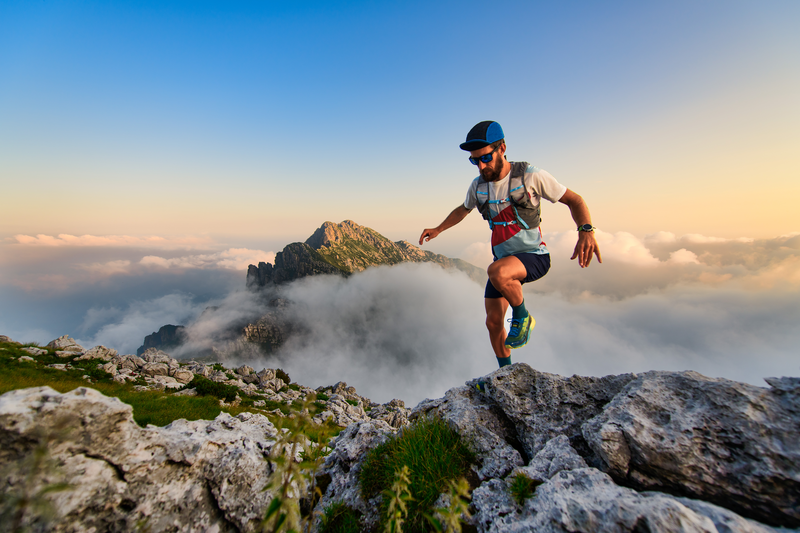
94% of researchers rate our articles as excellent or good
Learn more about the work of our research integrity team to safeguard the quality of each article we publish.
Find out more
REVIEW article
Front. Virtual Real. , 05 December 2023
Sec. Virtual Reality and Human Behaviour
Volume 4 - 2023 | https://doi.org/10.3389/frvir.2023.1141683
This article is part of the Research Topic Embodiment and Presence in Collaborative Mixed Reality View all 6 articles
The sense of embodiment refers to the sensations of being inside, having, and controlling a body. In virtual reality, it is possible to substitute a person’s body with a virtual body, referred to as an avatar. Modulations of the sense of embodiment through modifications of this avatar have perceptual and behavioural consequences on users that can influence the way users interact with the virtual environment. Therefore, it is essential to define metrics that enable a reliable assessment of the sense of embodiment in virtual reality to better understand its dimensions, the way they interact, and their influence on the quality of interaction in the virtual environment. In this review, we first introduce the current knowledge on the sense of embodiment, its dimensions (senses of agency, body ownership, and self-location), and how they relate the ones with the others. Then, we dive into the different methods currently used to assess the sense of embodiment, ranging from questionnaires to neurophysiological measures. We provide a critical analysis of the existing metrics, discussing their advantages and drawbacks in the context of virtual reality. Notably, we argue that real-time measures of embodiment, which are also specific and do not require double tasking, are the most relevant in the context of virtual reality. Electroencephalography seems a good candidate for the future if its drawbacks (such as its sensitivity to movement and practicality) are improved. While the perfect metric has yet to be identified if it exists, this work provides clues on which metric to choose depending on the context, which should hopefully contribute to better assessing and understanding the sense of embodiment in virtual reality.
The field of virtual reality (VR) is becoming more accessible to the public due to technological progress, leading to many applications such as entertainment (Zyda, 2005; Atkinson et al., 2020), interactive training (Claude et al., 2014; Bouville et al., 2015), scientific visualisation (Chandler et al., 2015; Dwyer et al., 2018), and virtual therapy (Wallach et al., 2009; Donker et al., 2019; Lindner, 2020). In most cases, virtual bodies (also referred to as avatars) are used in virtual environments (VEs) to represent users. In this way, it is essential to understand the sense of embodiment (SoE) [“the subjective experience of using and having a body” (Blanke and Metzinger, 2009)] in VR applications. Multiple communities study the SoE in their own way (Longo et al., 2008b; Blanke and Metzinger, 2009; de Vignemont, 2011; Kilteni et al., 2012a). For instance, in neuroscience, research focuses on understanding the neural correlates related to having a body and being in control (which underpins the concept of responsibility in human societies) (Haggard, 2017), while philosophy and cognitive science study the self and bodily self-consciousness (Gallagher, 2000).
Modulation of the SoE through modifications of the user’s avatar has been shown to have (at least short term) a perceptual and behavioural impact on the users’ SoE (Yee and Bailenson, 2007; Banakou et al., 2013; Kilteni et al., 2013). To better understand the SoE, relevant and reliable metrics of the phenomenon have to be defined. This is not an easy task as the SoE is a subjective feeling. Questionnaires have been widely used in the literature (Peck and Gonzalez-Franco, 2021), but other objective methods exist, such as proprioceptive drift (Botvinick and Cohen, 1998), response to threats (Armel and Ramachandran, 2003), or neuroimagery (Ehrsson et al., 2004; Sperduti et al., 2011). In this paper, we aim to review the current literature about the SoE and the existing assessment methods of this phenomenon in VR. The pros and cons of these assessment methods are depicted. We also provide a critical analysis of the existing measures to identify the most relevant measures in the context of VR studies.
This paper is structured as follows. Section 2 defines the SoE and its sub-components (with the help of previous literature), how the sub-components are linked, and how, in VR, the SoE towards an avatar impacts users’ behaviour and perception. Section 3 reviews the assessment methods found in the literature to measure the SoE, as well as suggestions on how to choose an assessment method. Finally, a discussion in Section 4 opens on the importance of having a real-time and reliable measure of the SoE and a recent discussion about the demanding effect [participants forming an interpretation of the experiment’s purpose and subconsciously changing their behaviour to match that interpretation (Orne, 2009)], before concluding in Section 5.
In this section, we define the SoE and how its sub-components interact together and review the perceptual and behavioural impacts of modulation of the SoE in VR.
In this paper, we focus on the VR community which studies the extent to which it is possible to feel towards a virtual body the same way we do towards our biological body (Kilteni et al., 2012a). While we usually do not question the feeling of owning our real body, except in the case of certain pathologies (Hassan and Josephs, 2016), VR offers unique opportunities to manipulate this feeling in controlled environments.
Based on the nomenclature proposed by Kilteni et al. (2012a), which is widely acknowledged in the VR community, we define the SoE as “the ensemble of sensations that arise in conjunction with being inside, having, and controlling a body, especially in relation to virtual reality applications.”
The SoE is characterised by three sub-components, defined as follows (Kilteni et al., 2012a). The sense of agency (SoA) is the feeling of being the cause of our actions. The sense of body ownership (SoBO) is the feeling that the virtual body is the source of our sensations (like our real body). Finally, the sense of self-location (SoSL) is the “spatial experience of being inside a body.” As detailed in Section 2.5, it is known that these three sub-components are not all independent of each other, but their relationship remains unclear.
Nevertheless, the definition of the SoE is still evolving, and new categories are continuously being suggested for the SoE, along with new assessment questionnaires (see Section 3.1).
In the following sections, we detail each component of the definition of the SoE, as proposed by Kilteni et al. (2012a).
The SoA is defined (across different communities) by statements like “I am the one in control of my actions” or “this is me who opened the door” (Braun et al., 2018). Gallagher (2000) proposed that the SoA is “the sense that I am the one who is causing or generating an action.” While this is related to the generation of an action, it is also linked to the outcome of the action in the environment, as Haggard and Chambon (2012) word it: “the experience of controlling one’s own actions, and, through them, events in the outside world.” One may, for instance, press a button to call an elevator, which will open the door, and in this way, one may feel an agent towards this action. Not only does one transform the environment with their action but also experience how one changed it. Kilteni et al. (2012a) followed the definition proposed by Blanke and Metzinger (2009), where the SoA refers to the sense of having “global motor control, including the subjective experience of action, control, intention, motor selection, and the conscious experience of will.”
The most commonly used theory to explain the SoA at a cognitive level is the comparator model (also called the central monitoring theory) (Frith, 1992; Blakemore et al., 1998; David et al., 2008). An action starts with an intention, followed by a prediction of the outcome of the motor command. Then, the motor command is performed, which provides a sensory feedback. Finally, the prediction is compared with the outcome to compute a prediction error. This prediction error allows three things: 1) to adjust the current motor command; 2) to attribute an agency towards the actions made (if the prediction error is null); and 3) to alleviate the sensations made by our own actions, which are predictable (see Figure 1).
FIGURE 1. Blakemore’s and Frith’s comparator model (also called the central monitoring theory) explaining the SoA. An action outcome is matched with a predicted outcome to attribute agency. Reproduced from by Braun et al. (2018), licensed under CC-BY 4.0.
However, this model has some limitations, as it fails to explain the feeling of agency in successful actions (Haggard, 2017), that is, when there is no prediction error. Indeed, it has been shown that even distorted action outcomes can be experienced as self-produced (Farrer et al., 2003). Moreover, people can attribute the same distorted feedback to their actions and sometimes to someone else’s actions (Farrer et al., 2003; Synofzik et al., 2008), which is not explained by the comparator model.
To account for these limitations, Synofzik et al. (2008) suggested an extension that not only takes into account the retrospective aspect of agency (inferring agency after the outcome is known, as explained by the comparator model) but also considers the prediction of the outcome of our actions. Indeed, the SoA is commonly separated into two components: the feeling of agency and the judgement of agency (JoA) (Farrer and Frith, 2002; Synofzik et al., 2008; Gallagher, 2012).
The feeling of agency relates to the intent to act. It is pre-reflective, implicit, low-level, and non-conceptual. It starts at the early stages of the action, before the perception of the feedback.
On the contrary, the JoA relates to the result of an action and occurs when we explicitly attribute agency to the self or other. It is reflective, explicit, high-order, and conceptual. The JoA is computed once the feedback has been perceived and processed (Synofzik et al., 2008). The JoA relies on three principles (Wegner and Wheatley, 1999): the priority principle, the consistency principle, and the exclusivity principle. The priority principle states that the thought should precede the action at a proper interval. According to the consistency principle, the thought should be coherent with the action. That is, the sensory outcome should match the predicted outcome. Finally, the exclusivity principle states that the thought should be the only apparent cause of action. In other words, there should not be any outside influence on the outcome.
In VR, the SoA is present when interacting with the VE. Indeed, the way users control their avatars changes the way they can interact with the VE and, thus, their level of immersion and their SoA (Slater, 2009). The experience will not be the same when using controllers to teleport their avatar or using full-body tracking to move themselves. Eubanks et al. (2021) found that having foot tracking significantly improves the SoA and the SoSL compared to head-and-hands tracking or no tracking at all. Moreover, Waltemate et al. (2016) observed that motor performance is affected by latency (induced by signal transmission and processing of tracking) when it is above 75 ms, and it also impacted negatively the SoA when the latency is higher than 125 ms. This echoes the priority principle defined by Wegner and Wheatley (1999), according to which the JoA is impacted if the action is too delayed from the thought of the action. Likewise, Jeunet et al. (2018) manipulated each of the three JoA principles in a VR experiment by introducing a biased sensory feedback (see Section 2.2.1.3). The priority principle was impacted by adding a visual latency in the hand tracking; the consistency principle was manipulated by inverting two fingers; and the exclusivity principle was impacted by having the avatar finger of the user to move by itself.
The SoBO is defined by statements like “this is my body” or “I am the one who is having this feeling” (Braun et al., 2018). The SoBO is, as Gallagher (2000) defines it, “one’s self attribution of a body.” This is the definition Kilteni et al. (2012a) and the VR community commonly follow. In some communities, there are references to the more general sense of ownership, which relates to ownership towards a body part (as opposed to the whole body) (Braun et al., 2018). However, in VR, the distinction is not always made and mostly refers to the SoBO. In this paper, we primarily focus on full-body avatars and, thus, aim to focus on the link between the self and the full body. In the following, we use the term SoBO even when only a body part is studied. A detailed review of the sense of ownership is provided in the work of Braun et al. (2018).
Many disciplines have investigated the conditions required for a SoBO to emerge, among which are phenomenology, philosophy of mind, and cognitive science (Tsakiris, 2010; de Vignemont, 2011; Braun et al., 2018). With the rubber hand illusion (RHI), Botvinick and Cohen (1998) showed that by synchronously stroking the (hidden) participant’s real hand and a rubber hand, an ownership illusion towards the rubber hand could be elicited. Each participant had their arm on a table, hidden behind an opaque screen, and a real-sized rubber hand was placed in front of them (see Figure 2). They were asked to look at the rubber hand while the experimenter brushed the real hand and the rubber hand. The experiment had two experimental conditions: the brush strokes on the real and virtual hands were either synchronous or asynchronous. The synchronous tactile stimulations resulted in participants having the feeling that the rubber hand was their own hand, which was not the case for the asynchronous tactile stimulations. Moreover, participants felt that their real hand “drifted” towards the rubber hand (see Section 2.2.3.2). The RHI was later expanded to VR (Lenggenhager et al., 2007) and generalised to full-body illusions (Slater et al., 2009). Kocur et al. (2022) found comparable effects to real-world RHI and validated the study of the RHI in VR. Some studies questioned if it was possible to induce a SoBO towards non-hand objects. Armel and Ramachandran (2003) reproduced the RHI towards the surface of a table, but the SoBO was reported to be lower than with the classical RHI. Ma and Hommel (2015) also demonstrated that a SoBO could be elicited towards a virtual balloon changing in size or a virtual square changing in size or colour. These findings can be explained by bottom–up influences (see the following) (Hohwy and Paton, 2010).
FIGURE 2. Classical rubber hand illusion, as originally introduced by Botvinick and Cohen (1998). The two hands (the participant’s real hand, hidden from view, and a rubber hand, visible to the participant) are stroked synchronously by the experimenter. Reproduced from by Braun et al. (2018), licensed under CC-BY 4.0.
To summarise, the SoBO seems to emerge from two processes: bottom–up influences (sensory information) and top–down influences (cognitive processes assuming an internal body map) (Tsakiris and Haggard, 2005; Tsakiris, 2010).
Bottom–up influences are enabled by afferent sensory information from one’s sensory organs, such as visual, tactile, and proprioceptive [the feeling of body position and movement (Tuthill and Azim, 2018)] inputs. Armel and Ramachandran (2003) measured the galvanic skin response (GSR) in an RHI experiment with either a rubber hand or only a table. They observed that the SoBO is significantly lower when the real hand is simultaneously visible during stroking, suggesting that the illusion is due to a spatiotemporal correlation between visual and tactile information. Thus, any object could be experienced as part of one’s body. According to the Bayesian perceptual learning theory (Hatfield, 1990; Armel and Ramachandran, 2003), the brain infers hidden causes from sensory signals [e.g., hearing a dog barking implies the existence of a dog (Kilteni et al., 2015)]. In this way, the RHI would work by merging the touch on the location of the rubber hand and the feeling of the touch on the real hand. Finally, Armel and Ramachandran (2003) stated that a visuo-tactile stimulus is necessary and sufficient to induce the RHI.
