- 1Department of Mechanical Systems Engineering, Nagoya University, Nagoya, Japan
- 2Department of Computer Science, Tokyo Metropolitan University, Hachioji, Japan
It is difficult for humans to perceive weight accurately, because the perception of weight is produced by the process of multisensory integration. This implies that, by manipulating certain factors, the weight of an object could be perceived differently from the actual weight. Here, the effects of vibration on weight perception were investigated using behavioral tests and questionnaires. As a behavioral test, from a number of identically appearing non-vibrating boxes of different weights, participants had to select a box that they perceived to be of the same weight as a vibrating box. They were also asked whether vibration affected the perceived weight of the box. Even though they reported that vibration created an illusion of weight, the results of the behavioral test did not show a statistically significant effect of vibration on perceived weight. Furthermore, we investigated how the frequency of vibration affected the illusion and found that the weight illusion requires smaller acceleration of vibration at lower frequencies, such as 30 Hz. The illusion was more intense at lower frequencies than at higher frequencies, such as 200 and 300 Hz. Thus, this study demonstrated that vibrotactile stimuli presented to the fingertips produce a weight illusion: vibrating objects are perceived to feel heavier. Even though the principles of this illusion are still unknown, the effect is concrete, and our approach allows easy implementation in virtual reality applications.
1 Introduction
Weight is expressed as mass, which is defined as the amount of force acting on an object, divided by gravitational acceleration. However, it is difficult for humans to perceive weight accurately, because the perception of weight is produced by the process of multisensory integration. In other words, by manipulating certain factors that affect the perception of weight, weight could be perceived differently from the actual weight.
The size-weight illusion is a representative example of weight illusion, in which a larger object feels lighter than a smaller one, even though their actual weights are the same (Charpentier, 1891). The process of correcting the discrepancy between the physical weight and the weight predicted by the internal model in the human brain before lifting is considered to be a reason for the weight illusion (Ross, 1969), although this is still debated (Flanagan and Beltzner, 2000). In addition to the size of the object, material (Ellis and Lederman, 1999), color (Warden and Flynn, 1926), and brightness (Walker et al., 2010) create similar illusory effects. Overall, objects that are expected to be heavier are perceived as being lighter. For example, before they are lifted up, darker objects are expected to be heavier than brighter objects. However, once they are lifted up, darker objects feel lighter, contrary to expectations (Walker et al., 2010). A similar illusion has also been reported for other modalities. For example, blue objects, which are perceived as looking colder, are perceived as being warmer when they are actually touched (Ho et al., 2014).
Proprioception is generally considered to be the dominant mediator in weight perception; however, cutaneous cues also significantly contribute to weight perception (Johansson and Westling, 1984; Johansson and Birznieks, 2004; van Beek et al., 2020). When the skin is stretched, the sense of force increases in the direction of this stretch (Matsui et al., 2013). In particular, when an object is lighter, the influence of cutaneous cues is more prominent compared to proprioception (Minamizawa et al., 2010; van Beek et al., 2020). Haptic interfaces that activate cutaneous sensations to present a sense of weight for virtual reality applications have been studied previously (Minamizawa et al., 2008, 2010; Guinan et al., 2012, 2014; Choi et al., 2017; Park et al., 2018).
Vibrotactile or vibratory stimuli have been investigated to present the sense of force and weight by adjusting wave parameters and the presentation timing of stimuli. Asymmetric vibrations create a sense of force in the direction of larger amplitude of the vibrations (Amemiya and Maeda, 2008; Culbertson et al., 2016; Tanabe et al., 2016). The presentation of vibrations in accordance with hand movements makes objects perceived to be heavier (Okamoto et al., 2011; Nagano et al., 2015).
Meanwhile, we found that presentation of sinusoidal vibrotactile stimuli, unrelated to the motion of the hands and objects, makes objects feel heavier during lifting. Broadly, this phenomenon may be called an illusion. There have been no reports about this phenomenon, and there is no obvious knowledge concerning this illusion. This study aimed to investigate the occurrences and properties of this phenomenon. The effect of vibration on weight perception and the frequency-dependence of the weight illusion were investigated. Once these properties are revealed, continuous vibrotactile stimuli, which are possible to implement with inexpensive motors, can be employed in handheld controllers of commercial virtual reality gadgets and smart phones to present illusory weight. This study was approved by the institutional review board of the Hino Campus of Tokyo Metropolitan University (H21-008).
2 Methods
2.1 Experiment 1: Effect of vibrotactile stimuli on behavioral responses and introspective weight perception
2.1.1 Participants
Fifteen male, right-handed and healthy university students over the age of 20 years participated in the experiment. All participants provided written informed consent.
