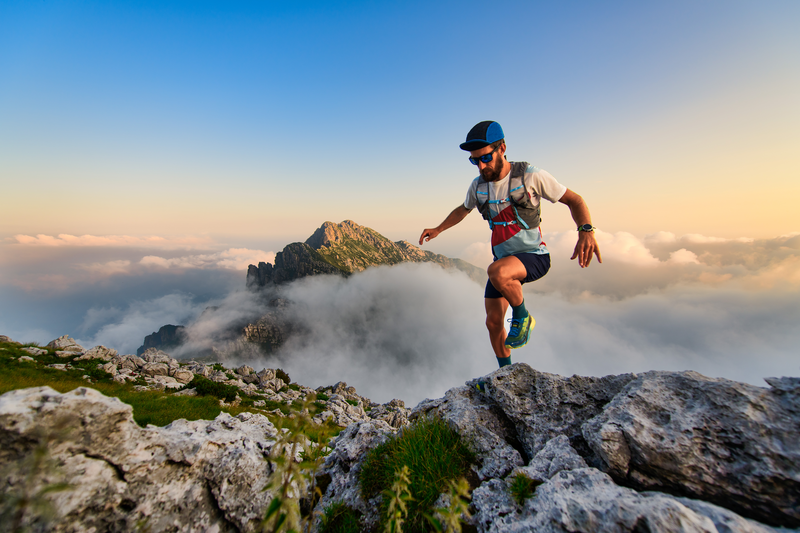
94% of researchers rate our articles as excellent or good
Learn more about the work of our research integrity team to safeguard the quality of each article we publish.
Find out more
ORIGINAL RESEARCH article
Front. Virtual Real. , 11 January 2024
Sec. Virtual Reality and Human Behaviour
Volume 4 - 2023 | https://doi.org/10.3389/frvir.2023.1302720
This article is part of the Research Topic Virtual Agents in Virtual Reality: Design and Implications for VR Users View all 5 articles
Stress-inducing virtual reality (VR) systems have various applications in research, ranging from training to therapy to the observation of biological stress responses. Stress in VR can be evoked through environmental, cognitive, and social stimuli. Although various VR tasks can induce an autonomic nervous system (ANS) stress response, hypothalamic-pituitary-adrenal (HPA) axis stress responses have only been confirmed in VR for the virtual Trier social stress test (V-TSST). Understanding the impact of a wider variety of tasks on HPA-axis stress response could lead to the development of more effective stress relief measures and treatments. This study aims to clarify whether a virtual communication simulation, using a static procedure with a predetermined dialog, can evoke an HPA-axis stress response. Employing a virtual customer service system, we varied the intensity of the presented stress by changing the tone and gestures of the virtual customer. The findings confirm that HPA-axis stress responses can be elicited by such static virtual customer service training systems, and the stress responses can be adjusted by altering the avatar’s attitude. These findings suggest potential applications in research for observing human physiological responses to stress and development of stress reduction strategies, thereby affirming the effectiveness of VR in communication training.
Virtual reality (VR) systems that induce stress in users have been extensively developed for research purposes, such as training, mental therapy, biological response monitoring, and stress reduction for individuals under stressful conditions. VR can induce primarily three types of stress in users: environmental stress such as heights that cause anxiety and fear; cognitive stress such as mathematical tasks; and social stress such as those arising from communication and domestic violence simulations (van Dammen et al., 2022). Virtual environments (VEs) can induce stress through exposure to fear-inducing stimuli, such as heights, darkness, and spiders (Toet et al., 2009; Notzon et al., 2015; Chessa et al., 2019), and by presenting environments that cause anxiety, such as being trapped in small spaces or disarming bombs (Lee and Jung, 2020). In terms of cognitive stressors, existing cognitive tasks such as mental calculation and n-back tests have been studied and incorporated into VR (Tremmel et al., 2019). Regarding social stressors, research has been conducted on the virtual Trier social stress test (V-TSST) and virtual experiences of domestic violence (DV) (Yao et al., 2016; Gonzalez-Liencres et al., 2020).
To evaluate whether VR stress tasks induce stress in users, both subjective evaluations using questionnaires such as the State-trait anxiety inventory (STAI) and physiological stress biomarkers that include heart rate variability (HRV), galvanic skin response (GSR), heart rate (HR), pupil diameter, salivary alpha-amylase (sAA), and cortisol, have been used. These biomarkers reflect the significant responses from the hypothalamic-pituitary-adrenal (HPA) axis and the autonomic nervous system (ANS). The ANS stress response is a rapid reaction that can occur within seconds to minutes of exposure to a stressor and is mediated primarily by the sympathetic nervous system, resulting in physiological changes like increased heart rate and blood pressure. The HPA axis stress response is slower than that of ANS. It involves the release of hormones, most notably cortisol, which has widespread effects in the body including suppressing inflammation, increasing energy supply, and influencing memory formation.
Differences in the stressors that trigger each stress response have been reported (Thayer and Lane, 2000; Kudielka et al., 2009). For the aforementioned VR stressors, a wide variety of tasks have been developed that induce anxiety or fear through the VR experience, including cognitive tasks within VR that have been shown to induce autonomic stress responses. Although it has been confirmed through various studies that HPA-axis stress responses occur due to social challenges (DeVries et al., 2003; Schwabe et al., 2008), in the context of VR tasks, these responses have only been evidenced in a specific task called V-TSST.
Contemporary stressors often have a social-evaluative nature, and chronic maladaptive responses to these stressors can lead to deterioration in physical and psychological health (Schneiderman et al., 2005). Among these, changes in HPA-axis regulation in response to such stressors are closely correlated with the onset and progression of various diseases (Kudielka and Wüst, 2010). Research has been conducted to characterize an individual’s HPA-axis activity and reactivity patterns to psychosocial stress. In advancing such research, it is important to experimentally induce stable HPA-axis stress responses, using stress tasks such as the Socially evaluated cold pressor test (SECPT) and the Trier social stress test (Schwabe et al., 2008; Birkett, 2011). However, these tasks often involve unusual situations, such as putting your hands in ice water or performing a speech after a calculation task. Using VR can allow for the replication of more realistic social situations, such as handling customer complaints, enabling more accurate assessment of stress impacts encountered in real life, and aiding in the design of measures to counteract them (Powers et al., 2013).
Social challenges in VR are being applied to interpersonal training such as customer service, and various systems are being researched and implemented in society. Conversational skills are one of the most important skills in modern society and are widely used in many professions, including management and commercial scenarios. The training cycle for managers and sales representatives often includes role-playing sessions with instructors; however, such training involves significant human and financial costs (Taupiac et al., 2019). Therefore, the implementation of interpersonal training using VR has been proposed, and various VR systems have been suggested for interpersonal skill training in situations such as managerial tasks, sales to customers, and doctor-patient interactions (Pan et al., 2016; Taupiac et al., 2019). If these VR training systems can be proven to induce HPA axis stress responses, they could be used as an indicator of training effectiveness, leading to the development of more effective social skill training systems.
