 3D virtual universe background (displaying SnowCanyon on the smallest virtual screen size possible in the “Virtual Desktop” app). While they were floating slowly through SnowCanyon on the virtual screen they saw in the HTC Vive Pro VR helmet, they also listened to a pre-recorded series of numbers between one and ten from a laptop speaker with Dolby sound, and said “now” any time they heard three odd numbers in a row.
During the Immersive VR condition, each participant received SnowCanyon with head tracking (no Virtual Desktop screen in this condition, they could see a full 110° field of view of SnowCanyon, in the HTC VIVE Pro VR helmet). As they moved slowly through the snow-laden 3D canyon, they could interact with virtual objects in the virtual world (e.g., Snowmen) by aiming with gaze direction and pushing a button on their mouse to throw Snowballs. At the same time, they listened to a pre-recorded series of numbers between one and ten from a laptop speaker and said “now” any time they heard three odd numbers in a row.
The current study used the same power analysis as Hoffman, 2021, p. 6. The statistical program GPower 3.10 was used a priori to estimate the sample size to test our primary hypothesis. Based on earlier research (Wender et al., 2009), we assumed an effect size (d) of .78, power of .95, and an alpha of .05. According to the power analyses, at least 24 participants would be needed to be able to detect whether the Immersive VR treatment reduced worst pain during the thermal pain stimulus, compared to the less immersive semi-immersive VR. Statistical power is the probability that a hypothesis test will find a significant effect if there is an effect to be found. We used a power analysis to estimate the minimum sample size required for an experiment, given a desired significance level, effect size, and statistical power. Collecting a pre-specified sample larger than minimum is ideal. The current (n = 72 participants) study used more participants than the minimum sample identified by the power analysis (n = 24), in case the effect size was smaller than estimated, and because we anticipated that secondary analyses (e.g., accuracy on the odd number task, and subgroup analyses of females only, etc.), would require extra power.
The sample was comprised of 64% females and 36% males. The ethnicity of the females was 47% white, 51% Asian, and 2% black. The ethnicity of the males was 33% white, 63% Asian, and 4% black.
Because each participant received two VR treatment conditions (semi-immersive and immersive VR), it is possible that the first treatment could “carry over” or influence the response on the second treatment, if so, this would complicate interpretation. This is one known potential drawback that can sometimes happen when using a repeated measures design.
To test for carry-over effects, we conducted a pre-analysis to see if the difference between the semi-immersive VR and immersive VR was stronger depending on which treatment order the participants received (Immersive VR 1st or Immersive VR 2nd). The difference between worst pain during semi-immersive VR vs. worst pain during immersive wide field of view SnowCanyon was the dependent variable, and treatment order was the between groups factor (people who received immersive VR first were considered one group, and those who received immersive VR second were considered a second group, but only for this “carry over” pre-analysis).
Fortunately, no significant carry over (treatment order) effects were found for Worst pain, Mann-Whitney Z = 1.25, p = .21 NS, pain unpleasantness, Z = 1.65, p = .10 NS, Time spent thinking about pain during the thermal stimulus, Z = 0.36, p = .72 NS, or Fun during the thermal stimulus, Z = 1.47, p = .14 NS. And no significant carry over (treatment order) effects were found for accuracy on the odd number task, Z = .21, p = .84, NS. Since there were no significant carry over effects, all of the following analyses were collapsed across treatment order (see Tables 1–5).
Table 5. Means, standard deviations (SD) and test statistics for “accuracy on the odd number ‘divided attention’ task.”
“The normality assumption means that the collected data follows a normal distribution, which is essential for parametric assumption” (Kim and Park, 2019) p 332. To help choose the appropriate statistical method, we tested whether the primary outcome data was normally distributed (worst pain ratings during the 3 treatment conditions, during No VR, during semi-immersive VR, and during immersive VR). Kolmogorov–Smirnov showed that the distribution of worst pain ratings departed significantly from normality for worst pain during No VR, D(72) = 0.17, p < 0.001, the distribution departed significantly from normality for worst pain during semi-immersive VR, D(72) = 0.13, p < 0.01, and departed significantly from normality for worst pain during Immersive VR, D(72) = 0.14, p < 0.005. Based on these outcomes, non-parametric statistics were used for all analyses. An omnibus Friedman test was used when three conditions were compared, and the Wilcoxon signed rank test was used for paired comparisons.
A non-parametric Omnibus Friedman test (testing if the three conditions differed) showed a significant main effect for Worst pain, χ2 (2) = 61.46, p < 0.001. Post hoc paired comparisons (Wilcoxon signed rank tests) for the variable “worst pain” are shown in Table 1. These paired comparisons compared (No VR vs. semi-immersive VR), (No VR vs. immersive VR), and (semi-immersive VR vs. immersive VR), see Table 1.
An omnibus Friedman test (testing if the three conditions differed) showed a significant main effect for pain unpleasantness, χ2 (2) = 51.50, p < 0.001. Post hoc paired comparisons (Wilcoxon signed rank tests) for the variable “pain unpleasantness” are shown in Table 2. These paired comparisons compared (No VR vs. semi-immersive VR), (No VR vs. immersive VR), and (semi-immersive VR vs. immersive VR), see Table 2.