Top–down influences refer to all cognitive processes linked to the treatment of sensory signals and stimulations. The main top–down explanation of the SoBO is the neurocognitive model of SoBO, in which the SoBO is based on pre-existing internal body maps and is integrated from different information sources (Tsakiris, 2010). This neurocognitive model is a comparator model of the SoBO working with three levels: the first level opposes the visual appearance of an observed object to a pre-existing, temporally stable body model; on the second level, the current body schema state is compared to the anatomical, structural, and postural features of the observed object; and finally, the different sensory information about the observed object is matched in the third level, to give rise to a SoBO if coherent. This neurocognitive model processes each level successively. If there are enough similarities, the second level is processed until the third and last level. Regarding the top–down effects in experiments, it has been shown, for instance, that to induce ownership towards an external object, a basic morphological similarity with the real body part (or whole body) is needed (Armel and Ramachandran, 2003; Tsakiris and Haggard, 2005; Tsakiris et al., 2010a). However, according to Ma and Hommel (2015), the resemblance between the viewed object and the real hand is not as important as it seems.
Currently, it is unclear how these two processes (bottom–up and top–down influences) interact. Braun et al. (2018) stated that while there is a general agreement that our somatosensory input processing is structured by at least some internal body maps, the weight of top–down modulation remains unclear. Slater et al. (2010) showed in a VR experiment that bottom–up perceptual mechanisms can momentarily override top–down knowledge by altering the sense of one’s body with an illusion of temporary transformation in the form and size of the body.
The SoBO is influenced by the avatar representation (Lugrin et al., 2015a; Lugrin et al., 2015b). In VR, the avatar can represent anything from a realistic human (Latoschik et al., 2017) to a less realistic but human-like/anthropomorphic avatar (Lugrin et al., 2015a; Lugrin et al., 2015b) or even to non-human avatars (Ahn et al., 2016; Spangenberger et al., 2022). The avatar can vary in height, shape, colour, or rendering style (e.g., cartoon or photo-realistic). Lugrin et al. (2015a) observed that having an avatar resulted in a higher SoBO (in a virtual fitness training setup) compared to when having no avatar representation [similar results were found by Eubanks et al. (2021)]. In another work, Lugrin et al. (2015b) showed that it is possible to experience a SoBO with full-body avatars with different degrees of anthropomorphism (a block-man, a robot, or a human avatar). They observed that there could be an uncanny valley effect (Mori, 2012) when using human avatars. The authors explain it as a decrease in acceptance for those avatars due to subtle imperfections of appearance. Avatars can also be personalised to fit the users’ real appearance (for instance with 3D scans). Waltemate et al. (2018) showed that individualised 3D-scanned versions of participants significantly increase the SoBO, presence, and dominance (the perceived state of one’s own social dominance or submission). Finally, it appears that the closer an avatar is to the user’s real appearance, the better it is for the experienced SoE (Waltemate et al., 2018). The avatar’s realism should, thus, be increased to maximise the SoBO, but one should be careful with the possible uncanny valley effect.
Self-location “is a determinate volume in space where one feels to be located” (Kilteni et al., 2012a). This volume in space is normally localised within the physical body (Blanke and Metzinger, 2009) but can be altered in situations of out-of-body experiences (Ehrsson, 2007). The SoSL refers to the spatial experience of being inside a body (Kilteni et al., 2012a). It is noteworthy that it is different from the experience of being inside a virtual world (which can also happen without a body or avatar), which relates to presence—the sense of “being there”—or the place illusion (Slater, 2009). Although having similar and complementary concepts, the SoSL relates to the body, while presence relates to the virtual world. This is why presence is left out of this paper, as we focus on the relationship between the self and the body in VR [for a review of presence, refer to the study by Felton and Jackson (2022)]. The main question in this section is then, as written by Lenggenhager et al. (2009), “Do we localise ourself according to where we feel our body to be (somatosensory cues), where we see our body to be (visual cues), or at the origin of our visual perspective?” Interestingly, it has been found that it is possible to feel localised at two different places at the same time (Wissmath et al., 2011). The SoSL is based on three aspects: the visuospatial perspective, vestibular signals, and tactile inputs (Kilteni et al., 2012a).
The visuospatial perspective relates to the user’s point of view, being a first-person perspective (1PP) or a third-person perspective (3PP) (see Figure 3). This aspect is important as, apart from pathological conditions, one feels consistently in a 1PP. It has been shown that modifying the origin of the visual perspective (which is easily carried out in VR experiments) impacts the place where one feels located, i.e., the SoSL (Ehrsson et al., 2007; Lenggenhager et al., 2007; Lenggenhager et al., 2009; Blanke and Metzinger, 2009). Moreover, when the virtual body is exposed to a threat (see Section 3.2.3 for details about threats and self-location), physiological changes are observed, and those changes are greater in a 1PP compared to a 3PP (Armel and Ramachandran, 2003; Petkova and Ehrsson, 2008; Petkova et al., 2011).
FIGURE 3. Third-person perspective embodiment induced by visuo-tactile stimulations. This is one possible way to achieve a 3PP in a “real” environment. It is noteworthy that in fully-digital VE, it is much easier to achieve such a perspective change by modifying the position of the virtual camera. Based on the work by Lenggenhager et al. (2007).
Vestibular signals also play an important role in the SoSL (Lopez et al., 2008). They consist of body information of rotation and translation, as well as orientation in relation to gravity (Blanke and Metzinger, 2009). It appears that patients who have experienced out-of-body experiences (i.e., the feeling of being outside of their body with the visual perspective also coming from outside the body boundaries) had a vestibular dysfunction (Blanke et al., 2004; Kilteni et al., 2012a).
Finally, tactile stimulations are linked to proxemics and surroundings. The former is divided into three categories (Halligan et al., 2003): 1) personal space, which is limited by the skin; 2) peripersonal space, which is everything at grasping range [reported at 45 ± 7 cm by Rabellino et al. (2020)]; and 3) extrapersonal space, which is all that is out of reach. This is linked to the way the brain encodes the surrounding space relatively to the body. Studies have shown that the SoSL can, indeed, be manipulated by synchronous visuo-tactile stimulations. Normand et al. (2011) embodied participants in avatars with body volumes different from those in their real biological body, which resulted in a differently perceived personal space.
More recent experiments show that users’ emotions (Freeling et al., 2022), personality traits, and preferences play a role in the SoE as well (Burin et al., 2019). The locus of control (describing how people attribute the control they have over the outcome of events in their lives) impacts the SoE (Jeunet et al., 2018; Dewez et al., 2019). Moreover, users’ preferences were studied by Fribourg et al. (2020). In this study, participants started with a “minimal” SoE condition (minimal anthropomorphic avatar, no control—automatic animation playing—and a 3PP) and progressed towards an “optimal” SoE condition (realistic avatar, full-body motion capture, and a 1PP). To do so, users increased the level of each factor in the order they wanted. Each factor relates to one of the sub-components, that is, the level of control to modulate the SoA, the avatar appearance to modulate the SoBO, and the point of view to modulate the SoSL. Since potential preferences of users could vary depending on the task, the authors designed four different tasks using a full-body avatar to cover more widely the range of possible actions in a VE: a task involving the upper body in which participants had to hit a punching bag; a task entailing the lower body by hitting a soccer ball; a third task to follow fitness movement, with no interaction with the environment; and finally, a walking task with obstacles to avoid. The results showed that the avatar appearance was increased last and that participants consistently preferred to increase first the level of control (from automatic animation playing to triggered animation to inverse kinematics to full-body motion capture) and the point of view. Moreover, some configurations (considered a “suboptimal” SoE) revealed an equivalent SoE as the one felt in the optimal configuration, as measured with a standardised questionnaire (see Section 3.1).
All three components of the SoE have been presented in isolation, reviewing their definition and factors impacting the SoE (see Table 1). However, they all work together to constitute the SoE and are not independent of each other. This is detailed in the following section.
TABLE 1. Summary of factors that favour the SoE, as described in Sections 2.1–2.4. This summary is based on the literature review presented in this paper and is, therefore, not a complete overview of the research area.
In this section, we review the literature in order to analyse the inter-dependencies existing between the components of the SoE. Two main questions are of interest: 1) What are the relationships between the SoE and its sub-components? 2) Are there any links between the sub-components?
According to the work of Kilteni et al. (2012a), it is yet unknown if there is a dominant component or if they all have the same weight. Some authors treated the SoSL as synonymous to the SoE (Lenggenhager et al., 2007; Blanke and Metzinger, 2009). Some other authors proposed that the SoBO is not essential to feel embodied (De Preester and Tsakiris, 2009); this is motivated by experiments eliciting tool embodiment [feeling a tool to be an extension of one’s body (Weser and Proffitt, 2019)] in which there is no SoBO involved (de Vignemont, 2011). Regarding the SoA, Tsakiris et al. (2010b) stated that, based on their experiment using functional magnetic resonance imaging (fMRI) and video-projected images (not in VR), the perceived agency is an important factor, as confirmed by Newport et al. (2010), concluding that a lack of agency may inhibit embodiment (in a non-VR experiment).
However, to the best of our knowledge, no research has been conducted to exclusively study the relative importance of the three components to experience an SoE. Kilteni et al. (2012b) suggested that this may be time-varying or even experiment-specific.
To summarise, there is currently no consensus about the weight of each sub-component of the SoE, and we believe it remains under-studied. As observed previously and concluded by Fribourg et al. (2020), users tend to prefer increasing the level of control and the point of view before increasing the avatar’s realism, but no conclusion can be drawn yet due to the impact of each factor on the sub-components. Finally, the dynamics and evolution of the relative importance of each sub-component remain unknown.
The way the SoA, SoBO, and SoSL interact with each other to form (or not) an SoE remains unclear as well. We review in the following sections the literature regarding the two-way interaction between each pair of components.
As explained in Section 2.3, the visual perspective is related to one’s SoSL. Petkova et al. (2011) found in a VR experiment that the visual perspective has an impact on the SoBO. It was unknown at the time whether breaking the SoSL weakened the SoBO and whether the 1PP was mandatory for experimenting a SoBO. Later, in a VR experiment, Maselli and Slater (2013) concluded that the 1PP was essential for the SoBO to occur. Nevertheless, Chen et al. (2018) stated that a SoBO is still possible in a 3PP in VR. In another VR experiment, Maselli and Slater (2014) stated that “self-location and ownership can be selectively altered,” although strongly coupled. Serino et al. (2013) reviewed VR and non-VR neuroimaging studies and concluded that the SoBO and the SoSL are associated with modulations of brain activity in different brain regions (in the premotor cortex—involved in movement planning of proximal muscles—and in the temporo-parietal junction—electrical stimulation of this region can produce perception illusions like out-of-body experiences, respectively (Blanke and Arzy, 2005); see Figure 4). Nevertheless, they consider bodily experience in isolation (that is, the SoBO on one side and the SoSL on the other side), although the body interacts with objects within the peripersonal space. A multisensory representation of the peripersonal space is created by combining body-related signals with information about external stimuli existing in the vicinity of the body (Graziano and Cooke, 2006).
FIGURE 4. Various brain regions mentioned in the literature regarding the SoE. Zones are approximate, for illustration purposes; top left: superior view of the brain; top right: lateral view of the brain; bottom left: coronal cut of the brain; and bottom right: sagittal cut of the brain. Zones in red are commonly associated with the SoA, zones in green are commonly associated with the SoBO, and zones in blue are commonly associated with the SoSL. Zones in grey are mentioned but not associated directly with one of the components. We can observe some overlaps, for instance, in the insula responsible for sensorimotor integration integrating information related to the SoA and the SoBO, prepared using biorender.com.
Regarding the link between the SoA and SoBO, there is currently no consensus in the community. There are two models (from the neuroscience community) of the relation between the SoBO and the SoA: an additive model, in which they are both strongly related (Tsakiris et al., 2010b), and an independent model, where the SoBO and the SoA are qualitatively different experiences, with distinct brain networks, as denoted by Tsakiris et al. (2010b). Tsakiris et al. (2007) stated that a SoBO does not imply an SoA, but an SoA “normally” implies a SoBO. Kalckert and Ehrsson (2012) found that, however, SoBO and SoA were independent of each other. Tsakiris et al. (2010b) found in an RHI-like experiment (not in VR) that the SoA is “responsible for the coherence of body ownership.” Indeed, sensory mechanisms used in the RHI elicit a SoBO based on a local representation of the body, while the action (therefore, the SoA) provides coherence of the whole bodily self-representation (Tsakiris et al., 2010b). In VR this time, some factors, like the avatar’s realism, may influence the SoBO. The hand design sometimes has an impact on the SoA (Argelaguet et al., 2016) and sometimes not (Lin and Jörg, 2016). The relationship between these two components of the SoE may vary depending on the experimental conditions, as stated by Chen et al. (2018). They performed multiple RHI experiment variations (not in VR). A key difference between their experiments and other RHI experiments is that Chen et al. implemented multiple manipulations (tactile stimulation and active movement) at the same time. They argue that the SoBO “was strengthened and extended” by the SoA. This could explain the varying relationship between the SoBO and the SoA. Nevertheless, more recently, in a meta-analysis on fMRI studies only, Seghezzi et al. (2019) concluded that the brain has three different regions that process the SoBO and the SoA: two regions to separately process them and one common region to aggregate the SoBO and SoA at a higher level. The SoBO seems to be processed in the left inferior parietal lobule and the left extra-striate body area, which may be “part of a network in which multisensory inputs are integrated” (see Figure 4). In turn, the SoA seems to be processed in the left sensorimotor area, the left posterior insula, the right postcentral gyrus, and the right superior temporal lobe. Finally, the SoBO and SoA are integrated in the left middle insula, having a role in sensorimotor integration (Cauda et al., 2011) (see Figure 4). The presence of distinct but also common regions in charge of processing the SoBO and the SoA could explain the discrepancy in the results found. This may be due to some experimental protocols in which the sensorimotor integration in the left middle insula is not carried out. Studies should be conducted to further understand these processes and to compare previous experiments with different experimental protocols.