2.1.2 Stimuli and apparatus
A cubic box (104.3 g), made of acrylic, with a side length of 65 mm, as shown in Figure 1A, was used as a weight stimulus. The outside of the box was enclosed evenly with black tape (MT-08-BK-50MM, DIATEX Co. Ltd., Japan) to make its inside invisible and to prevent the fingers from slipping. An eccentric rotating mass vibration motor (TP-2528C-24, Three Peace Co., Ltd., Japan) was fixed inside the box. The motor rotated around an axis perpendicular to the bottom surface of the box, and generated vibrations of 63.7 Hz, with an acceleration amplitude of 9.2 m/s2 in the normal direction of the contact surface of the fingers holding the box. The acceleration amplitude and vibration frequency of the box were determined by the authors based on an informal experiment, and the vibration was sufficient to give the authors the weight illusion.

FIGURE 1. (A) Vibrating box and its schematic diagram. An eccentric mass vibration motor present inside the box makes the box vibrate. (B) Non-vibrating boxes that are identical to the vibrating box in appearance, but with different weights. (C) Lifting the box with five fingers in Experiment 1. (D) Lifting the voice coil oscillator in Experiments 2 and 3.
To investigate the subjectively equivalent weight of the vibrating box, we prepared a total of 17 non-vibrating boxes (Figure 1B) that were identical to the vibrating box in appearance (Figure 1A). However, the weight of the non-vibrating boxes varied exponentially from 74.5 to 138.5 g. The increment ratio in the weight of the non-vibrating boxes was 1.04. The weight of the box was adjusted by fixing several weights inside the box, to the center of the bottom of the box. One of the non-vibrating boxes had a similar weight to the actual weight of the vibrating box, with a difference in weight of less than 1 g.
2.1.3 Task
First, to investigate the subjectively equivalent weight of the vibrating box, we conducted a matching task using the vibrating box as the standard stimulus and the non-vibrating boxes as the comparison stimuli. The participants alternately lifted the vibrating box and the non-vibrating box and compared their perceived weights. They were instructed to lift each box slowly to prevent them from judging the weight of the box based on the inertial forces. The participants used only their dominant hands for lifting, to eliminate the potential differences in muscle strength between dominant and non-dominant hands. There was no limit to the number of times the boxes could be lifted, considering the difficulty in comparing the weights. Then, participants selected one of the non-vibrating boxes that they perceived as being closest in weight to the vibrating box. This task was considered as one trial. Each participant completed nine trials, which differed in terms of the number and placement of the non-vibrating boxes. More specifically, in order to prevent the participants from learning the weight of the non-vibrating boxes in the repeated trials, a different number of non-vibrating boxes (9–17), which included the same weight as the vibrating box, were used for each trial, and they were placed randomly. To mitigate skin numbness owing to vibration, the participants were instructed to hold the box for no more than 3 s. Participants could rest to avoid fatigue after each trial.
Second, to investigate the perception of the weight of the box, participants compared the same box between the vibrating and non-vibrating conditions. They were then asked to report whether the box in the vibrating condition felt 1) heavier, 2) the same, or 3) lighter than in the non-vibrating condition. The vibration did not last longer than 3 s, as explained above.
In both experiments, participants were instructed to hold each box with the pads of all five fingers and to lift the box without placing their elbows or wrists on the desk, as shown in Figure 1C. Moreover, to cancel the sound generated by the vibration of the box, pink noise was played through headphones.
2.1.4 Analysis
The geometric mean of the weights of the non-vibrating boxes that participants selected as having a weight equivalent to that of the vibrating box was calculated for each participant. We then compared the arithmetic mean of these boxes among all participants with the weight of the standard stimulus, using a t-test.
The proportion of the different answers in the introspective experiment was tested by Z-test. We investigated whether the proportion of respondents who answered that vibration made the box feel heavier was significantly different from 1/3.
2.2 Experiment 2: Acceleration and frequency dependency of weight illusion
2.2.1 Participants
Thirteen male, right-handed and healthy university students over the age of 20 years participated in the experiment. All provided written informed consent.
2.2.2 Apparatus and stimuli
To control the frequency and acceleration independently, an oscillator that was different from Experiment 1 was used. A voice coil oscillator (Vp408, Acouve Laboratory, Inc., Japan, 85 g) was used to present the vibrotactile stimuli. The sinusoidal vibratory signals were generated by a function generator (SG-4105, Iwatsu Electric Co., Ltd., Japan) and were amplified using an audio amplifier (FX-AUDIO-FX252A, North Flat Japan Co., Ltd., Japan). Five frequencies (30 Hz, 60 Hz, 100 Hz, 200 Hz, and 300 Hz) were tested. Fourteen levels of acceleration were prepared for each frequency. Only for the 200 Hz, 18 levels were provided to present sufficiently large range such that all the participants could feel the illusory weight. These were in a geometric series, with a ratio of 1.25. The highest level of acceleration for each frequency was determined to be sufficiently greater than the value obtained in an informal experiment involving five participants. Similarly, the lowest level of acceleration was sufficiently smaller than an acceleration which did not cause the weight illusions. The amplitudes of the sinusoidal acceleration of the strongest stimuli for the five frequencies (30 Hz, 60 Hz, 100 Hz, 200 Hz, and 300 Hz) were 10.6 m/s2, 14.1 m/s2, 19.4 m/s2, 151.7 m/s2, and 154.5 m/s2, respectively. The acceleration amplitude of the weakest stimuli for the five frequencies were 0.6 m/s2, 0.8 m/s2, 1.7 m/s2, 4.3 m/s2, and 8.5 m/s2, respectively. Acceleration of the vibratory stimuli was measured using an accelerometer (Model-2320B, Showasokki Co., Ltd., Japan) attached to the side of the oscillator and amplifier (Model-4035-50, Showasokki Co., Ltd., Japan).