An omnibus Friedman test (testing if the three conditions differed) showed a significant main effect for Time spent thinking about pain, χ2 (2) = 56.07, p < 0.001. Post hoc paired comparisons (Wilcoxon signed rank tests) for the variable “Time Thinking About Pain” are shown in Table 3. These paired comparisons compared (No VR vs. semi-immersive VR), (No VR vs. immersive VR), and (semi-immersive VR vs. immersive VR), see Table 3.
An omnibus Friedman test (testing if the three conditions differed) showed a significant main effect for Fun, χ2 (2) = 87.50, p < 0.001. Post hoc paired comparisons (Wilcoxon signed rank tests) for the variable “Fun” are shown in Table 4. These paired comparisons compared (No VR vs. semi-immersive VR), (No VR vs. immersive VR), and (semi-immersive VR vs. immersive VR), see Table 4.
An omnibus Friedman Test showed a significant main effect of “No VR” versus “semi-immersive VR” versus “immersive VR” for accuracy (mean number of hits out of 10 possible hits) on the odd number task, χ2 (2) = 54.10, p < 0.001. Post hoc paired comparisons (Wilcoxon signed rank tests) for the variable accuracy on the odd number task are as follows. As shown in Table 5, participants were 92% accurate (mean = 9.21 correct out of 10 possible, SD = 1.54) on the odd number task during “No VR”, but made significantly more errors on the odd number task during semi-immersive VR, 89% accurate (mean = 8.88, SD = 1.39), Z = 2.46, p < 0.05. Semi-immersive VR used significantly more attention than No VR (see Table 5).
Please note that for the odd number monitoring task, lower accuracy on the odd number task means VR captured more attention (and would thus likely be a more effective pain distraction). An omnibus Friedman Test showed a significant main effect of “No VR” versus “semi-immersive VR” versus “immersive VR” for accuracy (mean number of hits out of 10 possible hits) on the odd number task, χ2 (2) = 54.10, p < 0.001. Accuracy results, (mean number of hits on the odd number task out of 10 possible hits during each 2 min odd number session, e.g., 7/10 hits = 7.00 = 70% accurate, with SD in parentheses) are shown in Table 5.
Similarly, participants were 92% accurate during No VR (mean = 9.21 correct, SD = 1.54), and compared to No VR, participants made significantly more errors during immersive VR, 76% accurate (mean = 7.63, SD = 1.81), Z = 5.30, p < 0.001. Immersive VR used significantly more attention than No VR.
Most importantly, there was also a significant difference in the accuracy on the odd number divided attention task comparing semi-immersive VR (M = 89% correct, mean = 8.88 (SD = 1.39) versus immersive VR 76% correct, mean = 7.63, (SD = 1.81), Z = 5.27, p < .001. Interactive VR used significantly more attention than semi-immersive VR.
Participants’ illusions of going into the computer-generated world were as follows. Compared to their illusion of presence (being there) during semi-immersive VR, Mean = 2.89 (SD = 2.19), participants reported having a significantly stronger illusion of presence in VR (“being there” in the virtual world), during “immersive VR”, Mean = 4.53 (SD = 2.18) (where higher presence ratings are better, on a zero to ten rating scale), Z = 5.00, p < 0.001.
Compared to “semi-immersive” VR, Mean = 2.04 (SD = 2.10), virtual objects in VR seemed significantly more real during “immersive VR,” Mean = 3.01 (SD = 2.22) (where higher ratings indicate higher realism), Z = 3.87, p < 0.001.
Nausea during VR was near zero for both treatment conditions. Compared to nausea during semi-immersive VR, Mean = 0.19 (SD = .62), participants reported no significant increase in nausea during immersive VR, (Mean = 0.19, SD = .51), Z < 1, p = 0.86 NS.
In secondary analyses, as detailed briefly below, we analyzed subsets of the sample to explore whether VR analgesia was significant when selecting the subset of participants who were Caucasians only, Asians only, males only, females only, and high catastrophizers only, and for the subset of participants reporting high pain intensity during No VR.
Compared to No VR, immersive VR significantly reduced worst pain for a subset analyses of Caucasians only, Z = 4.40, Asians only, Z = 4.18, males only, Z = 3.61, and females only, Z = 4.81, (p < .001 for each measure).
People with scores 30 and higher on the Pain Catastrophizing Scale, considered clinical levels of pain catastrophizing, still showed significant reductions in pain comparing No VR vs. immersive VR, Z = 2.54, p < .05.
Compared to No VR, semi-immersive VR significantly reduced mild to moderate pain intensity (5 or less on a zero to ten scale), Z = 3.61, p < .001, but semi-immersive VR was not statistically significant for reducing pain intensity levels higher than 5 on a zero to 10 scale, Z = 1.79, p > .05. In contrast, in the current study, compared to No VR, immersive VR significantly reduced mild to moderate pain (5 or less), Z = 5.20, p < .001, and immersive VR also significantly reduced pain intensity levels higher than 5 on a zero to 10 scale, Z = 3.50, p < .001.