Considering these findings, our study aims to clarify whether virtual communication simulations can evoke HPA-axis stress. Specifically, we examined the simulations implemented by a “static procedure,” with predetermined flow of conversation between the dialog avatar and the participant. This type of communication simulation with scripted dialog has already been utilized in areas such as customer service training, hereinafter referred to as CST, making further clarification of evoking HPA-axis stress, a valuable objective. If an HPA-axis stress response can indeed be triggered, its use in stress experiments could enhance standardization, with identical content for each participant, possibly reducing interference between researchers and participants (van Dammen et al., 2022).
In addition, this study also aims to elucidate how the simulated communication load alters the stress response. Adjusting the intensity of stress load according to objectives and target individuals is crucial when inducing stress responses through a VR system. In the context of training or mental therapy, excessive stress could potentially cause severe damage to the trainee or patient. Similarly, when applying stress for research purposes, the level of the stress applied to the participants of the experiment should be commensurate with the purpose.
To realize the research objectives, a specific VR experiment involving a CST was designed using the framework provided by Tanikawa et al. (2021), thereby adjusting it for the specific research needs. In the simulated scenario, participants are in the position of handling a complaint from a customer who is upset about a delayed flight due to airport mishaps. The dialog between the participant and the virtual customer is predetermined, making this a scripted dialog virtual communication. We modified this training system by changing only the tone of voice and gestures of the virtual customer while keeping the dialog content the same, to manipulate the intensity of the presented stress. This experiment allowed us to examine how differences in the presented stress intensity affect the HPA-axis stress response, specifically cortisol concentration in the saliva.
The renowned endocrinologist Hans Selye explored the physiological mechanisms underlying non-specific health problems, and borrowing from the field of engineering, defined stress as “the state manifested by a specific syndrome which consists of all the non-specifically induced changes within a biological system” (Selye, 1956). Factors that cause stress responses include environmental factors evoking fear or anxiety, social factors such as human relationships, interpersonal evaluations, feelings of loneliness, and physiological factors such as illness, injury, and lack of sleep (van Dammen et al., 2022). Three primary stressor elements have been identified by researchers to help distinguish the differences in stressor effects on individuals. These include the elements of social evaluative threat, defined as the fear of being negatively judged by others; uncontrollability, defined as the inability to change or alter the course of events; and unpredictability of future events.
Stress responses can be broadly categorized into as originating from the ANS and HPA-axis responses (Thayer and Lane, 2000; Kudielka et al., 2009). The ANS stress response occurs within seconds to minutes of stressor exposure, causing physiological changes such as an increase in heart rate, blood pressure, and breathing rate. The ANS stress response is primarily mediated by the sympathetic nervous system, and its activation triggers the release of adrenaline and noradrenaline. The HPA-axis stress response, on the other hand, is relatively slower, occurring within tens of minutes after exposure to stress. The activation of the HPA axis begins with the hypothalamus releasing corticotropin-releasing hormone (CRH). CRH acts on the pituitary gland, promoting the release of adrenocorticotropic hormone (ACTH). ACTH reaches the adrenal cortex through the bloodstream, where it stimulates the production and release of the stress hormone cortisol. Cortisol has widespread effects throughout the body, suppressing inflammatory responses, increasing energy supply, and influencing memory formation, among others. The response of the HPA axis provides a longer-term strategy for dealing with more persistent stress.
Differences have been reported in stressors triggering a stress-related response. For example, cognitive tasks, such as n-back and the Stroop test, as well as minor stressors in daily life, tend to induce ANS stress responses. However, social and physiological stressors have been reported to be more likely to induce HPA-axis stress responses. Autonomic measures are thought to react to physical or mental exertion, whereas endocrine measures are particularly reactive to social information processing (Bertsch et al., 2011; Seery, 2011). Although inducing an ANS stress response is easy, experimentally inducing an HPA stress response is difficult (Dickerson and Kemeny, 2004). Consequently, reports on stressors that cause an HPA-axis response are limited compared to those causing ANS stress responses. Experimental methods that have been reported to elicit HPA axis stress responses include the socially evaluated cold pressor test referred to as SECPT and the Trier social stress test referred to as TSST.
The SECPT stressor involves immersing the hand of a participant in ice water for a certain period while capturing on film their suffering. In previous studies, cortisol levels in saliva have been shown to increase before and after SECPT (Schwabe et al., 2008). SECPT is feasible to implement and does not require specially trained experimenters, provided ice water is available. Another advantage of SECPT is that the intensity of the stressor can be adjusted by modifying the temperature of the ice water or the duration of hand immersion. However, the situation of this task differs greatly from the stressful situations experienced in real-life society.
The TSST involves a process in which participants are allowed a certain preparation time, followed by a 5-min speech and a 5-min calculation task, while recording their pressured state during the process (Birkett, 2011), and the experimenters are required to ensure a strict atmosphere. In previous studies cortisol levels in the saliva were shown to increase significantly before and after the speech and calculation tasks, indicating that TSST is a stressor that triggers endocrine stress responses (Schommer et al., 2003; Wüst et al., 2005; Yao et al., 2016). Although TSST does not carry the risk of physical harm, adjusting the intensity of the stressor is difficult because the timing of adjusting the intensity is restricted primarily to the period the participants receive instructions from the experimenters. The interventions provided by the experimenters during TSST are limited to speech-related instructions and for highlighting mistakes during the calculation task if the participants finish before the allotted time. Consequently, there is still room for more research on stressors that trigger HPA-axis stress responses, as the number of reported stressors is limited.
Virtual reality stress tasks have attracted attention as a new methodology for stress presentation because they can reproduce stressful environments that are impossible or difficult to realize in the real world. VR stresses can be classified into those that cause anxiety or fear (high-altitude or tragedy), cognitive stress (computing task), and social stress (domestic violence).
Examples of VEs that induce anxiety or fear include scenarios of escaping from a fire or firefighting (Breuninger et al., 2017; Narciso et al., 2020), horror experiences (Cebeci et al., 2019; Lemmens et al., 2022), exposure to high-altitude (Diemer et al., 2016; Chessa et al., 2019), accidents and related rescue of people (Dibbets, 2020), and medical emergency training simulators in noisy environments (Viciana-Abad et al., 2004). Examples of cognitive stressors in VR include time-pressure tasks (Gradl et al., 2019), n-back (Luong et al., 2019), and real-life cognitive tasks transformed into VR, sometimes combined with virtual stressors, such as the virtual high-altitude penalty for wrong answers in subtraction tasks used by Finseth et al. (Finseth et al., 2018). Examples of social stressors in VR include public-speaking scenarios (Felnhofer et al., 2014; Kishimoto and Ding, 2019), virtual blind dates, job interviews (Hartanto et al., 2014), and V-TSST (Jönsson et al., 2010; Kothgassner et al., 2021).