2.2.3 Task
The minimum acceleration amplitudes, i.e., the thresholds that caused a weight illusion were investigated for each of the five frequencies. The experiment was conducted following method of limits which is a psychophysical test about sensory threshold. Participants held the oscillator in their dominant hands as shown in Figure 1D. The oscillator was held in a way that the vibration was presented to the finger in a direction horizontal to the ground. The oscillator was repeatedly switched on and off to present and withdraw the vibrotactile stimuli with 3-s intervals to mitigate skin numbness. The repetition was continuously conducted multiple times; hence order effects of vibrating and still conditions could be eased or cancelled. Participants were asked to judge whether the vibratory stimulus made the oscillator feel heavier, lighter, or not. In ascending trials, the initial amplitude of the acceleration was that for which the weight illusion was not expected. Then, the amplitude of the acceleration was increased gradually, and the trial was completed once the participant experienced the increase in the weight. Descending trials started with an acceleration at which the weight illusion was expected to occur. The amplitude of the acceleration was then decreased gradually, and the trial was completed when the participant no longer felt the increase in the weight. For each frequency, three ascending and three descending trials were alternately conducted. A total of 30 trials (three ascending and three descending trials for each of the five frequencies) were conducted for each participant.
As in Experiment 1, the participants lifted the oscillator using the five fingers, while avoiding touching the desktop with the elbows, and the sound cues were masked.
2.2.4 Analysis
In the ascending trials, we calculated the threshold as the average of the acceleration amplitude of the first stimulus that caused the weight illusion and that of the stimulus presented immediately prior. In the descending trials, the threshold was determined as the average of the acceleration amplitude of the first stimulus that no longer caused the weight illusion and that of the stimulus presented immediately prior. The thresholds for six trials were geometrically averaged for each frequency and each participant.
Additionally, the displacement thresholds for each participant were calculated by using the results of acceleration thresholds and their frequencies.
2.3 Experiment 3: Comparison of the subjective intensity of the weight illusion among different frequencies
2.3.1 Participants
Fifteen healthy university students (14 males and one female) over the age of 20 years, participated in the experiment after providing written consent. They were all right-handed.
2.3.2 Apparatus and stimuli
The voice coil oscillator (Vp408, Acouve Laboratory, Inc., 85 g), that was used in Experiment 2, was used to present the vibrotactile stimuli. Five sinusoidal vibration stimuli with different frequencies were presented, that is, 30 Hz, 60 Hz, 100 Hz, 200 Hz, and 300 Hz. The amplitudes of accelerations for the vibration stimuli were 4.4 m/s2 at 30 Hz, 9.5 m/s2 at 60 Hz, 13.8 m/s2 at 100 Hz, 59.8 m/s2 at 200 Hz, and 109.7 m/s2 at 300 Hz. The accelerations were 2 dB higher than the average acceleration thresholds calculated from five participants in the early stage of Experiment 2. This referred values were slightly different to the overall results of Experiment 2, which analyzed with 13 participants, with an average difference of 3.5%.
2.3.3 Task
Five types of vibration stimuli were presented to the participants, who were blinded to the frequencies, and the participants were asked to rank them in terms of the intensity of the weight illusion (the perceived weight). Different stimuli could not be assigned the same rank. Participants held the oscillator with their dominant hand, as in Experiment 2, and were presented stimuli one by one. Participants could experience the vibrotactile stimulus as many times as they required, until they could confidently compare the intensity of the illusion of the different stimuli. Each participant repeated this ranking task twice, with a few minutes apart. As in the previous experiments, the participants held the device with their five fingers, while refraining from placing their elbows on the desktop. The vibration did not last longer than 3 s, and the vibratory sounds were masked.
2.3.4 Analysis
We planned to screen out the participants from the analysis whose results differed significantly between the two trials in this experiment. However, there was no such participant. We used the mean ranks between the two trials as each participant’s results. The rank results of five different vibrotactile stimuli were compared using the Wilcoxon signed-rank test, with the Bonferroni correction of p-values.