Finally, the link between the SoA and SoSL has received, to date, little attention from the community. David et al. (2006) performed an fMRI study where agency and the visuospatial perspective are manipulated in a virtual ball-tossing game with 3D scenes and virtual characters on a screen. They found no significant interaction between the visuospatial perspective and the SoA. While using avatars, this experiment was, however, not in VR but on a screen-based desktop 3D environment, which limits the user’s experienced SoSL. More recently, Guy et al. (2022) found in a VR experiment an interaction between the SoA and the SoSL: shifting the user’s point of view to the right in a 3 PP lowered the SoA, and manipulating the user’s SoA with a biased visual feedback negatively impacted the SoSL but only in 1PP. However, the reason for this interaction remains unknown.
Guy et al. (2022) manipulated the three components in the same experiment and found that all manipulations interacted regarding the SoBO score, but further studies are necessary to understand the results. Nevertheless, the literature tends to show that the SoA and the SoBO are partly dependent and that the SoSL may be independent of the SoA while closely related to the SoBO (see Figure 5). This dependency makes the assessment and measurement of the SoE harder. Indeed, as we do not know how to fully assess the SoE, it is usually carried out by analysing each component separately. However, depending on the experimental protocol, the influence varies, and some components might be selectively altered, as stated by Maselli and Slater (2014). It is still unclear what factors impact this inter-dependency, and further studies should be pursued to answer these questions. Moreover, these results depend on how the SoE and its components are measured, which is reviewed in Section 3.
FIGURE 5. Visualisation of the current knowledge about the inter-dependence of the different components of the SoE. The SoA and SoBO are, depending on the context, sometimes found independent and sometimes found to be linked. Moreover, it has been found that they are processed separately by two distinct brain regions, and a third region aggregates information at a higher level (Seghezzi et al., 2019). The SoSL, while less studied, seems to have some interaction with the SoA. The SoSL and SoBO are strongly linked.
The study of the SoE has shown that the users’ perception of their body as well as their behaviour can be modified in VR (Banakou et al., 2013; Kilteni et al., 2013). Users see themselves in the VE through an avatar, and the characteristics of this avatar can modify their perception and behaviour. This is allegedly linked to the Proteus effect, stating that participants change their identity and behaviour by taking the perspective of an avatar different from them (Yee and Bailenson, 2007). In the following paragraphs, we review different articles showing this effect in different situations.
Feeling embodied towards an avatar can improve performance in cognitive tasks (Steed et al., 2016; Banakou et al., 2018). Indeed, Banakou et al. (2018) showed that people embodied in an avatar looking like Einstein manifested an improvement of performance in cognitive tasks compared to those who were not, as if, while being Einstein, participants had to “be smart.” This improvement was higher for people with low self-esteem. Furthermore, an SoE towards Einstein’s avatar in this experiment would reduce implicit bias towards old people [the fact of associating stereotypes to a group of people—old people—without conscious knowledge (Brownstein, 2019)]. However, it is not clear if this implicit bias reduction is because of embodying an old person or Einstein, who is an eminent and famous old person.
In another experiment, Kilteni et al. (2013) embodied Caucasian people in either a “casually dressed dark-skinned virtual body” or in a “formal suited light-skinned virtual body,” where they played a West-African djembe hand drum in VR. Both groups showed a strong SoBO; however, the casually dressed dark-skinned group also showed “significant increases in their movement patterns for drumming compared to the baseline condition,” although they were not djembe players. Again, it is as if users “played a role” guided by stereotypes (without being asked to). This modification of movement increased the more a participant felt a high SoBO (reported via a post-experiment questionnaire—see Section 3.1).
Banakou et al. (2013) embodied adult participants in a 4-year-old child or in adult virtual bodies scaled to the same height as the child by using immersive VR in 1PP with a head-mounted display (HMD). A SoBO could be experienced under both conditions, with similar strength, using visuomotor synchrony (the virtual and real body movements were synchronised). The authors observed an overestimation of the size of objects compared to a non-embodied baseline, and this overestimation is significantly greater when embodied in the child body than that in the “scaled-down” adult body. They performed a second experiment with additional participants, similar to the first experiment, except that there was no body-ownership illusion, by visuomotor asynchrony. The overestimation found in the first experiment did not appear in this second experiment. They concluded that “there are perceptual and probably behavioural correlates of body-ownership illusions that occur as a function of the type of body in which embodiment occurs” (Banakou et al., 2013).
However, feeling embodied in an avatar does not always have an impact on participants. Indeed, Verhulst et al. (2018) analysed shopping behaviour and the perception of different products in VR for participants embodied in an avatar with an average body mass index (BMI) or in an obese avatar. They expected stereotypes classically associated with obese people (called the “weight stigma”) to emerge, such as unhealthy diet by buying more food products and products with high energy intake, as well as rating unhealthy food as being tastier, compared to participants in normal (in the sense of the World Health Organization) BMI avatars. The results showed no modification in the behaviour of participants embodied in obese avatars towards negative stereotypes nor buying healthier products, except coke as less healthy and apple as tastier. In this experiment, a product is given a “healthiness score” by computing its nutrient profile score based on the nutritional content of the food/drink (the lower the nutrient profile, the healthier the product). However, these results have some limitations as the participant pool lacked diversity (all the same background and of Asian culture), and using BMI as an indicator of healthy nutrition can be challenged.
The former studies have in common that they have embodied participants in human-like avatars. However, some researchers also studied embodiment in non-human avatars. It is possible, for example, to embody in an animal or a vegetable. Ahn et al. (2016) embodied participants in a cow or a coral in a VR experiment. Participants revealed a greater connection between the self and nature compared to a video. Moreover, this higher interconnection with nature elicited “greater perceptions of the imminence of the environmental risk and involvement with nature, which persisted for 1 week” (Ahn et al., 2016). More recently, Spangenberger et al. (2022) embodied participants in a tree in an experiment using VR and a desktop monitor. Whichever medium is used, a higher SoE resulted in a greater relatedness to nature [as measured by the Nature Relatedness Scale (Nisbet and Zelenski, 2013)]. The effect was not stronger under the VR condition, suggesting that VR does not improve nature relatedness. However, participants reported to be more immersed in VR, which “provoked reflective processes on one’s role towards nature” more than with the desktop.
In the previous paragraphs, we observed that the SoE in VR has many impacts on the behaviour and perception of participants, depending on the type of avatar. It can also change implicit biases people have (Peck et al., 2013; Banakou et al., 2016; Banakou et al., 2018) up to 1 week after the experiment (Banakou et al., 2016). Indeed, in their VR experiment, Peck et al. (2013) observed that light-skinned females embodied in a dark-skinned avatar significantly reduced their implicit racial bias against dark-skinned people. Thus, the SoE can impact users’ experience in VR systems. Not surprisingly, to the best of our knowledge, these impacts have mostly been studied in the short term. As VR systems are becoming more and more accessible to the general public, these impacts should also be studied in the long term, even if such longitudinal studies are difficult to perform (mostly due to high abandon rates in participants).
In light of these impacts, it is important to better understand the SoE and its sub-components. To achieve this objective, relevant and reliable metrics of the phenomenon have to be defined. In the next section, we review the different assessment methods of the SoE and how the SoE is manipulated through each sub-component in VR.
In this section, we describe how the SoE has been assessed in the literature. First, we focus on self-reported questionnaires in general. Then, we elaborate on additional assessment methods for each sub-component of the SoE (see Table 2).
TABLE 2. Summary of the different assessment methods used in the literature to measure the SoE in VR.
Questionnaires are the main method used to evaluate the SoE (Kilteni et al., 2012a; Gonzalez-Franco and Peck, 2018).
In order to evaluate the ownership towards the rubber hand in the RHI, Botvinick and Cohen (1998) asked the participants to fill in a nine-item questionnaire (in a random order) on a seven-point Likert scale. Some questions, such as “I felt as if the rubber hand were my hand,” relate specifically to the SoBO towards the rubber hand. Some other questions were more general and exploratory. It is noteworthy that, at the time, only the SoBO was the focus, explaining the lack of questions regarding the SoA and SoSL. In further experiments (not necessarily in VR), new questions were added to evaluate not only the SoBO but also the SoA (e.g., “it seemed like I was in control of the rubber hand” (Longo et al., 2008b)) and the SoSL [e.g., “I experienced that I was located at some distance behind the visual image of myself, almost as if I was looking at someone else” (Ehrsson et al., 2007)]. Most, if not all, of the following experiments, inspired by the RHI, used questionnaires to assess the SoE. They were either referring to a physical body part [for instance, the rubber hand (Longo et al., 2008b)] or to a virtual body part (Slater et al., 2010). Some questions of the questionnaire used by Botvinick and Cohen (1998) have been reused in other studies (Ehrsson, 2005; Lenggenhager et al., 2007; Argelaguet et al., 2016), while some others adapted to the particular experiment (Normand et al., 2011). Furthermore, new questions were added, depending on the experiment, as exploratory questions (Longo et al., 2008a; Normand et al., 2011; Dobricki and de la Rosa, 2013). Normand et al. (2011) used this new question “I felt an after-effect as if my body had become swollen,” which makes sense in their experiment but not necessarily in other studies. This led to a lot of different questionnaires used in the literature, making it hard to compare results between the experiments.
To overcome this problem, many researchers intended to create standardised questionnaires by grouping the most frequent and relevant items from the literature (Dobricki and de la Rosa, 2013; Gonzalez-Franco and Peck, 2018; Eubanks et al., 2021; Peck and Gonzalez-Franco, 2021). Gonzalez-Franco and Peck (2018) identified six main categories of questions depending on the experiment: body ownership, agency, self-location, but also motor control, tactile sensations, external appearance, and response to an external stimulus. This echoes De Vignemont’s categories of measures of embodiment being spatial measures (“is the space surrounding the embodied object processed as peripersonal space”), motor measures (does one feel that the embodied object “directly obeys one’s will”), and affective measures (is the embodied object “protected from hazardous situations”) (de Vignemont, 2011). Peck and Gonzalez-Franco (2021) continued their work and refined this standardised questionnaire by collecting data from nine experiments with over 400 questionnaire answers. In this updated version, they removed non-universal or redundant questions, reducing the questionnaire from 25 to 16 items. This allowed them to reshape the different sub-components of embodiment, now named Appearance, Response, Ownership, and Multisensory. Peck and Gonzalez-Franco (2021) stated that this new version produces a wider range of embodiment scores than the older questionnaire. These categories are not the same components as the ones defined by Kilteni et al. (2012a). It should be noted how, for instance, there is no Agency sub-component in this new categorisation of embodiment. Instead, the SoA is considered a transversal factor, and SoA scores can be computed by merging questions of Appearance, Ownership, and Multisensory categories. This makes sense with the literature where, for instance, the visual appearance of the avatar has an impact on the SoA (Argelaguet et al., 2016; Lin and Jörg, 2016; Chen et al., 2018). It is noteworthy that the categories with the same name in the 2018 and 2021 versions of the questionnaires proposed by Peck and Gonzalez-Franco (Gonzalez-Franco and Peck, 2018; Peck and Gonzalez-Franco, 2021) are not identical, but previous categories are correlated to the new ones.
Although some questions still need to be adapted to a given experimental design, it is possible to do so without drastically changing them. For instance, questions about “feeling a touch” during the experiment can be used even if the user is not supposed to be touched, by referring to the feet touching the virtual floor. Peck and Gonzalez-Franco (2021) encourage adapting the questions following their guidelines instead of removing non-applicable questions to provide better comparability between experiments.
Meanwhile, Roth and Latoschik (2020) reviewed the literature to create their 12-item Virtual Embodiment Questionnaire (VEQ). They aimed to extend a previous SoBO questionnaire (Roth et al., 2017), tightly constrained to virtual mirror scenarios1 (making it hard to adapt to other experiments, thus reducing the comparability between experiments). Roth and Latoschik stated that their questionnaire also needed validation, consistency, and balance in a number of items of each component. The VEQ addresses these issues by adapting the questions to generic scenarios and balancing the number of items in each category while keeping the same categories.
The authors reviewed the literature to identify questionnaire items and performed a confirmatory factor analysis on the data from three experiments. They identified three factors: 1) ownership of a virtual body; 2) agency over a virtual body; and 3) change in the perceived body schema. Finally, they tested the questionnaire by confirming the reliability and validity of the scale. It is noteworthy that there is no self-location factor. This is voluntarily out of the scope of this questionnaire, as the authors argue that the main goal in VR is to be in a 1PP and not disturb the SoSL. They may address this issue in future works.
The broad use of one of these standardised questionnaires would greatly help the comparison of different experiments, and the authors of both these new questionnaires invite the community to do so. However, there are still new questionnaires arising in the domain (Eubanks et al., 2021), making it hard to converge towards a standardised way to measure embodiment via questionnaires in experiments. The questionnaire proposed by Eubanks et al. (2021) aims to address the three factors of embodiment, as defined by Kilteni et al. (2012a), with few questions. They validated this questionnaire using exploratory factor analysis methods based on data from two studies on the effects of inverse kinematics solutions on the SoE. Nevertheless, this questionnaire is still in the preliminary phase and will be extended in the future (as there is, for instance, only a single-body ownership question). Another goal of this questionnaire was to be usable as an in-VR questionnaire. Alexandrovsky et al. (2020) compared, in two user studies, paper/web questionnaires (outside VR) and those carried out within VR at the end of an experiment. They observed similar questionnaire results in both cases. While participants reported lower usability for in-VR questionnaires, the authors also observed higher enjoyment while using these questionnaires as compared to questionnaires outside VR.
Although they are easy to use, questionnaires, thus, have drawbacks. They are used as a reference, but it is hard to use them as a ground truth (see details in Section 3.3). This is why other assessment methods are needed to evaluate the SoE. In the following sections, we present such measures for each component of the SoE. Although we focus on VR, some experiments reviewed here are not carried out in VR (but could be adapted if necessary) and can be relevant to understand how the SoE is evaluated.
In addition to questionnaires, other assessment methods have been introduced to measure the SoE. Nevertheless, we do not know how to directly assess the SoE. Instead, we try to evaluate each component separately, in a way to approximate the SoE and obtain a surrogate measure. In this way, the assessment methods are often focused towards a sub-component of the SoE. However, it should be remembered that these sub-components are not mutually exclusive, and these assessment methods can have some overlaps, for instance, measures evaluating both the SoSL and the SoBO such as the proprioceptive drift (see the following).
As mentioned in Section 2.1, the SoA is constituted of the feeling of agency (the intent to act) and the judgement of agency (the comparison between the predicted and actual outcomes of the action).
As such, one way to study the SoA (in VR or not) is to focus on the explicit JoA (for instance, a participant stating “I am the one who did this”) and carry out an action-recognition experiment. The participant has to judge, according to a video or directly what they see in the VE, if the action they see is theirs or not. They perform a specific hand movement, and the video either shows their hand moving or the hand of an experimenter doing another movement. In VR, the hand movement can directly be manipulated. In a way, participants are asked, “did you do that?” (Haggard, 2017).
