- Department of Human and Engineered Environmental Studies Graduate School of Frontier Sciences, The University of Tokyo, Chiba, Japan
As the demand for realistic sensations in virtual reality (VR) environments escalates, interfaces that exploit cross-modal interactions between vision and haptics are gaining prominence. Pseudo-haptics, offering a tactile illusion without mechanical feedback devices, has emerged as a viable solution to enhance user immersion by simulating various sensations such as texture, fluid resistance, and weight. However, a potential issue in the long-term effectiveness of these illusions, particularly habituation, where the illusion diminishes after prolonged exposure, remains a concern. This study investigates whether habituation to pseudo-haptic weight illusions occurs with extended use. We conducted quantitative measurements of pseudo-haptic weight perception before and after prolonged exposure. The findings indicate a diminishing effect of pseudo-haptic weight illusion with repeated exposure, particularly among participants with high accuracy in weight perception. This study contributes important insights into the design of VR experiences, highlighting the need to consider the habituation effects when implementing pseudo-haptic illusions for weight perception.
1 Introduction
With the proliferation of mobile smart devices, virtual reality (VR) and augmented reality (AR) technologies are becoming the primary platforms for human communication. Recent advancements in 5G technology and consumer-grade VR hardware, such as the Meta Quest 3 and HTC Vive Cosmos Elite, have significantly expanded the potential for AR and VR applications, thereby increasing the demand for more realistic interactions within virtual environments.
Tactile feedback plays a crucial role in enhancing the VR and AR experience, providing a more immersive sense of presence when combined with visual and auditory stimuli (Coles et al., 2010). The ability to convey the texture and shape of virtual objects can significantly affect user behavior and emotional responses (Gatti et al., 2013; Jin, 2011), proving especially beneficial in educational, training, and entertainment applications (Ramírez et al., 2018).
However, the provision of realistic tactile feedback typically requires complex, bulky actuators and can be cost-prohibitive. To overcome these challenges, there is a need for innovative, compact, and cost-effective display technologies that can emulate tactile sensations.
In this context, interfaces that leverage cross-modal perceptions between vision and haptics are attracting interest. Pseudo-haptics (Lécuyer, 2009), which induce tactile sensations without mechanical feedback devices, utilize sensory inconsistencies between actual physical movements and the visual feedback of these movements. This discrepancy can create a tactile illusion in various contexts (Ujitoko and Ban, 2021; Ujitoko et al., 2019; Pusch et al., 2008; Samad et al., 2019; Hirao et al., 2020), including outside of AR/VR environments, such as with the deceleration of a mouse pointer simulating physical resistance.
Compared to traditional haptic technologies, pseudo-haptics offer a cost-effective solution without the encumbrance of additional hardware, aside from a head-mounted display (HMD). This method not only circumvents common issues associated with AR/VR interfaces, such as weak feedback, excessive weight, and low resolution but also facilitates broader application across various content domains (Kashihara and Shiota, 2014; Nomoto et al., 2016; Kang et al., 2019).
Nevertheless, the integration of pseudo-haptics into practical applications must address the variability of its effects, both between individuals (inter-individual instability (Ban and Ujitoko, 2023)) and within the same individual over different sessions (intra-individual instability). Habituation, or the reduction in sensory perception with prolonged exposure, plays a significant role in this intra-individual variability and is particularly pertinent to long-term applications (Stratton, 1896; Shiller et al., 2020).
The extent to which habituation impacts pseudo-haptic phenomena remains uncertain. This study aims to determine whether such effects diminish with repeated exposure, specifically focusing on the perception of weight, as it is both a robust and quantifiable attribute in pseudo-haptic applications. We have developed an experimental protocol wherein participants engage in repeated weight assessments of virtual objects under pseudo-haptic conditions to explore this phenomenon comprehensively.
The remainder of this paper is structured as follows: Section 2 reviews related literature on the causative factors of pseudo-haptics and the habituations. Section 3 details the experimental design for assessing habituation in pseudo-haptic weight perception. Section 4 presents the experimental results, and Section 5 discusses their implications. Finally, Section 7 concludes the paper.
2 Related works
2.1 Weight perception in VR
Weight perception is a complex process involving not only cutaneous and proprioceptive feedback but also visual cues such as color and material. For instance, larger objects are perceived as heavier (Charpentier, 1891), while lighter-colored objects tend to be perceived as lighter (Walker and Walker, 2012). Sensory information, including haptic and visual inputs, is integrated in the brain to form the final judgment of an object’s weight. This complexity of weight perception presents both challenges and opportunities in VR environments.
In fields where precision in manual tasks, such as surgical training, or realism in gaming is critical, the demand for accurate weight simulation technology in VR is increasing (Giachritsis et al., 2009). However, developing interfaces for weight perception in VR is particularly challenging. Unlike texture or temperature, which can be sensed through a single finger, weight requires the object to be lifted, necessitating more complex interface designs. The varying magnitudes and directions of forces further complicate this task (Lim et al., 2021). These challenges highlight the need for innovative approaches to weight simulation in VR.
Recent advancements in VR technology have led to new opportunities in addressing these challenges in weight perception. Lim et al. conducted a systematic review of weight perception techniques in VR, highlighting various approaches including force feedback devices, pseudo-haptics, and multi-modal stimulation (Lim et al., 2021). Their review identified key challenges such as the need for more realistic and immersive weight rendering, the importance of considering individual differences in perception, and the potential of combining multiple techniques for enhanced realism. These findings provide a foundation for understanding the current state of weight perception in VR and guide future research directions in this field.
2.2 Haptic feedback technologies in VR
To address the challenges of weight perception in VR, various tactile feedback technologies have been developed. These can be broadly categorized into passive haptics and active haptics.
Passive haptics involves the use of physical objects as tactile substitutes synchronized with virtual interactions to provide haptic feedback. Hoffman demonstrated that holding a physical object with similar material and weight to its virtual counterpart enhances the realism of virtual environments (Hoffman, 1998). Similarly, Gerini et al. showed that this approach leads to more realistic grasping movements compared to conditions without physical objects (Gerini et al., 2023). The effectiveness of passive haptics has led to various studies exploring its applications and limitations (Liu et al., 2023; Azmandian et al., 2016; Clarence et al., 2022; Ban et al., 2012).
Active haptics, on the other hand, uses powered devices to generate force feedback. While these systems can provide more dynamic and varied sensations, they often require complex and costly hardware setups.
2.3 Pseudo-haptics: an alternative approach
Building upon the concept of passive haptics, pseudo-haptics offers an innovative approach by creating the illusion of touch through the manipulation of visual and motor cues (Lécuyer, 2009). While most of the pseudo-haptic approaches utilize physical proxies similar to passive haptics, some of the pseudo-haptic methods can also operate without any physical objects. This versatility allows pseudo-haptics to be applied in a wide range of scenarios, from those with tangible interfaces to purely virtual interactions (Ujitoko and Ban, 2021).
