- 1Department of Sports Engineering and Movement Science, Institute III: Sports Science Otto-von-Guericke-University Magdeburg, Magdeburg, Germany
- 2Elbedome, Fraunhofer Institute for Factory Operation and Automation IFF, Magdeburg, Germany
Virtual reality (VR) training has become valuable in sports to improve motor behavior and train specific situations under standardized conditions. However, studies comparing conventional training with VR training are rare, especially for advanced athletes. Furthermore, it remains unclear whether the performance improvement achieved through VR training can be transferred to the real world (RW). Therefore, we present a study analyzing sports-specific response training using a head-mounted display (HMD) combined with conventional training and its transfer to RW. In ten training sessions over 6 weeks, a VR training group (VRG, n = 15) performed virtual karate training (10 min) combined with a conventional training (80 min), while a conventional training group (CG, n = 12) conducted only conventional training (90 min) at the same time. The VR training consisted of the athlete responding to various karate attacks performed by a virtual opponent in a karate-specific manner. The study design included a pretest, an intermediate test (after 5 training sessions), and a posttest. We analyzed sports-specific response behavior concerning the competition-relevant karate attacks Gyaku-Zuki jodan (GZj) and Kizami-Zuki (KZ) using the parameters ‘response time’, and the “response quality” when the athletes had to react to attacks of a virtual opponent in VR and a real opponent in RW. For the parameter “response time,” improvements were detected only for the VRG in VR concerning GZj and KZ. For the parameter “response quality” for both groups, no improvements could be found. Furthermore, athletes provided positive feedback regarding the integration of VR training into conventional training.
1 Introduction
VR technology enables physical training in standardized and controllable experimental conditions. Training situations can be created, which are not feasible in the real world (RW), such as training with opponents at international level (Zaal and Bootsma, 2011; Wang, 2012; Craig, 2013), or more teammates or opponents are available compared to RW. Thus, VR training can be used for standalone training without the predefined time, location and dependence on training partners, coaches, or gyms (Miles et al., 2012; Pan et al., 2012; Petri et al., 2018a). Due to no physical contact with other athletes (opponents or teammates), injury probability is reduced (Zaal and Bootsma, 2011; Miles et al., 2012; Pan et al., 2012).
VR applications in sports training and sports science have been known since 1990 (Neumann et al., 2018). In the last decade, progress has been made in integrating VR into motor learning (Yanovich and Ronen, 2015) or serious games (Dörner et al., 2016). Furthermore, several studies analyzed the effects of VR training in several sports, e.g. table tennis (Michalski et al., 2019), karate (Burns et al., 2011; Petri et al., 2018a; Petri et al., 2019a; Petri et al., 2019b) and baseball (Gray, 2017). Further examples can be found in the overview by Petri, Ohl, Danneberg, Emmermacher, Masik, and Witte (Petri et al., 2018b). As technology advances, HMDs are used instead of power walls and CAVEs, resulting in much greater freedom of movement and presence in VR (Petri et al., 2018c).
For applications of VR in combat sports, virtual opponents or virtual characters are of particular importance. To make the athlete’s reactions to a virtual opponent as realistic as possible, the opponent should be as autonomous as possible. However, as the literature review by Petri et al. (Petri et al., 2018c) states, there are hardly any practical applications of autonomous characters due to the high technological requirements. The study also shows that specific skills of the athlete can be trained with a rather passive character.
Intervention studies on the use of VR training tools have been conducted primarily with less-skilled athletes (Lammfromm and Gopher, 2011; Rauter et al., 2013; Covaci et al., 2015; Tirp et al., 2015; Hülsmann et al., 2019). For high-skilled athletes, there are only a few studies, which used VR intervention, and even fewer studies that analyzed VR training compared to training in a RW environment. Gray (Gray, 2017) had experienced athletes perform RW- and VR training and tested the transfer of perceptual-motor skills in baseball batting in RW. The results showed a greater improvement in the performance of the VR group compared to the RW group (Gray, 2017).
However, the majority of recent studies only investigated the improvements in VR based on VR training and the question regarding the transfer in RW is still open. To study the transfer, environments for real and virtual training situations need to be similar (Covaci et al., 2015; Oagaz et al., 2021).
Currently, there is a lack of intervention studies in different sports using immersive VR with high-skilled athletes and investigating transfer effects from VR to RW. A previous study (Petri et al., 2019a) used a small sample size and examined the improvement of performance only in VR without transfer to RW. The present study aims to repeat this study to verify the results with a larger sample size (n = 27) and an additional control group, as well as to analyze a possible transfer effect. Specifically, this study aims to examine the following assumption. We hypothesize that a combination of conventional training and VR training will result in similar or greater progress in the response behaviour in karate kumite in reality than conventional training itself. It would be possible to supplement conventional training with VR training, which would also have the advantage that a training partner would not necessarily have to be available. Therefore, the response behavior of karate athletes to two competition-relevant attacks was analyzed [Gyaku-Zuki Jodan (GZj) and Kizami-Zuki (KZ)] and compared before, after half of the intervention and after intervention in VR and RW. Both are fist punches, but they differ fundamentally in movement technique and execution time, so that a different response behavior must first be assumed. The parameters ‘response time’ and ‘response quality’ were used to analyze the response behavior. One of the final goals was to find out to what extent VR training is accepted by the athletes.
2 Materials and methods
2.1 Participants
Twenty-seven (n = 27) young karate athletes (17 male, 10 female, age: 17.4 ± 3.52 years) with international and national competitive level were divided into two groups (VRG—VR group, n = 15 and CG—conventional training group, n = 12) considering gender and expertise. All athletes (5th Kyu—1st Dan, shotokan style, average of 10 years experience) have karate kumite training four times per week, each of 90 minutes duration. Participants and their legal guardians were informed about the study and gave their written consent. Coaches with international competition expertise from the respective other training group acted as real opponents. All participants had no experience with HMD. The study was designed and conducted in accordance with the declaration of Helsinki. The approval of the Ethics Committee of the first author’s university was obtained under the number 132/16.