However, studies based on the explicit JoA present the cognitive bias of overestimating agency levels or attributing to themselves actions that they have not carried out (Haggard, 2017). This bias is even stronger when the outcome of the action is positive rather than neutral or negative (Haggard, 2017).
These cognitive biases could be avoided by using implicit assessments such as intentional binding, sensory attenuation, and biased sensory feedback. These different types of implicit assessments are described in the following paragraphs. It should be noted that these measures are only weakly correlated to explicit measures (Dewey and Knoblich, 2014).
The intentional binding effect (Haggard and Clark, 2003) is based on the fact that programming, executing actions, and predicting their outcomes influence time perception. In experiments using this effect, participants are asked to report the time (with a small clock in front of them) either of a voluntary action or a resulting sensory event (like a tone) (Haggard, 2017). Voluntary actions are perceived as shifted in time towards their resulting sensory event, and the outcome itself is perceived as shifted backwards towards the voluntary action that caused it. Time perception is also affected by attention, drug intake, and visual adaptation (Haggard, 2017). Therefore, shifts in time perception cannot be a diagnostic factor of the SoA, but the intentional binding effect can be used to evaluate a difference of agency between two conditions.
Sensory attenuation is based on Blakemore’s comparator model of agency (Blakemore et al., 2000) described in Section 2.1. More specifically, it uses the fact that the sensations made by our own actions, which are predictable, are attenuated compared to external actions. When one feels the agent of their actions, the physiological reaction will be weaker than for actions of other people. This phenomenon has been initially explored in psychophysical experiments studying why some people cannot tickle themselves (Weiskrantz et al., 1971; Blakemore et al., 1999). This sensory attenuation effect can be measured by two means.
The first type of measurement is based on a subjective assessment (Weiss et al., 2011). This process can happen in tactile (Blakemore et al., 1999; Tsakiris and Haggard, 2003; Bays et al., 2006), visual (Cardoso-Leite et al., 2010; Gentsch and Schütz-Bosbach, 2011), and auditory (Martikainen, 2004; Sato, 2008) modalities, and the principle is similar for each of them. A protocol that focuses on auditory perception includes a participant and an experimenter and a button in front of each of them (each associated to a tonality with a different frequency). The participant learns first the relation between the actions—button presses—and the sensory consequences—which tonality is played—for each button). Then, in the test phase, after a button press, a tone of the same frequency but with a different amplitude (either lower or higher) is played by a computer, and the participant must judge which tonality is the loudest. Regarding visual perception, instead of tonalities, images are shown on a screen.
The second type of measurement of sensory attenuation uses surface electroencephalography (EEG) (Gentsch and Schütz-Bosbach, 2011; Kühn et al., 2011; Horváth, 2015; Weller et al., 2017). The principle is the same as explained previously: either the participant performs a self-action of pressing a button producing a tone, or a sound is externally produced by a computer (see Figure 6). The event-related potential (ERP)2 response of sounds produced by our actions is weaker than the ERP responses resulting from external sounds. That is, the N100 component (a negative evoked potential3 elicited 100 ms after the stimulus) is smaller in amplitude for actions caused by a participant than the effects caused by a computer, which, thus, allows us to detect if it is a voluntary action or not.
FIGURE 6. Sensory attenuation assessment with surface EEG. Either the participant presses a button which plays a tone, or a computer generates a tone without any action from the participant. Comparing the amplitude of the ERP under the two conditions around the N100 component allows us to detect if the user was feeling the agent or not.
Other assessment methods rely on creating an artificial inconsistency between an action’s predicted outcome and its perceived outcome. For instance, Jeunet et al. (2018) conducted an experiment in VR where participants had to perform different hand movements. The users’ real hand was tracked in such a way that their avatar’s hand moved accordingly. The users’ perceived movement was punctually manipulated in VR by introducing visually biased feedback, such as a temporal delay, two fingers inverted, or a finger moving by itself.
By inducing these kinds of inconsistencies, studies attempt to find brain areas related to the SoA either with fMRI or EEG (see Figure 4).
fMRI studies of agency have found that the angular gyrus, in the parietal lobe (see Figure 4), is involved in the explicit attribution of the SoA (noticed by brain activation when there is an inconsistency between the expected and perceived outcomes) (Farrer and Frith, 2002; Farrer et al., 2003; Haggard, 2017). A meta-analysis also confirmed that the temporo-parietal junction, including the angular gyrus, is a neural correlate of non-agency (Sperduti et al., 2011). Some of these studies also associated the dorsomedial prefrontal cortex and the lateral prefrontal cortex with non-agency towards an action. The only area consistently associated with agency is the anterior insula (Haggard, 2017) [area found to be engaged in interoceptive awareness of experience (Critchley et al., 2004)]. Finally, as mentioned previously, in their meta-analysis, Seghezzi et al. (2019) concluded that SoA-specific regions include the left SMA, left posterior insula, right postcentral gyrus, and right superior temporal lobe. These studies used a screen and not VR to study the SoA. Nevertheless, they provide insights into the neural mechanisms involved in the SoA and which brain regions are expected to be activated in some situations.
Other studies used EEG to find neural correlates. Padrao et al. (2016) noticed that errors due to our actions elicited an N100 in the fronto-central areas, while external errors (e.g., because of the system) elicited an N400 in parietal areas. N400s are mainly observed in the case of semantic or conceptual violations (Haggard, 2017) [e.g., sentences like “I am going to eat a house” or observing someone introducing a screwdriver instead of a key into a keyhole (Padrao et al., 2016)]. Kang et al. (2015) analysed brain rhythms and suggested that the α band (8–12 Hz) would be the main neural oscillations involved in the SoA, which was found again by Alchalabi et al. (2019), where they found an activation in the premotor cortex in the μ band (8–12 Hz over the sensorimotor cortex). The latter also found, in the case of strong agency, an increase in spectral power in the α band over the fronto-central and central-parietal areas with respect to its average value. In the case of low agency, they observed an increase in spectral power in the α band over fronto-central and left frontal areas. Jeunet et al. (2018) observed an increase in spectral power in the θ band (4–8 Hz) over the left fronto-central and parietal areas under high-agency conditions and a decrease in spectral power in the θ band over the right centro-temporal areas.
In VR, apart from questionnaires, the SoA is measured by introducing biased sensory feedbacks (Padrao et al., 2016; Jeunet et al., 2018; Alchalabi et al., 2019), as VR allows us to easily perform these manipulations.
Studies focussing on ownership rely on inducing an illusion of ownership towards a (virtual) body (or a virtual body part) that is not ours. This illusion is induced by either visuo-tactile stimulations [such as in the RHI (Botvinick and Cohen, 1998)] or visuomotor synchrony. After the illusion is generated, the SoBO can be measured in different ways.
As explained in the RHI experiment in Section 2.2, the illusion of ownership is induced by synchronous visuo-tactile stimulations on the participant’s real hand and on the rubber hand. Physiological measures have been used in this context, and differences have been found between synchronous and asynchronous stimulation conditions. Moseley et al. (2008) observed that the RHI induced a decrease in the temperature of the hidden limb but not for other body parts. Likewise, Kammers et al. (2011) conducted an RHI experiment in which reducing the limb temperature (by using a thermoelectric metal plate connected to a heat pump) strengthened the RHI (and conversely weakened the illusion when warming the real hand).
In their VR experiment, Llobera et al. (2013) observed a change in temperature sensitivity (on the palm of the hand) under the asynchronous condition, and this change is correlated with the SoBO (as measured with questionnaires). Here, the asynchronous condition is the condition where the virtual full body representing the user is in an inconsistent posture (compared to the participant’s one), and the movements of the virtual right arm do not entirely match the user’s movement.
In a non-VR RHI experiment with fMRI, Ehrsson et al. (2004) observed premotor cortex activation when inducing a SoBO illusion, this activation being correlated with the strength of the illusion. Moreover, the timing of the illusion is consistent with the one of the activation. In another RHI study, Tsakiris et al. (2010b) associated the SoBO with the activation of midline cortical structures. The SoBO has also been studied in VR with fMRI. Bach et al. (2012) introduced a VR device suitable for use with fMRI systems to replicate the RHI in VR. It uses a pneumatic device to apply the tactile stimulation on the participant’s real hand and a special HMD designed for use in fMRI environments. Later, Bekrater-Bodmann et al. (2014) used this device to study the SoBO in VR with fMRI. They found, as did Ehrsson et al. (2004), an activation in the bilateral ventral premotor cortex due to visuo-tactile synchrony.
The SoBO has also been studied with surface EEG. Blefari et al. (2011) conducted an experiment based on the RHI, with the goal of finding EEG neuromarkers based on the results obtained by Ehrsson et al. (2004). Analyses revealed an increase in the power spectral density in the frontal, parietal, and central areas. However, it should be noted that it is only an introductory result as they used only N = 5 participants, six trials per participant, 16 EEG channels, and a sampling rate of 128 Hz. Nevertheless, 2 years later, Evans and Blanke (2013) conducted a similar experiment, this time in VR, using a 64-channel EEG headset. The results show that the SoBO is reflected by a modulation in the θ band (4–8 Hz) in the fronto-parietal cortex, which is consistent with the previous experiment by Blefari et al. (2011).
In some experiments, after the participant has had an illusion of ownership towards a rubber hand or a virtual body, the fake body part is threatened either actively (someone else approaches with a tool and hits the fake body part) or passively (by putting threats to avoid in the environment—mainly carried out in VR setups). This protocol has been introduced by Armel and Ramachandran (2003) in an experiment similar to the RHI. Under the synchronous stimulation condition, the authors noticed a variation in the GSR when the rubber hand was threatened, which was not observed under the asynchronous stimulation condition. It is unknown if participants incorporated external objects (the rubber hand) into their body image or if participants were just subject to a demanding effect (participants knowing what is the correct answer the experimenters want to obtain; see Section 4). This is why the authors introduced the GSR as an objective measure of this phenomenon. Threat has been used to test and evaluate the SoBO in many other experiments and exported to VR (Ehrsson et al., 2007; Petkova and Ehrsson, 2008; Slater et al., 2010; Yuan and Steed, 2010; Pomés and Slater, 2013; Zhang and Hommel, 2016). The response to threat can also be measured by a change in body motion (Gonzalez-Franco et al., 2010; Kilteni et al., 2012b; Fribourg et al., 2018) or neuroimagery (Ehrsson et al., 2007; González-Franco et al., 2014). The same kind of experiment has been conducted using electrocardiography. Indeed, the heart-rate deceleration has been found to correlate with the strength of illusion (Slater et al., 2010; Pomés and Slater, 2013). Ehrsson et al. (2007) discovered that threatening a rubber hand that feels “ours” elicits brain activity patterns associated with anxiety, the same as when the participant’s real hand is threatened. These experiments have been replicated in VR by González-Franco et al. (2014), who showed a correlation between the motor cortex activation in response to a threat and the SoBO.
In VR, apart from questionnaires, both physiological measures and response to threat are used to measure the SoBO, with the advantage of VR allowing us to threaten the user’s virtual body without threatening their real body physically.
Apart from pathological conditions (e.g., autoscopy—perceiving the surrounding environment from outside the body, from a different perspective), manipulating and studying the SoSL without using cameras or VR is complicated. Studies focussing on self-location rely on embodying a user in a virtual body, either in 1 PP or in 3PP. This illusion is induced by visuo-tactile stimulations [such as in the RHI (Botvinick and Cohen, 1998)]. Afterwards, the SoSL can be measured in different ways.
As mentioned in Section 2.3, the SoSL is based on a visuospatial perspective, vestibular signals, and tactile stimulations (which are related to personal spaces). Blanke et al. (2015) reviewed neuroscience research on bodily self-consciousness and noticed that the fronto-parietal and temporo-parietal areas are important for the peripersonal space.
Lenggenhager et al. (2011) conducted an experiment in VR using an EEG headset, where the point of view is modified. They noticed a modulation in the α band (8–12 Hz) in the sensorimotor cortex bilaterally. Moreover, the α band power in the median prefrontal cortex (mPFC) is correlated with the degree of experimental manipulation of the self-location.
This method uses proprioception [the feeling of body position and movement (Tuthill and Azim, 2018)]. In the RHI (Botvinick and Cohen, 1998), participants were also asked to blindly point to where they felt their real hand was located, before and after the stimulation. Under the synchronous stroking condition, participants tended to point towards the rubber hand. Proprioceptive drift is the difference between the position before and after the stimulation. This value is correlated with the SoBO towards the rubber hand (Botvinick and Cohen, 1998; Ehrsson, 2005; Tsakiris and Haggard, 2005). Participants pointing towards the fake hand after the stimulation indicates that their peripersonal space (and, thus, their SoSL) has been modified. It is noteworthy that correlations have been found with the SoBO, although we evaluate where the body is (and, thus, the SoSL): that is because the SoBO and SoSL are tightly coupled, in the way that in both SoBO and SoSL manipulations, the whole-body scheme is updated. Still, because this measure asks the participant to indicate where the hand/body is located, we wanted to include it in the SoSL measurement category.
This method has then been generalised to virtual arm (Slater, 2008) or full-body in VR (Lenggenhager et al., 2007). Normand et al. (2011) used another kind of proprioceptive drift in an RHI-like experiment: they embodied participants in avatars with an inflated belly, and they had to estimate the size of the belly before and after the stimulations. The estimation of the belly size of the avatar, indeed, positively correlates with the SoE, as measured using their questionnaire (a mix of questions based on the questionnaire proposed by Botvinick and Cohen (1998), new and exploratory questions).
The SoSL can also be measured by threatening the avatar to the perceived self-location. Indeed, Ehrsson (2007) measured the GSR to evaluate the SoSL in the situation of a threat. They noticed a variation in the GSR after a threat towards the perceived self-location. This provides an insight into the strength of the illusion. The difference with the SoBO is in the protocol, in which participants see themselves in 3PP, but in both cases, the SoBO and the SoSL evaluated via response to threat reflects the experienced fear and anxiety due to the illusion.
A locomotion task is an action-based judgement, where participants must move to their perceived location. Generally, after the synchronous visuo-tactile stimulation, participants are blindfolded (or the HMD is blacked out) and displaced. They are then asked to get back to their previous position (Lenggenhager et al., 2007). In most studies with a 3PP using this assessment method, participants tend to relocate themselves 10–30 cm towards their perceived self-location from their initial position, so this method evaluates a tradeoff between the participants’ real position and their perceived self-location (Lopez et al., 2015; Nakul et al., 2020). The locomotion task has the limitation that the participant has to move, which updates somatosensory, vestibular, and interoceptive signals (Nakul et al., 2020).