Consistent with the distraction hypothesis, (Hoffman, 1998; Hoffman et al., 2000), in the current study, immersive VR was significantly more effective at reducing pain. In addition, immersive VR significantly increased participants’ illusion of presence, and the more immersive VR system was significantly more attention grabbing than the semi-immersive VR system. These results support our proposal that VR reduces pain by using up/diverting attentional resources that would otherwise be used to process nociceptive signals. This study directly addresses the question of how VR influences pain perception, see also (Hoffman, 2021; Hoffman et al., 2023). Although other mechanisms may also be involved in VR analgesia, the results of the current study support our hypothesis that VR reduces acute pain via an attentional mechanism.
Several previous studies that did not measure attention have now shown that active immersive VR is significantly more effective at reducing pain than semi-immersive VR (Gutierrez-Maldonado et al., 2011; Wender et al., 2009; Dahlquist et al., 2007; Xiang et al., 2021; Payne et al., 2022). Only two previous studies also measured how much attention VR uses during less immersive VR vs. during more immersive VR condition (Hoffman, 2021; Hoffman et al., 2023). However, one of those two studies (Hoffman et al., 2023), only manipulated one immersion factor. In that study, both VR conditions were nearly identical, and both the less immersive and the more immersive VR conditions included head tracking, avatar, and interactivity. In (Hoffman et al., 2023), the only difference was the less immersive VR system did not include tactile feedback, and the more immersive condition did include tactile feedback when participants touched a virtual object. In that study, adding tactile feedback significantly increased analgesic effectiveness but did not measurably divert more attentional resources than the less immersive VR condition on the odd number task (Hoffman HG et al., 2023).
In contrast, as a stronger manipulation of immersiveness, in both (Hoffman, 2021) and in the current study, researchers manipulated several immersive factors simultaneously (field of view, interactivity, and head tracking in the current study), and found significant reductions in pain ratings and also found that the more immersive VR condition diverted more attentional resources than the less immersive VR condition (significantly lower accuracy on the divided attention task during immersive VR than during semi-immersive VR). The current study conceptually replicates the findings of the avatar study of (Hoffman, 2021). The current study had no avatars. Instead, immersiveness was strongly manipulated via field of view, interactivity, and head tracking. As predicted by an attention mechanism for how VR reduces pain (the distraction hypothesis), participants in the current study made significantly more mistakes on an attention-demanding odd-number divided-attention task during the immersive VR condition than during the less immersive VR condition. Beyond (Hoffman, 2021), the present study also showed that immersive VR was still effective at higher pain intensity levels, and was widely effective regardless of gender, race, or participant’s tendency to catastrophize.
Before VR became available (McCaul and Malott, 1984), predicted that traditional distraction would be effective for reducing mild pain, but would be less effective as pain intensity increased. In the current study, immersive VR significantly reduced mild pain, and also significantly reduced pain intensity levels higher than 5 on a zero to 10 scale, see also (Hoffman et al., 2019; Hoffman et al., 2020; Maani et al., 2011b). Thus, VR distraction is so powerful that even high levels of pain can be effectively reduced by immersive VR. And in fact, preliminary results suggest that VR is most effective for patients with severe or higher pain and may be less effective or may not be needed for patients experiencing minimal pain levels. For example, some studies used VR to reduce the amount of opioids used (Pandrangi et al., 2022). VR can also reduce fear and anxiety of patients in the pre-operation room waiting to go to surgery, even if they are not experiencing pain (Flores et al., 2023). Immersive VR analgesia is poised to become even more effective in the near future, as the technology rapidly becomes much more immersive, as several competing major tech companies invest billions of dollars into consumer level VR technology, making VR easier to use, more comfortable, more immersive, and less expensive.
The within-subject design used in the present study has several potential limitations. It is possible that the first treatment could “carry over” or influence the response on the second treatment. If so, this would complicate interpretation. This is one known potential drawback that can sometimes happen when using a repeated measures design. To reduce carry over effects, we used a 5 minute “wash out” period between treatments and our pre-analyses of treatment order found no carry over effects in the current study.
Another potential shortcoming of the within subject design we used, is that researchers and participants remained aware of the treatment conditions (Campbell and Stanley, 1963; Schulz and Grimes, 2002). Since each person receives both VR treatment conditions, participants are more likely to become aware of the hypothesis, and this awareness may potentially influence their responses (Campbell and Stanley, 1963). Future randomized controlled studies using between groups designs, blinding, and larger sample sizes are recommended. Also, compared to some medical procedures (e.g., burn wound care lasting approximately 25 min), (Hoffman et al., 2020; Hoffman et al., 2019), the stimulus levels and stimulus durations used in the current study may or may not generalize to clinical settings.
Researchers have long speculated (Hoffman et al., 2000; Hoffman, 1998) that immersive VR reduces acute pain by diverting attention, but to our knowledge, to date, only three VR analgesia studies have measured how much attention is distracted by VR (Hoffman et al., 2003; Hoffman, 2021; Hoffman et al., 2023). More mechanistic research on how VR reduces pain is needed.