There are various methods for manipulating weight perception using pseudo-haptic cues. The most common approaches involve displaying exaggerated movement relative to actual displacement (Samad et al., 2019) or altering the perceived speed of an object’s motion (Hirao and Kawai, 2018). In this study, we adopted a method of displaying exaggerated movements relative to actual displacements, the effectiveness of which has been quantitatively proven by Samad et al.
Pseudo-haptic feedback can be initiated by manipulating the virtual object’s position relative to its actual tracked position using a control/display (C/D) ratio denoted as
The control/display (C/D) ratio, denoted as
Figure 1 illustrates this concept of weight perception manipulation via pseudo-haptics. The method manipulates the perceived position of the user’s hand by adjusting the displayed movement, based on the principle that lighter objects move more easily and heavier objects move with more difficulty, thereby creating an illusion of weight perception. In this figure, an example where the C/D ratio is less than 1 is shown, making an object appear heavier than it actually is.
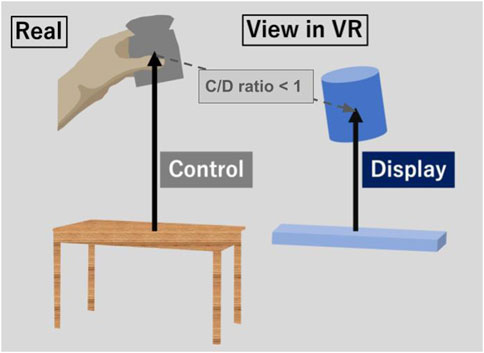
Figure 1. Conceptual diagram of weight perception manipulation using pseudo-haptics. This figure shows the case where the C/D ratio is less than 1, making the object feel heavier.
This approach of pseudo-haptics, whether used in conjunction with passive haptic elements or as a standalone technique, represents a promising direction in VR tactile feedback. It potentially offers more nuanced and adaptable sensory experiences while often maintaining simplicity and cost-effectiveness compared to complex active haptic systems.
2.4 Theoretical foundations of pseudo-haptics
Pseudo-haptics, which capitalizes on body awareness, is still not fully understood. Effective and robust self-localization relies on visual cues, while flexible position-sensing is based on body awareness (Harris, 1965). To further understand pseudo-haptics, various theories have been developed that explore the intricacies of body awareness to explain its mechanisms.
Research indicates that pseudo-haptic sensations may originate from bottom-up processes, beginning with sensory inputs and advancing to higher cognitive processing (Ernst and Banks, 2002). Conversely, other studies suggest top-down origins, where expectations and context-based predictions precede sensory information processing, incorporating memories and prior knowledge (Pusch and Lécuyer, 2011).
These theoretical perspectives on pseudo-haptics raise important questions about the persistence and adaptability of these effects over time, especially in the context of prolonged VR experiences.
2.5 Habituation and sensory adaptation in VR environments
The persistence and potential habituation of pseudo-haptic effects, particularly in weight perception, remain areas of ongoing research. While the immediate effectiveness of pseudo-haptic techniques has been demonstrated in various studies, the long-term stability of these effects, especially under repeated exposure, is less understood.
Habituation significantly affects perception, altering long-established perceptual modes. This phenomenon is closely related to sensory adaptation, where the brain adjusts its interpretation of sensory inputs in response to sustained stimuli. For instance, individuals who adapt to glasses that invert their left-right stereo vision eventually adjust to this altered reality; however, they experience notable disorientation upon removing the glasses (Stratton, 1896).
Similar adaptive phenomena have been observed in VR contexts. Shiller et al. reported that auditory delays during conversation lead to perceptual adjustments after continuous exposure for 30 min (Shiller et al., 2020). In the visual-tactile domain, Wright et al. explored prolonged exposure to mismatches between inertial and visual stimuli, demonstrating significant VR-induced sensory-motor habituations after sessions lasting longer than 2 minutes (Wright, 2013).
These habituations and adaptations, from a top-down perspective, are thought to occur through modifications in an individual’s predictive knowledge, necessitated by repeated exposure to the modified stimuli. This relearning process underscores the adaptability of human perception to sustained top-down influences, a crucial factor in VR experiences.
Given the top-down nature of pseudo-haptics suggested by some theories, we hypothesize that habituation and sensory adaptation are also likely in pseudo-haptic experiences, mirroring the adaptive changes observed in other sensory illusions driven by similar cognitive mechanisms. Understanding these potential habituation and adaptation effects is crucial for designing more effective and persistent pseudo-haptic experiences in VR environments, particularly for applications requiring sustained use such as extended training simulations or prolonged gaming sessions.
3 Experimental design
3.1 Overview
In this study, we investigated the impact of habituation on the perception of weight in virtual environments (VEs) enhanced by pseudo-haptics. Specifically, we compared the perceived weight of a cube-shaped virtual object under conditions with and without the application of pseudo-haptic feedback. We varied the duration of exposure to the pseudo-haptic environment prior to each weight assessment to explore the potential effects of habituation on weight perception.In other words, the two independent variables in this experiment are the C/D ratio and the exposure time to the virtual environment, and the dependent variable is weight perception. The C/D ratio, lifting height, and hardware equipment were based on the previous study by Samad et al. (2019).
3.2 Equipment
3.2.1 Hardware
As depicted in Figure 2A, the experimental setup was housed in an acoustically controlled room to eliminate external noise influences, although participants did not wear noise-canceling headphones to avoid device fatigue during extended sessions. We attached a tracker to a real object to continuously monitor its position. The VR environment was visualized through a VIVE Cosmos Elite HMD operating at a 90 Hz refresh rate, with positional tracking managed by VIVE Tracker v.3.0 software. Weight feedback was facilitated using TouchX technology from 3DSystem.
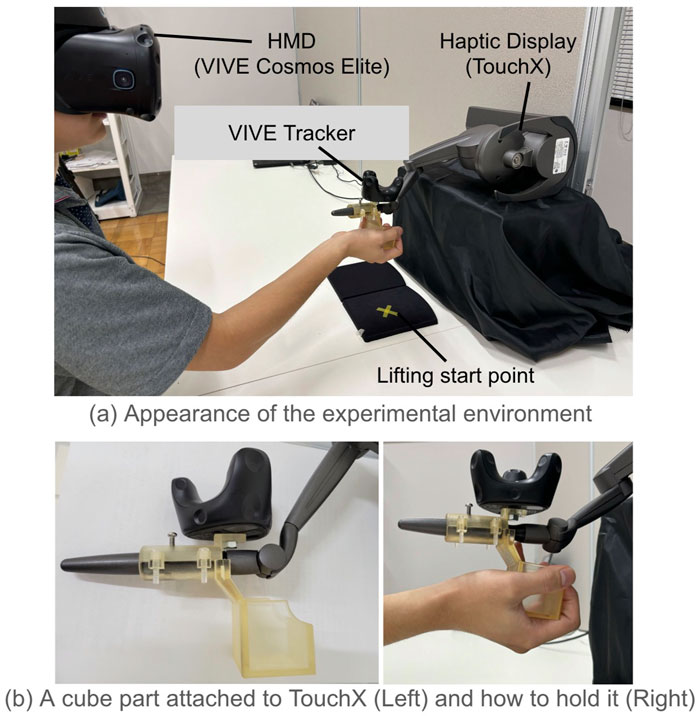
Figure 2. Experimental equipment. Participants lifted up the cube that is the same shape and size as the virtual one. (A) Appearance of the experimental environment. (B) A cup part attached to touchx (Left) and how to hold it (Right).