In this study, we selected CST as a stressor using VR for the reasons stated in the Introduction section and examined whether it would cause an HPA stress response through a between-subject experimental design. Specifically, we used a modified version of the customer-service VR system developed by Tanikawa et al. (2021). In this experimental system, the trainer interacted with the customer’s avatar at a virtual airport service counter (Figure 1). In Tanikawa’s system, the conversation route can branch depending on the response of the trainer and the training difficulty can be adjusted based on the emotional state of the trainer, which is calculated from biosignal data. To ensure that the participants perform the training task under the same conditions and experience the same conversation, we omitted the conversation-branching function of Tanikawa’s system in this experiment. The conversation involved customers complaining about missing their connecting flight because of a delay in scheduled departure, resulting in a long wait at the airport for the next flight. In this system, participants in the experiment wore a head mounted display (HMD) and were stranded at the airport within a virtual environment. The flow of the conversation was predetermined; the participant read the words displayed on the screen, and the timing of the customer avatar’s speech was controlled by the experimenter according to the participant’s speech.
FIGURE 1. Customer service training (CST) VR. A virtual customer stands in front of a virtual airport customer counter, complaining about airplane delay. Participants listen to the customer and read the lines at the center to respond. Reproduced with permission.
The effect of altering the load during the simulated conversation on HPA stress response was further examined. In this system, the level of conversation load can be altered by changing the gestures and tone of the customer avatar, while keeping the conversation content the same. To apply this feature to the experiment, we divided the participants into two groups with one group interacting with a ‘calm and kind, yet slightly dissatisfied’ customer avatar and the other interacting with an ‘angry and rude’ customer avatar. The HPA stress responses, specifically changes in cortisol levels in saliva, of the two groups were compared. We did not perform within-participant comparisons of stressor intensity because performing this method twice per person could result in the participants becoming accustomed to the stress stimulus, thus preventing a second occurrence of HPA stress responses. Previous studies have shown that the HPA-axis stress response is significantly reduced in the second occurrence of the TSST (Kirschbaum et al., 1995; Schommer et al., 2003; Wüst et al., 2005). The motion and sound data of the avatars were derived from measurements recorded in previous research (Tanikawa et al., 2021), which involved the performance of a professional actor using a motion-capture system.
Figure 2 shows the experimental procedure. The stressor protocol was based on the guidelines of TSST, which has been commonly used in previous studies as a stressor to induce HPA-axis stress responses (Birkett, 2011). As salivary cortisol concentration has been reported to increase after a short period under stress, in experimenting with this aspect, we observed that salivary cortisol concentration changed immediately after the participants experienced VR training and within a short period, compared to the state immediately before they experienced VR training. The detailed protocol is described below.
As part of the pre-experiment instructions for participants, they were instructed to avoid eating for 2 hours before the start of the experiment and to refrain from consuming caffeine or other stimulants, such as coffee during this period. They were also advised to avoid intense exercise during this period. These instructions were provided to the participants at the time of recruitment, and adherence to these instructions was confirmed through a questionnaire before the start of the experiment. It was verified that all participants complied with these instructions prior to participation.
Of the two rooms prepared for the experiment, as in TSST, one was for the VR CST and the other served as a relaxation room for waiting. These two rooms were provided to separate the stress and relaxation states. The participants were informed of the false experimental purpose and methodology similar to the TSST protocol. This process is called “deception” in psychological experiments. The participants were informed that the experiment was intended to evaluate customer service attitudes and that their customer service behaviors during the experiment would be recorded and evaluated later. In providing this false explanation, the aim was to increase participants’ perception of the seriousness of the VR CST and provide them with a level of tension equivalent to that of actual VR training. After the explanation, we obtained their consent to participate and attached an electrocardiogram (ECG) to measure their biological information (PLUX biosignals). The data were recorded at 1,000 Hz.
After the sensors were attached, the participants were guided to a relaxation room and instructed to wait for 45 min. During the waiting period, participants were informed that they could leave the relaxation room to use the restroom and read a magazine. They were also instructed to avoid vigorous exercise and remain calm and relaxed while waiting.
After 45 min, the experimenter entered the relaxation room to collect saliva samples and measure cortisol levels before the VR CST. Saliva was collected using swabs (SAL-5001.02-50; SAL). Participants were instructed to insert the swab into the back of their tongue for 1 minute, after which the swab was collected in a storage tube (SAL-5001.05-50; SAL). The collected swabs were frozen at −80°C within 1 hour.
To establish a baseline for the biological information before inducing stress, the participants were instructed to rest for 5 min in the sitting position. Once the 5-min rest period began, the experimenter immediately left the relaxation room. During the rest period, the participants were instructed to look at the crossed gaze points displayed on the monitor in the relaxation room (Figure 3). To analyze the autonomic stress response, which is discussed in Section 3.3, the participants’ electrocardiogram data were measured during this time as “Pre-stress” data.
After the 5-min rest period, the participants were led to the experiment room to experience VR CST. The participants wore an HMD (Vive Pro 2, HTC) to clearly see the VR images, and the controllers were attached to both hands. The customer avatar’s voice was presented through headphones attached to the HMD. Participants were given brief instructions on how to use the VR equipment. The positions of the controllers were reflected in VE as the avatar hands of the participant. To help participants become accustomed to VE and focus on customer service tasks, they were instructed to wait for 10 min at the VR airport displayed during the task and were shown the lines they were expected to say during the customer service task (Figure 4).
FIGURE 4. Participant waiting in the virtual environment preparing the lines. Reproduced with permission.
After 10 min, the participants were again provided with an overview of the customer service content prior to initiating the customer service response VR experience. The participants were informed that although the dialog was predetermined, their tone, intonation, speaking speed, and body language would be evaluated as part of the assessment.
The VR customer service task took approximately 5 min. During the experiment, the high-intensity group was presented with a virtual customer who complained severely, glared tightly at the participants, slam the desk, and talked rigorously. In contrast, the low-intensity group was presented with a virtual customer who complained, but had a calm attitude, did not glare at the participants, spoke softly, and made small gestures (Figure 5). The differences in avatars between conditions were shown in Table 1.
FIGURE 5. On the left, virtual customer with high-intensity stressor, and on the right, low-intensity stressor. In the high-intensity stressor condition, the virtual customer glares tightly at the participants, slams the desk, and talks rigorously. In the low-intensity stressor condition, the customer does not glare at the participants, instead speaking softly with small gestures. Reproduced with permission.
TABLE 1. Comparison of voice and gesture characteristics between high-intensity and low-intensity groups.
Upon completion of examination, saliva was collected immediately and labeled as “Post-stress.” The method of saliva collection was the same as that described above.