2.2 Timeline of the study
The study lasted 12 weeks (1st—2nd week pretest, 3rd—5th week intervention phase 1, 6th—7th week intermediate test, 8th—10th week intervention phase 2, and 11th—12th week posttest).
During pretest (pre), intermediate test (int), and posttest (post), the karate-specific response behavior was tested in VR and RW. In the pretest, questionnaires and feedback forms for subjective assessments were completed and stereoscopic vision was verified (Titmus Stereoacuity Test by EYESFIRST®).
In the two intervention phases with each five training sessions during 2–3 weeks, VRG absolved 10 min of VR training combined with 80 min of conventional training and CG absolved 90 min of conventional training in each session.
2.3 Intervention
The CG performed the conventional karate training (90 min) under the guidance of a karate coach, while the VRG combined 80 min of the same conventional karate training with 10 min of karate training in VR. The focus of the VR training was the training of karate-specific reactions to given attacks. The training sessions are designed with increasing difficulty regarding the number, type of attacks, and their individual variations (Petri et al., 2019a). In each training session, the athlete had to respond to four sets with each six to eight attacks. There was no direct feedback in VR. However, through the HMD, the athlete could estimate whether the attacker would have hit him. To guarantee regeneration and full concentration of the athletes after each attack, there was a break of 3 s. After each set, a break of 2 min was provided. As usual in conventional training, a coach also gave one or two tips to the athlete during the VR training.
2.4 Virtual opponent
The VR training was performed with a virtual opponent (Petri et al., 2019a). For the attacks GZj (arm attack with the rear hand towards the opponent’s head, Figure 1), KZ (arm attack with the front arm towards the opponent’s head, Figure 2), and other techniques intended to break up the training (but not later analyzed in VR), the karate techniques of five international successful karate kumite athletes (age: 24–56 years, 1st—4th Dan) were recorded using motion capturing with 12 cameras (MX-13, Vicon, United Kingdom) and real-time tracking (ART, Germany). The attacks were saved as animations and transferred to humanoid avatars with different meshes (e.g., female and male) and a karate suit. At least three animations were created for each virtual opponent and each attack technique. During the tests with the virtual opponent and during VR training, the VR was presented using a head-mounted display (HMD, HTC Vive Pro Eye, Taiwan; resolution of 1140x1660 per eye, horizontal field of view 110°). Figure 3 shows the virtual opponent from the athlete’s perspective.
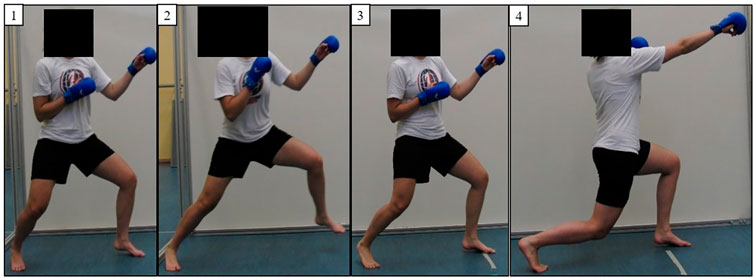
FIGURE 1. Series of pictures demonstrating the karate attack Gyaku-Zuki jodan (GZj). (1) starting position (kamae), (2) distance reduction towards the opponent, (3) pulling up the back leg, (4) pushing off the rear fist to the head of the opponent.
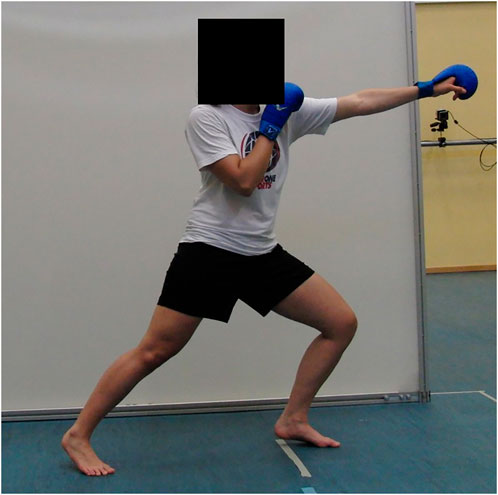
FIGURE 2. Picture demonstrating the final position of the karate attack Kizami Zuki jodan (KZ). Note the difference to GZj (Figure 1)/(4): pushing off the front fist to the head of the opponent. Starting position and distance reduction towards the opponent are the same as for GZj in Figure 1.
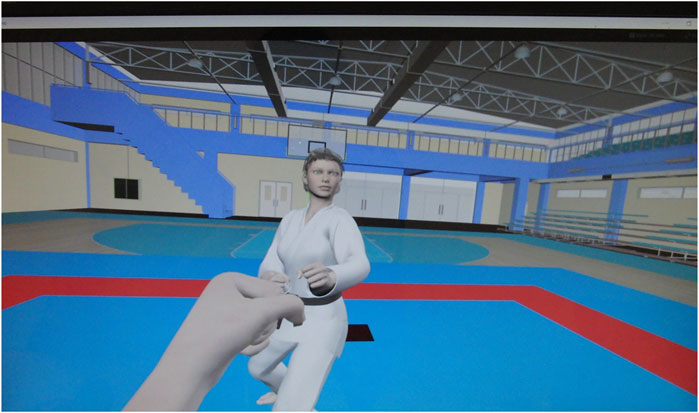
FIGURE 3. Example of a virtual opponent from the athlete’s point of view. In the front, you can see the visualized left fist of the athlete.