Cubic resin objects produced via 3D printing affixed to the TouchX device to maintain necessary rotational freedom and prevent arm movement interference (Figure 2B). Each cube, following dimensions used by Samad et al. (2019), measured 40 mm per side and weighed approximately 100 g—verified through preliminary testing to ensure it did not cause fatigue over prolonged use.
3.2.2 Software
The virtual scenario (Figure 3), constructed in Unity, reflected the precise dimensions and positions of real-world counterparts, consisting of a virtual table and cube. To control movement, two bars were set around the cube: a lower bar just above the table and an upper bar 200 mm higher, dictating the lifting range. A 100-mm diameter ring, moving in sync with the cube, served to limit horizontal excursions.For the pseudo-haptic weight perception, vertical movements of the real-world cube were tracked and translated into virtual heights based on the C/D ratio.
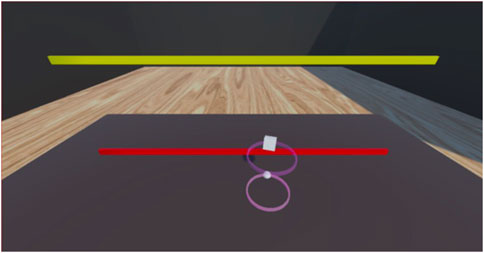
Figure 3. Virtual environment displayed on HMD. When the virtual cube is moved beyond the height of the upper bar, the bar turns red. When the virtual cube contacts with circular guide, it turns red to indicate a breach in movement protocol.
3.3 Experimental tasks
In this subsection, we describe the three types of tasks. The experiment consists lifting task, comparison task and weight perception accuracy verification task. In these tasks, participants equipped with a head-mounted display (HMD) and interacted with a virtual object that mimicked the real-world object in a virtual environment (see Figure 2).
The reason for applying a passive-haptic approach is that we needed to make the action of lifting an object as close to reality as possible (Gerini et al., 2023). have shown that the lack of haptic feedback significantly affects the gripping action, leading to odd movements that deviate from reality. To accurately assess weight, we considered that it was important to ensure that the gripping action closely resembled real-world movements.
The following sections will provide detailed explanations of each task.
3.3.1 Lifting task
Figure 4 shows the real world and the participant’s vision while performing the lifting task.
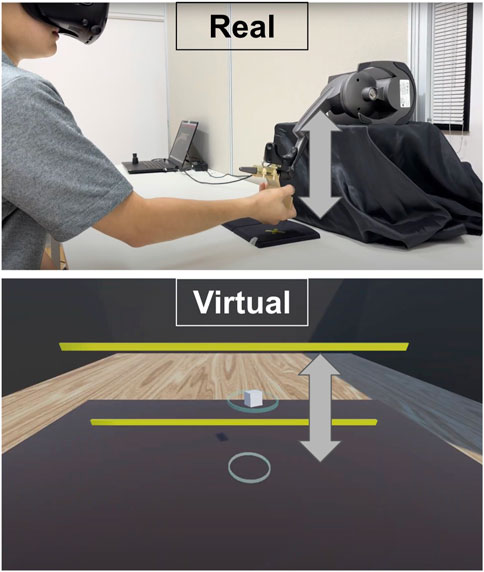
Figure 4. Overview of the lifting task. Participants lifted and put down the 230 g weighed virtual object a set number of times (10 or 100 times). Participants used their right hand to interact virtual objects. Participants could view the virtual cube, but the hand avatar was not used.
Participants lifted and put down the 230 g weighed virtual object a set number of times (10 or 100 times). Participants used their right hand to interact with virtual objects. The lifting height of the object was 20 cm on the virtual environment. C/D ratio was adjusted to either 0.8 or 1.0, influencing the weight perception.
This baseline of 230 g was selected to reduce fatigue of participants due to repetitive movements and to maintain weight discrimination accuracy. It is known that weight discrimination in a virtual environment is difficult when the weight is too light, such as around 50 g (Minamizawa et al., 2007). Following previous research (Samad et al., 2019), we selected a weight close to the weight of the controller of a commercially available VR device. This base weight also helped standardize the weight felt across different VR experiences. Additionally, pilot test confirmed that this weight did not cause significant fatigue to participants.
To optimize the effectiveness of the pseudo-haptic feedback, movement was restricted according to a detailed protocol. Participants were required to lift the object vertically to a height visually corresponding to 20 cm and maintain a lifting and lowering rhythm set to 55 beats per minute (BPM), regulated by a computerized metronome. When the virtual object went over the top bar, the bar turned red, and participants were instructed to lift the object until the top bar changed color. Lateral movements were minimized using a circular translucent guide displayed in the virtual environment, ensuring the object’s path remained as vertical as possible. When the virtual cube contacts this guide, it turns red, indicating a breach in movement protocol.
Participants were permitted to take brief, self-regulated breaks if deemed necessary, ensuring comfort and focus. Between different experimental conditions, sufficient rest periods of about 5 min were mandated, effectively preventing any significant fatigue among participants by the conclusion of the study.
3.3.2 Comparison task
A conceptual diagram of this task is shown in Figure 5. The purpose of this task was to assess the extent of weight perception alteration through pseudo-haptic effects. We measured the point of subjective equality (PSE) weight against a 230-g load held in the right hand. This measure helped quantify the effect size of the weight perception manipulation. For instance, if a participant perceived the weight in the right hand (230 g under manipulated displacement) to be equivalent to a 250-g load in the left hand, the PSE would be determined as greater than 230 g. This indicates that due to the pseudo-haptic effect, the 230-g load is perceived similarly to a 250-g load.
In the comparison task, participants lifted objects three times with each hand following the same procedure as the lifting task (see Figure 6). They then used a forced-choice selection to indicate which hand was holding the heavier object. In the C/D ratio condition of 0.8 (3rd condition and 4th condition in Figure 8), the amount of movement of the virtual object held in the right hand was restricted to 0.8 times its actual amount. On the other hand, in the C/D ratio condition of 1.0 (Weight Perception Accuracy Verification Task and 1st condition and 2nd condition in Figure 8), the amount of movement was not manipulated. Additionally, under any condition, the amount of movement of the object held in the left hand was not manipulated (C/D ratio 1.0).