The current study tested our hypothesis that VR reduces pain by diverting attentional resources that would otherwise be used to process nociceptive signals. As predicted, immersive VR increased how “present” participants felt in VR and was more effective than semi-immersive VR at reducing how much pain participants reported during brief thermal stimuli. In addition, results from the odd number task in the current study suggest that the immersive VR system captured more of participants’ attention than the semi-immersive VR, as evidenced by significantly lower accuracy on the attention demanding task, during immersive VR analgesia, conceptually replicating the avatar VR study of (Hoffman, 2021) for the first time.
Despite a growing number of research studies on VR analgesia (Trost et al., 2021; Colloca et al., 2020; Honzel et al., 2019; Achanccaray et al., 2018), there are very few studies exploring patient characteristics that may predict individual differences in VR analgesia effectiveness. Some traditional (non-VR) psychological pain reduction techniques are much more effective for some subsets of participants. For example, in research by (Colloca et al., 2020) on the placebo effect, people with low catastrophizing scores showed a significantly greater placebo effect. The placebo effect was much less effective for high catastrophizers. In contrast, secondary sub-analyses in the current study showed that immersive VR was still effective at higher pain intensity levels, and immersive VR was widely effective regardless of gender, race, or participant’s tendency to catastrophize.
The current laboratory pain study is designed to increase our understanding of how VR reduces pain, towards the goal of making VR more effective at reducing pain in clinical settings (e.g., during burn wound cleaning). More effective VR may help reduce reliance on opioid analgesics during painful medical procedures (i.e., providing insights into improving pain management in clinical research and practice). A clearer understanding of how VR reduces pain may help guide development of more effective VR analgesia systems in the future. Additional research and development of VR analgesia and especially more research on how VR reduces pain and how to make it more effective, is recommended.
The raw data supporting the conclusions of this article will be made available by the authors, without undue reservation.
The studies involving humans were approved by the University of Washington Human Research Review Committee, Study number 0007801. The studies were conducted in accordance with the local legislation and institutional requirements. The participants provided their written informed consent to participate in this study. Written informed consent was obtained from the individual(s) for the publication of any potentially identifiable images or data included in this article.
HH: Conceptualization, Investigation, Writing–original draft, Writing–review and editing, Data curation, Methodology. CS: Formal Analysis, Writing–original draft, Writing–review and editing. LC: Formal Analysis, Writing–original draft, Writing–review and editing. LS: Writing–original draft, Writing–review and editing, Conceptualization, Formal Analysis, Visualization. SD: Formal Analysis, Writing–original draft, Writing–review and editing, Conceptualization. SL: Writing–original draft, Writing–review and editing, Conceptualization. KM: Conceptualization, Writing–original draft, Writing–review and editing. HF: Conceptualization, Writing–original draft, Writing–review and editing, Methodology.
The author(s) declare that financial support was received for the research, authorship, and/or publication of this article. The current study was philanthropically funded by the Mayday Fund (VRAnalgesia2022) to the University of Washington, to HH and Miles Fontenot. The completion of this work was supported by a grant of the Deutsche Forschungsgemeinschaft (SFB1158/B07) to HF, and R21 AR079140/AR/NIAMS NIH HHS/United States to LS. None of the funding agencies were involved in the study design, collection, analysis, interpretation of data, the writing of this article or the decision to submit it for publication.
The authors declare that the research was conducted in the absence of any commercial or financial relationships that could be construed as a potential conflict of interest.
All claims expressed in this article are solely those of the authors and do not necessarily represent those of their affiliated organizations, or those of the publisher, the editors and the reviewers. Any product that may be evaluated in this article, or claim that may be made by its manufacturer, is not guaranteed or endorsed by the publisher.
The Supplementary Material for this article can be found online at: https://www.frontiersin.org/articles/10.3389/frvir.2024.1452486/full#supplementary-material
Achanccaray, D., Pacheco, K., Carranza, E., and Hayashibe, M. (2018). “Immersive virtual reality feedback in a brain computer interface for upper limb rehabilitation,” in 2018 IEEE International Conference on Systems, Man, and Cybernetics (SMC), Miyazaki, Japan, 1006–1010.
Anderson, J. R., Reder, L. M., and Lebiere, C. (1996). Working memory: activation limitations on retrieval. Cogn. Psychol. 30, 221–256. doi:10.1006/cogp.1996.0007
Asefi Rad, A., and Wippert, P. M. (2024). Insights into pain distraction and the impact of pain catastrophizing on pain perception during different types of distraction tasks. Front. Pain Res. (Lausanne) 5, 1266974. doi:10.3389/fpain.2024.1266974
Bartley, E. J., and Fillingim, R. B. (2013). Sex differences in pain: a brief review of clinical and experimental findings. Br. J. Anaesth. 111, 52–58. doi:10.1093/bja/aet127
Baumann, L., Bello, C., Georg, F. M., Urman, R. D., Luedi, M. M., and Andereggen, L. (2023). Acute pain and development of opioid use disorder: patient risk factors. Curr. Pain Headache Rep. 27, 437–444. doi:10.1007/s11916-023-01127-0
Campbell, D., and Stanley, J. C. (1963). Experimental and quasi-experimental designs for research. Boston, MA: Houghton Mifflin Company.