2.5 Procedure of measurements
2.5.1 Sport-unspecific reaction tests
Two tests of the Vienna test system (Schuhfried, Vienna, Austria) were used to measure the sport-unspecific reaction time. In the simple reaction test (S1) to a visual stimulus and in the recognition reaction test (S4) to a visual stimulus with visual and auditory distractors, the task for the participants was to move the index finger from one button to another one when a visual stimulus appeared. The Vienna test system records the reaction time (RT) and motor response time (MT).
2.5.2 Karate-specific reaction tests
The karate-specific reaction tests were performed in RW and VR. To guarantee that the attacks of the virtual opponent and the real opponent were as similar as possible for the respective athlete, the attacks were compared in terms of their temporal movement phase structure and only the virtual attacks that corresponded best to the real attacks were used. This means that the absolute times for the complete attack, but also for the preparatory phase (until the distance reduction) and the actual arm extension should be comparable. In addition, care was taken to ensure that real and virtual attacks did not differ in individual technical details. The athletes were asked to respond to 22 attacks from a real opponent (RW test) and a virtual opponent (VR test). In a randomized order, 50% of the athletes started with the reaction test in RW and the other 50% with the test in VR. Attacks were presented in four blocks of five to six randomly selected attacks each, with a 1–2 min break between blocks. The attacks in focus, 8 KZ and 8 GZj, were mixed with other karate attacks so that the athletes could not predict the specific attack technique. GZj and KZ were chosen due to previous analyses (Petri et al., 2018a; Petri et al., 2019a) showing that both attacks are the most often and successfully performed attacks in international karate kumite competitions.
The attack of the opponent and the corresponding response of the athlete were recorded synchronously with two high-speed cameras (Contemplas, Kempten, Germany, 100 Hz). The qualitative movement analysis for the response behavior to the attacks was based on the following parameters: “response time,” and “response quality.” The “response time” is defined by three karate experts as the difference between the time point at which the opponent reduces his distance to the athlete by starting a step-flight phase and the time point at which the athlete starts his response.
In order to assess the “response quality,” it is well-known that in karate kumite competition a time-adequate direct counterattack is the best solution to react to an attack and to score a point. On this basis, “response quality” was defined as the total number of time-adequate direct counterattacks. This means that if there are eight attacks (GZj or KZ) the maximum value of “response quality” is eight.
2.5.3 Subjective self-assessment of performance and simulator sickness questionnaire
For subjective self-assessment of their performance, feedback forms were completed by the athletes in the pretest and posttest. Response behavior and distance estimation in relation to GZj and KZ were evaluated with a grading system from 1 (very good) to 5 (very poor).
Both after the VR tests and each VR training session, the participants were asked to answer the simulator sickness questionnaire (SSQ) (Kennedy, Lane, Berbaum, and Lilienthal, 1993) to measure symptoms of cybersickness.
2.5.4 Subjective assessment of VR training by athletes
Athletes of VRG were asked to rate the following statements on a 10-point scale (1 - strongly disagree, 10 - strongly agree):
Item 1: I enjoy training in virtual reality.
Item 2: Training against a virtual opponent is more exhausting than against a real opponent.
Item 3: I can react to the attacks of the avatar in the same way as to attacks of a real athlete.
Item 4: I feel comfortable in VR
The assessments were evaluated after the first, the fifth and the 10th (last) training session.
2.6 Data analysis
All videos including the opponent’s attacks (eight GZj, eight KZ) and athlete’s response behavior were analyzed using the video analysis software Kinovea (version 0.8.15) by two karate experts. Interrater reliability (Harris et al., 2020) can be rated as good (Cohen’s kappa coefficient κ > 0.80). ‘Response time’ was averaged over the results of both raters. Since both fist strikes are fundamentally different from each other, the reactions to these attacks are examined separately. Further statistics were conducted with SPSS (IMB, Germany, version, 26). The level of significance for all tests was set at α = 0.05. The requirements (normal distribution, no outliers, sphericity, homogeneity of the error variances, homogeneity of covariances) for the mixed ANOVA and for single factorial ANOVA with repeated measures were checked. For significant interactions η2 (η2 = 0.01 small effect, η2 = 0.06 moderate effect, η2 = 0.14 large effect), Cohen’s d (d = 0.2 small effect, d = 0.5 moderate effect, d = 0.8 large effect) or Pearson correlation coefficient r (r = 0.1 small effect, r = 0.3 moderate effect, r = 0.5 large effect) are used as effect size (Koo and Li, 2016).
A mixed ANOVA with the between-subject factor group (CG, VRG) and the within-subject factors test time (pretest, intermediate test, posttest) and condition (RW, VR), for each attack (GZj, KZ) for the parameter ‘response time’ and ‘response quality’ was conducted. Post-hoc tests (t-test or Wilcoxon test) were used. For both sport-unspecific reaction tests (S1, S4) reaction time (RT) and motor response time (MT) were analyzed with a mixed ANOVA. For the symptoms of the cybersickness, the data of the SSQ are calculated as described in (Cohen, 2013). The classification from Stanney, Kennedy, and Drexler 1997) (Kennedy et al., 1993) was used to interpret the means from each group for the total score with: 0 - ‘no symptoms’, 0–5 - “negligible symptoms,” 5–10 “minimal symptoms,” 10–15 “significant symptoms,” 15–20 “symptoms that are a concern,” and >20—“problem simulator.”
3 Results
3.1 Response time
Table 1 shows the means and standard deviations of ‘response time’ in all conditions and the results of statistical analyses.
