- 1Interactive Media, Arts and Humanities Division, New York University Abu Dhabi, Abu Dhabi, United Arab Emirates
- 2Event Lab, Department of Clinical Psychology and Psychobiology, Institute of Neurosciences, University of Barcelona, Barcelona, Spain
Moving through a virtual environment that is larger than the physical space in which the participant operates has been a challenge since the early days of virtual reality. Many different methods have been proposed, such as joystick-based navigation, walking in place where the participant makes walking movements but is stationary in the physical space, and redirected walking where the environment is surreptitiously changed giving the illusion of walking in a long straight line in the virtual space but maybe a circle in the physical space. Each type of method has its limitations, ranging from simulator sickness to still requiring more physical space than is available. Stimulated by the COVID-19 lockdown, we developed a new method of locomotion which we refer to as interactive redirected walking. Here, the participant really walks but, when reaching a boundary, rotates the virtual world so that continuation of walking is always within the physical boundary. We carried out an exploratory study to compare this method with walking in place with respect to presence using questionnaires as well as qualitative responses based on comments written by the participants that were subjected to sentiment analysis. Surprisingly, we found that smaller physical boundaries favor interactive redirected walking, but for boundary lengths more than approximately 7 adult paces, the walking-in-place method is preferable.
1 Introduction
Since the early days of virtual reality, a fundamental problem has been how to enable people to move through a virtual space larger than the real space in which the action was taking place. A paradigm for this was essential in those earlier days of VR since the tracking space was typically limited to approximately 1 m radius due to the use of electromagnetic trackers. The obvious method (referred to as “joystick”) was to use a hand-held controller to virtually move in the direction of gaze, or in the controller pointing direction, while pressing a controller button. This has two main disadvantages: the first is simulator sickness, with the visual system indicating that the body is moving while the vestibular and sensorimotor systems indicate that it is stationary. According to sensory conflict theory (Stanney and Kennedy, 2009), this contributes to feelings of nausea and other symptoms. The second main disadvantage is that because movement through the virtual environment is not accompanied by corresponding bodily movement, participants can experience disorientation, with degraded wayfinding behavior, a result long-known in VR research (Darken and Sibert, 1996; Darken et al., 1999).
Due to these problems, various paradigms have been invented for locomotion. Fairchild et al. (1993) developed a metaphor where physically stationary participants leaned in the direction of desired travel to move forward, thus, to some extent, mobilizing the whole body as part of the movement. Rather than moving through the environment, Stoakley et al. (1995) developed the “worlds in miniature” paradigm, where participants could zoom out of it, see it in miniature, and pick up an avatar representing themselves and move it to a desired location, after which they would be located there. Not too different from the original joystick (point-and-move) paradigm, Mine (1995) originally referred to the possibility of “instant teleportation.” In this case, the participant points to a desired location and is instantly teleported there. One way to implement this, for example, is through a line or curve that emerges from the location of the hand-held controller to the desired location, and the participant is transported there on carrying out some action (such as pressing or releasing a button). Bozgeyikli et al. (2016) described experimental studies comparing this method with others, and a useful survey was provided by Prithul et al. (2021). This paradigm, often referred to simply as “teleportation,” is one of the most common virtual locomotion techniques and is widely used, for example, in gaming and online VR communities. While it is efficient, effective, and avoids the sensory conflict that can induce discomfort and simulator sickness, it runs the danger of disorientation and misses the sensation of actually walking.
One method to overcome this limitation and provide a more immersive and intuitive locomotion experience is a paradigm originally known as the “virtual treadmill” (Slater et al., 1995a) and also referred to as “walking-in-place” (WiP) (Slater et al., 1995b). In this paradigm, participants actually mimic walking actions while staying in their location, analogous to a real treadmill. This was implemented via a neural network that learned to distinguish between head movements that indicated WiP compared to any other movements, a method recently updated with more powerful machine learning techniques (Hanson et al., 2019).
Razzaque et al. (2001) introduced an influential paradigm known as “redirected walking.” A participant could walk in a straight line in VR over distances much larger than the physical space available; however, this was achieved by imperceptible rotation or shrinkage of the virtual world whenever a physical boundary was approached, sometimes using a distraction technique to shift attention to make it less likely that a transformation would be noticed. In reality, the participant might be walking in circles but in a straight line in VR. The technique was combined with WiP by Razzaque et al. (2002). Redirected walking is an area of avid interest over the years, and a comprehensive review was presented by Li et al. (2022).
The many locomotion paradigms also include unusual concepts, such as reliance on thoughts alone. For example, using a brain–computer interface, (Leeb et al., 2006; Pfurtscheller et al., 2006) achieved locomotion through a measurement of brain activation, where the participant would move forward whenever a particular brain pattern, as measured in real-time by EEG, was detected. This successfully allowed a paraplegic patient to move through a virtual environment solely by thought. Cherni et al. (2020) have provided a comprehensive literature review of locomotion techniques since 2012, and Boletsis (2017) provided a typology. Of course, the most obvious paradigm is simply to walk. This was not possible in the early days of VR because of restrictions on the tracking area, as explained previously; however, very-wide-area ceiling-mounted tracking systems were developed. Using one at UNC Chapel Hill, Usoh et al. (1999) carried out an experiment where real walking was compared to WiP and the standard “flying” technique (joystick). The results showed that, with respect to presence, the illusion of “being there,” there were significant differences between both WiP and walking in reality compared to flying, but the major advantage of walking in reality over WiP was accuracy—since the neural network-based WiP had a latency so that when the participants stopped moving, they would still move virtually for a few paces.
At present, the situation is rather different since inside-out tracking based on SLAM (simultaneous localization and mapping) (Fuentes-Pacheco et al., 2015) has been widely incorporated into commercial headsets for VR and AR. In principle, participants can walk over large distances using this method. This is because there is no tracking device external to the headset. Instead, tracking is accomplished wholly through analysis of images of the surrounding environment obtained by the headset cameras. However, in reality, people are always confined within a space—the room in which they might be playing a game or navigating an environment for an architectural walk-through, although outdoor use is possible. Modern head-mounted displays typically allow participants to specify a “guardian,” which is a boundary around the area of action that contains no obstacles or walls. The guardian remains invisible in VR while the participant is in a physically safe area and becomes visible when there is a bodily intersection with it. So the following question remains to be answered: how can people walk through virtual distances that are greater than the physical boundary of the guardian? Certainly, any of the techniques discussed previously could be employed.
In this paper, we describe an experiment designed to examine the effects of two different styles of walking through a virtual environment on presence, the illusion of “being there” (Held and Durlach, 1992; Sheridan, 1992; 1996; Sanchez-Vives and Slater, 2005), and that the events were really happening (plausibility) (Slater, 2009; Slater et al., 2022a). It was designed during and because of the COVID-19 lockdowns, where people were confined indoors, and VR would be a means by which people could have the effective illusion of being outdoors. However, as described previously, moving through an environment is usually problematic because using the simplest interface, moving by pressing a controller button, typically results in simulator sickness.
Although really walking through extensive spaces is entirely possible with currently available “inside-out” tracking systems, unlike the use of tracking systems that rely on fixed place sensors, several authors have discussed the minimum space requirements for redirected walking to be possible or effective. This was pointed out in the original paper by Razzaque et al. (2001), and Steinicke et al. (2009) found that a circular arc of at least 22 m was required. For people in their home environment, such space requirements may not be realizable. However, techniques have been proposed to overcome this limitation (Fan et al., 2023) which will be analyzed in the Discussion Section 4.
Hence, we designed an interactive version of redirected walking that uses, rather than attempts to avoid, the guardian. People walk along a virtual straight road until they hit the guardian which becomes visible. At this point, they are at the guardian boundary, and the extent of the guardian is behind them. Therefore, they swivel themselves and the road by approximately 180° until the road is again lined up with the guardian. They can then continue walking until they hit the guardian again, and once again can swivel themselves and the road around until it lines up with the available space within the guardian. In this way, they can keep walking for as long as they want. The experiment, which was exploratory in nature rather than hypothesis-driven, was designed for a Meta Quest device. There were two principal goals:
(1) To compare the interactive version of redirected walking with walking-in-place in terms of presence and preference.
(2) To make it simple for people to access the program without having to use sideload, which, in our experience, many people find difficult or at least tedious to use because it requires putting the Quest into developer mode and following various procedures. Therefore, the program was implemented entirely for the Quest browser and used several “WebVR” tools.
2 Materials and methods
2.1 Ethical approval
Ethical approval for the study was granted by the Institutional Review Boards of Comissió de Bioètica, Universitat de Barcelona (IRB00003099), and NYUAD (HRPP-2022-194), and all participants provided written and informed consent. Participants were compensated with 10 euros for their effort and time spent. Participation at NYUAD was voluntary, and all participating students were eligible to apply for course credit.