Caruso, T. J., George, A., Menendez, M., DE Souza, E., Khoury, M., Kist, M. N., et al. (2020). Virtual reality during pediatric vascular access: a pragmatic, prospective randomized, controlled trial. Paediatr. Anaesth. 30, 116–123. doi:10.1111/pan.13778
CDC (2013). Provisional data shows U.S. Drug overdose deaths top 100,000 in 2022. NCHS: a blog of the national center for health statisticds. Available at: https://blogs.cdc.gov/nchs/2023/05/18/7365/(Accessed November 15, 2023).
Colloca, L., Raghuraman, N., Wang, Y., Akintola, T., Brawn-Cinani, B., Colloca, G., et al. (2020). Virtual reality: physiological and behavioral mechanisms to increase individual pain tolerance limits. Pain 161, 2010–2021. doi:10.1097/j.pain.0000000000001900
Craik, F. (1983). On the transfer of information from temporary to permanent memory. Philosophical Trans. R. Soc. Lond. Ser. B302, 341–359. doi:10.1098/rstb.1983.0059
Dahlquist, L. M., Mckenna, K. D., Jones, K. K., Dillinger, L., Weiss, K. E., and Ackerman, C. S. (2007). Active and passive distraction using a head-mounted display helmet: effects on cold pressor pain in children. Health Psychol. 26, 794–801. doi:10.1037/0278-6133.26.6.794
Eccleston, C., and Crombez, G. (1999). Pain demands attention: a cognitive-affective model of the interruptive function of pain. Psychol. Bull. 125, 356–366. doi:10.1037//0033-2909.125.3.356
Eccleston, C., Fisher, E., Liikkanen, S., Sarapohja, T., Stenfors, C., Jääskeläinen, S. K., et al. (2022). A prospective, double-blind, pilot, randomized, controlled trial of an "embodied" virtual reality intervention for adults with low back pain. Pain 163, 1700–1715. doi:10.1097/j.pain.0000000000002617
Fabian, L. A., Mcguire, L., Goodin, B. R., and Edwards, R. R. (2011). Ethnicity, catastrophizing, and qualities of the pain experience. Pain Med. 12, 314–321. doi:10.1111/j.1526-4637.2010.01015.x
Feine, J. S., Bushnell, C. M., Miron, D., and Duncan, G. H. (1991). Sex differences in the perception of noxious heat stimuli. Pain 44, 255–262. doi:10.1016/0304-3959(91)90094-e
Flores, A., Hoffman, H. G., Navarro-Haro, M. V., Garcia-Palacios, A., Atzori, B., LE May, S., et al. (2023). Using immersive virtual reality distraction to reduce fear and anxiety before surgery. Healthc. (Basel) 11, 2697. doi:10.3390/healthcare11192697
Gold, J. I., and Mahrer, N. E. (2018). Is virtual reality ready for prime time in the medical space? A randomized control trial of pediatric virtual reality for acute procedural pain management. J. Pediatr. Psychol. 43, 266–275. doi:10.1093/jpepsy/jsx129
Gold, J. I., Soohoo, M., Laikin, A. M., Lane, A. S., and Klein, M. J. (2021). Effect of an immersive virtual reality intervention on pain and anxiety associated with peripheral intravenous catheter placement in the pediatric setting: a randomized clinical trial. JAMA Netw. Open 4, e2122569. doi:10.1001/jamanetworkopen.2021.22569
Gupta, A., Scott, K., and Dukewich, M. (2018). Innovative technology using virtual reality in the treatment of pain: does it reduce pain via distraction, or is there more to it? Pain Med. 19, 151–159. doi:10.1093/pm/pnx109
Gutierrez-Maldonado, J., Gutierrez-Martinez, O., and Cabas-Hoyos, K. (2011). Interactive and passive virtual reality distraction: effects on presence and pain intensity. Stud. Health Technol. Inf. 167, 69–73.