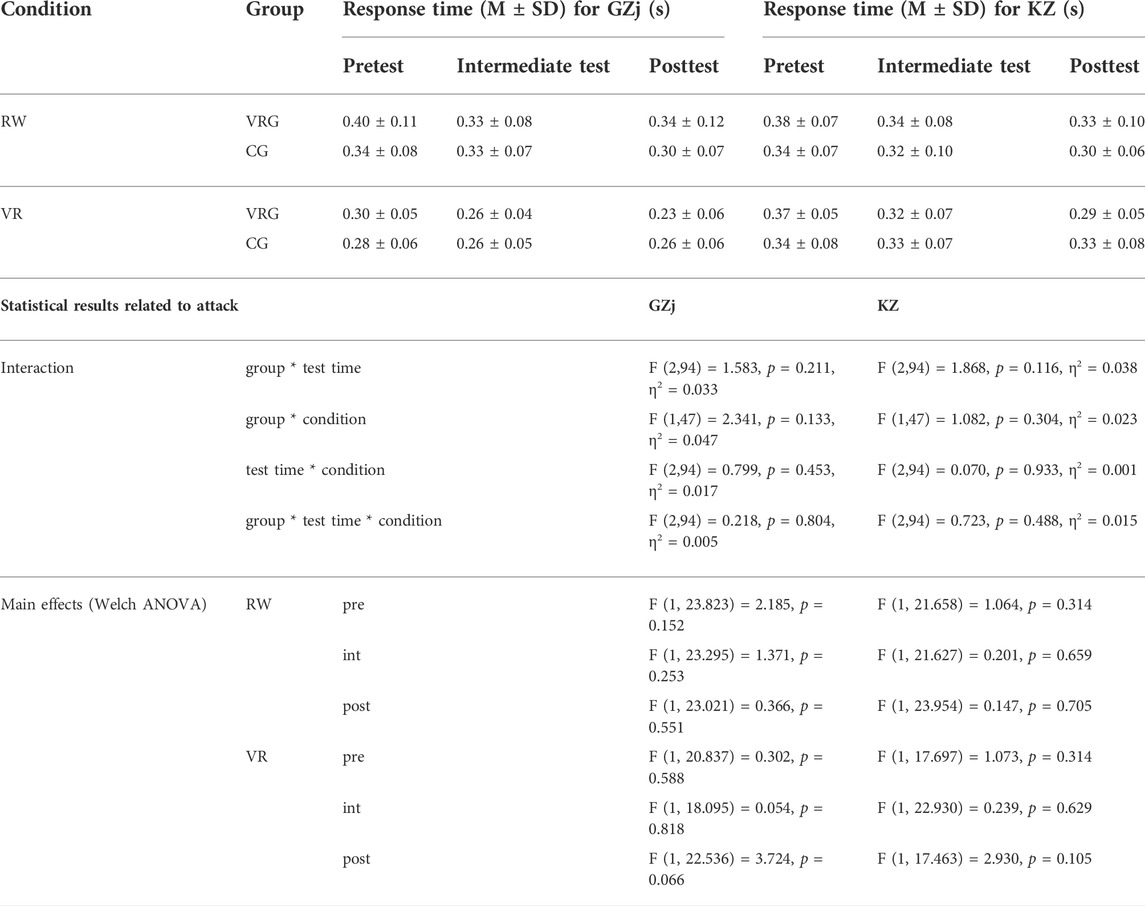
TABLE 1. Results for “response time” (s) for each attack (GZj, KZ), for each test time (pretest, intermediate test, posttest), for each condition (RW, VR), and each group (VRG, CG). For the parameter “response time,” a smaller value represents a shorter time until the athlete started his response towards the attack of the opponent.
First of all, it should be noted that interaction effects could not be found (see Table 1). Only the parameter ‘response time’ improved significantly over the course of the training without taking the group and the condition into account, with large effects with regard to GZj [F (2,94) = 9.323, p < 0.001, η2 = 0.166) and with regard to KZ (F (2, 94) = 7.323, p = 0.001, η2 = 0.135].
As the main effect of test time for both attacks (GZj, KZ) and for both conditions (RW, VR) was significant, we used a single factorial ANOVA with repeated measures (Table 2).
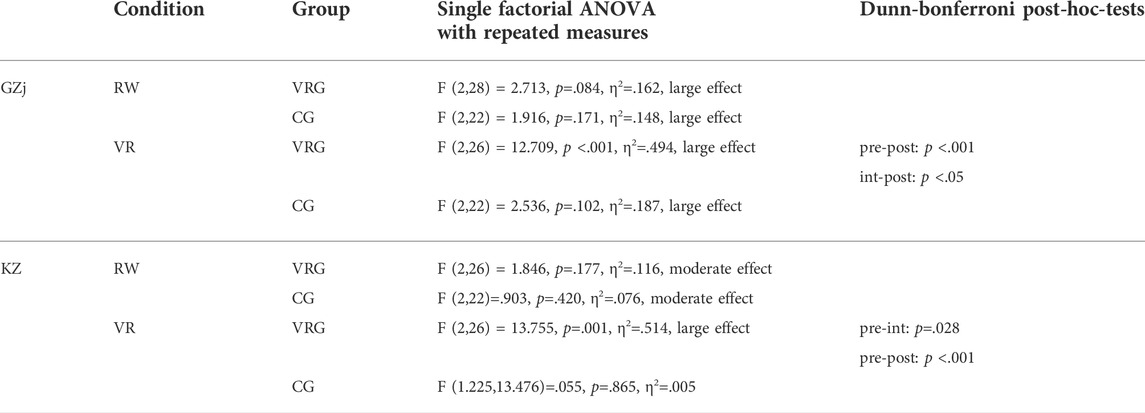
TABLE 2. Results of single factorial ANOVA with repeated measures for “response time” (s) for each attack (GZj, KZ), for each condition (RW, VR), each group (VRG, CG) and Dunn Bonferroni post-hoc-tests.
The results show, that only the VRG was able to improve the “response time” in VR related to GZj and KZ with a large effect. The response time was already reduced in the intermediate test (for KZ) or only in the posttest (for GZj).
3.2 Response quality
Table 3 shows the means and standard deviations of “response quality” in all conditions and the results of statistical analyses.