3 Results
3.1 Experiment 1: Subjectively equal weights and introspective reports
The results of the introspective reports and subjectively equivalent weights are summarized in Figure 2. Thirteen of 15 participants answered that the vibration made the box seem heavier (significantly greater than the chance level; z(14) = 16.97, p = 1.36 × 10–64, Z-test). On the other hand, many participants selected boxes that were lighter than the vibrating box as having subjectively equivalent weights. The mean and standard error of subjectively equivalent weights among the participants were 100.1 ± 2.0 g. This was 4.2 g (3.8%) less than the actual weight of the vibrating box (104.3 g), but the difference was not statistically significant (t (14) = − 2.08, p = 0.056, two-tailed t-test) and the difference was smaller than the discrimination threshold of weight. The discrimination threshold of weight perception depends on the method of lifting and the weights of the objects (Raj et al., 1985). According to studies in which the entire arm was used to lift objects weighing 50–200 g, the Weber ratio is between 6 and 13% (Brodie and Ross, 1984; Ross and Brodie, 1987; Hiramatsu et al., 2015). Therefore, the difference in this experiment (3.8%) was smaller than the Weber ratio and is not substantially meaningful.
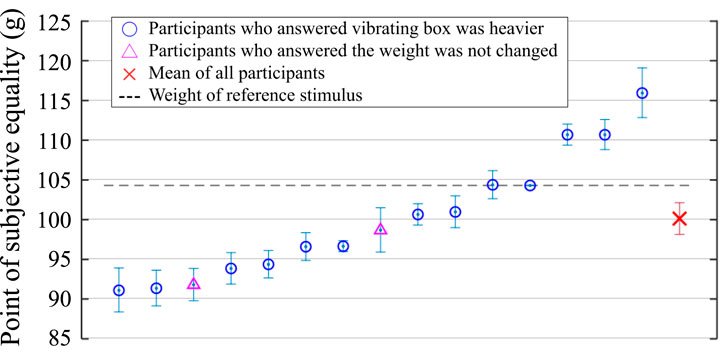
FIGURE 2. Point of subjective equality of weight and introspective report of participants. Error bars indicate standard errors.
3.2 Experiment 2: Minimum vibratory intensities to cause the illusion
Figure 3A shows the acceleration thresholds that cause the weight illusion at each frequency. The 30 Hz frequency, the lowest frequency in this experiment, required the lowest acceleration to create the weight illusion. As frequencies increased, larger accelerations were needed to create the illusion. The threshold did not mostly exceed 10 m/s2 below 100 Hz, but increased to approximately 35 m/s2 above 200 Hz. Figure 3B shows the amplitudes of the vibratory displacements computed from the acceleration values. Contrary to the acceleration thresholds, the displacement thresholds decreased as the frequency increased. This displacement threshold was more than 100 times larger than the threshold for perceiving vibration (Bolanowski et al., 1988; Brisben et al., 1999).
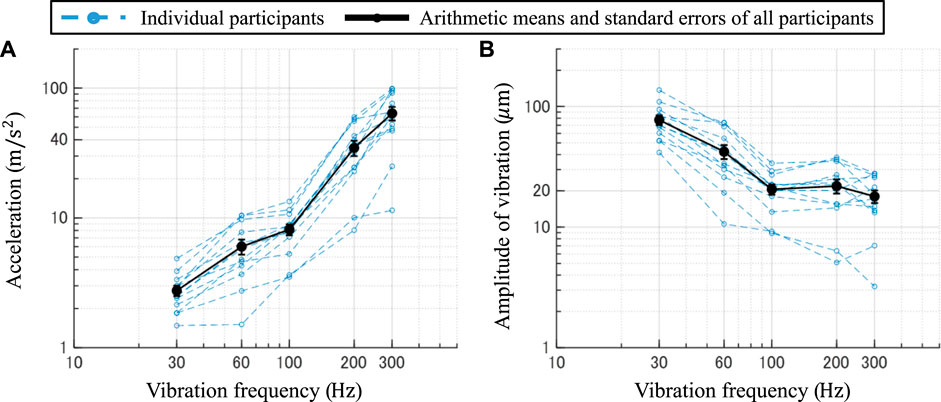
FIGURE 3. The threshold of acceleration (A) and displacement (B) to create a weight illusion for each frequency. The illusion occurred with vibratory accelerations or displacements above the line, but not those below the line.
3.3 Experiment 3: Intensities of illusions caused by different frequencies
Figure 4 shows box plots of the ranks of the intensity of the weight illusion for the five vibration frequencies. The larger ranks indicate greater perceived weight. Based on participants’ responses, the 30 Hz stimulus was associated with the greatest perceived weight, which was significantly different from the other stimuli, at a significance level of less than 1%. However, except for the 30-Hz stimulus, only the ranks for 60 and 300 Hz were significantly different at the 1% significance level (T = 184, p = 0.004).