To counteract the limitation of the locomotion task, mental imagery tasks have been introduced so that the participant could remain motionless. An example is the “mental ball dropping”: participants are asked to imagine themselves dropping a ball they would hold in their hand. By pressing buttons, they first indicate when they drop the ball and then indicate when they believe the ball touches the floor (Lenggenhager et al., 2009; Ionta et al., 2011; Bourdin et al., 2017). Researchers observed that, after synchronous visuo-tactile stimulation, participants’ estimates of the time required for the ball to reach the ground increased or decreased depending on whether they felt located above or below their body (Bourdin et al., 2017). Nakul et al. (2020) instead used a rolling ball: a red ball appears in front of the participant and rolls at a constant speed towards “them.” After a certain delay, the HMD is blacked out and the participant must indicate with a button press when they think the ball hits them (see Figure 7). This measure revealed higher self-location towards the avatar and higher reports of presence, bi-location, and disembodiment compared to the locomotion task (Nakul et al., 2020). The reason could be that mental imagery tasks allow the user to detach from sensory information from the real environment, whereas locomotion tasks force the user to walk in the real environment and, thus, shift attention to their bodily signals.
FIGURE 7. Mental imagery task as described by Nakul et al. (2020). A red ball appears in front of the participant and rolls at a constant speed towards “them.” After a certain delay, the vision in the HMD is blacked out, and the participant must indicate with a button press when they think the ball hit them. Reproduced from Nakul et al. (2020), licensed under CC-BY 4.0.
In an fMRI study, Vogeley et al. (2004) asked participants to count red balls from multiple viewpoints (not in VR but using a screen) in 1PP and in 3PP. Analyses revealed in both cases an activation of occipital, parietal, and prefrontal areas and a deactivation in mesial cortical and lateral superior temporal areas bilaterally. They also observed an increase in activity under the 1PP condition relative to the 3PP condition in the posterior cingulate cortex and superior temporal cortex.
In VR, apart from questionnaires, the SoSL is mainly measured by introducing a threat or using locomotion/mental imagery tasks, whichever is more appropriate (see Section 3.3).
Facing all these assessment methods, one can wonder how to choose a way of evaluating the SoE. First, we define different criteria that are important to consider in VR, and then, we discuss the advantages and drawbacks of the previously mentioned assessment methods.
In order to compare the different assessment methods of the SoE, we propose different criteria we believe are important for a VR use case.
VR is meant to be used, and an ideal assessment method should enable an ecological situation. These criteria were proposed with this in mind.
The criteria are separated into two categories, depending on whether they relate to the measures or the protocol. Indeed, some measurement tools impose constraints on the user, and some experimental protocols can be used with different measurement tools.
Measure-related criteria:
• Protocol dependent: The measure requires the use of a particular experimental protocol
• Ecological: The measure forces, restricts, or prohibits the user from carrying out some actions
• Self-reported: The measure is a subjective assessment from the user (given orally or written)
• Second task: The measure is a task in itself
• Real-time measurement: Data acquisition is conducted in real time (in the order of milliseconds)
• Real-time processing: Data processing (and interpretation) is conducted in real time
• Cognitive demand: The measure uses cognitive functions of the user (attention, memory, and inhibition)
• Need reference value (e.g., rest): The measure has inter-personal variations and needs a reference value to be interpreted
• Sensitive to artefacts: Measure acquisition contains artefacts
• Cost: Material cost and other costs related to using this measure
Protocol-related criteria:
• Ecological: The measure forces or prohibits the user from carrying out some actions
• Second task: An additional task from the participant is necessary to access the measured process
• Cognitive demand: The protocol uses cognitive functions of the user (attention, memory, and inhibition)
• Emotionally unpleasant: The protocol provokes unpleasant emotions in the user (boredom, fear, anger, unease, discomfort, etc.)
• Need other people: The protocol requires other people (interviewer, observer, etc.)
Although questionnaires are free and easy to use, they have drawbacks. Indeed, as they are self-reported, the answers are subject to the interpretation of questions and cognitive biases (Jahedi and Méndez, 2014; Haggard, 2017). Furthermore, questionnaires require performing a double task (the first task being the experimental protocol and the second task being answering the questionnaire), and the assessment by itself cannot be in real time: it is only at the end of the task, requiring participants to “remember” how they felt during the experiment. Moreover, it is possible to create a questionnaire about a completely new feeling and still obtain relevant questionnaire responses. Slater (2004) created a fake attribute being the “colourfulness of the experience” and created an associated set of questions. The analysis revealed significant differences between the 2 days, one being “more colourful than the other.” However, this has no meaning, insisting on the importance of carefully designing validated and standardised questionnaires of the SoE. Questionnaires are used as a reference, but using them as a ground truth is difficult. In this way, it may be worth coupling questionnaires with at least one other of the methods reviewed, as conducted in several studies. We represent and compare all of them in tables (see Tables 3, 4).
TABLE 3. Comparison of all reviewed measurement methods of the SoE according to our defined criteria. Cells with a “?” are missing information.
TABLE 4. Comparison of all reviewed protocols linked to the SoE according to our defined criteria. It should be noted that proprioceptive drift is only mentioned with the SoSL component as the validity of proprioceptive drift for evaluating the SoBO is questionable (see Section 3.3.2).
Abdulkarim and Ehrsson (2016) found, by moving participants’ hand without them noticing, that the strength of the illusion is not linked to changes in hand proprioception, questioning the pertinence of proprioceptive drift for evaluating the SoBO. Although correlations have been found between the proprioceptive drift and the SoBO, some studies found that a change in proprioception can occur with no impact on the hand SoBO (Holmes et al., 2004; Holmes et al., 2006; Makin et al., 2008), questioning the validity of this assessment method for evaluating the SoBO. Proprioceptive drift was still mentioned as its validity has not been, to the best of our knowledge, tested for evaluating the SoSL. Moreover, this measure is still worth mentioning for historical reasons.
Some experiments are bound to particular protocols. For instance, the response-to-threat assessment method forces experimenters to introduce a threat. However, does a threat have positive or negative impacts on VR experiments? This question was explored by Fribourg et al. (2021) by introducing a threat and verifying if it would impact the participants’ behaviour after the threat and measures of the SoE. Their results suggest that a threat has an impact on the participants’ behaviour but no impact on subjective and objective measurements of the SoE; so, depending on the scenario, this assessment can be used (although a repetitive threat could impact participants’ wellbeing). Physiological measures also have been used in combination with response to threat. Although they are affordable and could be used for real-time assessment of the SoE, they also have drawbacks: they are subject to noise sensitivity and often need a reference value. Their main drawback is their lack of specificity. Indeed, modulations of the heart rate or GSR can be due to not only alterations in the SoE but also other internal or external factors, such as the circadian rhythm or temperature changes, respectively.
Neuroimagery instruments are also promising measures. fMRI offers a good spatial resolution (in the order of millimetres), which enables the identification of brain structures related to the SoE. However, it suffers from poor temporal resolution (around 3–6 s) and portability, which complicates real-time assessment and the use in any real scenario (Weiskopf et al., 2004). Moreover, fMRI is expensive, and combining fMRI with a VR headset is complicated, although some devices exist (Bach et al., 2012). On the contrary, EEG has a rather poor spatial resolution (in the order of centimetres) but offers a high temporal resolution (around milliseconds) and high portability for a cost that, depending on the number of channels and the quality of the expected data, ranges from affordable to relatively expensive, which makes it a better candidate for real-time assessment and use in VR (Muller-Putz et al., 2006; Sauvan et al., 2009; George et al., 2012) and out-of-the-lab usage. EEG headsets are sensitive to electromagnetic noise, and combining them with VR headsets poses challenges. However, multiple studies showed that it is feasible. Tauscher et al. (2019) found that the EEG signal quality can be improved by reducing physical strain on the EEG headset. Si-Mohammed et al. (2020) showed that using an EEG headset combined with a HoloLens was possible and even that small head movements could be allowed when using the EEG headset. Yet, it has to be confirmed more generally (other headsets, more motion, etc.).
The goal of this paper was to review the current knowledge about the SoE in VR and its different assessment methods. We detailed in Section 2 the current separation of the SoE in VR in three sub-components (the SoA, the SoBO, and the SoSL) and their possible origins. Their inter-relation was also discussed, as well as the perceptual and behavioural impacts of the SoE on users. Then, in Section 3, we reviewed the different assessment methods of the SoE in VR. We first observed the emergence of new standardised questionnaires. Their usage would improve experiment comparability and help SoE research. Then, additional assessment methods were discussed, based on the target to be measured: does the user feel agent; does the user feel ownership over a body or a body part; and where the user feels their body/body part to be located, echoing the three sub-components of the SoE. Finally, we suggested criteria to compare the different assessment methods of the SoE and some benefits and drawbacks of some measurement methods.
What measure to choose depends on the use case, and we hope the criteria we propose can help the reader select a measure. In VR, having an assessment method that is real time (in the order of milliseconds) and objective (i.e., independent of the user’s emotional/cognitive/motivational state), while being ecologically valid and reliable, would greatly help embodiment research. Unfortunately, this is not an easy task. First, not all measures presented here have real-time measurement. The remaining measures are EEG, behavioural response to threat, and electrocardiography. As explained in Section 3.3, electrocardiography is not necessarily specific as it can be impacted, for instance, by the temperature of the room. Behavioural response to threat, while real time in the sense of “when the user is threatened, it is possible to measure their reaction,” is not ecological since it is not realistic to constantly threaten the user. EEG allows real-time measurement suitable for VR scenarios. However, the EEG signal-to-noise ratio is still poor and greatly impacted by (mainly head) movements, making the measure less accurate. It also forces the user to wear another headset, which can be cumbersome. Although some studies have found neural correlates for the SoA and the SoBO using EEG, it is not yet the case for the SoSL. Moreover, detecting these correlates with EEG is a first step but does not necessarily mean that it is yet possible to process in real time the EEG signals to measure the SoA or the SoBO. Improvement in EEG processing, with better knowledge about the SoE, will help with this objective.
As Kilteni et al. (2012a) stated, it is yet unknown if the SoE is time-varying and, if so, how it evolves during an experiment. Moreover, we also reviewed in Section 2.6 that the SoE has impacts on users. It is, therefore, important to have a real-time and reliable measure, without a second task, that can be used as a ground truth in order to correctly study the impacts and effects of embodiment on users. This is especially when VR is becoming more and more accessible to the general public but would also generate a new requirement on affordability if the evaluation of embodiment was required by end users. Currently, the main assessment method remains questionnaires and subjective reports. The other presented assessment methods were found to be correlated with some questionnaires. However, it is unclear whether questionnaires and other measures evaluate exactly the same phenomena, as, for instance, there is only a weak correlation between explicit judgement of agency and implicit measures (Dewey and Knoblich, 2014). This should be explored in future experiments, with the increasing knowledge about the cognitive processes related to the SoE. Finally, these correlations should be confirmed with the usage of standardised questionnaires (Roth and Latoschik, 2020; Peck and Gonzalez-Franco, 2021).
Most questionnaires and a significant amount of the literature on the SoE research are based on the RHI (Botvinick and Cohen, 1998) and on the notion of full-body illusions (Ehrsson, 2007; Lenggenhager et al., 2007). Nevertheless, recent publications by Lush (2020) questioned the relevance of using the RHI as a measure and addressed the issues of demand characteristics and imaginative suggestibility of the RHI. Demand characteristics are the impact of participants knowing what is the correct answer that the experimenters want to obtain. For instance, in the RHI, experimenters expect participants to feel ownership towards the rubber hand under the synchronous condition but not under the asynchronous condition. Participants do not necessarily know this but can guess the expected result. As reported by Seth et al. (2021), the participants not only tend to respond to expected answers and behaviour, but demand characteristics also change the subjective experience of the experiment (Kirsch and Council, 1989; Olson et al., 2020). Moreover, Lush (2020) described precisely the RHI to participants and asked them their expectations, without actually conducting the RHI experiment. They found that, across synchronous and asynchronous conditions, participants expected the same pattern of replies (whether considering subjective questionnaires or proprioceptive drift). Reader (2021) replicated the study conducted by Lush (2020): participants were presented the RHI with a video and were asked what they expected under both conditions. They answered with not only rating questionnaires but also free responses. Participants expected greater illusion under the synchronous condition than under the asynchronous condition. Interestingly, these expectations may be driven (at least partially) by exposure to the questionnaire items, as suggested by the free responses (Reader, 2021).
Imaginative suggestions are “requests to experience an imaginary state of affairs as if it were real” (Kirsch and Braffman, 2001). Suggestibility is measured by means of hypnotisability scales, which evaluate “individual differences in the ability to generate experience in response to imaginative suggestion” (Lush et al., 2020). Still, Lush et al. (2020) found that suggestibility was correlated with experienced ownership, as measured by questionnaires or proprioceptive drift. That is, participants who have a higher suggestibility experience a stronger illusion. Furthermore, they analysed how suggestibility variability over participants and their proportion might affect results of RHI studies with a smaller sample size. In other words, what is the impact of having a majority of participants with high or low suggestibility? RHI evidence is almost guaranteed with high-suggestibility participants, while the RHI appeared only half of the time with low-suggestibility participants. Finally, they insist on the importance of controlling the demand characteristics of the RHI (and more generally, in any experiment), especially regarding the effect of imaginative suggestibility. While this has only been tested for RHI studies, it is likely to be an issue for similar VR embodiment illusions. It is important to understand if an imaginative suggestion explains all the effects observed in the RHI or what effects are effectively occurring. Imaginative suggestion also impacts GSR [for instance, with changes in facial expression (Levenson et al., 1990)], fMRI measures, and histamine reactivity (Lush et al., 2019). However, the authors do not mention other measures, such as response to threat, and this should be investigated. Therefore, implicit measures of embodiment must also be interpreted with care, as, without valid subjective reports of embodiment, they may be just proxy measures of something else (for instance, proprioceptive drift may just be a measure of confusion about the location of the hand) (Lush, 2020).
Despite all of this, Ehrsson et al. (2022) reanalysed the data obtained by Lush et al. (2020) and found no specific relationship between hypnotic suggestibility and the RHI. In another article, Slater and Ehrsson (2022) also conducted new analyses of the data obtained by Lush et al. They found that while hypnotic suggestibility “modestly influences” the subjective reports, the major difference between synchronous and asynchronous conditions remains to be explained by the multisensory integration from the RHI. Moreover, they observed that the participants’ expectation towards the RHI was negligible to explain the effect. Trait suggestibility may modulate the illusion as an inter-subject factor, but the RHI remains a multisensory bodily illusion (Slater and Ehrsson, 2022). Finally, they observed the same results with proprioceptive drift. Further studies should be conducted in order to investigate if hypnotic suggestibility impacts other SoE measures or if it is negligible.