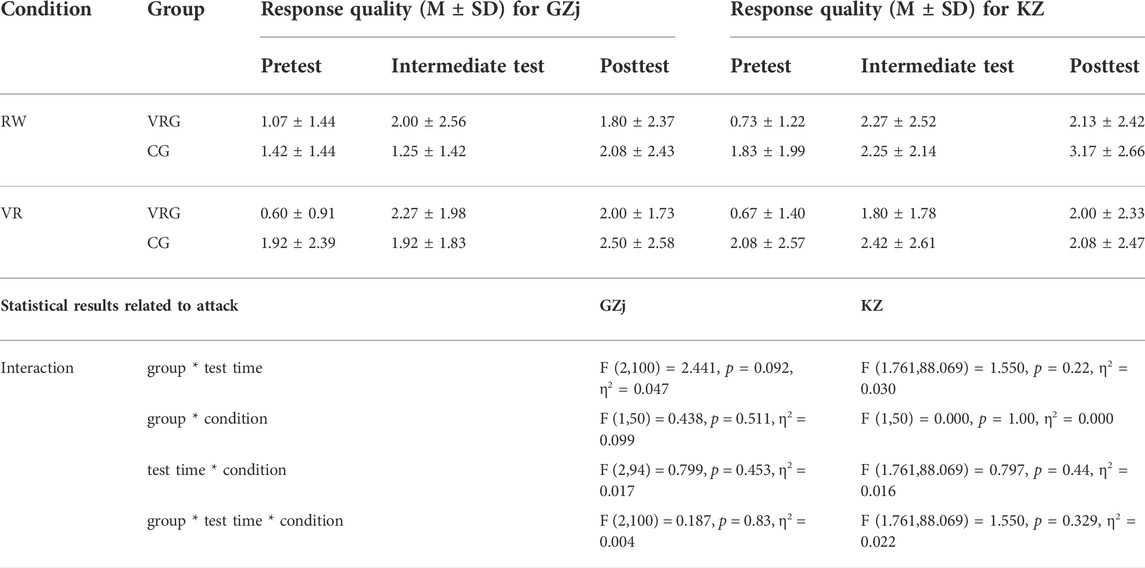
TABLE 3. Results for “response quality” for each attack (GZj, KZ), for each test time (pretest, intermediate test, posttest), for each condition (RW, VR), and for each group (VRG, CG). A higher value represents a better “response quality” (maximum = 8) towards the attack of the opponent.
In general, it shows that on average the athletes only responded to 1-3 attacks with a direct counterattack that would have resulted in a point. Using mixed ANOVA, the assumption that “response quality” improved significantly throughout the training without taking the group and the condition into account could be confirmed, with a moderate effect for GZj [F (2,100) = 3.723, p = 0.028, η2 = 0.069] and with a moderate effect for KZ [F (1.761,88.069) = 7.523, p = 0.002, η2 = 0.132]. For both responses to GZj and KZ, the response quality of VRG and CG did not differ from each other at all three test times.
3.3 Results for athletes with immediate performance level
Furthermore, we were interested in whether VR training would improve performance for athletes with intermediate level in RW. For this purpose, only athletes who scored zero for response quality related to GZj in the pretest (RW) were considered. This resulted in two subgroups: VRG-L (VRG with immediate performance level, n = 8) and CG-L (CG with immediate performance level, n = 5). It was possible to support the assumption that VR training for athletes with immediate level led to an improvement in response time with respect to GZj with a large effect (pre: MW = 0.462 s, SD = 0.096 s, post: MW = 0.365 s, SD = 0.138 s, Z = -1.960, p = 0.050, r = 0.69). No significant differences were found between pre and post with regard to KZ (pre: MW = 0.354 s, SD = 0.105 s, post: MW = 0.306 s, SD = 0.059 s, Z = -1.402, p = 0.161).
3.4 Sports-unspecific reaction time
The mixed ANOVA for the S1 and S4 revealed only for the reaction time (RT) from S4 a statistically significant main effect of test time with a large effect [F (Cohen, 2013; Craig, 2013) = 233.165, p < 0.001, η2 = 0.903]. Post-hoc tests showed that both groups had a significantly (p < 0.001) shorter reaction time (RT) in the posttest (VRG (251.46 ± 50.47) ms, CG: (240.25 ± 41.41) ms) compared to the pretest (VRG (358.73 ± 67.72) ms, CG: (341.81 ± 47.36) ms).
3.5 Qualitative performance assessment
In general, the qualitative performance was rated on the grading scale by athletes between 1.6 and 2.5. There was no significant difference in response behavior and distance estimation between the pretest and posttest. The one-factor analysis of variance with the factor group revealed significant differences only for the athletes’ self-assessment of responsiveness to the KZ with a large effect (F (1,25) = 6.803, p = 0.015, η2 = 0.253). The athletes in the control group (CG) rated their reaction time better in the posttest (1.58 ± 0.51) than in the pretest (2.00 ± 0.85). However, the coach’s assessments did not confirm this.
3.6 Simulator sickness questionnaire
The total score from the SSQ (Kennedy et al., 1993) ranged from ‘minimal symptoms’ to ‘problem simulator’ according to the classification of Stanney et al. 1997) for both groups VRG (pre: 13.96 ± 13.88, int: 6.48 ± 13.88, post: 8.47 ± 20.36) and CG (pre: 21.82 (±31.24, int: 17.76 ± 18.40, post: 8.72 ± 8.78). The mixed ANOVA detected no interaction effects between test time and group (F (2,50) = 0.972, p = 0.386, η2 = 0.036) as well as no main effects [time: F (2,50) = 2.680, p = 0.078, η2 = 0.097; group: F (Cohen, 2013; Craig, 2013) = 1.341, p = 0.258; η2 = 0.051].
3.7 Subjective assessment of VR training by athletes
Table 4 shows the descriptive and statistical results for subjective assessment of VR training by the athletes of VRG.
