- 1Expertise Unit Psychology, Technology & Society, Thomas More University of Applied Sciences, Antwerp, Belgium
- 2Mobilab & Care, Thomas More University of Applied Sciences, Geel, Belgium
- 3Spatial Effects, Antwerp, Belgium
- 4PHOBOS AR, Buenos Aires, Argentina
Exposure therapy is an effective treatment for specific phobia that could be further enhanced through Augmented Reality, a novel technology that can facilitate implementation of gradual exposure and promote treatment acceptability. Effective exposure interventions require stimuli evoking high levels of anxiety. Therefore, it is important to ascertain whether animals can induce anxiety in distinct Augmented Reality modalities, such as Head-Mounted Displays and smartphones, which can differ in user experience and technological embodiment. This study compared the anxiety inducing potential and experienced realism of a spider within the HoloLens 2 Augmented Reality headset and an Augmented Reality smartphone application. Sixty-five participants were exposed to a virtual spider in a 5-step Behavioral Approach Task through both the HoloLens 2 head-mounted display and the PHOBOS Augmented Reality smartphone application. Participants reported Subjective Units of Distress at each step and physiological arousal was measured using heart rate and Skin Conductance. Results show that both technological modalities induced self-reported anxiety for spiders in a Behavioral Approach Task task in a non-clinical sample. The Hololens 2 modality was also related to an skin conductance (SC) increase. Perceived realism did not differ between modalities but was associated with increased anxiety in the HoloLens 2 modality. Findings demonstrate that both implemented modalities have potential for enabling Augmented Reality Exposure Therapy, although the role of experienced realism merits additional investigation. Future research should assess the effectiveness of Augmented Reality Exposure Therapy in clinical samples and assess whether new extended reality modalities, such as passthrough virtual reality, could accommodate observed limitations and improve Augmented Reality Exposure Therapy experiences and outcomes.
1 Introduction
Specific phobia is a common anxiety disorder characterized by an excessive fear response to specific objects or situations, fears that are greater than justified, and accompanying avoidance that causes daily impairment (Oosterlink et al., 2009). A specific phobia for spiders (arachnophobia) has a prevalence rate of 2.7%, but research additionally shows that fear of spiders is experienced by 23.3% of the general sample (approximately 35.8% in women and 10.9% in men) and fears are particularly common in early adulthood (Oosterlink et al., 2009). Although many individuals do not seek treatment, success rates of exposure therapy in this population are high (Choy et al., 2007; Gadermann et al., 2012). Exposure therapy is an intervention from the field of cognitive behavioral therapy (CBT) which involves confronting individuals with the subject of their fears in a controlled and hierarchical manner. Single-session exposure treatment has demonstrated longstanding positive effects, with a significantly reduced burden of anxiety in 90% of treatment participants at 4-year follow-up (Öst, 1989).
Digital mental health (DMH) services can nevertheless provide a useful addition to this treatment. Extended Reality (XR), which includes Virtual Reality (VR) and Augmented Reality (AR), has gathered a considerable evidence base in anxiety treatment (De Witte et al., 2021). The main difference between VR and AR lies in the degree of contact with the real world. While VR aims to immerse you in a computer-generated environment, AR allows you to maintain your sense of presence in the actual world but adds virtual elements (e.g., computer models of animals) to it. Research has supported the therapeutic effectiveness of exposure therapy in VR, and the first studies exploring Augmented Reality Exposure Therapy (ARET) have also been promising (Botella et al., 2017; Suso-Ribera et al., 2019; Albakri et al., 2022). By viewing your own body and behaviors in interaction with a virtual element, AR can provide an ecologically valid exposure experience that can enhance therapy engagement (Baus & Bouchard, 2014). ARET can be defined as “an add-on to or type of exposure therapy in which clients can interact with a virtual representation of an object or situation in the actual world to help them reduce fear or avoidance” (De Witte et al., 2020).