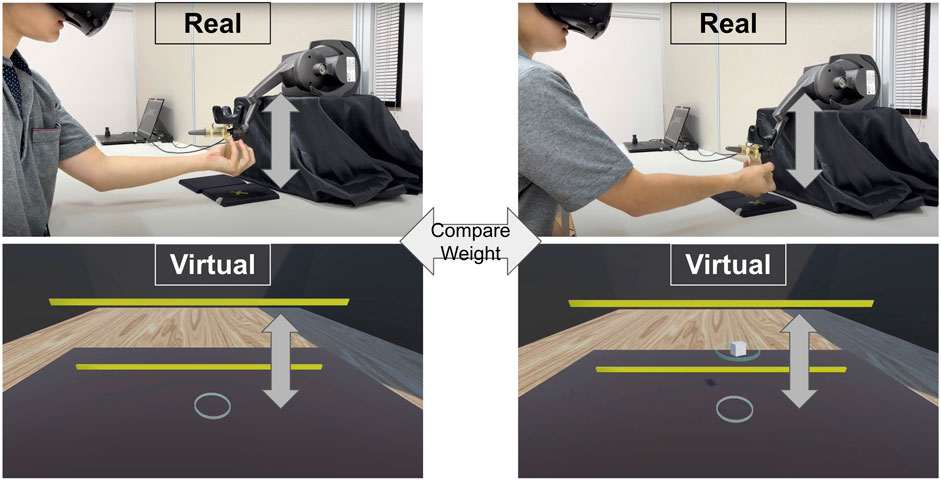
Figure 6. Overview of the comparison task. Participants lifted objects 3 times with each hand, then used a forced-choice selection to indicate which hand was holding the heavier object. Participants could view the virtual cube when they lifted with right hand, but they could not view it when the lifted with left hand.
It is important to note that during the comparison task, the virtual object was not displayed when participants lifted the physical object with their left hand. Participants lifted the physical object with their left hand until the bar changed color, despite the virtual object being invisible. The reason the object was made invisible during the left-hand lifting was to avoid eliminating the effects of habituation. Since no displacement manipulation by pseudo-haptics was applied when lifting the object with the left hand, there was a concern that making the virtual object visible could potentially disrupt any habituation to pseudo-haptics that may have developed during the lifting task.
To assess PSE, we employed the interleaved staircase method. This technique adjusts the weight presented by a haptic device to match the participant’s perceived weight under pseudo-haptic influence. Interleaved staircase method involved the following steps: The right hand consistently handled a 230-g load with manipulated displacement based on the C/D ratio. Adjustments to the load in the left hand, which underwent no displacement manipulation, were made based on participants’ feedback. If the participant felt the right hand was heavier, the weight in the left hand was increased by 10 g for the next comparison. Conversely, if the left hand was perceived as heavier, its load was reduced by 10 g. The process continued until five weight reversals were achieved across two interleaved sequences—one starting below the expected PSE and the other above it. These sequences were randomized and the method applied to both. The task concluded after five reversals, with the PSE calculated as the average of the last three reversals from both sequences.
The decision to use a 10 g step size for weight adjustments in the interleaved staircase method was based on results from a preliminary experiment. Initially, we tested a 5 g step size, but we found that the number of comparisons often exceeded 20, which resulted in participants reporting fatigue or discomfort. When we switched to a 10 g step size, these issues were mitigated, and we were still able to measure the PSE weight with sufficient precision. This was confirmed by the significant effects observed in the pseudo-haptic condition with a C/D ratio of 0.8, which indicated that the 10 g step size was adequate for detecting meaningful changes in perceived weight while reducing the overall strain on participants.
3.3.3 Weight perception accuracy verification task
At the beginning of the experiment, as part of the tutorial, participants completed a weight perception accuracy verification task. This task was based on the format of the comparison task, designed to assess each participant’s ability to accurately perceive and compare weights in the virtual environment.
In this task, participants were presented with six pairs of weights, and C/D ratios for both hands were set to 1.0. For each pair, they followed the same procedure as in the comparison task: lifting objects three times with each hand and then indicating which hand was holding the heavier object using a forced-choice selection. The weight in the right hand was consistently set at 230 g, while the left hand was presented with weights of 200 g, 210 g, 220 g, 240 g, 250 g, and 260 g in random order.
The accuracy of each participant’s weight perception was measured by the number of correct responses out of these six comparisons. A correct response was defined as successfully identifying the heavier weight in each pair. The results of this task provided a baseline for each participant’s weight discrimination ability, serving as a crucial reference point for considering individual differences in sensitivity to pseudo-haptic effects in subsequent analyses.
3.4 Experimental conditions
As shown in Figure 7, the experiment consisted of four conditions, manipulating two independent variables: the C/D ratio and the duration of exposure to the virtual environment (i.e., the number of repetitions in the lifting task). The experiment employed a within-subjects design, and each participant experienced all four experimental conditions in the order described below (see Figure 8).
We set the C/D ratio to two conditions: 1.0 and 0.8. A C/D ratio of 1.0 meant that the displayed movement matched the actual movement of the object, resulting in no pseudo-haptic effect. Conversely, a C/D ratio of 0.8 reduced the displayed movement to 0.8 times the actual movement (as illustrated in Figure 1), designed to induce a perception of increased weight through pseudo-haptic feedback.
The C/D ratio of 1.0 served as a control condition. The C/D ratio of 0.8 was selected based on a preliminary experiment with six participants and previous studies. In our preliminary testing, we compared C/D ratios of 0.8 and 1.25, finding significant pseudo-haptic effects only with 0.8, while 1.25 showed no notable effect. This aligns with Hannig et al.‘s research (Hannig and Deml, 2010), which showed that participants prefer feedback simulating heavier objects (C/D ratio <1) over lighter objects (C/D ratio >1).
For the lifting task, we employed two repetition conditions: 10 repetitions (short-term exposure) and 100 repetitions (long-term exposure). The 10-repetition condition served as a control to evaluate the impact of short-term pseudo-haptic feedback on weight perception. The reason for not setting the short-term condition to 0-repetitions was to avoid the influence of initial surprise toward the virtual environment during the comparison task. The choice of 100 repetitions for the long-term condition was confirmed through preliminary testing to ensure it was within a range that maintained participant concentration without inducing fatigue, thus preserving the integrity of weight perception over time. This setup allowed us to evaluate the impact of exposure duration on weight perception while ensuring consistent experimental conditions throughout the study.
3.5 Procedure
Figure 8 illustrates the experimental procedure, which consisted of a tutorial session followed by four experimental conditions.
3.5.1 Tutorial session
Prior to the main experiment, participants completed a comprehensive tutorial. This session included practice for both the lifting task and the comparison task. The weight perception accuracy verification task, which also served as practice for the comparison task, measured each participant’s baseline ability to discriminate weights in the virtual environment.
3.5.2 Main experiment
Following the tutorial, participants proceeded through four experimental conditions. In each condition, participants first performed the lifting task, immediately followed by the comparison task where the Point of Subjective Equality (PSE) weight was measured.