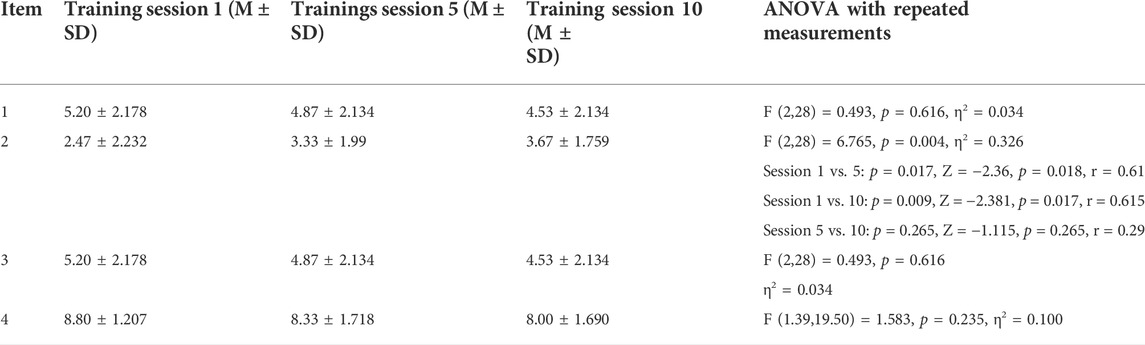
TABLE 4. Results for subjective assessment of VR training’ by VRG (n = 15) after 1st, 5th,, and 10th training session. Item 1: I enjoy training in virtual reality. Item 2: Training against a virtual opponent is more exhausting than against a real opponent. Item 3: I can react to the attacks of the avatar in the same way as to attacks of a real athlete. Item 4: I feel comfortable in VR. (1—strongly disagree, 10—strongly agree).
The VR training was equally enjoyable for the athletes from the beginning to the end of the training period. The differences between the sessions related to Item 2 were significant. The assessment of whether the athletes react to the virtual opponent in the same way as to a real opponent was rated independently of time with a score of 5. The statement “I feel comfortable in VR” was rated equally good across all training sessions.
4 Discussion
The aim of the present study was to show to what extent response training in VR improves response behavior also in RW. This was examined in karate kumite, in which additional VR training was integrated in regular training sessions.
While previous studies often found performance improvements from VR training by comparing it to a control group that received no training [e.g., (Michalski et al., 2019)], our study compared the effect of combined VR-RW training to RW training. In particular, it should be verified whether integrated VR training also leads to an improvement in RW performance. In general, it was observed that the response time in RW decreased for both groups after the intervention. It is obvious that, in contrast to CG, VRG reduced its response time in VR. This is consistent with the studies by Gray (Gray, 2017), La Nour, Hyoz, and Bresciany (Le Naour et al., 2020), and Oagaz et al. (Oagaz et al., 2021), which show significant performance improvements due to VR training. However, it could not be shown that the parameter “response time” was also shortened in RW for the VRG. In contrast, the athletes as well as the coach reported that in their perception the response time improved in RW. Our findings are consistent with the previous study (Petri et al., 2019a). The authors found response time improvements for all attacks studied. However, the study by Petri et al. (Petri et al., 2019a) was a cross-over design with a relatively small number of subjects and, in addition, negative response times (inclusion of pre-start reactions) were measured, which did not occur in the present study. Looking at the results of the intermediate-level athletes, improvements in response time can also be demonstrated for the GZ attack. This could indicate that such VR training is particularly suitable for lower-performing athletes. However, lower-performing athletes do not mean beginners, but athletes with lower performance in karate kumite.
Another reason for the relatively small effect of VR training could be that the time proportion of VR training compared to conventional training was 10 min/80 min. This short time was chosen to avoid possible discomfort in VR and to compare the results with those by Petri et al. (Petri et al., 2019a). With improved VR technology, it should be possible in the future to carry out VR training over a longer period of time. Already Petri, Feuerstein, Folster, Bariszlovich, and Witte (Petri et al., 2020) found that VR training with HMD of 20 min is also possible.
In contrast to the previous study (Petri et al., 2019a), significant improvements in response quality could not be demonstrated. However, this could be changed in the future if VR training focused on trying out different reaction strategies. After all, no painful contact occurred during the attack by the virtual opponent, which could reduce the risk of injuries.
Regarding the sports-unspecific reaction time, a significant improvement from pretest to posttest was found for both groups only for the reaction test S4 of the Vienna test system. The values for reaction time and motor response time are similar to the results from previous studies for combat athletes (Gierczuk et al., 2017). The reaction time is shorter than the ‘response time’ during an attack as the tasks are different. In contrast to (Petri et al., 2019a), we found a significant improvement from pretest to posttest. This can be explained by a higher sample size in the current study. The results may indicate that sport-specific reaction training also has an effect on sport-unspecific reaction behaviour with visual and auditory distractors. The non-existing differences in the other values for the reaction and motor response time are in line with results from Florkiewicz et al. (Florkiewicz et al., 2015).
The values for the total score of SSQ are extremely high and could raise concern. But in line with (Stanney et al., 1997), it should be noted that cybersickness is not a simulator sickness. The high values could be due to the development of the SSQ for military simulations. A meta-research from Saredakis et al. (Saredakis et al., 2020) showed that several studies using HMD reported high SSQ-Scores. As no participant felt uncomfortable in the virtual environment, the high values of the SSQ should not affect the results of the present study.
An important prerequisite for the introduction of VR training is the clarification of the extent to which VR training integrated into conventional training is accepted by the athletes. The present results (fun, effort level, similar reaction compared to a real opponent, and comfortable feeling in VR) advocate additional training with a virtual opponent. Similar results are obtained for free-throw training by Covaci et al. (Covaci et al., 2015). The score of 5 for response behavior related to the virtual opponent compared to the real opponent means that the virtual opponent does not move naturally enough, e.g., does not adapt optimally to the athlete’s movement and position. To solve this problem, an intelligent avatar with low latency should be used in the future, based on the results of Zhang et al. (33).