The participants were instructed to move to the relaxation room and wait for 40 min, using the same method as for the initial waiting period. They were also asked to provide saliva samples at the 20- and 40-min marks while waiting, thereby labeling them as “Recovery1” and “Recovery2,” respectively. Previous studies confirmed that the HPA-axis stress response is stronger approximately 20 min after the stress load than immediately after (Petrowski et al., 2012; Eisenlohr-Moul et al., 2018; Cettolin et al., 2020). Therefore, measurements were conducted at two time points immediately after the task, to confirm this tendency. The experimenters did not enter the relaxation room except during saliva collection.
After the 40-min waiting period, the participants were informed that the experiment was over. They were then asked whether they had experienced any stress during the experiment or noticed deception regarding the purpose of the experiment, which had been previously explained. Subsequently, participants were informed that they would not be screened for customer service attitudes.
The cortisol levels in the saliva samples collected during the experiment (Kirschbaum and Hellhammer, 1989) were measured to confirm the occurrence of an HPA-axis stress response, analyzing the collected samples using the enzyme-linked immunosorbent assay (ELISA) method (Shimada et al., 1995). The cortisol levels measured in the saliva, were examined to determine whether “Post-stress,” “Recovery1,” and “Recovery2” cortisol levels significantly increased compared to the levels at “Pre-stress.” Given the considerable variability in baseline salivary cortisol levels among participants (“Pre-stress” levels), following the methodology of prior research (Figueiro and Rea, 2012), “Post-stress,” “Recovery1,” and “Recovery2” cortisol levels were normalized by “Pre-stress” levels for each participant.
Statistical analysis was conducted to examine saliva measurement timing and VR training intensity, with saliva measurement timing as a within-participant factor and VR training intensity as a between-participant factor at a significance level of p = 0.05. Specifically, the normality of the data was first confirmed using the Shapiro-Wilk test. Subsequently, a mixed-design two-way ANOVA was conducted to analyze the data. For post hoc tests, Dunnett’s test was used within each VR training intensity group to examine whether the “Post-stress,” “Recovery1,” and “Recovery2” salivary cortisol levels significantly differed from those at “Pre-stress.”
Additionally, the effect sizes (d and ESsg) were calculated to compare the results of previous studies on TSST and V-TSST with ours. ESsg has been used in meta-analyses of multiple studies related to V-TSST (Helminen et al., 2019). Please refer to Supplemental Material for details on the ESsg calculation formula.
To avoid the potential influence of physiological indicators in answering the questionnaire, a subjective evaluation of stress levels was not conducted during or after the task. Instead, participants were asked whether they experienced task-related stress 40 min after their saliva was collected. Participants rated the perceived stress on a scale of 1–5 (1: no stress, 5: high stress). Additionally, to confirm whether stress responses of the autonomic nervous system occurred during the task, we investigated the change in electrocardiographic indicators during the task in relation to the pre-stress “Calm” phase. Using the low frequency/high frequency (LF/HF) ratio, we derived a common index from ECG data, as an indicator of stress response in the ANS. The LF/HF ratio is considered an indicator of sympathetic nervous system activity, which increases under stress. Previous studies have already revealed the occurrence of subjective and autonomic stress responses during communication with avatars (van Dammen et al., 2022). Therefore, clarifying these aspects was not within the scope of this study. A survey and ECG measurements were conducted to confirm that the experiment was conducted without any issues.
The study recruited participants who were between 20 and 30 years old, without any prior knowledge of the experiment, and capable of using Japanese at a conversational level or higher. Besides, as noted above, participants were subjected to restrictions regarding eating and exercising before the experiment, and it was planned not to conduct the experiment with participants who did not meet these criteria. As it turned out, no one violated these restrictions.
The sample size estimation was conducted using the statsmodels package in Python. The primary effect we wanted to confirm in this experiment was whether cortisol levels in the High intensity condition significantly increased at the Recover1 timing compared to the pre-stress period. Therefore, we recruited four participants for a preliminary validation and calculated an effect size of 0.96. Based on this, with a significance level of 0.05 and a power of 0.7, the estimated sample size for a one-sided test was 11. Consequently, we gathered 11 participants for each condition for the experiment. However, during post-experiment analysis, we identified samples from which cortisol levels and biometric data were not obtained, leading to their exclusion. As a result, total of 19 participants were recruited.
To ensure that there was no significant difference in communication skills between the high- and low-intensity groups, participants were initially asked to rate their subjective communication abilities on a 7-point Likert scale (1: very poor, 7: very good). Based on their responses, participants were then assigned to one of the two condition groups so that the average scores of subjective communication abilities would be equivalent in both groups. As a result, nine participants (eight males and one female) were placed in the high-intensity group, while 10 participants (nine males and one female) were placed in the low-intensity group. In addition, we considered that the magnitude of the stress response to the system may be dependent on the customer service experience. Considering that a difference in the number of participants with customer service experience between the high and low intensity groups could affect the results, recruitment was limited to include participants with no prior customer service experience. The experimental plan was approved by the Research Ethics Committee of the University of Tokyo (No.22-72), and written informed consent was obtained from all participants.
In Figure 6, the normalized values for each participant’s data are separately plotted for the high- and low-intensity groups, for avatars with severe and relatively moderate attitudes, respectively. In the data represented in this figure, the number of non-responders, i.e., participants who exhibited little to no HPA-axis stress response to the stressor, were two out of nine in the high difficulty and six out of ten in the low difficulty group, with the non-responders determined by the number of participants whose cortisol levels did not exceed 1.1 times their pre-stress levels.
FIGURE 6. Time course of normalized salivary cortisol levels for high- and low-intensity VR training groups. Cortisol concentrations were normalized to pre-stress levels. Error bars represent 95% confidence intervals.
As a first step in statistical analysis, the normality for each condition was checked using the Shapiro-Wilk test. Because no significant difference from the normal distribution was detected, a mixed-design two-way ANOVA was conducted. The results demonstrated the main effects of saliva measurement timing (F3,51 = 3.72, p < 0.05) and VR training intensity (F1,17 = 5.03, p < 0.05) and found a significant interaction between saliva measurement timing and VR training intensity (F3,51 = 3.71, p < 0.05).
As a post hoc test, Dunnett’s test revealed that the “Post-stress” and “Recovery1” values in the high-intensity group were significantly higher than that of “Pre-stress,” with p < 0.05 (Table 2a). In the high-intensity group, no significant difference was observed between “Recovery2” and “Pre-stress,” and in the low-intensity group, no significant differences were found between any of the conditions (p > 0.05).
TABLE 2. Results of Dunnett’s test and unpaired t-test as post hoc tests, indicated by p values and effect sizes (cohen’s d and ESsg).
In addition, salivary cortisol levels at each measurement timing were compared between the high- and low-intensity groups. The results of the unpaired t-test showed that the high-intensity group had significantly higher salivary cortisol levels than the low-intensity group for “Recovery1” and “Recovery2”, with p < 0.05, and there was no significant difference between the two conditions for “Post-stress” (Table 2b). Table 2 also presents Cohen’s d and the within-subject effect sizes as the standardized mean gain effect size (referred to as ESsg). These values suggest that the results with significant differences in the simple main effect of saliva measurement timing have a large effect size, and the results with significant differences in the simple main effect of VR training intensity have a moderate effect size.