This paper reviewed the current state of the knowledge about the sense of embodiment, its impacts on users, and the existing assessment methods. Questionnaires, the current reference measures, are constantly evolving, with the rise of new competing standardised questionnaires (Roth and Latoschik, 2020; Peck and Gonzalez-Franco, 2021). The definition and boundaries of embodiment themselves are redefined, emphasising the need to have better measurement methods to better understand this phenomenon.
MG contributed to the review of literature and wrote the first draft of the manuscript. All authors contributed to the article and approved the submitted version.
The authors declare that the research was conducted in the absence of any commercial or financial relationships that could be construed as a potential conflict of interest.
All claims expressed in this article are solely those of the authors and do not necessarily represent those of their affiliated organizations, or those of the publisher, the editors, and the reviewers. Any product that may be evaluated in this article, or claim that may be made by its manufacturer, is not guaranteed or endorsed by the publisher.
1Participants look at their avatar through a virtual mirror and have to perform different tasks such as “Look at your left hand” (Roth and Latoschik, 2020).
2Measured brain response resulting from a specific sensory, cognitive, or motor event (Luck, 2014).
3Electrical signal produced by the brain in response to an external stimulus.
Abdulkarim, Z., and Ehrsson, H. H. (2016). No causal link between changes in hand position sense and feeling of limb ownership in the rubber hand illusion. Atten. Percept. Psychophys. 78, 707–720. doi:10.3758/s13414-015-1016-0
Ahn, S. J. G., Bostick, J., Ogle, E., Nowak, K. L., McGillicuddy, K. T., and Bailenson, J. N. (2016). Experiencing nature: embodying animals in immersive virtual environments increases inclusion of nature in self and involvement with nature. J. Computer-Mediated Commun. 21, 399–419. doi:10.1111/jcc4.12173
Alchalabi, B., Faubert, J., and Labbe, D. R. (2019). “EEG can Be used to measure embodiment when controlling a walking self-avatar,” in 2019 IEEE Conference on Virtual Reality and 3D User Interfaces (VR), Osaka, Japan, March 23-27, 2019. 776. doi:10.1109/VR.2019.8798263
Alexandrovsky, D., Putze, S., Bonfert, M., Höffner, S., Michelmann, P., Wenig, D., et al. (2020). “Examining design choices of questionnaires in VR user studies,” in Proceedings of the 2020 CHI Conference on Human Factors in Computing Systems, New York, NY, USA, April 25-30, 2020.
Argelaguet, F., Hoyet, L., Trico, M., and Lecuyer, A. (2016). “The role of interaction in virtual embodiment: effects of the virtual hand representation,” in 2016 IEEE Virtual Reality (VR), Greenville, SC, USA, March 19-23, 2016. doi:10.1109/VR.2016.7504682
Armel, K. C., and Ramachandran, V. S. (2003). Projecting sensations to external objects: evidence from skin conductance response. Proc. R. Soc. Lond. Ser. B Biol. Sci. 270, 1499–1506. doi:10.1098/rspb.2003.2364
Atkinson, S., Stolz, A., and Kennedy, H. W. (2020). The future of film report 2020. London: King’s College London. doi:10.18742/PUB01-020
Bach, F., Çakmak, H., Maaß, H., Bekrater-Bodmann, R., Foell, J., Diers, M., et al. (2012). Illusory hand ownership induced by an MRI compatible immersive virtual reality device. Biomed. Eng./Biomed. Tech. 57, 718–720. doi:10.1515/bmt-2012-4084
Banakou, D., Groten, R., and Slater, M. (2013). Illusory ownership of a virtual child body causes overestimation of object sizes and implicit attitude changes. Proc. Natl. Acad. Sci. 110, 12846–12851. doi:10.1073/pnas.1306779110
Banakou, D., Hanumanthu, P. D., and Slater, M. (2016). Virtual embodiment of white people in a black virtual body leads to a sustained reduction in their implicit racial bias. Front. Hum. Neurosci. 10, 601. doi:10.3389/fnhum.2016.00601
Banakou, D., Kishore, S., and Slater, M. (2018). Virtually being Einstein results in an improvement in cognitive task performance and a decrease in age bias. Front. Psychol. 9, 917. doi:10.3389/fpsyg.2018.00917
Bays, P. M., Flanagan, J. R., and Wolpert, D. M. (2006). Attenuation of self-generated tactile sensations is predictive, not postdictive. PLoS Biol. 4, e28. doi:10.1371/journal.pbio.0040028
Bekrater-Bodmann, R., Foell, J., Diers, M., Kamping, S., Rance, M., Kirsch, P., et al. (2014). The importance of synchrony and temporal order of visual and tactile input for illusory limb ownership experiences – an fMRI study applying virtual reality. PLOS ONE 9, e87013. doi:10.1371/journal.pone.0087013
Blakemore, S.-J., Frith, C. D., and Wolpert, D. M. (1999). Spatio-temporal prediction modulates the perception of self-produced stimuli. J. Cognitive Neurosci. 11, 551–559. doi:10.1162/089892999563607
Blakemore, S.-J., Wolpert, D. M., and Frith, C. D. (1998). Central cancellation of self-produced tickle sensation. Nat. Neurosci. 1, 635–640. doi:10.1038/2870
Blanke, O., and Arzy, S. (2005). The out-of-body experience: disturbed self-processing at the temporo-parietal junction. Neurosci. 11, 16–24. doi:10.1177/1073858404270885
Blanke, O., Landis, T., Spinelli, L., and Seeck, M. (2004). Out-of-body experience and autoscopy of neurological origin. Brain 127, 243–258. doi:10.1093/brain/awh040
Blanke, O., and Metzinger, T. (2009). Full-body illusions and minimal phenomenal selfhood. Trends Cognitive Sci. 13, 7–13. doi:10.1016/j.tics.2008.10.003
Blanke, O., Slater, M., and Serino, A. (2015). Behavioral, neural, and computational principles of bodily self-consciousness. Neuron 88, 145–166. doi:10.1016/j.neuron.2015.09.029
Blefari, M. L., Cipriani, C., and Carrozza, M. C. (2011). A novel method for assessing sense of body ownership using electroencephalography. IEEE Trans. Biomed. Eng. 58, 12–15. doi:10.1109/TBME.2010.2076282
Botvinick, M., and Cohen, J. (1998). Rubber hands “feel” touch that eyes see. Nature 391, 756. doi:10.1038/35784
Bourdin, P., Barberia, I., Oliva, R., and Slater, M. (2017). A virtual out-of-body experience reduces fear of death. PLOS ONE 12, e0169343. doi:10.1371/journal.pone.0169343
Bouville, R., Gouranton, V., Boggini, T., Nouviale, F., and Arnaldi, B. (2015). “#FIVE: high-level components for developing collaborative and interactive virtual environments,” in 2015 IEEE 8th Workshop on Software Engineering and Architectures for Realtime Interactive Systems (SEARIS), Arles, France, March 24 2015 to March 24 2015. doi:10.1109/SEARIS.2015.7854099
Braun, N., Debener, S., Spychala, N., Bongartz, E., Sörös, P., Müller, H. H. O., et al. (2018). The senses of agency and ownership: a review. Front. Psychol. 9, 535. doi:10.3389/fpsyg.2018.00535
Brownstein, M. (2019). “Implicit bias,” in The stanford encyclopedia of philosophy. Editor E. N. Zalta (Metaphysics Research Lab, Stanford University).
Burin, D., Pignolo, C., Ales, F., Giromini, L., Pyasik, M., Ghirardello, D., et al. (2019). Relationships between personality features and the rubber hand illusion: an exploratory study. Front. Psychol. 10, 2762. doi:10.3389/fpsyg.2019.02762
Cardoso-Leite, P., Mamassian, P., Schütz-Bosbach, S., and Waszak, F. (2010). A new look at sensory attenuation: action-effect anticipation affects sensitivity, not response bias. Psychol. Sci. 21, 1740–1745. doi:10.1177/0956797610389187
Cauda, F., D’Agata, F., Sacco, K., Duca, S., Geminiani, G., and Vercelli, A. (2011). Functional connectivity of the insula in the resting brain. NeuroImage 55, 8–23. doi:10.1016/j.neuroimage.2010.11.049
Chandler, T., Cordeil, M., Czauderna, T., Dwyer, T., Glowacki, J., Goncu, C., et al. (2015). Immersive analytics. United States: IEEE Computer Society. doi:10.1109/BDVA.2015.7314296
Chen, W.-Y., Huang, H.-C., Lee, Y.-T., and Liang, C. (2018). Body ownership and the four-hand illusion. Sci. Rep. 8, 2153. doi:10.1038/s41598-018-19662-x
Claude, G., Gouranton, V., Berthelot, R. B., and Arnaldi, B. (2014). Short paper: #SEVEN, a sensor effector based scenarios model for driving collaborative virtual environment.
Critchley, H. D., Wiens, S., Rotshtein, P., Öhman, A., and Dolan, R. J. (2004). Neural systems supporting interoceptive awareness. Nat. Neurosci. 7, 189–195. doi:10.1038/nn1176
David, N., Bewernick, B. H., Cohen, M. X., Newen, A., Lux, S., Fink, G. R., et al. (2006). Neural representations of self versus other: visual-spatial perspective taking and agency in a virtual ball-tossing game. J. Cognitive Neurosci. 18, 898–910. doi:10.1162/jocn.2006.18.6.898
David, N., Newen, A., and Vogeley, K. (2008). The “sense of agency” and its underlying cognitive and neural mechanisms. Conscious. Cognition 17, 523–534. doi:10.1016/j.concog.2008.03.004
De Preester, H., and Tsakiris, M. (2009). Body-extension versus body-incorporation: is there a need for a body-model? Phenomenology Cognitive Sci. 8, 307–319. doi:10.1007/s11097-009-9121-y
de Vignemont, F. (2011). Embodiment, ownership and disownership. Conscious. Cognition 12. doi:10.1016/j.concog.2010.09.004
Dewey, J. A., and Knoblich, G. (2014). Do implicit and explicit measures of the sense of agency measure the same thing? PLoS ONE 9, e110118. doi:10.1371/journal.pone.0110118
Dewez, D., Fribourg, R., Argelaguet, F., Hoyet, L., Mestre, D., Slater, M., et al. (2019). “Influence of personality traits and body awareness on the sense of embodiment in virtual reality,” in 2019 IEEE International Symposium on Mixed and Augmented Reality (ISMAR), Beijing, China, Oct. 14 2019 to Oct. 18 2019, 123–134. doi:10.1109/ISMAR.2019.00-12
Dobricki, M., and de la Rosa, S. (2013). The structure of conscious bodily self-perception during full-body illusions. PLoS ONE 8, e83840. doi:10.1371/journal.pone.0083840
Donker, T., Cornelisz, I., van Klaveren, C., van Straten, A., Carlbring, P., Cuijpers, P., et al. (2019). Effectiveness of self-guided app-based virtual reality cognitive behavior therapy for acrophobia: a randomized clinical trial. JAMA Psychiatry 76, 682–690. doi:10.1001/jamapsychiatry.2019.0219
Dwyer, T., Marriott, K., Isenberg, T., Klein, K., Riche, N., Schreiber, F., et al. (2018). “Immersive analytics: an introduction,” in Immersive analytics. Editors K. Marriott, F. Schreiber, T. Dwyer, K. Klein, N. H. Riche, T. Itohet al. (Cham: Springer International Publishing), 11190, 1–23. doi:10.1007/978-3-030-01388-2_1
Ehrsson, H. H. (2007). The experimental induction of out-of-body experiences. Science 317, 1048. doi:10.1126/science.1142175
Ehrsson, H. H., Fotopoulou, A., Radziun, D., Longo, M. R., and Tsakiris, M. (2022). No specific relationship between hypnotic suggestibility and the rubber hand illusion. Nat. Commun. 13, 564–573. doi:10.1038/s41467-022-28177-z
Ehrsson, H. H., Holmes, N. P., and Passingham, R. E. (2005). Touching a rubber hand: feeling of body ownership is associated with activity in multisensory brain areas. J. Neurosci. 25, 10564–10573. doi:10.1523/JNEUROSCI.0800-05.2005
Ehrsson, H. H., Spence, C., and Passingham, R. E. (2004). That’s my hand! Activity in premotor cortex reflects feeling of ownership of a limb. Sci. New Ser. 305, 875–877. doi:10.1126/science.1097011
Ehrsson, H. H., Wiech, K., Weiskopf, N., Dolan, R. J., and Passingham, R. E. (2007). Threatening a rubber hand that you feel is yours elicits a cortical anxiety response. Proc. Natl. Acad. Sci. 104, 9828–9833. doi:10.1073/pnas.0610011104
Eubanks, J. C., Moore, A. G., Fishwick, P. A., and McMahan, R. P. (2021). A preliminary embodiment short questionnaire. Front. Virtual Real. 2. doi:10.3389/frvir.2021.647896
Evans, N., and Blanke, O. (2013). Shared electrophysiology mechanisms of body ownership and motor imagery. NeuroImage 64, 216–228. doi:10.1016/j.neuroimage.2012.09.027
Farrer, C., Franck, N., Georgieff, N., Frith, C., Decety, J., and Jeannerod, M. (2003). Modulating the experience of agency: a positron emission tomography study. NeuroImage 18, 324–333. doi:10.1016/S1053-8119(02)00041-1
Farrer, C., and Frith, C. (2002). Experiencing oneself vs another person as being the cause of an action: the neural correlates of the experience of agency. NeuroImage 15, 596–603. doi:10.1006/nimg.2001.1009
Felton, W. M., and Jackson, R. E. (2022). Presence: a review. Int. J. Human–Computer Interact. 38, 1–18. doi:10.1080/10447318.2021.1921368
Freeling, B., Lecuyer, F., and Capobianco, A. (2022). “Petting a cat helps you incarnate the avatar: influence on the emotions over embodiment in VR,” in 21st IEEE International Symposium on Mixed and Augmented Reality (ISMAR), Singapore, 17 Oct 2022 – 21 Oct 2022.