Benefits of DMH interventions include the provision of objects and contexts that are readily available for therapy, independent of seasonal variations (e.g., spiders) or local availability (e.g., snakes). Therapists can easily direct these animals and elicit behaviors that can activate individual fear responses (e.g., locomotion, vocalization), facilitating gradual and hierarchical exposure. Exposure experiences can easily be provided in multiple contexts and through homework exercises (to diminish the risk of renewal of fear; Bandarian-Balooch et al., 2015). Additionally, new technologies can promote acceptability of anxiety treatment in participants, since previous work has indicated a higher willingness to engage in exposure treatment with virtual spiders as opposed to their real counterparts (Garcia-Palacios et al., 2001,2007).
Similar to VR though, AR experiences need to be perceived as realistic (i.e., there needs to be a convergence between expectations and experiences of the user) and promote “co-presence” with the virtual elements (Baus & Bouchard, 2014). Additionally, the activation of the fear response is the key element of exposure therapy, as proposed by several theoretical frameworks, such as emotional processing theory (EPT; Foa & Kozak, 1986) and inhibitory learning theory (Craske et al., 2014). Therefore, the virtual representation in AR needs to be able to evoke discomfort and anxiety to be useful for exposure therapy in clinical samples. Immersive experiences can be offered through multiple technological modalities, which could influence AR experiences and resulting co-presence and activation of the fear response. Previous research on AR in specific phobia has employed several technology-based modalities, such as a projection-based display (Wrzesien et al., 2011), a smartphone application (Botella et al., 2011; Zimmer et al., 2021), or a Head-Mounted Display (HMD) (Juan et al., 2004; Botella et al., 2005). These modalities differ in technological embodiment, visual presentation, and user experience (UX). Technological embodiment refers to the level to which experiences are integrated with the human body and extend natural bodily capacities (Flavián et al., 2019). When looking at the technological embodiment continuum, smartphones are viewed as external portable devices while wearable HMDs are situated closer to the internal technologies (promoting higher levels of technological embodiment). In this capacity, HMDs can strengthen sense of presence and promote visual appeal in customer experience (Orús et al., 2021), which could potentially translate to better quality of the therapeutic AR experience. In terms of visual presentation, a smartphone displays the object superimposed over the real-world surroundings while the optical lenses of an AR headset (e.g., HoloLens 2) result in a more transparent object appearance. Finally, UX has been deemed important for DMH adoption and behavioral program engagement (Ramtohul, 2015; Hentati et al., 2021), and different AR devices vary in user interfaces and interactions. These three important factors could shape modality suitability for ARET, but no studies have compared AR modalities directly (to our knowledge).
The current study aims to investigate the potential of the Microsoft HoloLens 2 headset for future use in exposure therapy by comparing its performance and personal preferences in comparison with a smartphone AR application. A within-subjects counterbalanced study design with two hierarchical Behavioral Approach Tasks (BATs) was implemented in a general sample to gain insight into 1) the extent to which a virtual spider viewed through an AR headset or smartphone application evokes discomfort and anxiety (as shown by self-report and physiological measures) and 2) participants’ personal experiences with and preferences for both technological modalities. The more continuous and handsfree visual experience of a headset can contribute to technological embodiment, which could improve the quality of the AR experience as compared to a handheld device. Therefore, our primary hypothesis is that exposure to virtual spiders through the HoloLens 2 will result in higher anxiety levels compared to the smartphone condition. Since technological embodiment has been linked to immersion and higher levels of experienced realism can lead to a stronger increase in anxiety in a Behavioral Approach Task (BAT) with virtual animals (De Witte et al., 2020), our second hypothesis is that the HoloLens 2 will lead to higher levels of subjective experienced realism compared to the smartphone condition and that experienced realism is related to individual differences in anxiety levels in the BAT task. Finally, we explored personal preferences regarding the implemented technology modality.