2.2 Experimental design
The experimental design was between-groups, with one factor with two levels: walking—the interactive redirected walking method described previously and WiP—walking-in-place. We consider redirected walking the preferred method for moving through a confined space and WiP the only other method for mobilizing the whole body with movements akin to walking. Therefore, our interest was confined essentially to these two methods. There were 21 participants recruited to the WiP condition and 26 to the walking condition; the imbalance was due to practical limits on participant availability.
2.3 Recruitment
This work started during the lockdowns, and at first, the idea was to run the experiment entirely online, recruiting participants from services such as Amazon Mechanical Turk1 and later using Prolific2, a service specialized for conducting online experimental studies.
In order to encourage honesty in questionnaire responding (where participants might complete the questionnaire without actually carrying out the experiment), some simple techniques were used: first, the participants were asked, as part of their questionnaire responses (see below), to write in a phrase that was written on the strap of the Quest. With Amazon Mechanical Turk, it was found that out of approximately 1,000 respondents, only six were able to type in the correct phrase. Therefore, Amazon Mechanical Turk was abandoned, and we moved to Prolific. Second, the participants were asked to write down the name of the person in a large picture, at the end of the road, Marylin Monroe. However, since the program was written for the browser, it is possible for people to see the actual program and the resources (like images) that it used and thereby answer this question without actually going through the VR experience. With Prolific, the situation was somewhat better, but we could not rule out the possibility that groups of people might share the required answers. A third technique used was to compute the height of the participants from the y-axis coordinate returned to the program by the Quest head tracking. This was converted into a 4-digit code that was displayed to participants at the end of the exposure, and they were asked to write this code in the questionnaire. In addition, in the questionnaire, participants were asked to write down their height. A strong correlation between the codes and the self-reported heights would indicate that the participants were actually using the head-mounted display, and any outlier would indicate possible deception. Although this method worked, in order to completely rule out the possibility of cheating (and to reduce the considerable costs of using Prolific) and given that lockdowns were over, we moved to running the experiment in a laboratory. In the end, only six of the online results could be retained3. The remaining participants (41) were recruited around the Campus of New York University Abu Dhabi and by invitation through social media.
2.4 Procedures
Once participants had agreed to take part in the study, they began by responding to a questionnaire available on Qualtrics4. This started with a brief description of the experiment, data protection information, and an ethics consent form. Inclusion/exclusion criteria were as follows:
• At least 18 years of age.
• Able to walk at least 100 m without difficulty.
• Not having epilepsy.
• Not intending to drive a motorized vehicle or use any type of complex machinery that can be a danger to themselves or others for at least 3 h after finishing the virtual reality experience.
• Possessing a Meta Quest headset (for online-only participants since the laboratory participants used the headset available in the laboratory).
Then, a demonstration of how to use the Quest controllers with the names of the various buttons was given in the Qualtrics page, which was also demonstrated by the experimenter in the case of the laboratory participants.
The questionnaire contained questions about which type of Quest would be used (1 or 2), and the participants were asked to map out the guardian and report the maximum number of paces they could walk before hitting the boundary. Information about handedness, gender, age, and other details, as shown in the first column of Table 1, was recorded.
Participants then entered the virtual reality environment. The scene was a 100 m straight road. At equal intervals, there were small photographs of famous movie stars lying flat on the road (Figure 1). Audio and video instructions on how to move forward along the road were provided. For those under the WiP condition, a horizontal line appeared at eye height, and they were instructed that if they moved their head below and above the line, as would occur while walking-in-place, they would move forward along the road. In a practice session, they were asked to use WiP (illustrated by a video) to move out to the first small picture embedded on the ground and then turn back and return to the start and turn around once again and face the far end of the road. They were then instructed to walk the 100 m to the other end of the road.
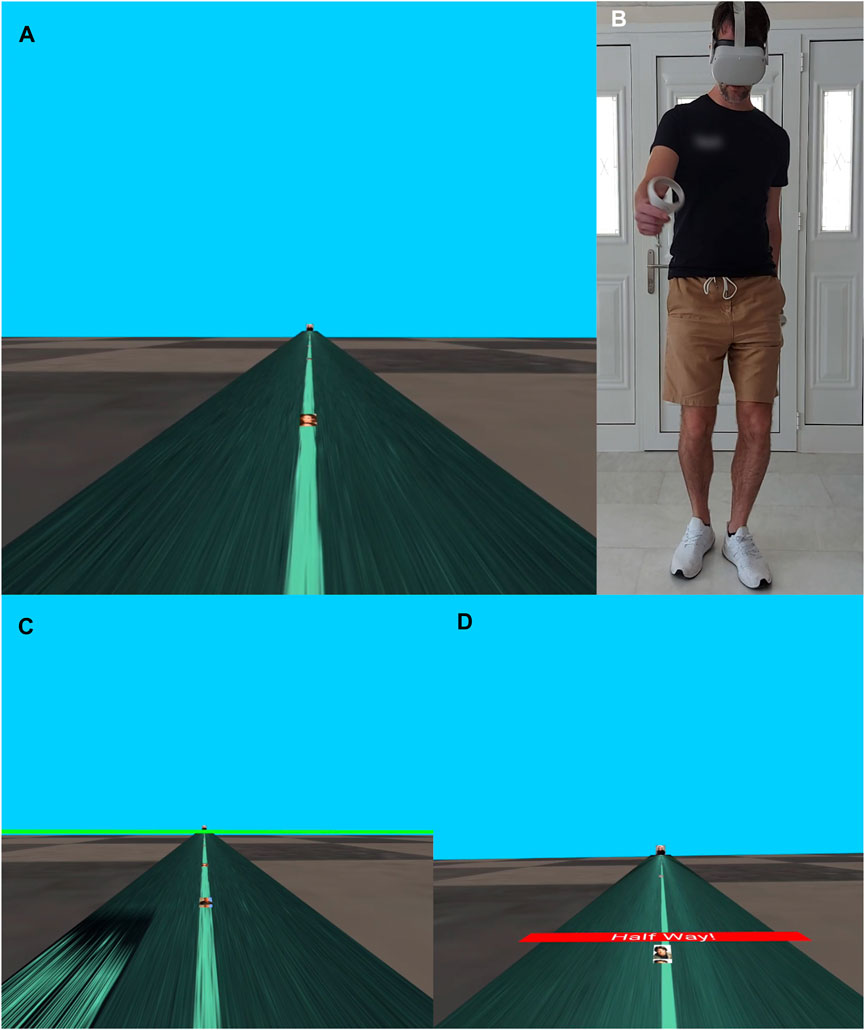
FIGURE 1. Scenario. (A) VR scene with the 100 m straight road and the small photographs of famous movie stars on the road. (B) Participant with the Quest 2 head-mounted display. (C) Initial state of the WiP method, where the green ribbon is shown, and participants learn that moving their head above and below this moves them forward. (D) Scenario showing the halfway mark and the picture of Marilyn Monroe at the end of the road.
Those under the walking condition were asked to walk until they hit the boundary of the guardian so that the guardian was visible. They were shown (illustrated by a video) that by holding down the trigger button on any controller and turning, the road would turn with them, and they could line it up within the boundary of the guardian behind them. They could then continue walking back to the start of the road and again turn using the same method. However, in VR, they would still be walking along the same straight road. Using this technique, they could walk the entire 100 m road, see Supplementary Movie S1. (The far end of the road has been blurred for copyright reasons).
Under both conditions, when they reached the end of the road, they were by the photograph of Marilyn Monroe they were asked to note down a code number that appeared and then completely remove the head-mounted display (HMD) and continue with the Qualtrics questionnaire. The questionnaire contains the series of questions shown in the first column of Table 2.
Note that the last three questions were open-ended. The question labeled technique was asked only to check the understanding of the participants about the method of locomotion.
2.5 Materials
The experiment was carried out using the Meta Quest 1 or 2. The Quest 1 has a Qualcomm Snapdragon 835 CUP, featuring OLED screens with a resolution of 1440 × 1600 pixels per eye with a refresh rate of 72 Hz, and weighs 503 g. The Quest 2 has an LED screen resolution of 1832 × 1920 pixels per eye with a refresh rate of 90 Hz and weighs 571 g. Both Quest 1 and Quest 2 have integrated speakers built directly into the headset straps, providing spatial audio, as well as Quest touch controllers with hand-tracking capabilities.
To carry out the experiment simulating a number of different physical spaces, participants in the laboratory were provided with preset guardian configurations of various sizes.
2.6 Implementation
The interactive environment was implemented using WebVR tools and run in the Quest browser. It made use of aframe.io and three.js sound files and videos for instructions that were incorporated into the program. The WiP implementation5 and the walking6 implementation can be tried interactively on a Quest using the Quest browser. In order to examine the code, these URLs can be opened, for example, using the Chrome browser, and then the menu “View/Developer/View Source” can be viewed.