The objectives for each condition were as follows:
1. 1st condition, 10-repetition with 1.0 C/D ratio: Establish a baseline for each participant’s weight perception.
2. 2nd condition, 100-repetition with 1.0 C/D ratio: Investigate the effects of prolonged interaction within the virtual environment.
3. 3rd condition, 10-repetition with 0.8 C/D ratio: Evaluate the effect of pseudo-haptics on weight perception.
4. 4th condition, 100-repetition with 0.8 C/D ratio: Examine the long-term effects of experiencing pseudo-haptics.
The order of conditions was strategically chosen. We started with a C/D ratio of 1.0 to minimize potential habituation effects that could complicate the transition to a C/D ratio of 0.8. Additionally, each C/D ratio condition began with 10 repetitions of the task to limit the influence of habituation potentially caused by the longer, 100-repetition tasks.
Between different experimental conditions, participants were given sufficient rest periods of about 5 min to prevent fatigue and maintain focus throughout the study.
3.6 Participants
Participants were enlisted for a study titled “Study on Weight Perception in VR Space,” aimed at examining the effects of pseudo-haptics on weight perception. To prevent performance bias, the participants were not informed that the experiment involved pseudo-haptics or that it aimed to investigate habituation effects.
A total of 27 healthy, right-handed individuals (14 males and 13 females) participated in the experiment. The average age was 25.7 years, with a standard deviation of 7.7 years. Of these participants, 15 had previous experience with VR technologies.
The study protocols were reviewed and approved by the Ethics Committee of the University of Tokyo (ethical review number: 19–173). Informed consent was obtained from all participants before commencing the experiments. Upon completion, the true purpose of the study was disclosed to the participants, and their consent to use their data was reaffirmed.
4 Result and analysis
Figure 9 displays a box plot of the Point of Subjective Equality (PSE) weight values as measured during the comparison task for each experimental condition.
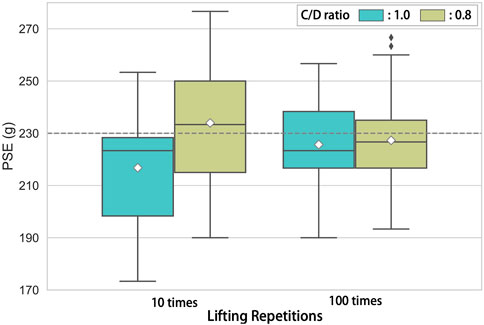
Figure 9. Box plot of PSE weight for each C/D ratio and lifting task repetition (white diamonds indicate mean, black diamonds indicate outliers, N = 27). Data points that are away from the median by more than 1.5 times the interquartile range are denoted as outliers.
4.1 Statistical analysis
We performed a two-way repeated-measures ANOVA to assess the impact of long-term exposure with weight perception manipulation using pseudo-haptics on the PSE weight. The Shapiro-Wilk test confirmed the normality of the data for each feature. Factors included in the ANOVA were the C/D ratio (0.8, 1.0) and the number of repetitions in the lifting task (10, 100), with a significance level set at p < 0.05. The ANOVA results indicated a significant main effect of the C/D ratio (F (1, 26) = 7.49, p = 0.0110) and a significant interaction between the C/D ratio and lifting repetitions (F (1, 26) = 7.58, p = 0.0106). However, the main effect of the lifting repetitions was not significant (F (1, 26) = 0.337, p = 0.567).
4.2 Post hoc tests
Following the ANOVA, post hoc tests with Holm’s correction were conducted. The results (see Table 1A) showed significant differences in PSE weights: participants perceived the weight at a 0.8 C/D ratio with 10 repetitions as significantly heavier than at a 1.0 C/D ratio with 10 repetitions (F (1, 26) = 10.55, p = 0.0128). Additionally, PSE weights at a 1.0 C/D ratio with 100 repetitions were significantly heavier than with 10 repetitions (F (1, 26) = 6.16, p = 0.0396). However, the difference between 100 repetitions and 10 repetitions at a 0.8 C/D ratio was not statistically significant (F (1, 26) = 4.24, p = 0.0661).
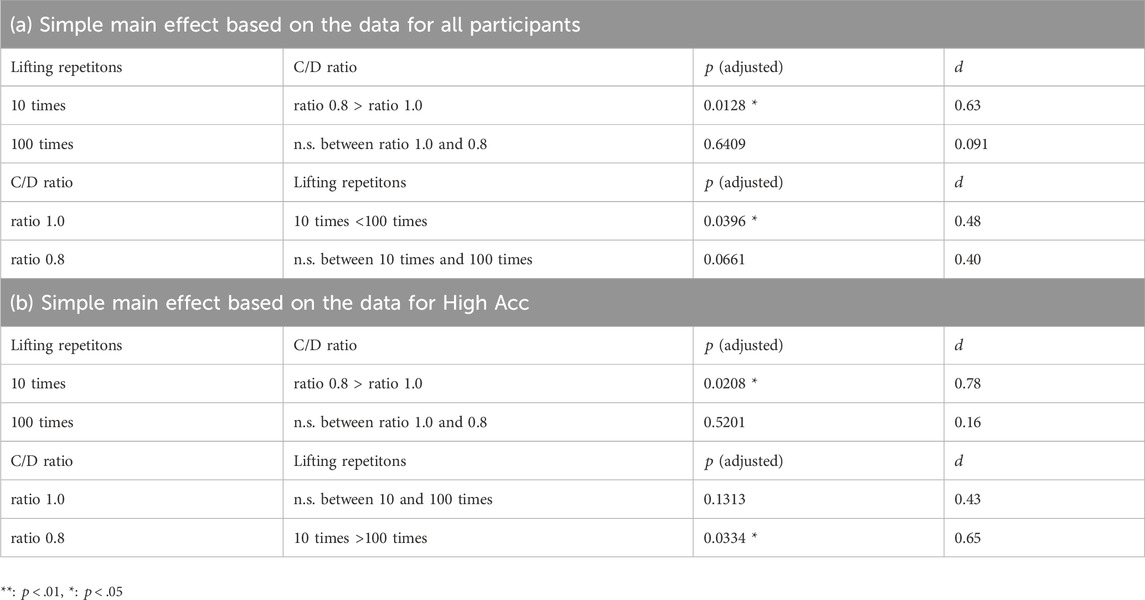
Table 1. Results of paired t-test as post hoc tests for PSE weight.
4.3 Control condition analysis
In a control setting, with a C/D ratio of 1.0 and 10 lifting repetitions, a one-sample t-test comparing the PSE weight to a reference weight of 230 g showed a significant decrease in perceived weight (t (26) = −3.025, p = 0.0055), indicating the PSE weight was significantly lower than 230 g.