5 Limitations
There are some limitations of the current study. First, VR training was short compared to conventional training. Second, the athletes did not receive any haptic feedback during the VR training from the opponent. Third, the virtual character was a passive one and was not able to react to the athlete’s movement. The training consisted only of attacks from the avatar and responses of the athlete. Forth, the raters were not blinded and knew which participant belonged to which group.
6 Conclusion
In summary, we can conclude that karate athletes accept an integrated VR training. The results of the shorter ‘response time’ to a virtual opponent for the VRG suggest that a 10 min VR training integrated into the conventional training leads to an improvement in the response time. The assumption that the effects of VR training can be transferred into RW settings cannot be substantiated. There is initial evidence that VR training tools can be used efficiently for athletes at intermediate performance levels. In addition, future VR training tools should integrate an autonomously interacting opponent, which is able to react to the athlete’s responses and attacks. VR training in karate kumite can be seen as a diverse training tool that increases the motivation of athletes who still have potential for development. In order to realize longer training times in VR, a biofeedback system should be used in the future to obtain indicators of cybersickness.
Data availability statement
The original contributions presented in the study are included in the article/supplementary material, further inquiries can be directed to the corresponding author.
Ethics statement
The studies involving human participants were reviewed and approved by the Ethics Committee of Otto-von-Guericke university was obtained under the number 132/16. Written informed consent to participate in this study was provided by the participants’ legal guardian/next of kin.
Author contributions
All authors have contributed to the work in a meaningful way. KW, KP, and PE were involved in the conceptualization, the design of the methodology and the data analysis. KP, SM, and KW developed the virtual opponent. YR and MD rated the videos of the attacks. YR, MD, PM, and KW conducted the research. KW and YR analysed statistically the data. The results were critically discussed with DB. KW, DB, and KP revised the first draft of the by YR. All authors agree with publication of the final version of the manuscript.
Funding
This work was supported by the German Reserach Foundation (DFG) (WI 1456/22-1).
Acknowledgments
We would like to thank the athletes and coaches of two local karate clubs who participated in this research.
Conflict of interest
The authors declare that the research was conducted in the absence of any commercial or financial relationships that could be construed as a potential conflict of interest.
Publisher’s note
All claims expressed in this article are solely those of the authors and do not necessarily represent those of their affiliated organizations, or those of the publisher, the editors and the reviewers. Any product that may be evaluated in this article, or claim that may be made by its manufacturer, is not guaranteed or endorsed by the publisher.
References
Burns, A. M., Kulpa, R., Durny, A., Spanlang, B., Slater, M., and Multon, F. (2011). Using virtual humans and computer animations to learn complex motor skills: A case study in karate. BIO Web Conf. 1, 00012. doi:10.1051/bioconf/20110100012
Cohen, J. (2013). Statistical power analysis for the behavioral sciences. New York: Routledge. doi:10.4324/9780203771587
Covaci, A., Olivier, A. H., and Multon, F. (2015). Visual perspective and feedback guidance for VR free-throw training. IEEE Comput. Graph. Appl. 35 (5), 55–65. doi:10.1109/mcg.2015.95
Craig, C. (2013). Understanding perception and action in sport: How can virtual reality technology help? Sports Technol. 6 (4), 161–169. doi:10.1080/19346182.2013.855224
Florkiewicz, B., Fogtmann, S., Lesiakowski, P., and Zwierko, T. (2015). The effect of visual perception training on sensorimotor function in handball players. J. Kinesiol Exerc Sci. 69 (25), 25–32.
Gierczuk, D., Lyakh, V., Sadowski, J., and Bujak, Z. (2017). Speed of reaction and fighting effectiveness in elite greco-roman wrestlers. Percept. Mot. Ski. 124 (1), 200–213. doi:10.1177/0031512516672126
Gray, R. (2017). Transfer of training from virtual to real baseball batting. Front. Psychol. 8, 2183. doi:10.3389/fpsyg.2017.02183
Harris, D. J., Buckingham, G., Wilson, M. R., Brookes, J., Mushtaq, F., Mon-Williams, M., et al. (2020). The effect of a virtual reality environment on gaze behaviour and motor skill learning. Psychol. Sport Exerc. 50, 101721. doi:10.1016/j.psychsport.2020.101721
Hülsmann, F., Frank, C., Senna, I., Ernst, M. O., Schack, T., and Botsch, M. (2019). Superimposed skilled performance in a virtual mirror improves motor performance and cognitive representation of a full body motor action. Front. Robot. AI 6, 43. doi:10.3389/frobt.2019.00043
Kennedy, R. S., Lane, N. E., Berbaum, K. S., and Lilienthal, M. G. (1993). Simulator sickness questionnaire: An enhanced method for quantifying simulator sickness. Int. J. Aviat. Psychol. 3 (3), 203–220. doi:10.1207/s15327108ijap0303_3
Koo, Terry K., and Li, Mae Y. (2016). A guideline of selecting and reporting intraclass correlation coefficients for reliability research. J. Chiropr. Med. 15 (2), 155–163. doi:10.1016/j.jcm.2016.02.012
Lammfromm, R., and Gopher, D. (2011). Transfer of skill from a virtual reality trainer to real juggling. BIO Web Conf. 1, 00054. doi:10.1051/bioconf/20110100054