The implementation of the WiP method was very simple. The height of the participant’s eye position was obtained from the y component of the world position, and if the participant’s real-time-tracked head position bobbed up and down more than 0.03 m from this height, the participant would be moved forward with a stride length of 0.762 m. In the initial phase prior to the actual locomotion, the eye height was represented in the environment by a horizontal green ribbon, as described previously, and a video was played so that participants could see how walking-in-place would shift the head above and below that height. Such a simple implementation, rather than using a neural network, was determined by the resources of the WebVR-based program.
The walking method relied on participants indicating that they had reached the boundary of the guardian by pressing the trigger button on either hand, then, as they turned their body, specifically their wrist, the entire environment would rotate about them.
2.7 Statistical methods
2.7.1 The ordinal and binary questionnaire variables
In Table 2, all of the response variables are ordinal on a scale of 1–7, except for lockdown, which is binary, and the last three questions, which were open-ended (the variable photo is not considered a response variable but part of the method of testing whether the online participants had actually experienced the VR).
For the ordinal response variables, we use a Bayesian ordered logistic regression and binary logistic regression for lockdown. This results in posterior distributions for each of the parameters of the model, from which we can compute any probabilities of interest. The model includes all response variables simultaneously, so there is no issue with multiple comparisons (which results in problems in the interpretation of significance levels in classical null hypothesis significance testing).
Suppose
Here,
Sex, age, and Quest are included to allow for possible differences between the participants and conditions influencing the outcomes.
In ordered logistic regression, it is assumed that there is a latent continuous variable
Whenever the value of
Rearranging (1), we obtain
Hence, the interpretation of each
Binary logistic regression is a special case when there are only two outcomes (e.g., “Home” (0) or “VR” (1) in the case of lockdown). In this case,
or
We use these models for each of the response variables simultaneously (and the parameters
We use weakly informative prior distributions for each of the parameters: normal (mean = 0, standard deviation = 10), which means that the prior 95 % equal tail credible intervals are between −20 and 20. For the binary response variable lockdown, we use binary logit regression with the same linear predictor.
We used the Stan library (Stan Development Team, 2011-2019) rstan7 in RStudio8, for analysis. All the simulation chains converged and mixed (all Rhat = 1).
2.7.2 Sentiment analysis for the open-ended questions
Along with the statistical results from the Likert scale questions, we were also interested in a deeper understanding using sentiment analysis of the texts in the open-ended questions. In previous research (Slater et al., 2022b), this approach resulted in unexpected findings that would never have been found from the standard questionnaire responses alone. Sentiment analysis (Liu, 2012; Bakshi et al., 2016) is based on a multitude of classifications of words in dictionaries that have been assigned positive or negative valence through the use of natural language processing, text analysis, and computational linguistics. A score is derived for each piece of text, for example, as the average score over all the relevant words in the text, though modified by natural language analysis to take negations into account.
We adopted the same strategy as Slater et al. (2022b). Four different sentiment analysis methods were used that were available through the R statistical computing language. The R package sentimentr (Rinker, 2021) uses nine dictionaries and specializes in “valence shifters,” that is, modifiers where “I do not like it” is correctly recognized as negative and “I really like it” has greater positive valence than “I like it.” Rinker (2021)9 evaluated several sentiment packages. The VADER system (Hutto and Gilbert, 2014) was used originally for evaluating text from social media but can also be used generally. We used the R implementation10 by Katherine Roehrick. The syuzhet package (Jockers, 2017)11 was originally proposed for the analysis of the latent structure in narrative, although has also been used more generally. Finally, the fourth R package we used was SentimentAnalysis12 (Feuerriegel et al., 2018; Feuerriegel and Proellochs, 2019), which has been used for the analysis of financial text, but again has also been used more widely. See also Yoon et al. (2017) and Naldi (2019) for a wider discussion on sentiment analysis packages.
For each individual, we combined the texts for choice and overall shown in Table 2 since these answers included the participants’ opinions about their experience, whereas technique simply described the method. Hence, there are n = 47 texts. The four sentiment methods were applied to each, resulting in an
A principal component analysis was carried out on the
where
The non-informative prior distributions for
3 Results
3.1 Participant statistics
Detailed statistics about the participants and their distribution by conditions are given in Table 1. This shows that the two groups, WiP and walking, are similar over a range of characteristics: the use of Meta Quests 1 and 2, age, gender, level of past VR experience, and others that are shown in the first column of Table 1.
3.2 Analysis of the main questionnaire responses
The models described in Section 2.6.1 were applied to the response variables in Table 2, and summaries of the resulting posterior distributions are shown in Table 3. The variables sex, age, and Quest are only included to remove their effects and are not of intrinsic interest.
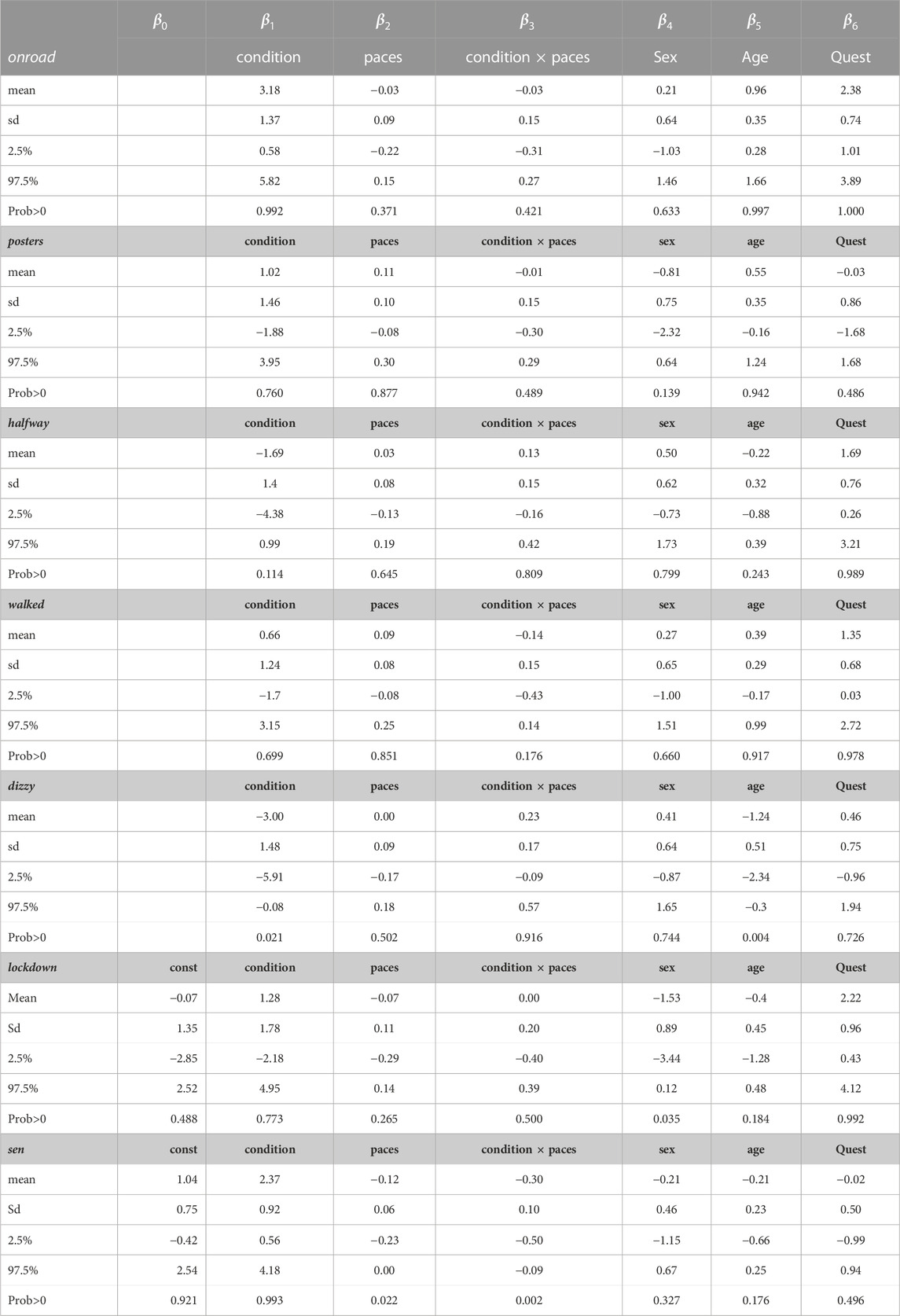
TABLE 3. Summaries of the posterior distributions, showing for each distribution its mean and standard deviation, 95% credible interval, and the posterior probability of the parameter being positive (Prob >0). For the parameter
The variables onroad and walked relate to presence, the illusions of being there (place illusion), and that the events were actually happening (plausibility) (Slater, 2009). The questions poster and halfway were included as additional possible measures of plausibility. It was thought that greater plausibility would lead to people avoiding stepping on the pictures of the faces of the movie stars, and halfway along the road, there was a small barrier that if it were in physical reality, people would need to step over.