4.4 Weight perception accuracy verification task
Figure 10 presents a histogram of weight comparison accuracy during the weight perception accuracy verification task. Since the task involved binary choices, the chance level of correct answers is 0.50. The median accuracy was 0.67, showing most participants could identify the heavier of two weights in four out of six trials. Participants were divided into High Accuracy (High Acc, accuracy
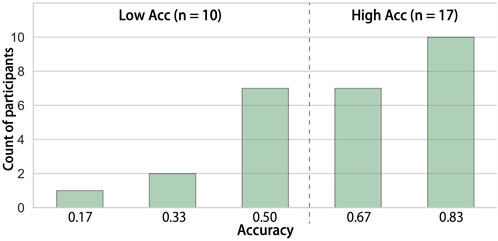
Figure 10. Histogram of the accuracy in the weight perception accuracy verification task. Participants were divided into two groups, named High Acc and Low Acc, based on whether their accuracy was above or below the median value of 0.67.
4.5 Differential analysis by accuracy
Figure 11 shows the PSE weight box plot for each group. The two-factor ANOVA for the High Acc group revealed a significant main effect of the C/D ratio (F (1, 16) = 8.07, p = 0.0118) and a significant interaction between the C/D ratio and lifting repetitions (F (1, 16) = 6.09, p = 0.0253), but no significant effect of lifting repetitions alone (F (1, 16) = 0.604, p = 0.448). Post hoc tests with Holm’s correction indicated significant differences in PSE weights between a 0.8 C/D ratio at 10 repetitions and a 1.0 ratio at the same number of repetitions (F (1, 16) = 10.44, p = 0.0208), and a significant decrease in PSE weight at 100 repetitions compared to 10 at a 0.8 C/D ratio (F (1, 16) = 7.15, p = 0.0334). For the Low Acc group, the ANOVA showed no significant differences in any main effects or interactions.
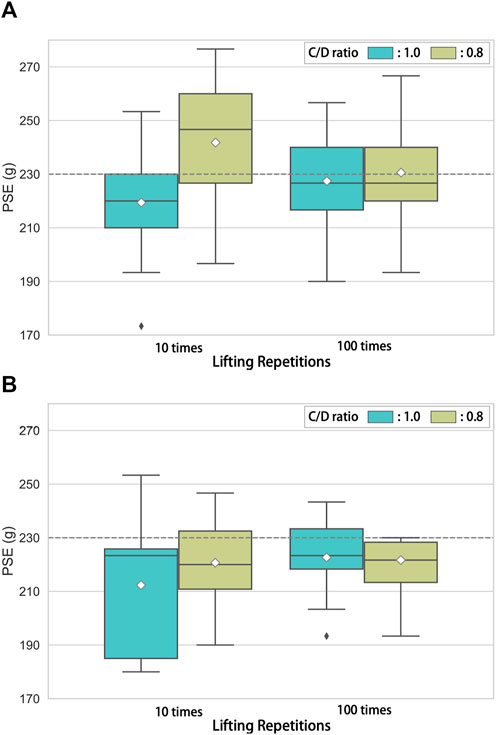
Figure 11. Box plots of PSE weight for each C/D ratio and lifting task repetition in each group. (A) Result of High Acc group (N = 17). (B) Result of Low Acc group (N = 10).
5 Discussion
5.1 PSE weight perception and dominant hand
Under the control condition with a C/D ratio of 1.0 and 10 lifting repetitions, the PSE weight was significantly lower than the actual weight of 230 g, suggesting that the load of approximately 217 g applied to the left hand was perceived as equivalent to the 230 g on the right hand. This difference can be attributed to the handedness of the participants, as all were right-handed (see Section 3.6). Typically, the non-dominant hand (in this case, the left) is less adept at weight handling, potentially leading to a heightened sensitivity to weight differences (Sato and Kikuchi, 1967). This is supported by one participant’s comment post-experiment, indicating a perceived heavier weight in the less dominant hand. This result indicates that an offset, accounting for the strength difference between the left and right hands, was consistently present in all four PSE weights depicted in Figure 9. However, since this offset is homogeneous, this offset does not compromise the validity of our relative comparisons across the PSE weights.
5.2 Increased PSE weight with prolonged lifting repetitions
Significant increases in PSE weight were observed with a C/D ratio of 1.0 after 100 lifting repetitions compared to just 10. This phenomenon is likely due to fatigue, as suggested by participants who reported arm tiredness post-task. Fatigue is known to lead to an overestimation of the force (or mass) being exerted, and individuals tend to apply greater force when lifting objects (Jones and Burgess, 1997).
5.3 PSE weight in the group with low weight perception accuracy
In participants categorized within the Low Acc group, no significant differences of pseudo-haptic effects were observed, likely due to high variability in PSE weight reporting. This variability may mask any subtle pseudo-haptic effects, leading to inconclusive results regarding habituation in this group.
5.4 PSE weight in the group with high weight perception accuracy
In the High Acc group, the PSE weight differences were significant between the 0.8 and 1.0 C/D ratios with 10 lifting repetitions. These results underscore the effectiveness of pseudo-haptic manipulation in those with high weight perception accuracy and confirm the appropriateness of C/D ratios. Moreover, a notable decrease in PSE weight with 100 repetitions compared to 10 at a 0.8 C/D ratio, despite expected fatigue-related increases, suggests a habituation effect, diminishing the pseudo-haptic impact over time (see Figure 12). This also indicates that the 100 lifting repetitions in the long-term condition effectively addressed the research question.
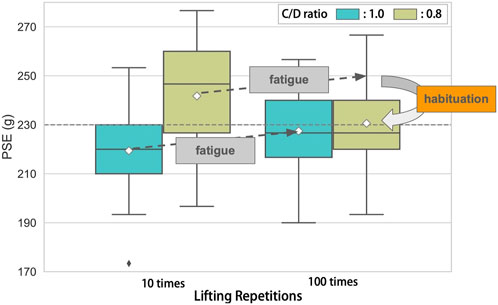
Figure 12. Our interpretation of the result of High Acc group (N = 17). Considering fatigue, the PSE should have been higher in the 100-repetition condition even with a C/D ratio of 0.8. However, the results show a significant decrease in PSE weight after 100 repetitions compared to 10 repetitions with a C/D ratio of 0.8. We consider this phenomenon to be a result of habituation to the pseudo-haptics.
The effect sizes (Cohen’s d) also indicate that the implementation and sample size design of this study were appropriate, with an effect size of 0.78 for the pseudo-haptics effect itself and 0.65 for the effect of prolonged exposure to pseudo-haptics (see Table 1).
5.5 Consideration of habituation causes
The observed habituation to pseudo-haptic effects could be due to the alignment of prior knowledge over repeated exposure (see Section 2). This alignment might recalibrate prior knowledge, reducing the effectiveness of pseudo-haptics.
6 Limitations and future work
This study demonstrates habituation under specific conditions (100 repetitions at a 0.8 C/D ratio). However, it has several limitations. Firstly, it does not establish whether similar effects would be observed with other sensory modalities or different C/D ratios. Secondly, the exact exposure duration at which habituation begins to occur remains unidentified. Lastly, the study focused on a specific demographic, which may limit the generalizability of the findings.