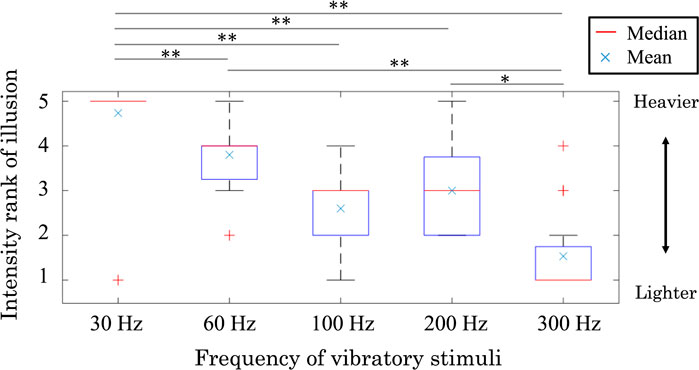
FIGURE 4. Box plots of intensity ranks of illusion by frequencies. * and ** indicate significant differences at 5 and 1% levels, respectively. Significant differences were investigated using the Wilcoxon signed-rank test with Bonferroni correction.
4 Discussion
4.1 General discussion
In Experiment 1, 13 of 15 participants reported experiencing weight illusions, even though the subjectively perceived equal weight was not substantially different between the vibrating and non-vibrating boxes. Earlier studies have reported that the behaviors of participants are adapted through repeated trials (Flanagan and Beltzner, 2000; Grandy and Westwood, 2006; Shadmehr et al., 2010). In the size-weight illusion, after a few trials, the effects of different volumes on the lifting motions disappear; however, the illusion remains (Flanagan and Beltzner, 2000; Grandy and Westwood, 2006). We consider that a similar phenomenon to the size-weight illusion occurred in Experiment 1. This is potentially because the illusion of weight is caused not only by proprioception but also by perceptual mechanisms, which include other factors (Flanagan and Beltzner, 2000).
In Experiment 1, two of fifteen participants did not experience weight illusion. This may be because the intensity of the vibration stimuli used in Experiment 1 was not sufficiently large to create an illusion for them. The frequency and acceleration amplitude of the vibration stimuli used in Experiment 1 were 63.7 Hz and 9.2 m/s2. In Experiment 2, as shown in Figure 3A, some people did not experience illusions at this frequency and acceleration amplitude; however, when the amplitude of the acceleration was increased in Experiment 2, they did experience the weight illusion.
The weight illusion by symmetric vibrations in this study and the method to generate the force illusion by asymmetric vibrations (Amemiya and Maeda, 2008; Culbertson et al., 2016; Tanabe et al., 2016) are similar since they both use vibratory stimuli. However, we speculate that they are based on different principles. In the asymmetric vibration presentations, the illusion of force is generated in the direction of the greater vibration acceleration (Amemiya and Maeda, 2008). If this acceleration is in the direction of gravity, the object feels heavier. The effect of illusion is strong when the asymmetry of the stimulus is salient (Amemiya and Maeda, 2008; Tanabe et al., 2016). On the other hand, the vibration stimulus presented in this study was vertical to gravity, and the vibration was symmetrical. Hence, the principles underlying the illusory weight can be different between the method using the symmetric vibration and that using the asymmetric vibration.
Based on the results of Experiment 3, the frequency that generated the strongest weight illusion was 30 Hz. In this frequency range, fast-adapting type I (FAI) mechanoreceptor units are readily activated (Bolanowski et al., 1988). Considering the limitations of our apparatus, it was not possible to test frequencies below 30 Hz. In addition, since the vibration could stimulate other types of afferent units and could be transferred to the hand and arm, it is not possible to conclude that the FAI units in the fingertips mediated the weight illusion. Hence, the present study does not exclusively support the role of the FAI units for the weight illusion.
One of the possible causes of the weight illusion is the tonic vibration reflex. When muscle spindles are activated by mechanical stimuli applied from above the skin, unrelated to actual muscle activity, proprioceptive sensations are induced (Goodwin et al., 1972; Burke et al., 1976). This phenomenon is known as the tonic vibration reflex. In the present study, the vibrotactile stimuli might have activated the flexor muscles of the fingers and thereby created a weight illusion. The frequency range that is sensitive to the tonic oscillatory reflex differs, depending on the muscle. The leg muscles are most activated at 30–50 Hz (Cardinale and Lim, 2003; Zaidell et al., 2013), which is consistent with the experimental results of this study. On the other hand, the forearm muscles are most strongly activated at 100–150 Hz (Martin and Park, 1997). We could not find earlier studies that reported investigating suitable frequencies for the tonic vibration reflexes of the fingers. Moreover, depending on the activated muscle, objects may not only feel heavy, but may also feel light (Martin and Park, 1997). Therefore, further experiments are needed to determine whether the tonic vibration reflex was the cause of weight illusion in the present experiments.
In the present study, extra grip force (motor command) might have been generated to prevent the vibrating objects from slipping, which might have influenced the perceived weight. Some participants commented on the automatic or involuntary increase in grip force because of the vibration. If the grip force increases, both the afferent signals regarding muscle activity and central motor commands to the related muscles, that is, a sense of effort (McCloskey et al., 1974; Gandevia et al., 1981; Ross and Bischof, 1981; Enoka and Stuart, 1992) could make the vibrating objects feel heavier. However, we did not measure grip force during the experiments, and there is no evidence to support this possibility.