Figure 2A includes the box plots for the presence variables. For onroad in Table 3, the posterior probability that the coefficient of the condition walking (
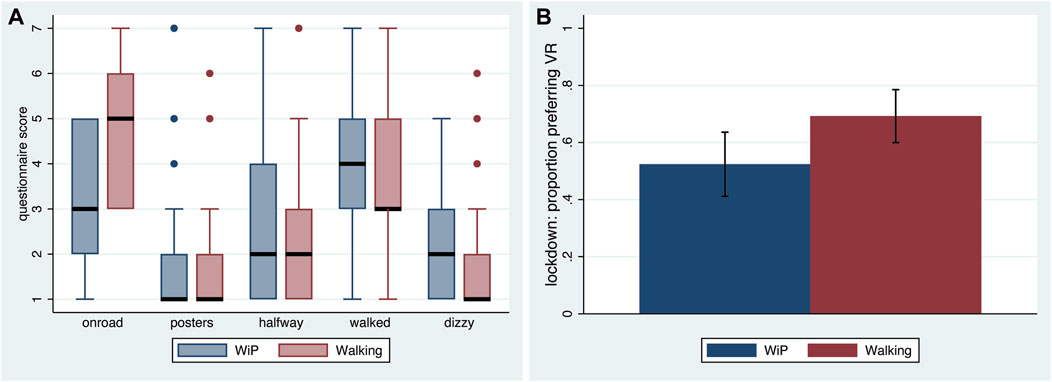
FIGURE 2. Box plots of the presence-related questions shown in Table 2, the dizziness and the lockdown question, by conditions. (A) Presence and dizziness questions. The thick horizontal lines are the medians. The boxes are the interquartile range. The whiskers range from max (lower quartile −1.5*IQR, min value) to min (upper quartile +1.5*IQR, max value). (B) Lockdown question, where the box heights are proportions and the whiskers are standard errors.
The number of paces that participants could walk in the Quest guardian could influence responses in various ways, ranging from, on the positive side, more of a feeling of having walked some distance without any barriers, to on the negative side, tiredness and dizziness. Hence, it was included as a covariate in the model (Eq. 1). The response variable posters is positively associated with paces (prob = 0.877), but there is little evidence that it is associated with the condition.
The variable halfway had a negative main effect for the condition (prob = 1–0.114 = 0.886), but there were no other notable effects (Figure 3A).
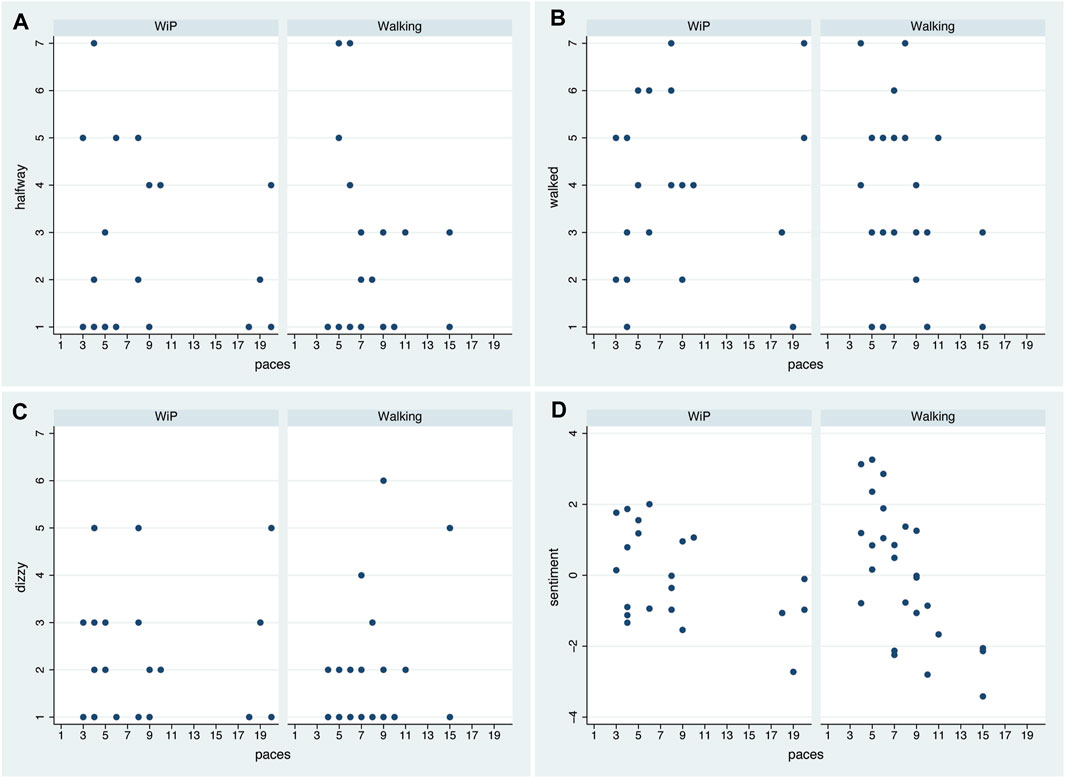
FIGURE 3. Scatter diagrams of response variables on paces by conditions. (A) Halfway, (B) walked, (C) dizzy, and (D) sentiment.
For walked, there was a small positive relationship with paces in the WiP condition (prob = 0.851), but the slope was negative for walking (prob = 1–0.176 = 0.824) (Figure 3B). For dizzy, although there was a strong negative main effect on walking (prob = 1–0.021 = 0.979), the interaction effect was also positive so that in the walking condition, the greater the number of paces, the greater the reported dizziness (Figure 3C, prob = 0.916).
The bar chart for lockdown (Figure 2B) suggests that people in the walking condition were more likely to prefer using VR for walking around compared to just walking around their home in periods of lockdown. However, although that reflects the raw observed data, the effect vanishes when the other variables in the linear predictor are taken into account. There were no main effects for condition or paces for lockdown, but female participants were less likely to prefer VR than male participants (prob = 1–0.035 = 0.965), and those with a Quest 2 were more likely to prefer VR than those with a Quest 1 (prob = 0.992).
To summarize, presence in the sense of “being there,” as represented by onroad, was greater in the walking condition, irrespective of the size of the guardian. Avoidance of stepping over the posters was positively associated with the size of the guardian, irrespective of the condition. In the WiP condition, the size of the guardian was positively associated with the sensation of having walked, but in the walking condition, the slope of this relationship was reduced—the further they walked, the less there was the feeling of walking. This is probably associated with the finding that in the walking condition, but not in the WiP condition, the greater the size of the guardian, the greater the dizziness.
3.3 Analysis of the open-ended questions
Figure 3D shows that both for the WiP and the walking conditions, there was a negative association between the size of the guardian and sentiment—the greater the size of the guardian, the lower the sentiment, and more so for those in the walking condition. This is shown in Table 3, where the probability of the negative slope is 1–0.022 = 0.978 for the WiP condition and 1–0.002 = 0.998 for the interaction term. Figure 4A, which brings together the two parts of Figure 3D, clearly shows that there is higher sentiment for the walking condition for a small number of paces, and then it switches to higher sentiment for the WiP condition. This difference has strong evidence, shown by the posterior distributions of the two slopes in Figure 4B. For example, the posterior probability that the slope < −0.2 is 0.071 for the WiP condition and 0.988 for the walking condition, and the 95% equal tail credible intervals are WiP: (−0.230 to −0.004) and walking: (−0.600 to −0.228).
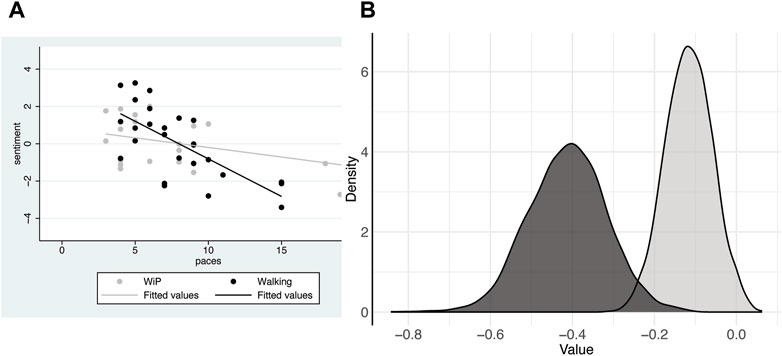
FIGURE 4. Slopes of sentiment on paces by conditions. (A) Scatter diagram and fitted regression lines. (B) Posterior distributions of the slope parameters. The light gray corresponds to WiP, and the dark gray corresponds to walking.
3.4 Interpretations of the sentiment scores
Next, we interpret the sentiment scores. Recall that four sentiment methods were used to derive the sentiments of the combined texts that were answers to the questions of choice and overall in Table 2. Figures 5A–D show the distributions. The VADER method (Figure 5B) stands out as having a different distribution from the other three methods, being reverse J-shaped, whereas the others were approximately symmetrical about a central value. The differences between the scores are useful since it means that the different methods do not respond to the texts in the same way, justifying using four methods rather than one.