Hemphill, S., Nguyen, A., Kwong, J., Rodriguez, S. T., Wang, E., and Caruso, T. J. (2021). Virtual reality facilitates engagement in physical therapy in the pediatric CVICU. Pediatr. Phys. Ther. 33, E7–e9. doi:10.1097/pep.0000000000000769
Hemphill, S., Rodriguez, S., Wang, E., Koeppen, K., Aitken-Young, B., Jackson, C., et al. (2022). Virtual reality augments movement during physical therapy: a pragmatic randomized trial. Am. J. Phys. Med. Rehabil. 101, 229–236. doi:10.1097/phm.0000000000001779
Hitching, R., Hoffman, H. G., Garcia-Palacios, A., Adamson, M. M., Madrigal, E., Alhalabi, W., et al. (2023). The emerging role of virtual reality as an adjunct to procedural sedation and anesthesia: a narrative review. J. Clin. Med. 12, 843. doi:10.3390/jcm12030843
Hoffman, H. G. (1998). Virtual reality: a new tool for interdisciplinary psychology research. CyberPsychol. Behav. 1, 195–200. doi:10.1089/cpb.1998.1.195
Hoffman, H. G. (2021). Interacting with virtual objects via embodied avatar hands reduces pain intensity and diverts attention. Sci. Rep. 11, 10672. doi:10.1038/s41598-021-89526-4
Hoffman, H. G., Doctor, J. N., Patterson, D. R., Carrougher, G. J., and Furness, T. A. (2000). Virtual reality as an adjunctive pain control during burn wound care in adolescent patients. Pain 85, 305–309. doi:10.1016/s0304-3959(99)00275-4
Hoffman, H. G., Fontenot, M. R., Garcia-Palacios, A., Greenleaf, W. J., Alhalabi, W., Curatolo, M., et al. (2023). Adding tactile feedback increases avatar ownership and makes virtual reality more effective at reducing pain in a randomized crossover study. Sci. Rep. 13, 7915. doi:10.1038/s41598-023-31038-4
Hoffman, H. G., Garcia-Palacios, A., Kapa, V., Beecher, J., and Sharar, S. R. (2003). Immersive virtual reality for reducing experimental ischemic pain. Int. J. Human-Computer Interact. 15, 469–486. doi:10.1207/s15327590ijhc1503_10
Hoffman, H. G., Patterson, D. R., Magula, J., Carrougher, G. J., Zeltzer, K., Dagadakis, S., et al. (2004a). Water-friendly virtual reality pain control during wound care. J. Clin. Psychol. 60, 189–195. doi:10.1002/jclp.10244
Hoffman, H. G., Patterson, D. R., Rodriguez, R. A., Pena, R., Beck, W., and Meyer, W. J. (2020). Virtual reality analgesia for children with large severe burn wounds during burn wound debridement. Front. Virtual Real 1, 602299. doi:10.3389/frvir.2020.602299
Hoffman, H. G., Richards, T. L., Coda, B., Bills, A. R., Blough, D., Richards, A. L., et al. (2004b). Modulation of thermal pain-related brain activity with virtual reality: evidence from fMRI. Neuroreport 15, 1245–1248. doi:10.1097/01.wnr.0000127826.73576.91
Hoffman, H. G., Richards, T. L., VAN Oostrom, T., Coda, B. A., Jensen, M. P., Blough, D. K., et al. (2007). The analgesic effects of opioids and immersive virtual reality distraction: evidence from subjective and functional brain imaging assessments. Anesth. Analg. 105, 1776–1783. doi:10.1213/01.ane.0000270205.45146.db
Hoffman, H. G., Rodriguez, R. A., Gonzalez, M., Bernardy, M., Pena, R., Beck, W., et al. (2019). Immersive virtual reality as an adjunctive non-opioid analgesic for pre-dominantly Latin American children with large severe burn wounds during burn wound cleaning in the intensive care unit: a pilot study. Front. Hum. Neurosci. 13, 262. doi:10.3389/fnhum.2019.00262
Hoffman, H. G., Seibel, E. J., Richards, T. L., Furness, T. A., Patterson, D. R., and Sharar, S. R. (2006). Virtual reality helmet display quality influences the magnitude of virtual reality analgesia. J. Pain 7, 843–850. doi:10.1016/j.jpain.2006.04.006
Hoffman, H. G., Sharar, S. R., Coda, B., Everett, J. J., Ciol, M., Richards, T., et al. (2004c). Manipulating presence influences the magnitude of virtual reality analgesia. Pain 111, 162–168. doi:10.1016/j.pain.2004.06.013
Hoffman Hg, S. C., Coron, L., Simons, L. E., Drever, S., LE May, S., Mason, K. P., et al. (2023). Increasing presence enhances virtual reality analgesia and draws more attention into virtual reality in a randomized crossover study.
Honzel, E., Murthi, S., Brawn-Cinani, B., Colloca, G., Kier, C., Varshney, A., et al. (2019). Virtual reality, music, and pain: developing the premise for an interdisciplinary approach to pain management. Pain 160, 1909–1919. doi:10.1097/j.pain.0000000000001539
Iidaka, T., Anderson, N. D., Kapur, S., Cabeza, R., and Craik, F. I. (2000). The effect of divided attention on encoding and retrieval in episodic memory revealed by positron emission tomography. J. Cogn. Neurosci. 12, 267–280. doi:10.1162/089892900562093
Jacoby, L. L., Woloshyn, V., and Kelley, C. (1989). Becoming famous without being recognized - unconscious influences of memory produced by dividing attention. J. Exp. Psychology-General 118, 115–125. doi:10.1037/0096-3445.118.2.115