The weight illusion occurred over a wide range of frequencies (30–300 Hz) in Experiments 2 and 3. However, the acceleration thresholds and magnitude of the weight illusion depended on the vibratory frequency. Thus, it is necessary to select suitable frequencies when implementing with commercial vibratory actuators. The frequency at which the weight illusion was generated with the lowest power in Experiment 2 was 60 Hz. Hence, a frequency close to 60 Hz should be used when the power source of a voice coil oscillator is of concern. If the magnitude of the weight illusion is prioritized, use of 30 Hz is recommended, because this frequency causes the most intense perception of weight increase. It should be noted that small size vibrotactile oscillators are typically designed to exhibit resonant frequencies at approximately 100 Hz or higher (Jones and Singhal, 2018). Therefore, effective illusions may not be achieved when using the resonances of small vibrotactile actuators.
4.2 Limitations
The present study investigated the conditions under which the vibration weight-illusion occurred, and the causes of this perceptual effect were discussed, but not concluded. In Experiment 3, the acceleration amplitudes of stimuli were 2 dB greater than the thresholds. We speculate that the more intense vibratory stimuli are, the clearer changes in the weight perception are evoked. Optimal acceleration values for the perceptual effect remain studied. Further, most of the participants were male, and this gender imbalance may limit the generalizability of our results.
4.3 Summary
In this paper, three different experiments were conducted to investigate the illusory phenomenon that sinusoidal vibrations make objects feel heavy. In Experiment 1, 13 of 15 participants reported that sinusoidal vibrations made the box feel heavier, whereas their behaviors were rarely influenced by the vibration. In Experiment 2, all participants felt the weight illusion caused by vibrations of 30–300 Hz. The vibratory amplitudes to evoke the weight illusion differed among the frequencies. In Experiment 3, lower frequency vibrations led to more intense effects. The stimulus with 30 Hz vibration felt heaviest among the five different vibrations.
Previously introduced tactile interfaces for weight and force presentation used skin stretching (Minamizawa et al., 2008, 2010; Guinan et al., 2012, 2014; Choi et al., 2017; Kamikawa and Okamura, 2018), dynamically controlled vibrotactile stimuli (Okamoto et al., 2011; Nagano et al., 2015), and asymmetric vibration (Amemiya and Maeda, 2008; Tanabe et al., 2016). They require a specialized hardware or an oscillator with high responsiveness. On the other hand, for the method used in the present study, continuous vibrotactile stimuli can be implemented even with an eccentric motor oscillator, which has relatively low responsiveness. Further, our approach requires acceleration amplitude of 70 m/s2 at the most to induce the illusion, whereas asymmetric vibration requires peak accelerations of greater than 80 m/s2 (Amemiya and Maeda, 2008; Tanabe et al., 2016). Thus, our approach of using continuous vibrotactile stimuli have implementation advantages when compared to the methods used in earlier studies. However, the effect of this weight illusion differs by individuals, so it is not suitable for quantitative weight expression.
Data availability statement
The original contributions presented in the study are included in the article/supplementary material, further inquiries can be directed to the corresponding author.
Ethics statement
The studies involving human participants were reviewed and approved by Hino Campus of Tokyo Metropolitan University. The patients/participants provided their written informed consent to participate in this study.
Author contributions
GK contributed to the design and implementation of the study and writing. SO contributed to the design of the study and revision. YA and YY contributed to the revision. The ideas of the paper were formulated through a series of meetings to which all authors contributed.
Funding
This study was in part supported by MEXT Kakenhi #21H05819.
Conflict of interest
The authors declare that the research was conducted in the absence of any commercial or financial relationships that could be construed as a potential conflict of interest.
Publisher’s note
All claims expressed in this article are solely those of the authors and do not necessarily represent those of their affiliated organizations, or those of the publisher, the editors and the reviewers. Any product that may be evaluated in this article, or claim that may be made by its manufacturer, is not guaranteed or endorsed by the publisher.
References
Amemiya, T., and Maeda, T. (2008). Asymmetric oscillation distorts the perceived heaviness of handheld objects. IEEE Trans. Haptics 1, 9–18. doi:10.1109/toh.2008.5
Bolanowski, S. J., Gescheider, G. A., Verrillo, R. T., and Checkosky, C. M. (1988). Four channels mediate the mechanical aspects of touch. J. Acoust. Soc. Am. 84, 1680–1694. doi:10.1121/1.397184
Brisben, A., Hsiao, S., and Johnson, K. (1999). Detection of vibration transmitted through an object grasped in the hand. J. Neurophysiol. 81, 1548–1558. doi:10.1152/jn.1999.81.4.1548
Brodie, E. E., and Ross, H. E. (1984). Sensorimotor mechanisms in weight discrimination. Percept. Psychophys. 36, 477–481. doi:10.3758/bf03207502
Burke, D., Hagbarth, K.-E., Löfstedt, L., and Wallin, B. G. (1976). The responses of human muscle spindle endings to vibration of non-contracting muscles. J. Physiol. 261, 673–693. doi:10.1113/jphysiol.1976.sp011580
Cardinale, M., and Lim, J. (2003). Electromyography activity of vastus lateralis muscle during whole-body vibrations of different frequencies. J. Strength Cond. Res. 17, 621–624. doi:10.1519/00124278-200308000-00032
Charpentier, A. (1891). Analyse experimentale de quelques elements de la sensation de poids. Arch. de Physiol. Norm. Pathol. 3, 122–135.