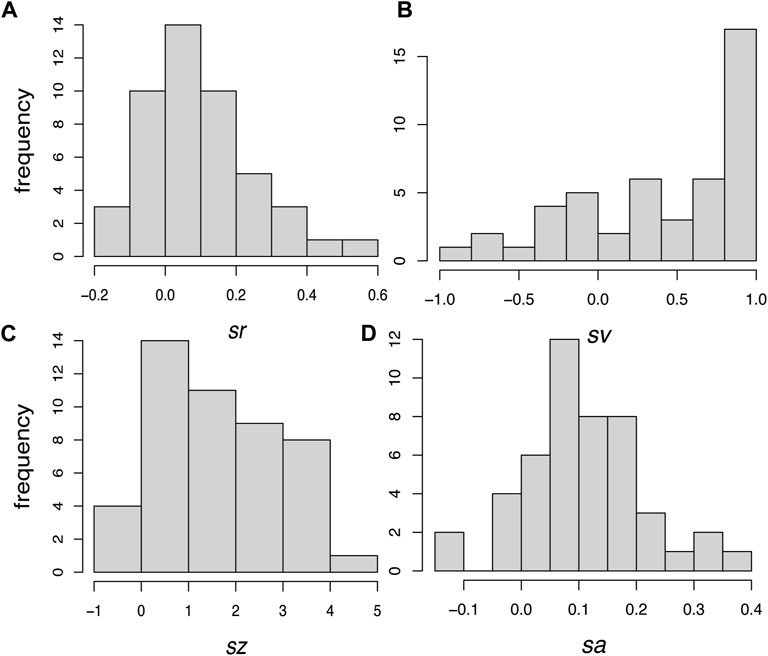
FIGURE 5. Histograms of the sentiment scores using the different sentiment methods. (A) (sr) sentimentr, (B) (sv) VADER, (C) (sz) syuzhet, and (D) (sa) SentimentAnalysis.
K-means clustering (using kmeans in R) was used to cluster the standardized matrix of sentiment scores by individuals. We used two clusters to maintain separation between them and to obtain approximately the same sizes. There were 22 points in cluster 1 and 25 points in cluster 2. An efficient way to display the clusters is to find the principal components (PCs) of the four standardized (mean 0, variance 1) sentiment scores and plot the scores per individual of the first two PCs. To achieve this, the R package factoextra13 (Kassambara, 2017; Kassambara and Mundt, 2017) that includes the function fviz_cluster was used. Figure 6 shows the clusters plotted on the first two PCs. The first PC accounts for 67% of the total variance, and the second PC accounts for 16%.
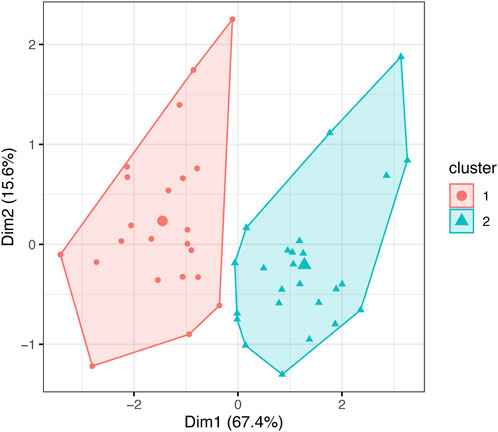
FIGURE 6. First two principal components (Dim1 and Dim2) of the
Table 4 shows the factor loadings for the first two principal components. The first factor loading shows that the scores of the first principal component are approximately the average of the results of the four methods. The second factor loading is dominated by sa and negatively associated with sv and sz. Figure 6 shows that most of the variation is along the first principal component, with cluster 1 having lower sentiment scores than cluster 2.

TABLE 4. Factor loadings for the first two principal components (PC1 and PC2) and their correlations with the original sentiment variables. All significance levels for the correlations are extremely small.
We can summarize the text associated with the two clusters using a keyword extraction technique and sentence summaries. We used the LexRank (Erkan and Radev, 2004)14 method to summarize the text in the clusters. The results are shown in Table 5, choosing the first 10 summaries. The statements in the lower sentiment column are more concerned with the lack of realism and discomfort of the method, especially in the WiP condition. The higher sentiment column statements are concerned more with interest and the experience itself, even for those in the WiP condition. This leads to a consideration of the cross between (WiP, walking) × (lower sentiment, higher sentiment).
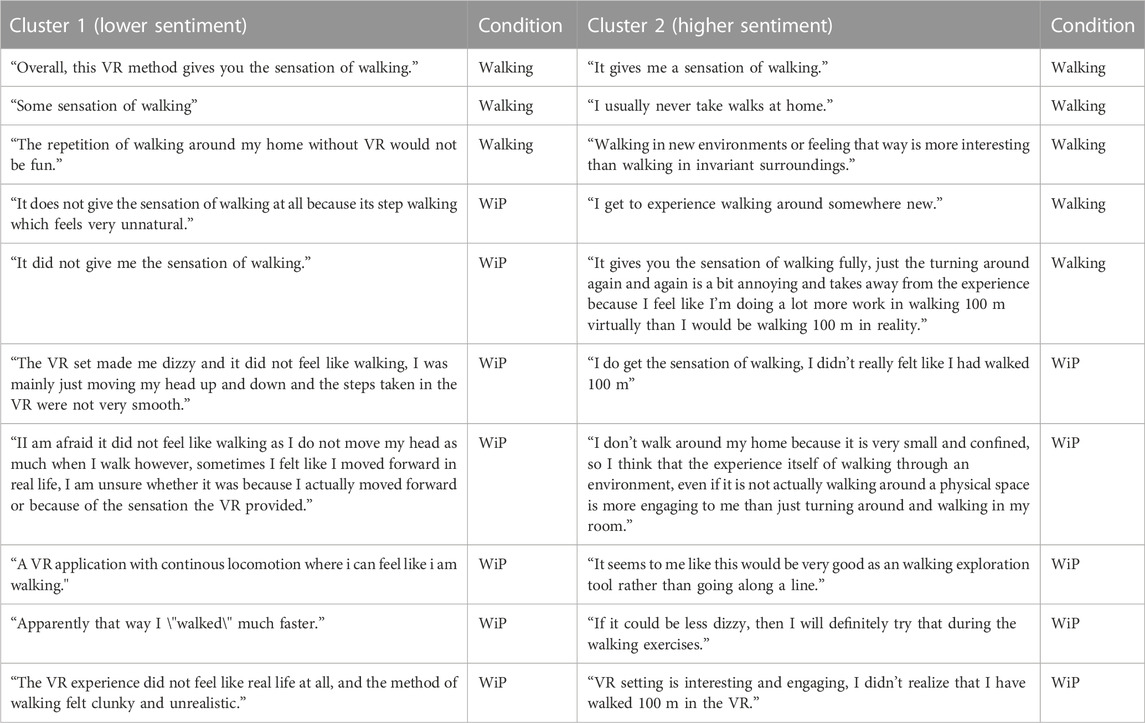
TABLE 5. First 10 summary sentences selected by the LexRank method. Each column has been sorted by condition. The original text written by participants has not been modified.
Table 6 shows the first five selected statements for each of these four possibilities. Comparing (WiP, lower sentiment) with (walking, higher sentiment), in the first group most of the statements are concerned with the fact that the experience did not feel like walking, and in the second group most of the statements are concerned with the positive benefits of the method. Those in the WiP group with higher sentiment concentrated on the fact that it felt like walking around even though in the home. Those in the walking group with lower sentiment concentrated more on the disadvantages of the method such as having to frequently turn, although it gave the sensation of walking. Overall, (lower sentiment, WiP) predominantly emphasized the unnatural feeling of the method. The issue of dizziness was mentioned, and that the virtual movement did not seem like the real sensation of walking. (Lower sentiment, walking) was slightly more positive; although it referred to the sensation of walking, it also pointed out the interruptions due to turning as a distraction. (Higher sentiment, WiP) expressed a preference for the VR environment over walking in confined real-world spaces, even mentioning that the method felt more like jogging. Finally, (higher sentiment, walking) expressed the utility of VR, especially for those confined to smaller spaces in reality.