The ANS stress response during the VR training task was analyzed to determine whether stress was induced. Figure 7 shows the LF/HF value during the stress task for each participant, normalized by the LF/HF value of “Pre-stress.” Values larger than the baseline value of 1.0 (dashed line) indicate that the LF/HF increases during the task compared to the resting state before the task, suggesting that a stronger ANS stress response is induced by the task. For both high- and low-intensity groups, statistical analyses were conducted to determine whether the normalized LF/HF value was greater than the baseline value. The Shapiro-Wilk test was conducted to check the normality of the data, and the results confirmed that the data did not follow a normal distribution. Therefore, a Wilcoxon signed-rank test was used, revealing that the normalized LF/HF value in the high-intensity group was significantly higher than the baseline value of 1.0 (p < 0.01). However, no significant difference was found between the normalized LF/HF value and the baseline criterion in the low-intensity group (p = 0.39).
FIGURE 7. Box plots of LF/HF values during the stress task, normalized by the “Pre-stress” values for high- and low-intensity VR training groups. The box represents the interquartile range (IQR), with the median indicated by the horizontal line inside the box. The mean is represented by an ‘X’ marker. A dashed line at 1.0 indicates the baseline level, representing each participant’s normalized pre-stress value.
In the post-experiment questionnaire, participants rated the stress they felt on a scale of 1–5 (1: no stress, 5: high stress). The distribution of responses for each condition is shown in Figure 8. In the high-intensity group, six out of nine participants reported feeling stressed (score of 4 or higher) during the task, whereas in the low-intensity group, three out of 10 participants reported feeling stressed. The Wilcoxon rank-sum test revealed a significant difference between the high- and low-intensity groups (p = 0.031), and a large effect size was observed (d = 0.91).
In the open-ended response section conducted at the end of the experiment, the participants indicated the reasons for being stressed or not stressed by the task. In the high-difficulty group, five people felt stressed because they felt scolded by the avatar’s actions, such as leaning on or slamming the desk, and four people felt stressed by the tone of the avatar’s voice. In the low-difficulty group, two people felt stressed by the avatar’s attitude, and one person felt stressed by the tone of the voice. Among the reasons for not feeling stressed, in the low-difficulty group, three people indicated not receiving any complaints, and one person in each condition mentioned the low realism of the VR as the reason for not feeling stressed.
In the high-intensity group, salivary cortisol concentrations were significantly higher at 20 and 40 min after completing the high-intensity VR training task than before starting it, indicating that HPA-axis stress was induced by the VR training task. The number of non-responders in each condition also suggests that the intensities of stress responses were perceived differently between the two conditions. Consistent with the results of previous studies, the salivary cortisol concentration was highest for Recovery1, which was 20 min after the end of the VR training task, in the high-intensity group (Petrowski et al., 2012; Eisenlohr-Moul et al., 2018; Cettolin et al., 2020). The standardized mean gain effect size (ESsg) for the increase in cortisol concentration in transitioning from Pre-stress to Recovery1 in the high-intensity group was 0.89, which is larger than the average effect size of 0.65 (SE = 0.0069) observed in previous studies on HPA-axis stress response in the V-TSST for the increase in cortisol concentration from baseline to peak measurement (Helminen et al., 2019). This result suggests that VR communication simulations may elicit HPA-axis stress responses equivalent to or greater than those of V-TSST. We believe the reason for the results obtained in this study lies in the differences between our experimental system and the interaction between avatars and participants in V-TSST. In V-TSST, avatars mostly react to participants’ speeches, whereas in our system, avatars actively complain to the participants, incorporating exaggerated gestures such as raising their voices, banging on desks, and making large hand movements. Responses from open-ended questions also indicated that the attitude and tone of the avatars were stressful, which differs from the existing V-TSST. This aspect likely enhanced the effectiveness of our system as a stressor. This interpretation was supported by the open-ended responses after the experimental task, indicating that both the tone and gestures of the avatar were stressful to the participants.
In addition to the comparison with V-TSST results, we also compared with the traditional TSST results. A recent meta-analysis evaluated 186 studies to determine the effectiveness of the traditional TSST and indicated that this stress task was effective in eliciting a cortisol response with a large effect size (d = 0.925 (Goodman et al., 2017)). By contrast, existing meta-reviews suggest that although V-TSST is effective as a stressor, it may not achieve the same effectiveness as traditional TSST in terms of eliciting cortisol responses. However, in our experiment, the VR training task in the high-intensity group was effective in eliciting a cortisol response with a large effect size (d = 0.94, ESsg = 0.89). This suggests that the aggressive attitude of the interactive avatar in the communication simulation may potentially elicit stress-load effects equivalent to those of TSST.
Previous studies measuring HPA-axis stress responses in VR tasks that induce social stress have only been confirmed for the V-TSST task, where participants generate their own responses in speech. Such responses have not been confirmed in static communication simulations, where the conversation content is predefined or scripted. The results obtained in this study suggest new possibilities for designing HPA-axis stressors using VR.
Additionally, the difference in the results between the high- and low-intensity groups suggests that the degree of HPA-axis stress can be changed by the behavior of the avatar. Previous studies confirmed that the level of ANS stress, judging from heart rate information, changes depending on the dialog feedback response (positive or negative) between humans and virtual characters (Hartanto et al., 2014). However, this study suggests that the HPA-axis stress response can be elicited by VR communication simulation and that the response level varies depending on the tone and attitude of the conversational avatar.
Furthermore, in the communication training environment used in this study, the LF/HF significantly increased in the high-intensity group compared to that in the pre-task resting state, confirming the occurrence of ANS stress responses. This trend was also evident in subjective stress evaluations, with the high-intensity group indicating significantly higher stress assessment values on a 5-point Likert scale than the low-intensity group. These results are consistent with previous findings and show that the avatar’s behavior can indeed modulate not only HPA-axis stress responses but also autonomic nervous system stress responses and subjective stress levels.
In our experiment, we confirmed that HPA-axis stress responses can be induced by the attitude of the avatar in a VR communication simulation with scripted dialog. However, it is unclear which factors of the avatars’ behavior, such as gestures or tone of voice, have a greater influence on the HPA-axis stress response. The participants’ open-ended responses suggest that both tone of voice and gestures contribute to stress. However, separating and clarifying the effects of each element, as well as understanding the impact of other factors such as the expressions of avatars, would be beneficial in constructing effective VR communication simulation systems for use as training systems and stressors.
Moreover, this experiment was conducted using a between-subjects design, with approximately 10 participants in each condition. This number is small compared to previous studies of V-TSST (Helminen et al., 2019). Therefore, although the difference between the high- and low-intensity groups was significant in terms of effect size, comparable to prior research, in terms of the generalizability of the results, limitations exist.