Choi, I., Culbertson, H., Miller, M. R., Olwal, A., and Follmer, S. (2017). “Grabity: A wearable haptic interface for simulating weight and grasping in virtual reality,” in Proceedings of Annual ACM Symposium on User Interface Software and Technology, Quebec City, Canada, October 22–25, 2017, 119–130.
Culbertson, H., Walker, J. M., and Okamura, A. M. (2016). “Modeling and design of asymmetric vibrations to induce ungrounded pulling sensation through asymmetric skin displacement,” in Proceedings of IEEE Haptics Symposium,Philadelphia, Pennsylvania, April 8–11, 2016, 27–33.
Ellis, R. R., and Lederman, S. J. (1999). The material-weight illusion revisited. Percept. Psychophys. 61, 1564–1576. doi:10.3758/bf03213118
Enoka, R. M., and Stuart, D. G. (1992). Neurobiology of muscle fatigue. J. Appl. Physiol. 72, 1631–1648. doi:10.1152/jappl.1992.72.5.1631
Flanagan, J. R., and Beltzner, M. A. (2000). Independence of perceptual and sensorimotor predictions in the size–weight illusion. Nat. Neurosci. 3, 737–741. doi:10.1038/76701
Gandevia, S., Killian, K., and Campbell, E. (1981). The effect of respiratory muscle fatigue on respiratory sensations. Clin. Sci. 60, 463–466. doi:10.1042/cs0600463
Goodwin, G. M., McCloskey, D. I., and Matthews, P. B. (1972). Proprioceptive illusions induced by muscle vibration: Contribution by muscle spindles to perception? Science 175, 1382–1384. doi:10.1126/science.175.4028.1382
Grandy, M. S., and Westwood, D. A. (2006). Opposite perceptual and sensorimotor responses to a size-weight illusion. J. Neurophysiol. 95, 3887–3892. doi:10.1152/jn.00851.2005
Guinan, A. L., Montandon, M. N., Caswell, N. A., and Provancher, W. R. (2012). “Skin stretch feedback for gaming environments,” in Proceedings of IEEE International Workshop on Haptic Audio Visual Environments and Games, Munich, Germany, October 8–9, 2012, 101–106.
Guinan, A. L., Montandon, M. N., Doxon, A. J., and Provancher, W. R. (2014). “Discrimination thresholds for communicating rotational inertia and torque using differential skin stretch feedback in virtual environments,” in Proceedings of IEEE Haptics Symposium, Houston, TX, Feburary 23–26, 2014, 277–282.
Hiramatsu, Y., Kimura, D., Kadota, K., Ito, T., and Kinoshita, H. (2015). Control of precision grip force in lifting and holding of low-mass objects. Plos One 10, e0138506. doi:10.1371/journal.pone.0138506
Ho, H.-N., Iwai, D., Yoshikawa, Y., Watanabe, J., and Nishida, S. (2014). Combining colour and temperature: A blue object is more likely to be judged as warm than a red object. Sci. Rep. 4, 5527. doi:10.1038/srep05527
Johansson, R. S., and Birznieks, I. (2004). First spikes in ensembles of human tactile afferents code complex spatial fingertip events. Nat. Neurosci. 7, 170–177. doi:10.1038/nn1177
Johansson, R. S., and Westling, G. (1984). Roles of glabrous skin receptors and sensorimotor memory in automatic control of precision grip when lifting rougher or more slippery objects. Exp. Brain Res. 56, 550–564. doi:10.1007/bf00237997
Jones, L. A., and Singhal, A. (2018). “Perceptual dimensions of vibrotactile actuators,” in Proceedings of IEEE Haptics Symposium, Francisco, CA, March 25–28, 2018, 307–312.