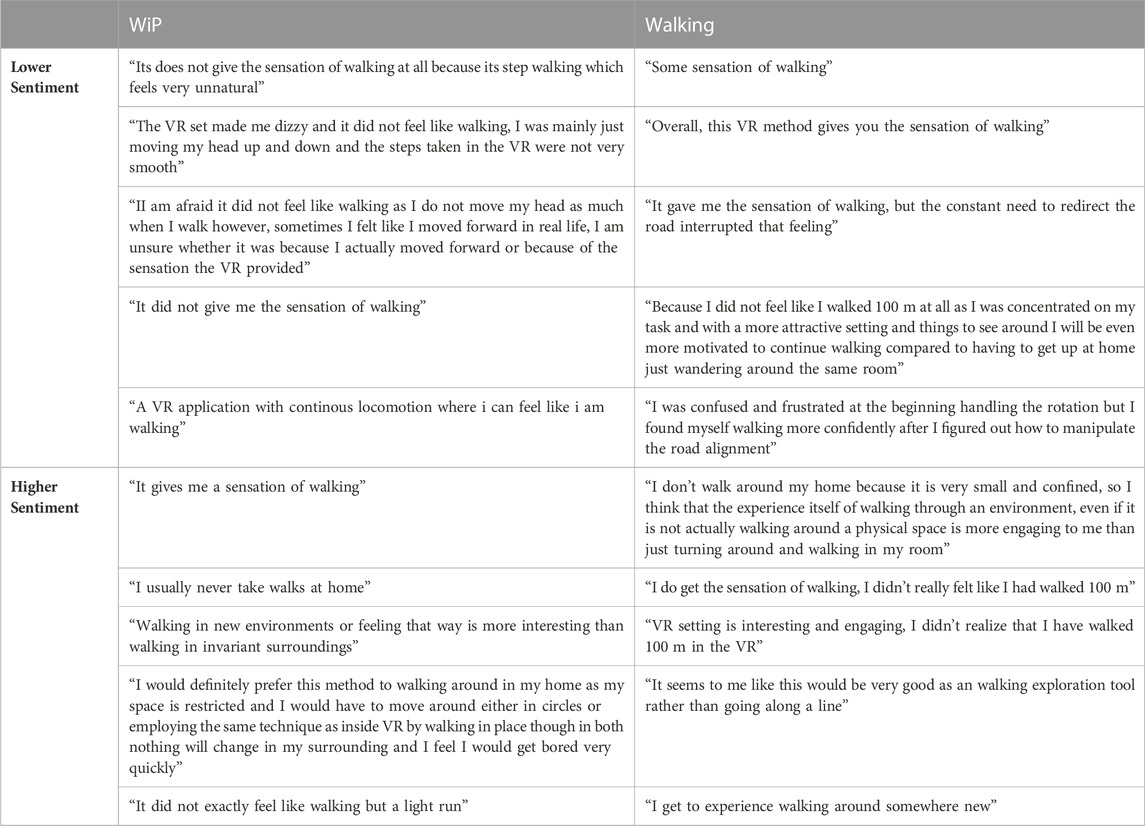
TABLE 6. First five summary sentences selected by the LexRank method by sentiment levels and conditions. The original text written by participants has not been modified.
4 Discussion
There are several findings and observations that can be drawn from this experiment. The first is that the walking method is unconditionally associated with a higher level of the sense of being there (the feeling of being on the road) compared with the WiP method. However, for plausibility (walked), the evidence suggests that the greater the size of the guardian, the greater the plausibility for WiP and the lower the plausibility for walking. Moreover, sentiment follows a similar pattern to walked, though with stronger statistical support—the greater the size of the guardian, the higher the sentiment in the WiP condition, but the lower the sentiment in the walking condition. Hence, smaller guardian sizes favor the walking condition, and larger sizes favor WiP. This is associated with the similar finding that although overall there is less dizziness in the walking condition, the greater the guardian size, the greater the dizziness, whereas there seems to be no effect of guardian size in the WiP condition. Therefore, greater guardian size is associated with lower plausibility and sentiment in the walking condition compared with WiP and greater dizziness, and conversely, smaller guardian sizes favor the walking condition with respect to these aspects.
Second, lower sentiment is associated, irrespective of method, with more discomfort, and higher sentiment with the feeling of walking and the overall utility of VR in the context of the scenario (walking around during confinement). The clearest contrast is between lower sentiment and WiP (dizziness and unlike walking) and higher sentiment and walking (it is like walking and interesting). Hence, an overall conclusion that would need to be confirmed by further studies, since the present study was exploratory, is that for smaller spaces, the walking method proposed here would be preferable.
Redirected walking is an excellent technique in principle, involving no conscious knowledge by participants that it is operating behind the scenes. However, Azmandian et al. (2015) found a minimum space requirement of 6 m × 6 m for its optimal operation. Messinger et al. (2019) discussed how a variety of differently shaped and sized and irregular tracking areas impact the performance of redirected walking. Kim et al. (2022) also discussed virtual room size, objects within it, and the height of the participant in determining the operation of redirected walking. Hwang et al. (2022) introduced a method for walking in virtual indoor environments in an “infinite” space, although there were certain requirements such as the physical room being rectangular, and the method does not allow environments to have a virtual rectangular room that does not fit entirely inside the real space, although there can be infinitely many such virtual rooms. Figure 4A suggests that the number of paces where walking is preferred to, or not worse than, WiP is up to approximately six or seven, which is equivalent to approximately 5 m for an adult. Moreover, the interactive method does not require a square shape but only a linear path wide enough for people to turn while remaining within the guardian. Comprehensive recent reviews of redirected walking can be found in Nilsson et al. (2018), Li et al. (2022), and Fan et al. (2023).
In spaces larger than approximately 6 m × 6 m, redirected walking would be preferable to our interactive walking method since people only walk and there is no interaction with the guardian. However, participants seemed to favor WiP for the larger spaces compared to walking. Kim and Rhiu (2021) compared three different methods of locomotion: joystick, teleportation, and WiP. They found that WiP resulted in the slowest speeds, and the teleportation interface was the fastest but had the highest number of errors (both not surprising). WiP and joystick had the highest sense of presence, likely because participants lost their sense of their surroundings with teleportation due to the instant movement across the space. WiP had the greatest mental workload and required the most physical effort. Simulator sickness overall was low but was highest with WiP. Qualitative results indicated that WiP resulted in greater presence, realism, immersion, naturalness, and ecological validity. With regard to WiP, the authors argued that it was not recommended on grounds of safety (simulator sickness and people afraid of bumping into physical objects—even though they do not walk, there is typically a non-conscious location drift). However, WiP was recommended as superior for the formation of cognitive maps and spatial knowledge. It seems that some involvement of the whole body is preferable for locomotion rather than using a joystick alone. Riecke et al. (2010) found that enhancing joystick navigation with physical turning (i.e., using the joystick to traverse distances but turning the body physically for turns) could be as good as really walking in some circumstances.
As pointed out previously, real walking is somewhat advantageous compared to WiP (Usoh et al., 1999), and this result was replicated by Peck et al. (2011). However, Brotons-Mas et al. (2006) suggested that any technique that actually mobilizes the body, such as walking for navigation or WiP, can significantly enhance the formation of cognitive maps of the environment, although actual walking would be the most ideal. Langbehn et al. (2018), for example, showed that redirected walking was superior to joystick navigation and teleportation with respect to the formation of cognitive maps. This confirms the previous findings of Ruddle et al. (2013).
Sousa et al. (2019) combined real walking with WiP—a participant could walk around a real place but use WiP to go further than any boundaries or obstacles. Conversely, in a study about agency that required significant movement across space, Gorisse et al. (2021) combined teleportation with real walking—teleportation to traverse the distance to a target and real walking around the location of the target. These may be regarded as hybrid methods. In the context of redirected walking, Lee et al. (2023) introduced a method called user-centered redirected walking that employs virtual “feelers” attached to the participant when potential obstacles in the real world are detected. In that case, the method subtly redirects the user away from them. This avoids some of the complexities of redirected walking implementation, and a user study demonstrated that participants were enabled to stay within the physical boundaries with no adverse effects on simulator sickness or efficiency.
Overall, the hypothesis we would put forward for further study is that in small and cluttered spaces, the interactive walking-in-place method that we have introduced should be used in order to give people the feeling of walking and for them to have a positive sentiment. For larger spaces, redirected walking should be used. Where these are not possible, for example, when there are multiple obstacles, then WiP should be used. If WiP cannot be used, for example, if the participant is unable to physically carry out the actions, then teleportation would minimize the likelihood of simulator sickness, although it would also reduce the chance of building a cognitive map, whereas a joystick method would have a greater chance of building a cognitive map but with an increased chance of simulator sickness. Simulator sickness can be modulated by very slow movement or a method such as limiting the field of view (Fernandes and Feiner, 2016). Future studies should also consider the specific content of the VR experience itself.
A limitation to our study is that the setup used a simple virtual environment with minimal graphics and no distractions, where participants were tasked with walking to the end of a straight road without speed instructions. It is worth noting that participant preferences and recommended locomotion techniques may vary based on the nature of the VR experience, such as exercising or playing a first-person shooter game versus a leisurely walk in a natural setting, and studies should address how these experiences relate to locomotion techniques and the physical space. Moreover, participants only walked in a straight line without any turns due to the layout of the environment. The WiP method could also be used to walk in any direction, for example, by basing the direction of the movement as normal to the frontal plane of the body or simply based on gaze direction. The walking method would allow participants to walk in arbitrary spaces by making use of the rotations, always lining up the guardian with what would be required by the turn. We intend to investigate this technique for more complex spaces.