2 Materials and methods
2.1 Sample
Sixty-five, first-year applied psychology students at Thomas More University of Applied Sciences participated in the study. Fourteen were male, 49 female, and one person identified as non-binary (1 missing value). Mean age was 19.03 (SD = 3.40; range: 17–43). Since this is the first study, that we know of, directly comparing AR modalities for user experience and effects, we did not want to induce undue stress in patients with anxiety disorders when the underlying principles and user experiences could also be assessed in a general sample (where subclinical fear of spider is very prevalent). There was substantial variability in total scores on the Fear of Spiders Questionnaire (FSQ; described in section 2.5.2), with a mean of 43.42 (SD = 31.93; range 0–126). Participants were invited for participation through e-mail and received course credit for participation. The study was approved by the Social and Societal Ethics Committee of KU Leuven University (G-2020-2591) and participants provided informed consent prior to study participation.
2.2 Virtual rendering of the spider
A spider was the most feared animal in our previous work, and we consequently selected the spider model of the iOS PHOBOS AR app that provoked the highest levels of anxiety to implement in both modalities (De Witte et al., 2020). The commercially available iOS app PHOBOS AR (Tarnogol, 2018) was used for the presentation of the virtual spider on a smartphone (iPhone 12 Pro; 6.1 inch screen size, 60 Hz refresh rate) (Figure 1). A description of the app can be found in De Witte et al. (2020). The headset modality made use of the Microsoft HoloLens 2 (Field of view: 52°, 75 Hz refresh Rate). Through built-in sensors, cameras, and algorithms, such as Simultaneous Localization And Mapping (SLAM), the HoloLens’ optical system displays computer models in a relatively fixed position in the physical space. The wearer can interact with the holograms through gestures and can change their position and viewpoint while the virtual object remains visually stable in its location. For the current study a dedicated HoloLens 2 application, which consisted of the five BAT steps, was developed in Unity 2019.4.10f1, using the Mixed Reality Toolkit (MRTK) version 2.4.0 by Microsoft. Position of User Interface (UI) elements was made configurable through grab gestures and placing the spider on the starting position was implemented using the “tap to place” gesture. The HoloLens 2 application has been made available through Github (https://github.com/SpatialEffects/Hololens-Specific-Phobia-Build).
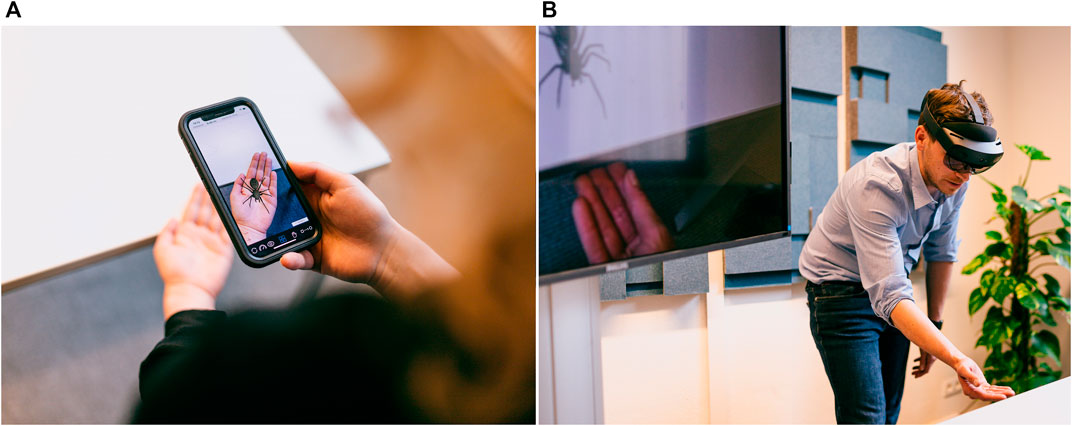
FIGURE 1. Visualization of the last step from the Behavioral Approach Task in the smartphone (A) and HoloLens 2 condition (B).