Future research should address these limitations by investigating a wider range of C/D ratios, exploring different sensory modalities, and identifying the precise onset of habituation. Additionally, to maintain engagement and enhance the longevity of the pseudo-haptic effect, future studies could explore incorporating varied activities or intermittent interventions. For instance, augmenting the pseudo-haptic experience with electrical stimulation (Kim et al., 2022) has shown promise in sustaining user attention and increasing the long-term efficacy of pseudo-haptics. Introducing periodic non-pseudo-haptic experiences may also prove effective. These strategies could enable the design of more compelling and effective VR experiences that utilize pseudo-haptic technology.
Additionally, while this study measured PSE before and after 10 and 100 repetitions, intermediate points (e.g., after 50 repetitions) were not tested. Incorporating more frequent PSE measurements could provide further insight into how habituation progresses. However, this may disrupt the flow of the Lifting Task and interfere with the accuracy of habituation measurements. Future studies should consider balancing these trade-offs to pinpoint when habituation begins.
7 Conclusion
This study set out to quantitatively assess the impact of habituation on weight perception manipulation using pseudo-haptics in VR environments. Through a carefully designed experimental setup, we examined the changes in perception before and after prolonged exposure to pseudo-haptic effects. Our findings reveal a significant decrease in the effectiveness of pseudo-haptic weight manipulations with repeated use, particularly among participants with high weight perception accuracy. This suggests a habituation to the pseudo-haptic effects, likely due to an alignment between participants’ expectations and their actual sensory experiences, which diminishes the initial perceptual discrepancy induced by pseudo-haptics.
These results provide essential insights for VR user experience design, highlighting the critical need to consider habituation when implementing pseudo-haptic weight manipulation. To counteract the effects of habituation, VR content developers should avoid lengthy, repetitive exposure to uniform stimuli and consider incorporating varied sensory experiences.
The implications of this study extend beyond weight perception, offering insights into the design and implementation of various pseudo-haptic interfaces in VR. By understanding the effects of habituation on pseudo-haptic feedback, we can work towards creating more robust and effective VR experiences that maintain their perceptual impact over time. This research contributes to the ongoing development of pseudo-haptic technology in VR, highlighting the importance of considering long-term user adaptation in future designs and applications.
Data availability statement
The raw data supporting the conclusions of this article will be made available by the authors, without undue reservation.
Ethics statement
The studies involving humans were approved by the Ethics Committee of the University of Tokyo. The studies were conducted in accordance with the local legislation and institutional requirements. The participants provided their written informed consent to participate in this study.
Author contributions
KI: Conceptualization, Data curation, Formal Analysis, Investigation, Methodology, Software, Validation, Visualization, Writing–original draft, Writing–review and editing, Resources. YB: Funding acquisition, Investigation, Methodology, Resources, Software, Validation, Visualization, Writing–original draft, Writing–review and editing, Data curation. S’iW: Funding acquisition, Methodology, Project administration, Resources, Supervision, Validation, Writing–original draft, Writing–review and editing.
Funding
The author(s) declare that financial support was received for the research, authorship, and/or publication of this article. This work was supported by JSPS KAKENHI Grant Numbers JP21H03478, JP23H04333.
Acknowledgments
We extend our gratitude to Koito for his expertise in the experimental setup and software, and to Yokota for her invaluable assistance in conducting the experiments.
Conflict of interest
The authors declare that the research was conducted in the absence of any commercial or financial relationships that could be construed as a potential conflict of interest.
Publisher’s note
All claims expressed in this article are solely those of the authors and do not necessarily represent those of their affiliated organizations, or those of the publisher, the editors and the reviewers. Any product that may be evaluated in this article, or claim that may be made by its manufacturer, is not guaranteed or endorsed by the publisher.
References
Azmandian, M., Hancock, M., Benko, H., Ofek, E., and Wilson, A. D. (2016). “Haptic retargeting: dynamic repurposing of passive haptics for enhanced virtual reality experiences,” in Proceedings of the 2016 chi conference on human factors in computing systems, USA, May 7 - 12, 2016, 1968–1979. doi:10.1145/2858036.2858226
Ban, Y., Narumi, T., Tanikawa, T., and Hirose, M. (2012). “Magic pot: interactive metamorphosis of the perceived shape,” in ACM SIGGRAPH 2012 posters (China: Association for Computing Machinery), 1.
Ban, Y., and Ujitoko, Y. (2023). Age and gender differences in the pseudo-haptic effect on computer mouse operation in a desktop environment. IEEE Trans. Vis. Comput. Graph. 30, 5566–5580. doi:10.1109/tvcg.2023.3295389
Charpentier, A. (1891). Experimental analysis of some elements of the feeling of weight (analyse experimentale de quelgues elements de la sensation de poids). Archives de Physiologie Normale Pathologique 3, 122–135. doi:10.1007/978-3-319-77770-2_13
Clarence, A., Knibbe, J., Cordeil, M., and Wybrow, M. (2022). “Investigating the effect of direction on the limits of haptic retargeting,” in 2022 IEEE international symposium on mixed and augmented reality (ISMAR) (Germany: IEEE), 612–621.
Coles, T. R., Meglan, D., and John, N. W. (2010). The role of haptics in medical training simulators: a survey of the state of the art. IEEE Trans. haptics 4, 51–66. doi:10.1109/toh.2010.19
Ernst, M. O., and Banks, M. S. (2002). Humans integrate visual and haptic information in a statistically optimal fashion. Nature 415, 429–433. doi:10.1038/415429a
Gatti, E., Caruso, G., Bordegoni, M., and Spence, C. (2013). “Can the feel of the haptic interaction modify a user’s emotional state?,” in 2013 World Haptics Conference (WHC), China, 14-17 April 2013 (IEEE), 247–252.
Gerini, L., Solari, F., and Chessa, M. (2023). “Passive haptic feedback for more realistic and efficient grasping movements in virtual environments,” in International conference on extended reality (Germany: Springer), 3–22.
Giachritsis, C., Barrio, J., Ferre, M., Wing, A., and Ortego, J. (2009). “Evaluation of weight perception during unimanual and bimanual manipulation of virtual objects,” in World Haptics 2009-Third Joint EuroHaptics conference and Symposium on Haptic Interfaces for Virtual Environment and Teleoperator Systems, China, 18-20 March 2009 (IEEE), 629–634.
Hannig, G., and Deml, B. (2010). “Efficient bimodal haptic weight actuation,” in Haptics: Generating and Perceiving Tangible Sensations: International Conference, EuroHaptics 2010, Amsterdam, July 8-10, 2010. Proceedings, Part I, China, July 8-10, 2010 (Springer), 3–10.