Jeffs, D. A., Spray, B. J., Baxley, L., Braden, E., Files, A., Marrero, E., et al. (2023). Comparing novel virtual reality and nursing standard care on burn wound care pain in adolescents: a randomized controlled trial. J. Spec. Pediatr. Nurs. 29, e12419. doi:10.1111/jspn.12419
Jeffs, D., Dorman, D., Brown, S., Files, A., Graves, T., Kirk, E., et al. (2014). Effect of virtual reality on adolescent pain during burn wound care. J. Burn Care Res. 35, 395–408. doi:10.1097/bcr.0000000000000019
Jensen, M. P. (2003). The validity and reliability of pain measures in adults with cancer. J. Pain 4, 2–21. doi:10.1054/jpai.2003.1
Kanad, N., Özalp Gerçeker, G., Eker, İ., and Şen Susam, H. (2023). The effect of virtual reality on pain, fear and emotional appearance during blood draw in pediatric patients at the hematology-oncology outpatient clinic: a randomized controlled study. Eur. J. Oncol. Nurs. 68, 102495. doi:10.1016/j.ejon.2023.102495
Keefe, F. J., Huling, D. A., Coggins, M. J., Keefe, D. F., Rosenthal, Z. M., Herr, N. R., et al. (2012). Virtual reality for persistent pain: a new direction for behavioral pain management. Pain 153, 2163–2166. doi:10.1016/j.pain.2012.05.030
Keefe, F. J., Main, C. J., and George, S. Z. (2018). Advancing psychologically informed practice for patients with persistent musculoskeletal pain: promise, pitfalls, and solutions. Phys. Ther. 98, 398–407. doi:10.1093/ptj/pzy024
Kim, T. K., and Park, J. H. (2019). More about the basic assumptions of t-test: normality and sample size. Korean J. Anesthesiol. 72, 331–335. doi:10.4097/kja.d.18.00292
Law, E. F., Dahlquist, L. M., Sil, S., Weiss, K. E., Herbert, L. J., Wohlheiter, K., et al. (2011). Videogame distraction using virtual reality technology for children experiencing cold pressor pain: the role of cognitive processing. J. Pediatr. Psychol. 36, 84–94. doi:10.1093/jpepsy/jsq063
Lind, A., Ahsan, M., Totzeck, M., AL-Rashid, F., Haddad, A., Dubler, S., et al. (2023). Virtual reality-assisted distraction during transcatheter aortic valve implantation under local anaesthesia: a randomised study. Int. J. Cardiol. 387, 131130. doi:10.1016/j.ijcard.2023.131130
Maani, C. V., Hoffman, H. G., Fowler, M., Maiers, A. J., Gaylord, K. M., and Desocio, P. A. (2011a). Combining ketamine and virtual reality pain control during severe burn wound care: one military and one civilian patient. Pain Med. 12, 673–678. doi:10.1111/j.1526-4637.2011.01091.x
Maani, C. V., Hoffman, H. G., Morrow, M., Maiers, A., Gaylord, K., Mcghee, L. L., et al. (2011b). Virtual reality pain control during burn wound debridement of combat-related burn injuries using robot-like arm mounted VR goggles. J. Trauma 71, S125–S130. doi:10.1097/ta.0b013e31822192e2
Matamala-Gomez, M., Donegan, T., Bottiroli, S., Sandrini, G., Sanchez-Vives, M. V., and Tassorelli, C. (2019). Immersive virtual reality and virtual embodiment for pain relief. Front. Hum. Neurosci. 13, 279. doi:10.3389/fnhum.2019.00279
Mccaul, K. D., and Malott, J. M. (1984). Distraction and coping with pain. Psychol. Bull. 95, 516–533. doi:10.1037//0033-2909.95.3.516
Mcsherry, T., Atterbury, M., Gartner, S., Helmold, E., Searles, D. M., and Schulman, C. (2018). Randomized, crossover study of immersive virtual reality to decrease opioid use during painful wound care procedures in adults. J. Burn Care Res. 39, 278–285. doi:10.1097/BCR.0000000000000589
National Academies of Sciences (2009). National Academies of sciences, engineering, and medicine. Washington DC: The National Acaademies Press.
Paller, C. J., Campbell, C. M., Edwards, R. R., and Dobs, A. S. (2009). Sex-based differences in pain perception and treatment. Pain Med. 10, 289–299. doi:10.1111/j.1526-4637.2008.00558.x
Pandrangi, V. C., Shah, S. N., Bruening, J. D., Wax, M. K., Clayburgh, D., Andersen, P. E., et al. (2022). Effect of virtual reality on pain management and opioid use among hospitalized patients after head and neck surgery: a randomized clinical trial. JAMA Otolaryngology–Head & Neck Surg. 148, 724–730. doi:10.1001/jamaoto.2022.1121
Payne, O., Smith, V., Rolnik, D. L., Davies-Tuck, M., Warty, R., Seo, D., et al. (2022). Virtual reality and its use in post-operative pain following laparoscopy: a feasibility study. Sci. Rep. 12, 13137. doi:10.1038/s41598-022-17183-2
Peterson, B. N., Hitching, R., Howard, L., Zhu, K., Fontenot, M. R., Alhalabi, W., et al. (2021). Immersive virtual reality: a safe, scalable, non-opioid analgesic for military and veteran patients. Front. Virtual Real. 2. doi:10.3389/frvir.2021.742290
Raja, S. N., Carr, D. B., Cohen, M., Finnerup, N. B., Flor, H., Gibson, S., et al. (2020). The revised International Association for the Study of Pain definition of pain: concepts, challenges, and compromises. Pain 161, 1976–1982. doi:10.1097/j.pain.0000000000001939