Additionally, there was an imbalance in the gender ratio of participants, with only one female in each group. This imbalance could have influenced the results. For example, it has been reported that women have quicker and stronger HPA-axis stress responses to stressors compared to men (Goel et al., 2011). However, no significant gender difference has been reported in the cortisol response induced by V-TSST (Santl et al., 2019). Future studies should address this imbalance in participants and examine the generalizability of HPA-axis stress responses in fixed dialog VR communication simulations.
Furthermore, although the impact of immersion in a virtual environment on stress has been discussed in previous studies on V-TSST (Ling et al., 2012), it is unclear whether immersion also contributes to the occurrence of HPA-axis stress responses in communication simulations. Regarding immersion, in our experimental system, there were challenges related to the avatar quality, in particular caused by the limitations of the capture system which affected the realism of the avatar models. The impact of avatar realism on stress tasks such as V-TSST has not been directly verified. However, there are reports suggesting that high arousal emotions like fear and anxiety are susceptible to the quality and immersion of VR (Diemer et al., 2015). Therefore, the quality of the avatar could influence the intensity of stress in VR communication simulations, which needs to be clarified in future experiments.
Identifying the factors that trigger HPA-axis stress responses in VR is important for VR utilization as a stressor in the research and development of VR communication training systems, which should be addressed in future research. The factors causing such stress are likely to differ based on individual user characteristics, underscoring the need for future investigations into personalized VR communication simulations.
Furthermore, the extent to which the intensity of the load can be adjusted should be determined by changing the attitude of the avatar. In the low-intensity training group of this study, salivary cortisol levels did not significantly increase from pre-stress levels at any time point. Thus, methods should be developed to adjust the attitude of the avatar for more precise load adjustment.
From the perspective of applying VR communication simulation to training, it is important to eliminate the “habituation” that occurs through repeated training. In our experiment, changes in the cortisol response from repeated exposure to VR communication simulations were not measured, necessitating future investigation. However, it has been reported that HPA responses quickly habituate in TSST, with cortisol responses significantly decreasing after repeated stress exposure (Wüst et al., 2005), making it highly likely that such habituation will also occur in VR communication simulations. In a training system, an appropriate level of stress should be maintained on the trainee throughout repeated training sessions. Therefore, it is essential to develop a method to prevent stress load reduction from habituation. Because the magnitude of HPA-axis stress responses has been reported to decrease upon continuous exposure to the same stressor (Roos et al., 2019), reduction in stress load may be avoided by changing the appearance or voice of the avatar, even if the conversation scenario remains the same.
In this study, a user study was conducted to investigate whether a virtual communication simulation with scripted dialog can elicit HPA-axis stress response. The experiment employed a virtual customer service system in which participants addressed a complaining customer in a scenario involving a flight delay due to airport mishandling. By changing only the tone and gestures of the virtual customer while keeping the content of the conversation the same, we examined how varying the intensity of the presented stress affects HPA-axis stress response, specifically the cortisol levels in saliva.
The results of the experiment confirmed that HPA-axis stress responses can be elicited by a static virtual CST system, and the stress responses can be adjusted by differences in the attitude of a virtual customer. Because the only established VR social stress task that can generate HPA-axis stress is the V-TSST, the results of this study have potential applications in observing human physiological responses to stress and formulating stress reduction strategies. Furthermore, the finding that a VR-based communication simulation can elicit an HPA-axis stress response similar to actual verbal interactions, with predetermined conversation flow, provides evidence of the effectiveness of communication training using VR.
The raw data supporting the conclusions of this article will be made available by the authors, without undue reservation.
The studies involving humans were approved by the Research Ethics Committee of the University of Tokyo. The studies were conducted in accordance with the local legislation and institutional requirements. The participants provided their written informed consent to participate in this study. Written informed consent was obtained from the individual(s) for the publication of any potentially identifiable images or data included in this article.
YB: Conceptualization, Formal Analysis, Investigation, Methodology, Validation, Writing–original draft. MI: Investigation, Methodology, Resources, Writing–review and editing. CK: Investigation, Writing–review and editing. S’IW: Supervision, Writing–review and editing.
The author(s) declare financial support was received for the research, authorship, and/or publication of this article. This research was partially supported by Council for Science, Technology and Innovation, “Cross-ministerial Strategic Innovation Promotion Program (SIP), Big-data and AI-enabled Cyberspace Technologies.” (funding agency: NEDO).
The authors declare that the research was conducted in the absence of any commercial or financial relationships that could be construed as a potential conflict of interest.
All claims expressed in this article are solely those of the authors and do not necessarily represent those of their affiliated organizations, or those of the publisher, the editors and the reviewers. Any product that may be evaluated in this article, or claim that may be made by its manufacturer, is not guaranteed or endorsed by the publisher.
The Supplementary Material for this article can be found online at: https://www.frontiersin.org/articles/10.3389/frvir.2023.1302720/full#supplementary-material
Bertsch, K., Böhnke, R., Kruk, M. R., Richter, S., and Naumann, E. (2011). Exogenous cortisol facilitates responses to social threat under high provocation. Hormones Behav. 59, 428–434. doi:10.1016/j.yhbeh.2010.12.010
Birkett, M. A. (2011). The trier social stress test protocol for inducing psychological stress. JoVE J. Vis. Exp., e3238. doi:10.3791/3238