Le Naour, T., Hayoz, L., and Bresciani, J. P. (2020). Human-avatar interaction in virtual environment to assess and train sensorimotor: Application to the slap shot in hockey. Int. J. Virtual Real. 20 (2), 36–54. doi:10.20870/IJVR.2020.20.2.4576
Michalski, S. C., Szpak, A., Saredakis, D., Ross, T. J., Billinghurst, M., and Loetscher, T. (2019). Getting your game on: Using virtual reality to improve real table tennis skills. PloS One 14 (9), e0222351. doi:10.1371/journal.pone.0222351
Miles, H. C., Pop, S. R., Watt, S. J., Lawrence, G. P., and John, N. W. (2012). A review of virtual environments for training in ball sports. Comput. Graph. 36 (6), 714–726. doi:10.1016/j.cag.2012.04.007
Neumann, D. L., Moffitt, R. L., Thomas, P. R., Loveday, K., Watling, D. P., Lombard, C. L., et al. (2018). A systematic review of the application of interactive virtual reality to sport. Virtual Real. 22 (3), 183–198. doi:10.1007/s10055-017-0320-5
Oagaz, H., Schoun, B., and Choi, M.-H. (2021). Performance improvement and skill transfer in table tennis through training in virtual reality. IEEE Trans. Vis. Comput. Graph. 1. doi:10.1109/TVCG.2021.3086403
Pan, L. A., Cheng, X. C., and Du, Q. L. (2012). Study of table tennis training system. Adv. Mat. Res. 472-475, 3117–3120. doi:10.4028/www.scientific.net/AMR.472-475.3117
Petri, K., Bandow, N., and Witte, K. (2018). Using several types of virtual characters in sports - a literature survey. Int. J. Comput. Sci. Sport 17 (1), 1–48. doi:10.2478/ijcss-2018-0001
Petri, K., Emmermacher, P., Danneberg, M., Masik, S., Eckardt, F., Weichelt, S., et al. (2019). Training using virtual reality improves response behavior in karate kumite. Sports Eng. 22 (1), 2. doi:10.1007/s12283-019-0299-0
Petri, K., Emmermacher, P., Masik, S., and Witte, K. (2019). Comparison of response quality and attack recognition in karate kumite between reality and virtual reality – A pilot study. Int. J. Phys. Educ. Fit. Sports 8 (4), 55–63. doi:10.26524/ijpefs1946
Petri, K., Feuerstein, K., Folster, S., Bariszlovich, F., and Witte, K. (2020). Effects of age, gender, familiarity with the content, and exposure time on cybersickness in immersive head-mounted display based virtual reality. Am. J. Biomed. Sci. 12 (2), 107–121. doi:10.5099/aj200200107
Petri, K., Ohl, C.-D., Danneberg, M., Emmermacher, P., Masik, S., and Witte, K. (2018). Towards the usage of virtual reality for training in sports. Biomed. J. Sci. Tech. Res. 7 (1), 1–3. doi:10.26717/BJSTR.2018.07.001453
Petri, K., Witte, K., Bandow, N., Emmermacher, P., Masik, S., Danneberg, M., et al. (2018). “Development of an autonomous character in karate kumite,” in Advances in intelligent systems and computing. Proceedings of the 11th international symposium on computer science in sport (IACSS 2017). Editors M. Lames, D. Saupe, and J. Wiemeyer (Cham: Springer International Publishing), 663, 124–135. doi:10.1007/978-3-319-67846-7_13
Rauter, G., Sigrist, R., Koch, C., Crivelli, F., van Raai, M., Riener, R., et al. (2013). Transfer of complex skill learning from virtual to real rowing. PloS One 8 (12), e82145. doi:10.1371/journal.pone.0082145
R. Dörner, S. Göbel, W. Effelsberg, and J. Wiemeyer (Editors) (2016). “Springer eBook collection computer science,” Serious games: Foundations, concepts and practice (Cham: Springer International Publishing). doi:10.1007/978-3-319-40612-1
Saredakis, D., Szpak, A., Birckhead, B., Keage, H. A. D., Rizzo, A., and Loetscher, T. (2020). Factors associated with virtual reality sickness in head-mounted displays: A systematic review and meta-analysis. Front. Hum. Neurosci. 14, 96. doi:10.3389/fnhum.2020.00096
Stanney, K. M., Kennedy, R. S., and Drexler, J. M. (1997). Cybersickness is not simulator sickness. Proc. Hum. Factors Ergonomics Soc. Annu. Meet. 41 (2), 1138–1142. doi:10.1177/107118139704100292
Tirp, J., Steingröver, C., Wattie, N., Baker, J., and Schorer, J. (2015). Virtual realities as optimal learning environments in sport—a transfer study of virtual and real dart throwing. Psychol. Test Assess. Model. 57 (1), 57–69.
Wang, J. (2012). Research on application of virtual reality technology in competitive sports. Procedia Eng. 29, 3659–3662. doi:10.1016/j.proeng.2012.01.548
Yanovich, E., and Ronen, O. (2015). The use of virtual reality in motor learning: A multiple pilot study review. Adv. Phys. Educ. 5, 188–193. doi:10.4236/ape.2015.53023
Keywords: virtual reality training, exercise, response behavior, karate kumite, headmounted display, transfer
Citation: Witte K, Droste M, Ritter Y, Emmermacher P, Masik S, Bürger D and Petri K (2022) Sports training in virtual reality to improve response behavior in karate kumite with transfer to real world. Front. Virtual Real. 3:903021. doi: 10.3389/frvir.2022.903021
Received: 01 April 2022; Accepted: 05 September 2022;
Published: 29 September 2022.
Edited by:
Kyoung-Yun Kim, Wayne State University, United StatesReviewed by:
Christophe Guillet, Université de Bourgogne, FranceAlessandro Evangelista, Politecnico di Bari, Italy
Copyright © 2022 Witte, Droste, Ritter, Emmermacher, Masik, Bürger and Petri. This is an open-access article distributed under the terms of the Creative Commons Attribution License (CC BY). The use, distribution or reproduction in other forums is permitted, provided the original author(s) and the copyright owner(s) are credited and that the original publication in this journal is cited, in accordance with accepted academic practice. No use, distribution or reproduction is permitted which does not comply with these terms.
*Correspondence: Kerstin Witte, S2Vyc3Rpbi53aXR0ZUBvdmd1LmRl