Rohilla, L., Agnihotri, M., Trehan, S. K., Sharma, R. K., and Ghai, S. (2018). Effect of music therapy on pain perception, anxiety, and opioid use during dressing change among patients with burns in India: a quasi-experimental, cross-over pilot study. Ostomy Wound Manage 64, 40–46. doi:10.25270/owm.2018.10.4046
Rosenberg, L., Rosenberg, M., Robert, R., Richardson, L., Sharp, S., Holzer, C. E., et al. (2015). Does acute stress disorder predict subsequent posttraumatic stress disorder in pediatric burn survivors? J. Clin. Psychiatry 76, 1564–1568. doi:10.4088/jcp.14m09365
Rosenberg, L., Rosenberg, M., Sharp, S., Thomas, C. R., Humphries, H. F., Holzer, C. E., et al. (2018). Does acute propranolol treatment prevent posttraumatic stress disorder, anxiety, and depression in children with burns? J. Child. Adolesc. Psychopharmacol. 28, 117–123. doi:10.1089/cap.2017.0073
Schulz, K. F., and Grimes, D. A. (2002). Blinding in randomised trials: hiding who got what. Lancet 359, 696–700. doi:10.1016/s0140-6736(02)07816-9
Slater, M. (2018). Immersion and the illusion of presence in virtual reality. Br. J. Psychol. 109, 431–433. doi:10.1111/bjop.12305
Slater, M., Usoh, M., and Steed, A. (1994). Depth of presence in virtual environments. Presence teleoper. Virtual Environ. 3, 130–144. doi:10.1162/pres.1994.3.2.130
Slater, M., and Wilbur, S. (1997). A framework for immersive virtual environments (FIVE): speculations on the role of presence in virtual environments. Presence teleoper. Virtual Environ. 6, 603–616. doi:10.1162/pres.1997.6.6.603
Smith, K. L., Wang, Y., and Colloca, L. (2022). Impact of virtual reality technology on pain and anxiety in pediatric burn patients: a systematic review and meta-analysis. Front. Virtual Real 2, 751735. doi:10.3389/frvir.2021.751735
Sullivan, M. J. L., Bishop, S. R., and Pivik, J. (1995). The pain catastrophizing scale: development and validation. Psychol. Assess. 7, 524–532.
Trost, Z., France, C., Anam, M., and Shum, C. (2021). Virtual reality approaches to pain: toward a state of the science. Pain 162, 325–331. doi:10.1097/j.pain.0000000000002060
VAN DER Heijden, M. J. E., Jeekel, J., Rode, H., Cox, S., VAN Rosmalen, J., Hunink, M. G. M., et al. (2018). Can live music therapy reduce distress and pain in children with burns after wound care procedures? A randomized controlled trial. Burns 44, 823–833. doi:10.1016/j.burns.2017.12.013
Wandell, B. A., Dumoulin, S. O., and Brewer, A. A. (2007). Visual field maps in human cortex. Neuron 56, 366–383. doi:10.1016/j.neuron.2007.10.012
Wender, R., Hoffman, H. G., Hunner, H. H., Seibel, E. J., Patterson, D. R., and Sharar, S. R. (2009). Interactivity influences the magnitude of virtual reality analgesia. J. Cyber Ther. Rehabil. 2, 27–33.
Williamson, A., and Hoggart, B. (2005). Pain: a review of three commonly used pain rating scales. J. Clin. Nurs. 14, 798–804. doi:10.1111/j.1365-2702.2005.01121.x
Xiang, H., Shen, J., Wheeler, K. K., Patterson, J., Lever, K., Armstrong, M., et al. (2021). Efficacy of smartphone active and passive virtual reality distraction vs standard care on burn pain among pediatric patients: a randomized clinical trial. JAMA Netw. Open 4, e2112082. doi:10.1001/jamanetworkopen.2021.12082
Keywords: virtual reality, pain, distraction, analgesia, non-pharmacologic
Citation: Hoffman HG, Seibel CC, Coron L, Simons LE, Drever S, Le May S, Mason KP and Flor H (2024) Increasing presence via a more immersive VR system increases virtual reality analgesia and draws more attention into virtual reality in a randomized crossover study. Front. Virtual Real. 5:1452486. doi: 10.3389/frvir.2024.1452486
Received: 21 June 2024; Accepted: 13 August 2024;
Published: 04 September 2024.
Edited by:
Michael J. Roy, Uniformed Services University, United StatesReviewed by:
Vishnunarayan Girishan Prabhu, University of North Carolina at Charlotte, United StatesCopyright © 2024 Hoffman, Seibel, Coron, Simons, Drever, Le May, Mason and Flor. This is an open-access article distributed under the terms of the Creative Commons Attribution License (CC BY). The use, distribution or reproduction in other forums is permitted, provided the original author(s) and the copyright owner(s) are credited and that the original publication in this journal is cited, in accordance with accepted academic practice. No use, distribution or reproduction is permitted which does not comply with these terms.
*Correspondence: Hunter G. Hoffman, aHVudGhvZmY5QGdtYWlsLmNvbQ==
Disclaimer: All claims expressed in this article are solely those of the authors and do not necessarily represent those of their affiliated organizations, or those of the publisher, the editors and the reviewers. Any product that may be evaluated in this article or claim that may be made by its manufacturer is not guaranteed or endorsed by the publisher.
Research integrity at Frontiers
Learn more about the work of our research integrity team to safeguard the quality of each article we publish.