Breuninger, C., Sláma, D. M., Krämer, M., Schmitz, J., and Tuschen-Caffier, B. (2017). Psychophysiological reactivity, interoception and emotion regulation in patients with agoraphobia during virtual reality anxiety induction. Cognitive Ther. Res. 41, 193–205. doi:10.1007/s10608-016-9814-9
Cebeci, B., Celikcan, U., and Capin, T. K. (2019). A comprehensive study of the affective and physiological responses induced by dynamic virtual reality environments. Comput. Animat. Virtual Worlds 30, e1893. doi:10.1002/cav.1893
Cettolin, E., Dalton, P. S., Kop, W., and Zhang, W. (2020). Cortisol meets garp: the effect of stress on economic rationality. Exp. Econ. 23, 554–574. doi:10.1007/s10683-019-09624-z
Chessa, M., Maiello, G., Borsari, A., and Bex, P. J. (2019). The perceptual quality of the oculus rift for immersive virtual reality. Human–computer Interact. 34, 51–82. doi:10.1080/07370024.2016.1243478
DeVries, A. C., Glasper, E. R., and Detillion, C. E. (2003). Social modulation of stress responses. Physiology Behav. 79, 399–407. doi:10.1016/s0031-9384(03)00152-5
Dibbets, P. (2020). A novel virtual reality paradigm: predictors for stress-related intrusions and avoidance behavior. J. Behav. Ther. Exp. psychiatry 67, 101449. doi:10.1016/j.jbtep.2019.01.001
Dickerson, S. S., and Kemeny, M. E. (2004). Acute stressors and cortisol responses: a theoretical integration and synthesis of laboratory research. Psychol. Bull. 130, 355–391. doi:10.1037/0033-2909.130.3.355
Diemer, J., Alpers, G. W., Peperkorn, H. M., Shiban, Y., and Mühlberger, A. (2015). The impact of perception and presence on emotional reactions: a review of research in virtual reality. Front. Psychol. 6, 26. doi:10.3389/fpsyg.2015.00026
Diemer, J., Lohkamp, N., Mühlberger, A., and Zwanzger, P. (2016). Fear and physiological arousal during a virtual height challenge—effects in patients with acrophobia and healthy controls. J. anxiety Disord. 37, 30–39. doi:10.1016/j.janxdis.2015.10.007
Eisenlohr-Moul, T. A., Miller, A. B., Giletta, M., Hastings, P. D., Rudolph, K. D., Nock, M. K., et al. (2018). Hpa axis response and psychosocial stress as interactive predictors of suicidal ideation and behavior in adolescent females: a multilevel diathesis-stress framework. Neuropsychopharmacology 43, 2564–2571. doi:10.1038/s41386-018-0206-6
Felnhofer, A., Kothgassner, O. D., Hetterle, T., Beutl, L., Hlavacs, H., and Kryspin-Exner, I. (2014). Afraid to be there? evaluating the relation between presence, self-reported anxiety, and heart rate in a virtual public speaking task. Cyberpsychology, Behav. Soc. Netw. 17, 310–316. doi:10.1089/cyber.2013.0472
Figueiro, M. G., and Rea, M. S. (2012). Short-wavelength light enhances cortisol awakening response in sleep-restricted adolescents. Int. J. Endocrinol. 2012, 1–7. doi:10.1155/2012/301935
Finseth, T. T., Keren, N., Dorneich, M. C., Franke, W. D., Anderson, C. C., and Shelley, M. C. (2018). Evaluating the effectiveness of graduated stress exposure in virtual spaceflight hazard training. J. Cognitive Eng. Decis. Mak. 12, 248–268. doi:10.1177/1555343418775561
Goel, N., Workman, J. L., Lee, T. T., Innala, L., and Viau, V. (2011). Sex differences in the hpa axis. Compr. Physiol. 4, 1121–1155. doi:10.1002/cphy.c130054
Gonzalez-Liencres, C., Zapata, L. E., Iruretagoyena, G., Seinfeld, S., Perez-Mendez, L., Arroyo-Palacios, J., et al. (2020). Being the victim of intimate partner violence in virtual reality: first-versus third-person perspective. Front. Psychol. 11, 820. doi:10.3389/fpsyg.2020.00820
Goodman, W. K., Janson, J., and Wolf, J. M. (2017). Meta-analytical assessment of the effects of protocol variations on cortisol responses to the trier social stress test. Psychoneuroendocrinology 80, 26–35. doi:10.1016/j.psyneuen.2017.02.030
Gradl, S., Wirth, M., Mächtlinger, N., Poguntke, R., Wonner, A., Rohleder, N., et al. (2019). “The stroop room: a virtual reality-enhanced stroop test,” in Proceedings of the 25th ACM Symposium on Virtual Reality Software and Technology, Sydney, Australia, November 12-15, 2019, 1.
Hartanto, D., Kampmann, I. L., Morina, N., Emmelkamp, P. G., Neerincx, M. A., and Brinkman, W.-P. (2014). Controlling social stress in virtual reality environments. PloS one 9, e92804. doi:10.1371/journal.pone.0092804
Helminen, E. C., Morton, M. L., Wang, Q., and Felver, J. C. (2019). A meta-analysis of cortisol reactivity to the trier social stress test in virtual environments. Psychoneuroendocrinology 110, 104437. doi:10.1016/j.psyneuen.2019.104437
Jönsson, P., Wallergård, M., Österberg, K., Hansen, Å. M., Johansson, G., and Karlson, B. (2010). Cardiovascular and cortisol reactivity and habituation to a virtual reality version of the trier social stress test: a pilot study. Psychoneuroendocrinology 35, 1397–1403. doi:10.1016/j.psyneuen.2010.04.003
Kirschbaum, C., and Hellhammer, D. H. (1989). Salivary cortisol in psychobiological research: an overview. Neuropsychobiology 22, 150–169. doi:10.1159/000118611
Kirschbaum, C., Prussner, J. C., Stone, A. A., Federenko, I., Gaab, J., Lintz, D., et al. (1995). Persistent high cortisol responses to repeated psychological stress in a subpopulation of healthy men. Psychosom. Med. 57, 468–474. doi:10.1097/00006842-199509000-00009
Kishimoto, T., and Ding, X. (2019). The influences of virtual social feedback on social anxiety disorders. Behav. cognitive psychotherapy 47, 726–735. doi:10.1017/s1352465819000377
Kothgassner, O. D., Goreis, A., Glenk, L. M., Kafka, J. X., Pfeffer, B., Beutl, L., et al. (2021). Habituation of salivary cortisol and cardiovascular reactivity to a repeated real-life and virtual reality trier social stress test. Physiology Behav. 242, 113618. doi:10.1016/j.physbeh.2021.113618
Kudielka, B. M., Hellhammer, D. H., and Wüst, S. (2009). Why do we respond so differently? reviewing determinants of human salivary cortisol responses to challenge. Psychoneuroendocrinology 34, 2–18. doi:10.1016/j.psyneuen.2008.10.004
Kudielka, B. M., and Wüst, S. (2010). Human models in acute and chronic stress: assessing determinants of individual hypothalamus–pituitary–adrenal axis activity and reactivity. Stress 13, 1–14. doi:10.3109/10253890902874913
Lee, D. H., and Jung, T.-P. (2020). A virtual reality game as a tool to assess physiological correlations of stress. arXiv preprint arXiv:2009.14421.
Lemmens, J. S., Simon, M., and Sumter, S. R. (2022). Fear and loathing in vr: the emotional and physiological effects of immersive games. Virtual Real. 26, 223–234. doi:10.1007/s10055-021-00555-w
Ling, Y., Brinkman, W.-P., Nefs, H. T., Qu, C., and Heynderickx, I. (2012). Effects of stereoscopic viewing on presence, anxiety, and cybersickness in a virtual reality environment for public speaking. Presence Teleoperators Virtual Environ. 21, 254–267. doi:10.1162/pres_a_00111
Luong, T., Martin, N., Argelaguet, F., and Lécuyer, A. (2019). “Studying the mental effort in virtual versus real environments,” in 2019 IEEE Conference on virtual reality and 3D user interfaces (VR), Osaka, Japan, March 23 2019 to March 27 2019, 809–816.