Finally, the experiment showed that it is possible to build quite sophisticated interactive applications only using WebVR tools. These are relatively straightforward to use but, most importantly, are ideal for the end user—they do not have to download anything; they only have to enter a browser and type in a URL. This is very simple for remote participants of an experimental study. There has been growing interest in running experiments in the “wild” (Steed et al., 2016; Mottelson et al., 2021; Radiah et al., 2021; Loetscher et al., 2023), enhanced by the pandemic. However, researchers must always be certain that participants have actually participated rather than only completed questionnaires for monetary gain.
Data availability statement
The original contributions presented in the study are publicly available. This data can be found here: Doi: 10.34740/KAGGLE/DSV/6465876. The data and R and Stan programs can be found on https://www.kaggle.com/code/melslater/walking-code.
Ethics statement
The studies involving humans were approved by the Institutional Review Boards of Comissió de Bioètica, Universitat de Barcelona (IRB00003099), and NYUAD (HRPP-2022-194). The studies were conducted in accordance with the local legislation and institutional requirements. The participants provided their written informed consent to participate in this study. Written informed consent was obtained from the individual(s) for the publication of any potentially identifiable images or data included in this article.
Author contributions
DB: data curation, investigation, methodology, resources, visualization, and writing–review and editing. MS: conceptualization, formal analysis, funding acquisition, methodology, project administration, software, supervision, validation, writing–original draft, and writing–review and editing.
Funding
The author(s) declare that financial support was received for the research, authorship, and/or publication of this article. This work was financially supported by the European Research Council (ERC) Advanced Grant MoTIVE (Moments in Time in Immersive Virtual Environments) (#742989).
Acknowledgments
The authors would like to thank Jexi Lo for helping with recruitment at NYUAD and the setup of the laboratory experiment.
Conflict of interest
The authors declare that the research was conducted in the absence of any commercial or financial relationships that could be construed as a potential conflict of interest.
The author(s) declared that they were an editorial board member of Frontiers, at the time of submission. This had no impact on the peer review process and the final decision.
Publisher’s note
All claims expressed in this article are solely those of the authors and do not necessarily represent those of their affiliated organizations, or those of the publisher, the editors, and the reviewers. Any product that may be evaluated in this article, or claim that may be made by its manufacturer, is not guaranteed or endorsed by the publisher.
Supplementary material
The Supplementary Material for this article can be found online at: https://www.frontiersin.org/articles/10.3389/frvir.2023.1294539/full#supplementary-material
Supplementary Video S1 | Illustration of the two methods of walking in VR.
Footnotes
3It turns out that there is a more direct way to find out if a user is in XR mode on the device using AFrame. see https://stackoverflow.com/questions/65957141/how-to-find-when-xr-session-startsthree-js-webxr.
5https://www.ub.edu/eventlab/WiP
6https://www.ub.edu/eventlab/Rotations
7https://mc-stan.org/users/interfaces/rstan)
9https://github.com/trinker/sentimentr#comparing-sentimentr-syuzhet-meanr-and-stanford
10https://CRAN.R-project.org/package=vader
11https://github.com/mjockers/syuzhet
12https://CRAN.R-project.org/package=SentimentAnalysis
13https://cran.r-project.org/web/packages/factoextra/index.html
14https://www.rdocumentation.org/packages/lexRankr/versions/0.5.2/topics/lexRank
References
Azmandian, M., Grechkin, T., Bolas, M. T., and Suma, E. A. (2015). “Physical space requirements for redirected walking: how size and shape affect performance,” in ICAT-EGVE), 93–100.
Bakshi, R. K., Kaur, N., Kaur, R., and Kaur, G. (2016). “Opinion mining and sentiment analysis,” in 2016 3rd International Conference on Computing for Sustainable Global Development (INDIACom): IEEE), 452–455.
Boletsis, C. (2017). The new era of virtual reality locomotion: a systematic literature review of techniques and a proposed typology. Multimodal Technol. Interact. 1, 24. doi:10.3390/mti1040024
Bozgeyikli, E., Raij, A., Katkoori, S., and Dubey, R. (2016). “Point & teleport locomotion technique for virtual reality,” in Proceedings of the 2016 annual symposium on computer-human interaction in play), 205–216.
Brotons-Mas, J. R., O'mara, S., and Sanchez-Vives, M. V. (2006). Neural processing of spatial information: what we know about place cells and what they can tell us about presence. Presence-Teleoperators Virtual Environ. 15, 485–499. doi:10.1162/pres.15.5.485
Cherni, H., Métayer, N., and Souliman, N. (2020). Literature review of locomotion techniques in virtual reality. Int. J. Virtual Real. 20, 1–20. doi:10.20870/ijvr.2020.20.1.3183
Darken, R. P., Allard, T., and Achille, L. B. (1999). Spatial orientation and wayfinding in large-scale virtual spaces II. Presence Teleoperators Virtual Environ. 8, 3–6. doi:10.1162/105474699566495
Darken, R. P., and Sibert, J. L. (1996). “Wayfinding strategies and behaviors in large virtual worlds,” in Proceedings of the SIGCHI conference on Human factors in computing systems: common ground, 142–149.
Erkan, G., and Radev, D. R. (2004). Lexrank: graph-based lexical centrality as salience in text summarization. J. Artif. Intell. Res. 22, 457–479. doi:10.1613/jair.1523
Fairchild, K. M., Lee, B. H., Loo, J., Ng, H., and Serra, L. (1993). “The heaven and earth virtual reality: designing applications for novice users,” in Virtual Reality Annual International Symposium, 1993 (IEEE), 47–53.
Fan, L., Li, H., and Shi, M. (2023). Redirected walking for exploring immersive virtual spaces with HMD: a comprehensive review and recent advances. IEEE Trans. Vis. Comput. Graph. 29, 4104–4123. doi:10.1109/tvcg.2022.3179269
Fernandes, A. S., and Feiner, S. K. (2016). “Combating VR sickness through subtle dynamic field-of-view modification,” in 2016 IEEE Symposium on 3D User Interfaces (3DUI): IEEE), 201–210.
Feuerriegel, S., Proellochs, N., and Feuerriegel, M. S. (2018). Package ‘SentimentAnalysis’. London, UK: CRAN.
Feuerriegel, S., and Proellochs, N. (2019). SentimentAnalysis vignette. Massachusetts Institute of Technology. Available at: https://mran.microsoft.com/snapshot/2017-08-06/web/packages/SentimentAnalysis/vignettes/SentimentAnalysis.html.
Fuentes-Pacheco, J., Ruiz-Ascencio, J., and Rendón-Mancha, J. M. (2015). Visual simultaneous localization and mapping: a survey. Artif. Intell. Rev. 43, 55–81. doi:10.1007/s10462-012-9365-8
Gorisse, G., Senel, G., Banakou, D., Beacco, A., Oliva, R., Freeman, D., et al. (2021). Self-observation of a virtual body-double engaged in social interaction reduces persecutory thoughts. Sci. Rep. 11, 23923. doi:10.1038/s41598-021-03373-x
Hanson, S., Paris, R. A., Adams, H. A., and Bodenheimer, B. (2019). “Improving walking in place methods with individualization and deep networks,” in 2019 IEEE Conference on Virtual Reality and 3D User Interfaces (VR): IEEE), 367–376.
Held, R. M., and Durlach, N. I. (1992). Telepresence. Presence Teleoperators Virtual Environ. 1, 109–112. doi:10.1162/pres.1992.1.1.109
Hutto, C., and Gilbert, E. (2014). “Vader: a parsimonious rule-based model for sentiment analysis of social media text,” in Proceedings of the International AAAI Conference on Web and Social Media).
Hwang, J.-Y., Kwon, S.-U., Cho, Y.-H., Jeon, S.-B., and Lee, I.-K. (2022). Redirected walking in infinite virtual indoor environment using change-blindness. arXiv preprint arXiv:2212.13733.
Jockers, M. (2017). Package ‘syuzhet. Available at: https://cran.r-project.org/web/packages/syuzhet.
Kassambara, A., and Mundt, F. (2017). Package ‘factoextra’. Extract and visualize the results of multivariate data analyses 76.
Kassambara, A. (2017). Practical guide to principal component methods in R: PCA, M (CA), FAMD, MFA, HCPC, factoextra. Sthda.
Kim, D., Kim, J., Shin, J.-E., Yoon, B., Lee, J., and Woo, W. (2022). “Effects of virtual room size and objects on relative translation gain thresholds in redirected walking,” in 2022 IEEE conference on virtual reality and 3D user interfaces (VR): IEEE), 379–388.