Fribourg, R., Argelaguet, F., Hoyet, L., and Lecuyer, A. (2018). “Studying the sense of embodiment in VR shared experiences,” in 2018 IEEE Conference on Virtual Reality and 3D User Interfaces, 27 March – 3 April, 273–280. doi:10.1109/VR.2018.8448293
Fribourg, R., Argelaguet, F., Lecuyer, A., and Hoyet, L. (2020). Avatar and sense of embodiment: studying the relative preference between appearance, control and point of view. IEEE Trans. Vis. Comput. Graph. 26, 2062–2072. doi:10.1109/TVCG.2020.2973077
Fribourg, R., Blanpied, E., Hoyet, L., Lécuyer, A., and Argelaguet, F. (2021). Does virtual threat harm VR experience? impact of threat occurrence and repeatability on virtual embodiment and threat response. Comput. Graph. 100, 125–136. doi:10.1016/j.cag.2021.07.017
Frith, C. D. (1992). The cognitive neuropsychology of schizophrenia. United Kingdom: Psychology press.
Gallagher, S. (2000). Philosophical conceptions of the self: implications for cognitive science. Trends Cognitive Sci. 4, 14–21. doi:10.1016/S1364-6613(99)01417-5
Gallagher, S. (2012). Multiple aspects in the sense of agency. New Ideas Psychol. 30, 15–31. doi:10.1016/j.newideapsych.2010.03.003
Gentsch, A., and Schütz-Bosbach, S. (2011). I did it: unconscious expectation of sensory consequences modulates the experience of self-agency and its functional signature. J. Cognitive Neurosci. 23, 3817–3828. doi:10.1162/jocn_a_00012
George, L., Marchal, M., Glondu, L., and Lécuyer, A. (2012). “Combining brain-computer interfaces and haptics: detecting mental workload to adapt haptic assistance,” in Haptics: perception, devices, mobility, and communication. Editors P. Isokoski, and J. Springare (Berlin, Heidelberg: Springer). doi:10.1007/978-3-642-31401-8_12
Gonzalez-Franco, M., and Peck, T. C. (2018). Avatar embodiment. Towards a standardized questionnaire. Front. Robotics AI 5, 74. doi:10.3389/frobt.2018.00074
González-Franco, M., Peck, T. C., Rodríguez-Fornells, A., and Slater, M. (2014). A threat to a virtual hand elicits motor cortex activation. Exp. Brain Res. 232, 875–887. doi:10.1007/s00221-013-3800-1
Gonzalez-Franco, M., Perez-Marcos, D., Spanlang, B., and Slater, M. (2010). “The contribution of real-time mirror reflections of motor actions on virtual body ownership in an immersive virtual environment,” in 2010 IEEE Virtual Reality Conference (VR), Waltham, MA, 111–114. doi:10.1109/VR.2010.5444805
Graziano, M. S. A., and Cooke, D. F. (2006). Parieto-frontal interactions, personal space, and defensive behavior. Neuropsychologia 44, 845–859. doi:10.1016/j.neuropsychologia.2005.09.009
Guy, M., Jeunet-Kelway, C., Moreau, G., and Normand, J.-M. (2022). “Manipulating the sense of embodiment in virtual reality: a study of the interactions between the senses of agency, self-location and ownership,” in ICAT-EGVE 2022 - international conference on artificial reality and telexistence and eurographics symposium on virtual environments. Editors H. Uchiyama, and J.-M. Normand (Yokohama, Japan: The Eurographics Association). doi:10.2312/egve.20221281
Haggard, P. (2017). Sense of agency in the human brain. Nat. Rev. Neurosci. 18, 196–207. doi:10.1038/nrn.2017.14
Haggard, P., and Chambon, V. (2012). Sense of agency. Curr. Biol. 22, R390–R392. doi:10.1016/j.cub.2012.02.040
Haggard, P., and Clark, S. (2003). Intentional action: conscious experience and neural prediction. Conscious. Cognition 12, 695–707. doi:10.1016/S1053-8100(03)00052-7
Halligan, P. W., Fink, G. R., Marshall, J. C., and Vallar, G. (2003). Spatial cognition: evidence from visual neglect. Trends Cognitive Sci. 7, 125–133. doi:10.1016/S1364-6613(03)00032-9
Hassan, A., and Josephs, K. A. (2016). Alien hand syndrome. Curr. Neurology Neurosci. Rep. 16, 73. doi:10.1007/s11910-016-0676-z
Hatfield, G. C. (1990). The natural and the normative: theories of spatial perception from kant to helmholtz. United States: MIT Press. Google-Books-ID: JikeeDbYeUQC.
Hohwy, J., and Paton, B. (2010). Explaining away the body: experiences of supernaturally caused touch and touch on non-hand objects within the rubber hand illusion. PLOS ONE 5, e9416. doi:10.1371/journal.pone.0009416
Holmes, N. P., Crozier, G., and Spence, C. (2004). When mirrors lie: “Visual capture” of arm position impairs reaching performance. Cognitive, Affect. Behav. Neurosci. 4, 193–200. doi:10.3758/CABN.4.2.193
Holmes, N. P., Snijders, H. J., and Spence, C. (2006). Reaching with alien limbs: visual exposure to prosthetic hands in a mirror biases proprioception without accompanying illusions of ownership. Percept. Psychophys. 68, 685–701. doi:10.3758/BF03208768
Horváth, J. (2015). Action-related auditory ERP attenuation: paradigms and hypotheses. Brain Res. 1626, 54–65. doi:10.1016/j.brainres.2015.03.038
Ionta, S., Heydrich, L., Lenggenhager, B., Mouthon, M., Fornari, E., Chapuis, D., et al. (2011). Multisensory mechanisms in temporo-parietal cortex support self-location and first-person perspective. Neuron 70, 363–374. doi:10.1016/j.neuron.2011.03.009
Jahedi, S., and Méndez, F. (2014). On the advantages and disadvantages of subjective measures. J. Econ. Behav. Organ. 98, 97–114. doi:10.1016/j.jebo.2013.12.016
Jeunet, C., Albert, L., Argelaguet, F., and Lecuyer, A. (2018). “Do you feel in control?”: towards novel approaches to characterise, manipulate and measure the sense of agency in virtual environments. IEEE Trans. Vis. Comput. Graph. 24, 1486–1495. doi:10.1109/TVCG.2018.2794598
Kalckert, A., and Ehrsson, H. H. (2012). Moving a rubber hand that feels like your own: a dissociation of ownership and agency. Front. Hum. Neurosci. 6, 40. doi:10.3389/fnhum.2012.00040
Kammers, M. P., Rose, K., and Haggard, P. (2011). Feeling numb: temperature, but not thermal pain, modulates feeling of body ownership. Neuropsychologia 49, 1316–1321. doi:10.1016/j.neuropsychologia.2011.02.039
Kang, S. Y., Im, C.-H., Shim, M., Nahab, F. B., Park, J., Kim, D.-W., et al. (2015). Brain networks responsible for sense of agency: an EEG study. PLOS ONE 10, e0135261. doi:10.1371/journal.pone.0135261
Kilteni, K., Bergstrom, I., and Slater, M. (2013). Drumming in immersive virtual reality: the body shapes the way we play. IEEE Trans. Vis. Comput. Graph. 19, 597–605. doi:10.1109/tvcg.2013.29
Kilteni, K., Groten, R., and Slater, M. (2012a). The sense of embodiment in virtual reality. Presence Teleoperators Virtual Environ. 21, 373–387. doi:10.1162/PRES_a_00124
Kilteni, K., Maselli, A., Kording, K. P., and Slater, M. (2015). Over my fake body: body ownership illusions for studying the multisensory basis of own-body perception. Front. Hum. Neurosci. 9, 141. doi:10.3389/fnhum.2015.00141
Kilteni, K., Normand, J.-M., Sanchez-Vives, M. V., and Slater, M. (2012b). Extending body space in immersive virtual reality: a very long arm illusion. PLoS ONE 7, e40867. doi:10.1371/journal.pone.0040867
Kirsch, I., and Braffman, W. (2001). Imaginative suggestibility and hypnotizability. Curr. Dir. Psychol. Sci. 10, 57–61. doi:10.1111/1467-8721.00115
Kirsch, I., and Council, J. R. (1989). “Response expectancy as a determinant of hypnotic behavior,” in Hypnosis: the cognitive-behavioral perspective (Amherst, NY, US: Prometheus Books), 360–379.
Kocur, M., Kalus, A., Bogon, J., Henze, N., Wolff, C., and Schwind, V. (2022). “The rubber hand illusion in virtual reality and the real world-comparable but different,” in 28th ACM Symposium on Virtual Reality Software and Technology, Christchurch, New Zealand, 9-11 October 2023.
Kühn, S., Nenchev, I., Haggard, P., Brass, M., Gallinat, J., and Voss, M. (2011). Whodunnit? Electrophysiological correlates of agency judgements. PLoS ONE 6, e28657. doi:10.1371/journal.pone.0028657
Latoschik, M. E., Roth, D., Gall, D., Achenbach, J., Waltemate, T., and Botsch, M. (2017). “The effect of avatar realism in immersive social virtual realities,” in Proceedings of the 23rd ACM Symposium on Virtual Reality Software and Technology, New York, NY, USA, November 8 - 10, 2017, 1–10. doi:10.1145/3139131.3139156
Lenggenhager, B., Halje, P., and Blanke, O. (2011). Alpha band oscillations correlate with illusory self-location induced by virtual reality. Eur. J. Neurosci. 33, 1935–1943. doi:10.1111/j.1460-9568.2011.07647.x
Lenggenhager, B., Mouthon, M., and Blanke, O. (2009). Spatial aspects of bodily self-consciousness. Conscious. Cognition 8, 110–117. doi:10.1016/j.concog.2008.11.003
Lenggenhager, B., Tadi, T., Metzinger, T., and Blanke, O. (2007). Video ergo sum: manipulating bodily self-consciousness. Science 317, 1096–1099. doi:10.1126/science.1143439
Levenson, R. W., Ekman, P., and Friesen, W. V. (1990). Voluntary facial action generates emotion-specific autonomic nervous system activity. Psychophysiology 27, 363–384. doi:10.1111/j.1469-8986.1990.tb02330.x
Lin, L., and Jörg, S. (2016). “Need a hand? how appearance affects the virtual hand illusion,” in Proceedings of the ACM Symposium on Applied Perception, Anaheim, California, USA, July 22-23, 2016, 69–76. doi:10.1145/2931002.2931006
Lindner, P. (2020). Better, virtually: the past, present, and future of virtual reality cognitive behavior therapy. Int. J. Cognitive Ther. 14, 23–46. doi:10.1007/s41811-020-00090-7
Llobera, J., Sanchez-Vives, M. V., and Slater, M. (2013). The relationship between virtual body ownership and temperature sensitivity. J. R. Soc. Interface 10, 20130300. doi:10.1098/rsif.2013.0300
Longo, M. R., Cardozo, S., and Haggard, P. (2008a). Visual enhancement of touch and the bodily self. Conscious. Cognition 17, 1181–1191. doi:10.1016/j.concog.2008.01.001
Longo, M. R., Schüür, F., Kammers, M. P., Tsakiris, M., and Haggard, P. (2008b). What is embodiment? A psychometric approach. Cognition 107, 978–998. doi:10.1016/j.cognition.2007.12.004
Lopez, C., Falconer, C., Deroualle, D., and Mast, F. (2015). In the presence of others: self-location, balance control and vestibular processing. Neurophysiol. Clinique/Clinical Neurophysiol. 45, 241–254. doi:10.1016/j.neucli.2015.09.001
Lopez, C., Halje, P., and Blanke, O. (2008). Body ownership and embodiment: vestibular and multisensory mechanisms. Neurophysiol. Clinique/Clinical Neurophysiol. 38, 149–161. doi:10.1016/j.neucli.2007.12.006
Luck, S. J. (2014). An introduction to the event-related potential technique. United states: MIT press.
Lugrin, J.-L., Landeck, M., and Latoschik, M. E. (2015a). “Avatar embodiment realism and virtual fitness training,” in 2015 IEEE Virtual Reality (VR), Arles, Camargue, Provence, France, 23-27 March 2015, 225–226. doi:10.1109/VR.2015.7223377
Lugrin, J.-L., Latt, J., and Latoschik, M. E. (2015b). “Anthropomorphism and illusion of virtual body ownership,” in ICAT-EGVE 2015 - International Conference on Artificial Reality and Telexistence and Eurographics Symposium on Virtual Environments, Kyoto, Japan, October 28 2015.