Harris, C. S. (1965). Perceptual adaptation to inverted, reversed, and displaced vision. Psychol. Rev. 72, 419–444. doi:10.1037/h0022616
Hirao, Y., and Kawai, T. (2018). Augmented cross-modality: translating the physiological responses, knowledge and impression to audio-visual information in virtual reality. Electron. Imaging 31, 060402-1–060402-8. doi:10.2352/j.imagingsci.technol.2018.62.6.060402
Hirao, Y., Takala, T. M., and Lécuyer, A. (2020). “Comparing motion-based versus controller-based pseudo-haptic weight sensations in vr,” in 2020 IEEE Conference on Virtual Reality and 3D User Interfaces Abstracts and Workshops (VRW), USA, 22-26 March 2020 (IEEE), 305–310.
Hoffman, H. G. (1998). “Physically touching virtual objects using tactile augmentation enhances the realism of virtual environments,” in Proceedings. IEEE 1998 virtual reality annual international symposium (cat. No. 98CB36180) (China: IEEE), 59–63.
Jin, S.-A. A. (2011). The impact of 3d virtual haptics in marketing. Psychol. and Mark. 28, 240–255. doi:10.1002/mar.20390
Jones, L. F., and Burgess, P. R. (1997). Perceptions of effort and heaviness during fatigue and during the size-weight illusion. Somatosens. and Mot. Res. 14, 189–202. doi:10.1080/08990229771051
Kang, H., Lee, G., and Han, J. (2019). “Visual manipulation for underwater drag force perception in immersive virtual environments,” in 2019 IEEE Conference on Virtual Reality and 3D User Interfaces (VR), China, 23-27 March 2019 (IEEE), 38–46.
Kashihara, A., and Shiota, G. (2014). “Knowledge construction with pseudo-haptics,” in Intelligent Tutoring Systems: 12th International Conference, ITS 2014, Honolulu, HI, USA, June 5-9, 2014. Proceedings 12, USA, June 5-9, 2014 (Springer), 61–68.
Kim, J., Kim, S., and Lee, J. (2022). The effect of multisensory pseudo-haptic feedback on perception of virtual weight. IEEE Access 10, 5129–5140. doi:10.1109/access.2022.3140438
Lécuyer, A. (2009). Simulating haptic feedback using vision: a survey of research and applications of pseudo-haptic feedback. Presence Teleoperators Virtual Environ. 18, 39–53. doi:10.1162/pres.18.1.39
Lim, W. N., Yap, K. M., Lee, Y., Wee, C., and Yen, C. C. (2021). A systematic review of weight perception in virtual reality: techniques, challenges, and road ahead. IEEE Access 9, 163253–163283. doi:10.1109/access.2021.3131525
Liu, Y., Ishikawa, Y., Fukuma, Y., and Nakagawa, Y. (2023). “Augmented haptic vr experience combining two weight-shifting versatile controllers,” in ACM SIGGRAPH 2023 emerging technologies China, (Association for Computing Machinery), 1–2.
Minamizawa, K., Kajimoto, H., Kawakami, N., and Tachi, S. (2007). “A wearable haptic display to present the gravity sensation-preliminary observations and device design,” in Second Joint EuroHaptics Conference and Symposium on Haptic Interfaces for Virtual Environment and Teleoperator Systems (WHC’07), Japan, 22-24 March 2007 (IEEE), 133–138.
Nomoto, A., Ban, Y., Narumi, T., Tanikawa, T., and Hirose, M. (2016). “Supporting precise manual-handling task using visuo-haptic interaction,” in Proceedings of the 7th Augmented Human International Conference 2016, USA, 25 February 2016, 1–8.
Pusch, A., and Lécuyer, A. (2011). “Pseudo-haptics: from the theoretical foundations to practical system design guidelines,” in Proceedings of the 13th international conference on multimodal interfaces, Spain, November 14 - 18, 2011, 57–64. doi:10.1145/2070481.2070494
Pusch, A., Martin, O., and Coquillart, S. (2008). “Hemp-hand-displacement-based pseudo-haptics: a study of a force field application,” in 2008 IEEE symposium on 3D user interfaces (Germany: IEEE), 59–66.
Ramírez, A. G. R., Luna, F. J. G., Villegas, O. O. V., and Nandayapa, M. (2018). Applications of haptic systems in virtual environments: a brief. Adv. Top. Comput. Vis. Control Robotics Mechatronics, 349.
Samad, M., Gatti, E., Hermes, A., Benko, H., and Parise, C. (2019). “Pseudo-haptic weight: changing the perceived weight of virtual objects by manipulating control-display ratio,” in Proceedings of the 2019 CHI Conference on Human Factors in Computing Systems (ACM), UK, May 4 - 9, 2019, 320.
Sato, M., and Kikuchi, Y. (1967). An analysis of subjective weight sensation. J. Anthropol. Soc. Nippon 75, 147–150. doi:10.1537/ase1911.75.147
Shiller, D. M., Mitsuya, T., and Max, L. (2020). Exposure to auditory feedback delay while speaking induces perceptual habituation but does not mitigate the disruptive effect of delay on speech auditory-motor learning. Neuroscience 446, 213–224. doi:10.1016/j.neuroscience.2020.07.041
Stratton, G. M. (1896). Some preliminary experiments on vision without inversion of the retinal image. Psychol. Rev. 3, 611–617. doi:10.1037/h0072918
Ujitoko, Y., and Ban, Y. (2021). Survey of pseudo-haptics: haptic feedback design and application proposals. IEEE Trans. Haptics 14, 699–711. doi:10.1109/toh.2021.3077619
Ujitoko, Y., Ban, Y., and Hirota, K. (2019). “Presenting static friction sensation at stick-slip transition using pseudo-haptic effect,” in 2019 IEEE World Haptics Conference (WHC), China, 9-12 July 2019 (IEEE), 181–186.
Walker, P., and Walker, L. (2012). Size–brightness correspondence: crosstalk and congruity among dimensions of connotative meaning. Atten. Percept. and Psychophys. 74, 1226–1240. doi:10.3758/s13414-012-0297-9
Keywords: pseudo-haptics, cross-modal effect, tactile sensation, habituation, virtual reality
Citation: Ito K, Ban Y and Warisawa S (2024) Influence of habituation on pseudo-haptic weight perception of virtual objects. Front. Virtual Real. 5:1442829. doi: 10.3389/frvir.2024.1442829
Received: 03 June 2024; Accepted: 31 October 2024;
Published: 12 November 2024.
Edited by:
Peter Berkelman, University of Hawaii, United StatesCopyright © 2024 Ito, Ban and Warisawa. This is an open-access article distributed under the terms of the Creative Commons Attribution License (CC BY). The use, distribution or reproduction in other forums is permitted, provided the original author(s) and the copyright owner(s) are credited and that the original publication in this journal is cited, in accordance with accepted academic practice. No use, distribution or reproduction is permitted which does not comply with these terms.
*Correspondence: Kenta Ito, itokenta@lelab.t.u-tokyo.ac.jp