Narciso, D., Melo, M., Raposo, J. V., Cunha, J., and Bessa, M. (2020). Virtual reality in training: an experimental study with firefighters. Multimedia tools Appl. 79, 6227–6245. doi:10.1007/s11042-019-08323-4
Notzon, S., Deppermann, S., Fallgatter, A., Diemer, J., Kroczek, A., Domschke, K., et al. (2015). Psychophysiological effects of an itbs modulated virtual reality challenge including participants with spider phobia. Biol. Psychol. 112, 66–76. doi:10.1016/j.biopsycho.2015.10.003
Pan, X., Slater, M., Beacco, A., Navarro, X., Bellido Rivas, A. I., Swapp, D., et al. (2016). The responses of medical general practitioners to unreasonable patient demand for antibiotics-a study of medical ethics using immersive virtual reality. PloS one 11, e0146837. doi:10.1371/journal.pone.0146837
Petrowski, K., Wintermann, G.-B., and Siepmann, M. (2012). Cortisol response to repeated psychosocial stress. Appl. Psychophysiol. biofeedback 37, 103–107. doi:10.1007/s10484-012-9183-4
Powers, M. B., Briceno, N. F., Gresham, R., Jouriles, E. N., Emmelkamp, P. M., and Smits, J. A. (2013). Do conversations with virtual avatars increase feelings of social anxiety? J. anxiety Disord. 27, 398–403. doi:10.1016/j.janxdis.2013.03.003
Roos, L. G., Janson, J., Sturmbauer, S. C., Bennett, J. M., and Rohleder, N. (2019). Higher trait reappraisal predicts stronger hpa axis habituation to repeated stress. Psychoneuroendocrinology 101, 12–18. doi:10.1016/j.psyneuen.2018.10.018
Santl, J., Shiban, Y., Plab, A., Wüst, S., Kudielka, B. M., and Mühlberger, A. (2019). Gender differences in stress responses during a virtual reality trier social stress test. Int. J. Virtual Real. 19, 2–15. doi:10.20870/ijvr.2019.19.2.2912
Schneiderman, N., Ironson, G., and Siegel, S. D. (2005). Stress and health: psychological, behavioral, and biological determinants. Annu. Rev. Clin. Psychol. 1, 607–628. doi:10.1146/annurev.clinpsy.1.102803.144141
Schommer, N. C., Hellhammer, D. H., and Kirschbaum, C. (2003). Dissociation between reactivity of the hypothalamus-pituitary-adrenal axis and the sympathetic-adrenal-medullary system to repeated psychosocial stress. Psychosom. Med. 65, 450–460. doi:10.1097/01.psy.0000035721.12441.17
Schwabe, L., Haddad, L., and Schachinger, H. (2008). Hpa axis activation by a socially evaluated cold-pressor test. Psychoneuroendocrinology 33, 890–895. doi:10.1016/j.psyneuen.2008.03.001
Seery, M. D. (2011). Challenge or threat? cardiovascular indexes of resilience and vulnerability to potential stress in humans. Neurosci. Biobehav. Rev. 35, 1603–1610. doi:10.1016/j.neubiorev.2011.03.003
Shimada, M., Takahashi, K., Ohkawa, T., Segawa, M., and Higurashi, M. (1995). Determination of salivary cortisol by elisa and its application to the assessment of the circadian rhythm in children. Hormone Res. Paediatr. 44, 213–217. doi:10.1159/000184628
Tanikawa, T., Shiozaki, K., Ban, Y., Aoyama, K., and Hirose, M. (2021). “Semi-automatic reply avatar for vr training system with adapted scenario to trainee’s status,” in HCI international 2021 - late breaking papers: multimodality, eXtended reality, and artificial intelligence. Editors C. Stephanidis, M. Kurosu, J. Y. C. Chen, G. Fragomeni, N. Streitz, S. Konomiet al. (Cham: Springer International Publishing), 350–355.
Taupiac, J.-D., Rodriguez, N., Strauss, O., and Beney, P. (2019). “Social skills training tool in virtual reality, intended for managers and sales representatives,” in 2019 IEEE Conference on Virtual Reality and 3D User Interfaces (VR), Osaka, Japan, March 23 2019 to March 27 2019, 1183.
Thayer, J. F., and Lane, R. D. (2000). A model of neurovisceral integration in emotion regulation and dysregulation. J. Affect. Disord. 61, 201–216. doi:10.1016/s0165-0327(00)00338-4
Toet, A., van Welie, M., and Houtkamp, J. (2009). Is a dark virtual environment scary? CyberPsychology Behav. 12, 363–371. doi:10.1089/cpb.2008.0293
Tremmel, C., Herff, C., Sato, T., Rechowicz, K., Yamani, Y., and Krusienski, D. J. (2019). Estimating cognitive workload in an interactive virtual reality environment using eeg. Front. Hum. Neurosci. 13, 401. doi:10.3389/fnhum.2019.00401
van Dammen, L., Finseth, T. T., McCurdy, B. H., Barnett, N. P., Conrady, R., Leach, A. G., et al. (2022). Evoking stress reactivity in virtual reality: a systematic review and meta-analysis. Neurosci. Biobehav. Rev. 138, 104709. doi:10.1016/j.neubiorev.2022.104709
Viciana-Abad, R., Reyes-Lecuona, A., García-Berdonés, C., Díaz-Estrella, A., and Castillo-Carrión, S. (2004). The importance of significant information in presence and stress within a virtual reality experience. Annu. Rev. Cyberther Telemed. 2, 111–118.
Wüst, S., Federenko, I. S., van Rossum, E. F., Koper, J. W., and Hellhammer, D. H. (2005). Habituation of cortisol responses to repeated psychosocial stress-further characterization and impact of genetic factors. Psychoneuroendocrinology 30, 199–211. doi:10.1016/j.psyneuen.2004.07.002
Keywords: VR communication simulation, HPA axis stress, VR stress task, social stress, virtual reality
Citation: Ban Y, Inazawa M, Kato C and Warisawa S (2024) VR communication simulation with scripted dialog elicits HPA axis stress. Front. Virtual Real. 4:1302720. doi: 10.3389/frvir.2023.1302720
Received: 26 September 2023; Accepted: 21 December 2023;
Published: 11 January 2024.
Edited by:
Beatrice Biancardi, LINEACT CESI, FranceReviewed by:
Meredith Carroll, Florida Institute of Technology, United StatesCopyright © 2024 Ban, Inazawa, Kato and Warisawa. This is an open-access article distributed under the terms of the Creative Commons Attribution License (CC BY). The use, distribution or reproduction in other forums is permitted, provided the original author(s) and the copyright owner(s) are credited and that the original publication in this journal is cited, in accordance with accepted academic practice. No use, distribution or reproduction is permitted which does not comply with these terms.
*Correspondence: Yuki Ban, YmFuQGVkdS5rLnUtdG9reW8uYWMuanA=
Disclaimer: All claims expressed in this article are solely those of the authors and do not necessarily represent those of their affiliated organizations, or those of the publisher, the editors and the reviewers. Any product that may be evaluated in this article or claim that may be made by its manufacturer is not guaranteed or endorsed by the publisher.
Research integrity at Frontiers
Learn more about the work of our research integrity team to safeguard the quality of each article we publish.