Lush, P. (2020). Demand characteristics confound the rubber hand illusion. Collabra Psychol. 6, 22. doi:10.1525/collabra.325
Lush, P., Botan, V., Scott, R. B., Seth, A., Ward, J., and Dienes, Z. (2019). Phenomenological control: response to imaginative suggestion predicts measures of mirror touch synaesthesia, vicarious pain and the rubber hand illusion. Tech. Rep. PsyArXiv. doi:10.31234/osf.io/82jav
Lush, P., Botan, V., Scott, R. B., Seth, A. K., Ward, J., and Dienes, Z. (2020). Trait phenomenological control predicts experience of mirror synaesthesia and the rubber hand illusion. Nat. Commun. 11, 4853. doi:10.1038/s41467-020-18591-6
Ma, K., and Hommel, B. (2015). Body-ownership for actively operated non-corporeal objects. Conscious. cognition 36, 75–86. doi:10.1016/j.concog.2015.06.003
Makin, T. R., Holmes, N. P., and Ehrsson, H. H. (2008). On the other hand: dummy hands and peripersonal space. Behav. Brain Res. 191, 1–10. doi:10.1016/j.bbr.2008.02.041
Martikainen, M. H. (2004). Suppressed responses to self-triggered sounds in the human auditory cortex. Cereb. Cortex 15, 299–302. doi:10.1093/cercor/bhh131
Maselli, A., and Slater, M. (2013). The building blocks of the full body ownership illusion. Front. Hum. Neurosci. 7, 83. doi:10.3389/fnhum.2013.00083
Maselli, A., and Slater, M. (2014). Sliding perspectives: dissociating ownership from self-location during full body illusions in virtual reality. Front. Hum. Neurosci. 8, 693. doi:10.3389/fnhum.2014.00693
Moseley, G. L., Olthof, N., Venema, A., Don, S., Wijers, M., Gallace, A., et al. (2008). Psychologically induced cooling of a specific body part caused by the illusory ownership of an artificial counterpart. Proc. Natl. Acad. Sci. 105, 13169–13173. doi:10.1073/pnas.0803768105
Muller-Putz, G., Scherer, R., Neuper, C., and Pfurtscheller, G. (2006). Steady-state somatosensory evoked potentials: suitable brain signals for brain-computer interfaces? IEEE Trans. Neural Syst. Rehabilitation Eng. 14, 30–37. doi:10.1109/TNSRE.2005.863842
Nakul, E., Orlando-Dessaints, N., Lenggenhager, B., and Lopez, C. (2020). Measuring perceived self-location in virtual reality. Sci. Rep. 10, 6802. doi:10.1038/s41598-020-63643-y
Newport, R., Pearce, R., and Preston, C. (2010). Fake hands in action: embodiment and control of supernumerary limbs. Exp. Brain Res. 204, 385–395. doi:10.1007/s00221-009-2104-y
Nisbet, E., and Zelenski, J. (2013). The NR-6: a new brief measure of nature relatedness. Front. Psychol. 4, 813. doi:10.3389/fpsyg.2013.00813
Normand, J.-M., Giannopoulos, E., Spanlang, B., and Slater, M. (2011). Multisensory stimulation can induce an illusion of larger belly size in immersive virtual reality. PLoS ONE 6, e16128. doi:10.1371/journal.pone.0016128
Olson, J. A., Suissa-Rocheleau, L., Lifshitz, M., Raz, A., and Veissière, S. P. L. (2020). Tripping on nothing: placebo psychedelics and contextual factors. Psychopharmacology 237, 1371–1382. doi:10.1007/s00213-020-05464-5
Orne, M. T. (2009). Demand characteristics and the concept of quasi-controls. Artifacts Behav. Res. Robert Rosenthal Ralph L. Rosnow’s Class. books 110, 110–137. doi:10.1093/acprof:oso/9780195385540.003.0005
Padrao, G., Gonzalez-Franco, M., Sanchez-Vives, M. V., Slater, M., and Rodriguez-Fornells, A. (2016). Violating body movement semantics: neural signatures of self-generated and external-generated errors. NeuroImage 124, 147–156. doi:10.1016/j.neuroimage.2015.08.022
Peck, T. C., and Gonzalez-Franco, M. (2021). Avatar embodiment. A standardized questionnaire. Front. Virtual Real. 1. doi:10.3389/frvir.2020.575943
Peck, T. C., Seinfeld, S., Aglioti, S. M., and Slater, M. (2013). Putting yourself in the skin of a black avatar reduces implicit racial bias. Conscious. Cognition 22, 779–787. doi:10.1016/j.concog.2013.04.016
Petkova, V. I., and Ehrsson, H. H. (2008). If I were you: perceptual illusion of body swapping. PLoS ONE 3, e3832. doi:10.1371/journal.pone.0003832
Petkova, V. I., Khoshnevis, M., and Ehrsson, H. H. (2011). The perspective matters! Multisensory integration in ego-centric reference frames determines full-body ownership. Front. Psychol. 2, 35. doi:10.3389/fpsyg.2011.00035
Pomés, A., and Slater, M. (2013). Drift and ownership toward a distant virtual body. Front. Hum. Neurosci. 7, 908. doi:10.3389/fnhum.2013.00908
Rabellino, D., Frewen, P. A., McKinnon, M. C., and Lanius, R. A. (2020). Peripersonal space and bodily self-consciousness: implications for psychological trauma-related disorders. Front. Neurosci. 14, 586605. doi:10.3389/fnins.2020.586605
Reader, A. T. (2021). What do participants expect to experience in the rubber hand illusion? a conceptual replication of lush.
Roth, D., and Latoschik, M. E. (2020). Construction of the virtual embodiment questionnaire (VEQ). IEEE Trans. Vis. Comput. Graph. 26, 3546–3556. doi:10.1109/tvcg.2020.3023603
Roth, D., Lugrin, J.-L., Latoschik, M. E., and Huber, S. (2017). “Alpha IVBO - construction of a scale to measure the illusion of virtual body ownership,” in Proceedings of the 2017 CHI Conference Extended Abstracts on Human Factors in Computing Systems, New York, NY, USA, May 06-11, 2017, 2875–2883. doi:10.1145/3027063.3053272
Sato, A. (2008). Action observation modulates auditory perception of the consequence of others’ actions. Conscious. Cognition 17, 1219–1227. doi:10.1016/j.concog.2008.01.003
Sauvan, J.-B., Lécuyer, A., Lotte, F., and Casiez, G. (2009). “A performance model of selection techniques for p300-based brain-computer interfaces,” in Proceedings of the SIGCHI Conference on Human Factors in Computing Systems, Boston MA USA, November 9-10, 2009, 2205–2208. doi:10.1145/1518701.1519037
Seghezzi, S., Giannini, G., and Zapparoli, L. (2019). Neurofunctional correlates of body-ownership and sense of agency: a meta-analytical account of self-consciousness. Cortex 121, 169–178. doi:10.1016/j.cortex.2019.08.018
Serino, A., Alsmith, A., Costantini, M., Mandrigin, A., Tajadura-Jimenez, A., and Lopez, C. (2013). Bodily ownership and self-location: components of bodily self-consciousness. Conscious. Cognition 22, 1239–1252. doi:10.1016/j.concog.2013.08.013
Seth, A., Roseboom, W., Dienes, Z., and Lush, P. (2021). What’s up with the rubber hand illusion? Preprint. PsyArXiv. doi:10.31234/osf.io/b4qcy
Si-Mohammed, H., Petit, J., Jeunet, C., Argelaguet, F., Spindler, F., Évain, A., et al. (2020). Towards BCI-based interfaces for augmented reality: feasibility, design and evaluation. IEEE Trans. Vis. Comput. Graph. 26, 1608–1621. doi:10.1109/TVCG.2018.2873737
Slater, M. (2004). How colorful was your day? Why questionnaires cannot assess presence in virtual environments. Presence Teleoperators Virtual Environ. 13, 484–493. doi:10.1162/1054746041944849
Slater, M. (2009). Place illusion and plausibility can lead to realistic behaviour in immersive virtual environments. Philosophical Trans. R. Soc. B Biol. Sci. 364, 3549–3557. doi:10.1098/rstb.2009.0138
Slater, M., and Ehrsson, H. H. (2022). Multisensory integration dominates hypnotisability and expectations in the rubber hand illusion. Front. Hum. Neurosci. 317, 834492. doi:10.3389/fnhum.2022.834492
Slater, M., Pérez Marcos, D., Ehrsson, H., and Sanchez-Vives, M. (2009). Inducing illusory ownership of a virtual body. Front. Neurosci. 3, 29. doi:10.3389/neuro.01.029.2009
Slater, M., Perez-Marcos, D., Ehrsson, H. H., and Sanchez-Vives, M. V. (2008). Towards a digital body: the virtual arm illusion. Front. Hum. Neurosci. 2, 6. doi:10.3389/neuro.09.006.2008
Slater, M., Spanlang, B., Sanchez-Vives, M. V., and Blanke, O. (2010). First person experience of body transfer in virtual reality. PLoS ONE 5, e10564. doi:10.1371/journal.pone.0010564
Spangenberger, P., Geiger, S. M., and Freytag, S.-C. (2022). Becoming nature: effects of embodying a tree in immersive virtual reality on nature relatedness. Sci. Rep. 12, 1311. doi:10.1038/s41598-022-05184-0
Sperduti, M., Delaveau, P., Fossati, P., and Nadel, J. (2011). Different brain structures related to self- and external-agency attribution: a brief review and meta-analysis. Brain Struct. Funct. 216, 151–157. doi:10.1007/s00429-010-0298-1
Steed, A., Pan, Y., Zisch, F., and Steptoe, W. (2016). “The impact of a self-avatar on cognitive load in immersive virtual reality,” in 2016 IEEE Virtual Reality (VR), Greenville, SC, USA, 67–76. doi:10.1109/VR.2016.7504689
Synofzik, M., Vosgerau, G., and Newen, A. (2008). Beyond the comparator model: a multifactorial two-step account of agency. Conscious. Cognition 17, 219–239. doi:10.1016/j.concog.2007.03.010
Tauscher, J.-P., Schottky, F. W., Grogorick, S., Bittner, P. M., Mustafa, M., and Magnor, M. (2019). “Immersive EEG: evaluating electroencephalography in virtual reality,” in 2019 IEEE Conference on Virtual Reality and 3D User Interfaces (VR), Osaka, March 27th, 2019, 1794–1800. doi:10.1109/VR.2019.8797858
Tsakiris, M. (2010). My body in the brain: a neurocognitive model of body-ownership. Neuropsychologia 48, 703–712. doi:10.1016/j.neuropsychologia.2009.09.034
Tsakiris, M., Carpenter, L., James, D., and Fotopoulou, A. (2010a). Hands only illusion: multisensory integration elicits sense of ownership for body parts but not for non-corporeal objects. Exp. Brain Res. 204, 343–352. doi:10.1007/s00221-009-2039-3
Tsakiris, M., and Haggard, P. (2003). Awareness of somatic events associated with a voluntary action. Exp. Brain Res. 149, 439–446. doi:10.1007/s00221-003-1386-8
Tsakiris, M., and Haggard, P. (2005). The rubber hand illusion revisited: visuotactile integration and self-attribution. J. Exp. Psychol. Hum. Percept. Perform. 31, 80–91. doi:10.1037/0096-1523.31.1.80
Tsakiris, M., Longo, M. R., and Haggard, P. (2010b). Having a body versus moving your body: neural signatures of agency and body-ownership. Neuropsychologia 48, 2740–2749. doi:10.1016/j.neuropsychologia.2010.05.021
Tsakiris, M., Schütz-Bosbach, S., and Gallagher, S. (2007). On agency and body-ownership: phenomenological and neurocognitive reflections. Conscious. Cognition 16, 645–660. doi:10.1016/j.concog.2007.05.012
Tuthill, J. C., and Azim, E. (2018). Proprioception. Curr. Biol. 28, R194–R203. doi:10.1016/j.cub.2018.01.064
Verhulst, A., Normand, J.-M., Lombart, C., Sugimoto, M., and Moreau, G. (2018). Influence of being embodied in an obese virtual body on shopping behavior and products perception in VR. Front. Robotics AI 5, 113. doi:10.3389/frobt.2018.00113
Vogeley, K., May, M., Ritzl, A., Falkai, P., Zilles, K., and Fink, G. R. (2004). Neural correlates of first-person perspective as one constituent of human self-consciousness. J. Cognitive Neurosci. 16, 817–827. doi:10.1162/089892904970799
Wallach, H. S., Safir, M. P., and Bar-Zvi, M. (2009). Virtual reality cognitive behavior therapy for public speaking anxiety: a randomized clinical trial. Behav. Modif. 33, 314–338. doi:10.1177/0145445509331926
Waltemate, T., Gall, D., Roth, D., Botsch, M., and Latoschik, M. E. (2018). The impact of avatar personalization and immersion on virtual body ownership, presence, and emotional response. IEEE Trans. Vis. Comput. Graph. 24, 1643–1652. doi:10.1109/TVCG.2018.2794629
Waltemate, T., Senna, I., Hülsmann, F., Rohde, M., Kopp, S., Ernst, M., et al. (2016). “The impact of latency on perceptual judgments and motor performance in closed-loop interaction in virtual reality,” in Proceedings of the 22nd ACM Conference on Virtual Reality Software and Technology, Munich Germany, 27–35. doi:10.1145/2993369.2993381
Wegner, D. M., and Wheatley, T. (1999). Apparent mental causation. Am. Psychol. 13. doi:10.1037/0003-066X.54.7.480
Weiskopf, N., Mathiak, K., Bock, S., Scharnowski, F., Veit, R., Grodd, W., et al. (2004). Principles of a brain-computer interface (BCI) based on real-time functional magnetic resonance imaging (fMRI). IEEE Trans. Biomed. Eng. 51, 966–970. doi:10.1109/TBME.2004.827063
Weiskrantz, L., Elliott, J., and Darlington, C. (1971). Preliminary observations on tickling oneself. Nature 230, 598–599. doi:10.1038/230598a0
Weiss, C., Herwig, A., and Schütz-Bosbach, S. (2011). The self in action effects: selective attenuation of self-generated sounds. Cognition 121, 207–218. doi:10.1016/j.cognition.2011.06.011
Weller, L., Schwarz, K. A., Kunde, W., and Pfister, R. (2017). Was it me? – Filling the interval between action and effects increases agency but not sensory attenuation. Biol. Psychol. 123, 241–249. doi:10.1016/j.biopsycho.2016.12.015
Weser, V., and Proffitt, D. R. (2019). Tool embodiment: the tool’s output must match the user’s input. Front. Hum. Neurosci. 12, 537. doi:10.3389/fnhum.2018.00537
Wissmath, B., Weibel, D., Schmutz, J., and Mast, F. W. (2011). Being present in more than one place at a time? Patterns of mental self-localization. Conscious. Cognition 20, 1808–1815. doi:10.1016/j.concog.2011.05.008
Yee, N., and Bailenson, J. (2007). The Proteus effect: the effect of transformed self-representation on behavior. Hum. Commun. Res. 33, 271–290. doi:10.1111/j.1468-2958.2007.00299.x
Yuan, Y., and Steed, A. (2010). “Is the rubber hand illusion induced by immersive virtual reality?,” in 2010 IEEE Virtual Reality Conference, Waltham, Massachusetts, USA, March 20-24, 2010. January 2010. doi:10.1109/VR.2010.5444807
Zhang, J., and Hommel, B. (2016). Body ownership and response to threat. Psychol. Res. 80, 1020–1029. doi:10.1007/s00426-015-0698-1
Keywords: virtual reality, review, embodiment, evaluation, assessment method
Citation: Guy M, Normand J-M, Jeunet-Kelway C and Moreau G (2023) The sense of embodiment in Virtual Reality and its assessment methods. Front. Virtual Real. 4:1141683. doi: 10.3389/frvir.2023.1141683
Received: 10 January 2023; Accepted: 06 November 2023;
Published: 05 December 2023.
Edited by:
Arindam Dey, Facebook Reality Labs Research, United StatesCopyright © 2023 Guy, Normand, Jeunet-Kelway and Moreau. This is an open-access article distributed under the terms of the Creative Commons Attribution License (CC BY). The use, distribution or reproduction in other forums is permitted, provided the original author(s) and the copyright owner(s) are credited and that the original publication in this journal is cited, in accordance with accepted academic practice. No use, distribution or reproduction is permitted which does not comply with these terms.
*Correspondence: Martin Guy, bWFydGluZ3V5LWNyZW5hdUBwcm90b25tYWlsLmNvbQ==
†Present address: Jean-Marie Normand, Nantes Université, École Centrale Nantes, CNRS, LS2N, UMR 6004, Nantes, France; Inria Hybrid, Rennes, France
Disclaimer: All claims expressed in this article are solely those of the authors and do not necessarily represent those of their affiliated organizations, or those of the publisher, the editors and the reviewers. Any product that may be evaluated in this article or claim that may be made by its manufacturer is not guaranteed or endorsed by the publisher.
Research integrity at Frontiers
Learn more about the work of our research integrity team to safeguard the quality of each article we publish.