2.3 Behavioral approach task
A BAT was designed to assess levels of avoidance and anxiety according to Subjective Units of Distress (SUDs). Since the study included general sample participants, the outcome of interest was reported anxiety during each step as opposed to the number of completed steps. All participants were able to complete all BAT steps in both modalities. The BAT consisted of five consecutive steps with increasing difficulty, achieved through a closer distance and movement of the animal - in line with Bretón-López et al. (2010), and a duration of approximately 20 s each. The five steps of the BAT were: 1) the spider is stationary on the table at 1 m distance, 2) the participant moves around the room and continues to look at the stationary spider, 3) the spider walks to 50 cm distance, 4) the participant moves around and continues to look at the stationary spider, 5) the spider walks from 50 cm to the hand and stops on the participant’s hand. To avoid participants making errors using the novel HoloLens 2 technology, the spider automatically stopped walking at the end of step 3 and 5 in this modality. Participants pushed a button to stop the spider in the PHOBOS AR app. Steps 2 and 4 allowed participants to move in the room and observe the stationary spider from different angles, which was hypothesized to increase the levels of realism. After each step, participants were asked to report their anxiety in SUDs through the following instruction: “After each step I’m going to ask you, on a scale of 0–10 how anxious you feel at that moment. A score of 0 means you are free of anxiety or tension and a score of 10 means you are extremely anxious or tense."
2.4 Physiological measurement
Heart Rate (HR) and Skin Conductance (SC) were measured continuously at the wrist with the Empatica E4. This wearable device measures the Blood Volume Pulse (BVP) signal using green and red LED technologies at a sampling rate of 64 Hz. BVP data was filtered with a bandpass filter (cutoff frequencies of 22.5 and 200 bpm) followed by a median filter (filter length 35) and a mean filter (filter length 35) to remove noise. This filtered signal was used to estimate HR. Two silver (Ag) electrodes measured SC at the inner wrist at a 4 Hz sampling rate. Raw SC data was filtered using a low pass filter with a cutoff frequency of 0.4 Hz. Data was analyzed through a sliding window of 60s and we compared HR and SC during the 60s before the start and at the highest difficulty level of each of the BAT tasks (i.e., 60s around the end of step 5).
2.5 Questionnaires
2.5.1 The Igroup Presence Questionnaire
The Igroup Presence Questionnaire (IPQ; Regenbrecht & Schubert, 2002) was implemented to assess experienced realism in each AR condition. While this questionnaire is tailored to a full virtual environment, the experienced realism subscale can also be applied to AR. It contains four items and has an acceptable reliability (Cronbach’s α = 0.68 over both questionnaire administrations).
2.5.2 Fear of Spiders Questionnaire
Self-reported fear of spiders was measured through the Dutch translation of the FSQ (Szymanski & Donohue, 1995; Muris & Merckelbach, 1996). The scale contains 18 items and has excellent reliability (Cronbach’s α = 0.97 in the current sample).
2.6 Procedure
All participants were subjected to both technological modalities in an A-B design. Participants completed the FSQ online prior to testing. At arrival, participants were briefed and the Empatica E4 was put on the non-dominant hand. Participants then performed the BAT task in both modalities. The order in which conditions were presented was controlled for in a counterbalanced measure design. After each BAT task the IPQ was completed and at the end of the experiment participants were presented with additional multiple choice and open-ended questions to collect qualitative information regarding modality preference, realism and level of anxiety related to the spiders, and whether their attitude towards spiders was changed after the experiment. Last-year students of applied psychology collected the data, under supervision of a clinical psychologist (TVD).
2.7 Data reduction and statistical analysis
Data were tested for normality and sphericity. Due to violation of assumptions, non-parametric tests were used for all statistical analyses. The first hypothesis was tested using two non-parametric Friedman tests assessing whether increasing levels of difficulty in the BAT were associated with differences in levels of self-reported anxiety from the SUDs in both AR modalities. Wilcoxon signed-rank tests were used to assess differences between the modalities during the specific BAT steps. Changes from pre-test to post-test in SC and HR were also analyzed using two Wilcoxon signed-rank tests. Since settling-in effects influence the amplitude of SC data, the physiology analysis only included participants who wore the device for 5 min or more before the start of the task (N = 42). To test hypothesis 2, we conducted a Wilcoxon signed-rank tests to assess overall differences in the IPQ - perceived realism scores between the two modalities. The wilcoxon effect size (r) was calculated. Additionally, Spearman’s correlations were conducted for each modality separately to assess whether experienced realism is related with increases in SUDs, SC, and HR. Participants’ experiences and preferences were analyzed through thematic and frequency analysis, performed by author NDW in discussion with TVD.