Kim, Y. M., and Rhiu, I. (2021). A comparative study of navigation interfaces in virtual reality environments: a mixed-method approach. Appl. Ergon. 96, 103482. doi:10.1016/j.apergo.2021.103482
Langbehn, E., Lubos, P., and Steinicke, F. (2018). “Evaluation of locomotion techniques for room-scale vr: joystick, teleportation, and redirected walking,” in Proceedings of the Virtual Reality International Conference-Laval Virtual), 1–9.
Lee, C.-G., Kang, D., Hwang, S., and Kwon, O. (2023). User-centered redirected walking and resetting with virtual feelers. Virtual Real. 27, 717–734. doi:10.1007/s10055-022-00682-y
Leeb, R., Keinrath, C., Friedman, D., Guger, C., Scherer, R., Neuper, C., et al. (2006). Walking by thinking:the brainwaves are crucial, not the muscles. Presence-Teleoperators Virtual Environ. 15, 500–514. doi:10.1162/pres.15.5.500
Li, Y.-J., Steinicke, F., and Wang, M. (2022). A comprehensive review of redirected walking techniques: taxonomy, methods, and future directions. J. Comput. Sci. Technol. 37, 561–583. doi:10.1007/s11390-022-2266-7
Liu, B. (2012). Sentiment analysis and opinion mining. Synthesis Lect. Hum. Lang. Technol. 5, 1–167. doi:10.2200/s00416ed1v01y201204hlt016
Loetscher, T., Jurkovic, N. S., Michalski, S. C., Billinghurst, M., and Lee, G. (2023). Online platforms for remote immersive Virtual Reality testing: an emerging tool for experimental behavioral research. Multimodal Technol. Interact. 7, 32. doi:10.3390/mti7030032
Messinger, J., Hodgson, E., and Bachmann, E. R. (2019). Effects of tracking area shape and size on artificial potential field redirected walking, in: 2019 IEEE Conference on Virtual Reality and 3D User Interfaces (VR): IEEE), 72–80.
Mottelson, A., Petersen, G. B., Lilija, K., and Makransky, G. (2021). Conducting unsupervised virtual reality user studies online. Front. Virtual Real. 2, 681482. doi:10.3389/frvir.2021.681482
Naldi, M. (2019). A review of sentiment computation methods with R packages. arXiv preprint arXiv:1901.08319.
Nilsson, N. C., Peck, T., Bruder, G., Hodgson, E., Serafin, S., Whitton, M., et al. (2018). 15 years of research on redirected walking in immersive virtual environments. IEEE Comput. Graph. Appl. 38, 44–56. doi:10.1109/mcg.2018.111125628
Peck, T. C., Fuchs, H., and Whitton, M. C. (2011). The design and evaluation of a large-scale real-walking locomotion interface. IEEE Trans. Vis. Comput. Graph. 18, 1053–1067. doi:10.1109/tvcg.2011.289
Pfurtscheller, G., Leeb, R., Keinrath, C., Friedman, D., Neuper, C., Guger, C., et al. (2006). Walking from thought. Brain Res. 1071, 145–152. doi:10.1016/j.brainres.2005.11.083
Prithul, A., Adhanom, I. B., and Folmer, E. (2021). Teleportation in virtual reality; A mini-review. Front. Virtual Real. 2, 730792. doi:10.3389/frvir.2021.730792
Radiah, R., Mäkelä, V., Prange, S., Rodriguez, S. D., Piening, R., Zhou, Y., et al. (2021). Remote VR studies: a framework for running virtual reality studies remotely via participant-owned HMDs. ACM Trans. Computer-Human Interact. (TOCHI) 28, 1–36. doi:10.1145/3472617
Razzaque, S., Kohn, Z., and Whitton, M. C. (2001). Redirected walking. Proc. Eurogr., 289–294. doi:10.2312/egs.20011036
Razzaque, S., Swapp, D., Slater, M., Whitton, M. C., and Steed, A. (2002). “Redirected walking in place,” in Proceedings of the workshop on Virtual environments 2002, 123–130.
Riecke, B. E., Bodenheimer, B., Mcnamara, T. P., Williams, B., Peng, P., and Feuereissen, D. (2010). “Do we need to walk for effective virtual reality navigation? physical rotations alone may suffice,” in International Conference on Spatial Cognition (Springer), 234–247.
Rinker, T. W. (2021). Sentimentr: calculate text polarity sentiment. version 2.9.0. Available at: https://github.com/trinker/sentimentr.
Ruddle, R. A., Volkova, E., and Bülthoff, H. H. (2013). Learning to walk in virtual reality. ACM Trans. Appl. Percept. (TAP) 10, 1–17. doi:10.1145/2465780.2465785
Sanchez-Vives, M. V., and Slater, M. (2005). From presence to consciousness through virtual reality. Nat. Rev. Neurosci. 6, 332–339. doi:10.1038/nrn1651
Sheridan, T. B. (1996). Further musings on the psychophysics of presence. Presence Teleoperators Virtual Environ. 5, 241–246. doi:10.1162/pres.1996.5.2.241
Sheridan, T. B. (1992). Musings on telepresence and virtual presence. Presence Teleoperators Virtual Environ. 1, 120–126. doi:10.1162/pres.1992.1.1.120
Slater, M., Banakou, D., Beacco, A., Gallego, J., Macia-Varela, F., and Oliva, R. (2022a). A separate reality: an update on place illusion and plausibility in virtual reality. Front. Virtual Real 3. doi:10.3389/frvir.2022.914392
Slater, M., Cabriera, C., Senel, G., Banakou, D., Beacco, A., Oliva, R., et al. (2022b). The sentiment of a virtual rock concert. Virtual Real. 27, 651–675. doi:10.1007/s10055-022-00685-9
Slater, M., and Sanchez-Vives, M. V. (2009). Place illusion and plausibility can lead to realistic behaviour in immersive virtual environments. Philos. Trans. R. Soc. Lond 364, 3549–3557. doi:10.1098/rstb.2009.0138
Slater, M., Steed, A., and Usoh, M. (1995a). “The virtual treadmill: a naturalistic metaphor for navigation in immersive virtual environments,” in Virtual Environments ’95. Editors M. A. Göbel (Vienna: Eurographics. Springer). doi:10.1007/978-3-7091-9433-1_12
Slater, M., Usoh, M., and Steed, A. (1995b). Taking steps: the influence of a walking technique on presence in virtual reality. ACM Trans. Comput.-Hum. Interact. 2, 201–219. doi:10.1145/210079.210084
Sousa, M., Mendes, D., and Jorge, J. (2019). Safe walking in vr using augmented virtuality. arXiv preprint arXiv:1911.13032.
Stanney, K. M., and Kennedy, R. S. (2009). “Simulation sickness,” in Human factors in simulation and training. Editors D. A. Vicenzi, J. A. Wise, M. Mouloua, and P. A. Hancock (Boca Raton, Florida: CRC Press), 117–127.
Steed, A., Friston, S., Lopez, M. M., Drummond, J., Pan, Y., and Swapp, D. (2016). An ‘in the Wild’Experiment on presence and embodiment using consumer virtual reality equipment. IEEE Trans. Vis. Comput. Graph. 22, 1406–1414. doi:10.1109/tvcg.2016.2518135
Steinicke, F., Bruder, G., Jerald, J., Frenz, H., and Lappe, M. (2009). Estimation of detection thresholds for redirected walking techniques. IEEE Trans. Vis. Comput. Graph. 16, 17–27. doi:10.1109/tvcg.2009.62
Stoakley, R., Conway, M. J., and Pausch, R. (1995). “Virtual reality on a WIM: interactive worlds in miniature,” in Proceedings of the SIGCHI conference on Human factors in computing systems), 265–272.
Usoh, M., Arthur, K., Whitton, M. C., Bastos, R., Steed, A., Slater, M., et al. (1999). “Walking > walking-in-place > flying, in virtual environments,” in Proceedings of the 26th annual conference on Computer graphics and interactive techniques (SIGGRAPH).
Keywords: virtual reality, locomotion, wayfinding, redirected walking, walking-in-place, navigation, room-scale virtual reality
Citation: Banakou D and Slater M (2023) A comparison of two methods for moving through a virtual environment: walking in place and interactive redirected walking. Front. Virtual Real. 4:1294539. doi: 10.3389/frvir.2023.1294539
Received: 14 September 2023; Accepted: 13 October 2023;
Published: 20 November 2023.
Edited by:
Daniel Thalmann, Swiss Federal Institute of Technology Lausanne, SwitzerlandReviewed by:
Stefan Marks, Auckland University of Technology, New ZealandGeorge Papagiannakis, University of Crete, Greece
Copyright © 2023 Banakou and Slater. This is an open-access article distributed under the terms of the Creative Commons Attribution License (CC BY). The use, distribution or reproduction in other forums is permitted, provided the original author(s) and the copyright owner(s) are credited and that the original publication in this journal is cited, in accordance with accepted academic practice. No use, distribution or reproduction is permitted which does not comply with these terms.
*Correspondence: Mel Slater, bWVsc2xhdGVyQHViLmVkdQ==