Kamikawa, Y., and Okamura, A. M. (2018). Comparison between force-controlled skin deformation feedback and hand-grounded kinesthetic force feedback for sensory substitution. IEEE Robot. Autom. Lett. 3, 2174–2181. doi:10.1109/lra.2018.2810940
Martin, B. J., and Park, H.-S. (1997). Analysis of the tonic vibration reflex: Influence of vibration variables on motor unit synchronization and fatigue. Eur. J. Appl. Physiol. 75, 504–511. doi:10.1007/s004210050196
Matsui, K., Okamoto, S., and Yamada, Y. (2013). Relative contribution ratios of skin and proprioceptive sensations in perception of force applied to fingertip. IEEE Trans. Haptics 7, 78–85. doi:10.1109/toh.2013.71
McCloskey, D., Ebeling, P., and Goodwin, G. (1974). Estimation of weights and tensions and apparent involvement of a “sense of effort”. Exp. Neurol. 42, 220–232. doi:10.1016/0014-4886(74)90019-3
Minamizawa, K., Fukamachi, S., Kawakami, N., and Tachi, S. (2008). “Interactive representation of virtual object in hand-held box by finger-worn haptic display,” in Proceedings of IEEE Symposium on Haptic Interfaces for Virtual Environment and Teleoperator Systems, Reno, Nevada, March 13-14, 2008, 367–368.
Minamizawa, K., Prattichizzo, D., and Tachi, S. (2010). “Simplified design of haptic display by extending one-point kinesthetic feedback to multipoint tactile feedback,” in Proceedings of IEEE Haptics Symposium, Waltham, Massachusetts, March 25–26, 2010, 257–260.
Nagano, H., Okamoto, S., and Yamada, Y. (2015). Vibrotactile cueing for biasing perceived inertia of gripped object. Haptic Interact. 277, 17–20. doi:10.1007/978-4-431-55690-9_4
Okamoto, S., Konyo, M., and Tadokoro, S. (2011). Vibrotactile stimuli applied to finger pads as biases for perceived inertial and viscous loads. IEEE Trans. Haptics 4, 307–315. doi:10.1109/TOH.2011.16
Park, J., Oh, Y., and Tan, H. Z. (2018). Effect of cutaneous feedback on the perceived hardness of a virtual object. IEEE Trans. Haptics 11, 518–530. doi:10.1109/toh.2018.2854721
Raj, D. V., Ingty, K., and Devanandan, M. (1985). Weight appreciation in the hand in normal subjects and in patients with leprous neuropathy. Brain 108, 95–102. doi:10.1093/brain/108.1.95
Ross, H. E., and Bischof, K. (1981). Wundt’s views on sensations of innervation: a reevaluation. Perception 10, 319–329. doi:10.1068/p100319
Ross, H. E., and Brodie, E. E. (1987). Weber fractions for weight and mass as a function of stimulus intensity. Q. J. Exp. Psychol. Sect. A 39, 77–88. doi:10.1080/02724988743000042
Ross, H. E. (1969). When is a weight not illusory? Q. J. Exp. Psychol. 21, 346–355. doi:10.1080/14640746908400230
Shadmehr, R., Smith, M. A., and Krakauer, J. W. (2010). Error correction, sensory prediction, and adaptation in motor control. Annu. Rev. Neurosci. 33, 89–108. doi:10.1146/annurev-neuro-060909-153135
Tanabe, T., Yano, H., and Iwata, H. (2016). “Properties of proprioceptive sensation with a vibration speaker-type non-grounded haptic interface,” in Proceedings of IEEE Haptics Symposium, Philadelphia, Pennsylvania, April 8–11, 2016, 21–26.
van Beek, F. E., King, R. J., Brown, C., Luca, M. D., and Keller, S. (2020). Static weight perception through skin stretch and kinesthetic information: Detection thresholds, jnds, and pses. IEEE Trans. Haptics 14, 20–31. doi:10.1109/toh.2020.3009599
Walker, P., Francis, B. J., and Walker, L. (2010). The brightness-weight illusion. Exp. Psychol. 57, 462–469. doi:10.1027/1618-3169/a000057
Warden, C. J., and Flynn, E. L. (1926). The effect of color on apparent size and weight. Am. J. Psychol. 37, 398. doi:10.2307/1413626
Keywords: fingertips, haptics, threshold, vibration, weight illusion
Citation: Kim G, Okamoto S, Akiyama Y and Yamada Y (2022) Weight illusion by presenting vibration to the fingertip. Front. Virtual Real. 3:797993. doi: 10.3389/frvir.2022.797993
Received: 19 October 2021; Accepted: 27 June 2022;
Published: 15 July 2022.
Edited by:
Jeremy Cooperstock, McGill University, CanadaReviewed by:
Pascal E. Fortin, École de Technologie Supérieure (ÉTS), CanadaVincent Levesque, École de Technologie Supérieure (ÉTS), Canada
Copyright © 2022 Kim, Okamoto, Akiyama and Yamada. This is an open-access article distributed under the terms of the Creative Commons Attribution License (CC BY). The use, distribution or reproduction in other forums is permitted, provided the original author(s) and the copyright owner(s) are credited and that the original publication in this journal is cited, in accordance with accepted academic practice. No use, distribution or reproduction is permitted which does not comply with these terms.
*Correspondence: Giryeon Kim, kim.giryeon.y2@s.mail.nagoya-u.ac.jp