3 Results
3.1 Modality differences in anxiety responses in the BAT task
Non-parametric Friedman tests showed significant differences in SUDs between the steps of the BAT in the smartphone, χ2 (4) = 163.68, p < 0.001, and the HoloLens 2 modality, χ2 (4) = 172.01, p < 0.001 (Figure 2). Wilcoxon signed-rank tests indicated that there was only a significant difference between the two modalities at step 3, when the spider started walking, Z = -3.59, p < 0.001, r = 0.31, with higher SUDs in the HoloLens 2 modality (M = 2.89, SD = 2.20) as compared to the smartphone modality (M = 2.02, SD = 1.81). The FSQ total score shows a positive Spearman rank-order correlation with the increase in SUDs from step 1 to step 5 in the smartphone, r (65) = 0.28, p = 0.02, and HoloLens 2 modality, r (65) = 0.36, p = 0.003, indicative of stronger increases in SUDs in participants with more symptoms of fear of spiders.
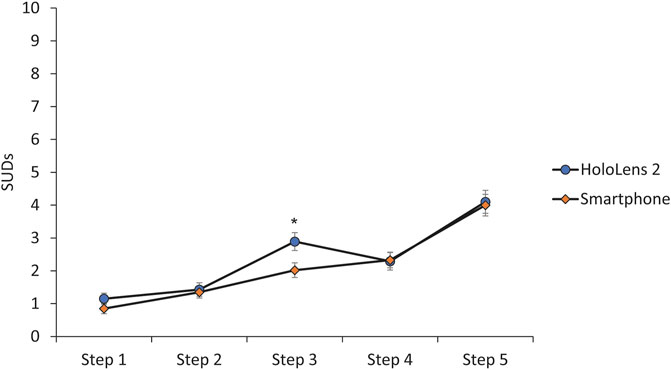
FIGURE 2. Self-reported subjective Units of Distress (SUDs) at each of the five steps of the BAT in both conditions (error bars represent the standard error of the mean). * marks a significant difference at p < 0.05 at step 3.
Wilcoxon signed-rank tests showed that there was a significant difference in SC from the start to the end of the BAT in the HoloLens 2 modality, Z = -3.07, p = 0.002, r = 0.37, but not in the smartphone modality, Z = -1.88, p = 0.06 (Figure 3). Heart rate did not change during the BAT task in the smartphone, Z = -1.72, p = 0.09, and HoloLens 2 modality, Z = -0.181, p = 0.86.
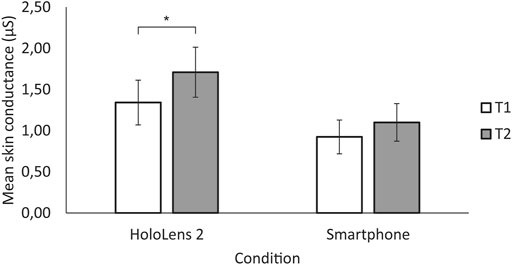
FIGURE 3. Mean skin conductance level before the start of the BAT task (T1) and at the most difficult level of the BAT task (T2) (error bars represent the standard error of the mean). * marks a significant difference at p < 0.05 in the HoloLens 2 modality.
3.2 Effects of experienced realism
A Wilcoxon signed-rank test did not reveal a difference in experienced realism (as measured by the IPQ subscale) between the HoloLens 2 (M = 2.26, SD = 1.04) and smartphone modality (M = 2.12, SD = 1.13), Z = -0.402, p = 0.69 (Supplementary Table S1). There was a significant relation between experienced realism and the increase in SUDs in the HoloLens 2 modality, r (65) = 0.34, p = 0.01 but not the smartphone modality, r (65) = 0.20 p = 0.12. Figure 4 shows that individuals with higher levels of experienced realism report higher SUDs throughout the BAT task in the HoloLens 2 condition. Similarly, higher levels of experienced realism were related to stronger increases in SC in the HoloLens 2 modality, r (42) = 0.42, p = 0.01, but not in the smartphone modality, r (42) = 0.14, p = 0.38. Experienced realism was not associated with an HR increase in the smartphone, r (42) = 0.17, p = 0.28, and HoloLens 2 modalities, r (42) = 0.09, p = 0.55.
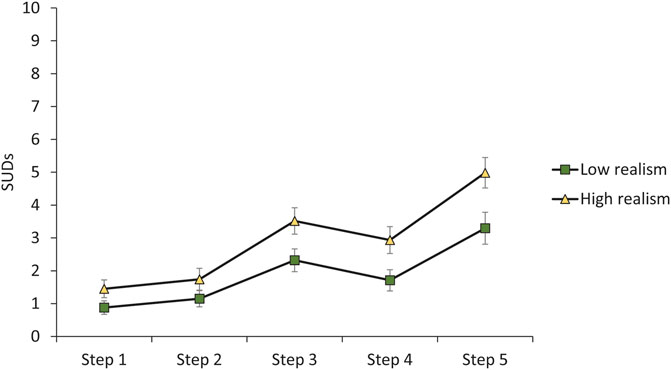
FIGURE 4. Self-reported subjective units of distress (SUDs) in each of the steps of the BAT in the HoloLens 2 condition, depending on experienced realism. The groups were created through a median split (low realism, N = 34; high realism; N = 31) (error bars represent the standard error of the mean).
3.3 Experiences and preferences
Participants were asked which device would be most suitable and comfortable to use in future therapy. Eight participants chose the device (smartphone, n = 4; HoloLens 2, n = 4) which they thought offered the least realism (and lower resulting fear). Of the remaining participants, 32 preferred the HoloLens 2 condition, mainly because they experienced the spider as a more realistic integration with the surroundings (n = 23), preferred the handsfree experience (n = 3), or experienced its lower avoidance opportunities as an added value (n = 3). Three participants simply liked the attractive design. The smartphone condition was preferred by 25 participants since they felt this was more realistic due to the colors (n = 23), which were more pronounced as compared to the HoloLens 2 hologram, or because it was easier to use (n = 2). A similar approximate 50–50 split was found regarding which medium was experienced as most realistic (HoloLens 2, n = 26; smartphone, n = 33; both, n = 2; neither, n = 2) and concerning in which medium the spider was most frightening (HoloLens 2, n = 30; smartphone, n = 28; both, n = 1; neither, n = 4). When asked whether their attitude towards spiders changed after this brief experiment, most reported that it had not and that they were still anxious (n = 28) or still not anxious (n = 24) towards spiders. Eleven participants did report that they were less anxious of spiders. None reported being more anxious after experiment completion.
4 Discussion
In both AR modalities self-reported discomfort increased during the BAT task, indicating that increasing proximity and movement of a virtual spider can induce subjective anxiety. This was also supported by an increase in SC in the HoloLens 2 modality. This finding is in line with previous work stating that virtual animals can implicate a physiological challenge and evoke associated subjective anxiety (Diemer et al., 2014; De Witte et al., 2020). Self-reported anxiety levels during the BAT tasks indicate that movement of the spider itself induces a stronger rise in fear as compared to movement of the participant in the room, which is in line with findings from Van Uijen et al. (2015) suggesting that active approach adds to exposure effects.
About half of participants reported the spider being more frightening in the HoloLens 2 modality while the other half thought the spider in the smartphone condition was more frightening and, while anxiety increased with difficulty in both conditions, there was no general effect of modality on the SUDs. However, anxiety levels did differ between conditions when the spider started walking towards the participant for the first time. This spider locomotion was perceived as more threatening in the continuous headset experience. Higher levels of technological embodiment could make this activity a stronger sensory stimulation, contributing to an elevated fear response. However, a difference in perceived level of control could also influence the experience since participants in the smartphone condition were asked to press a button that stopped the movement of the spider when it reached the 50 cm mark, as opposed to the spider stopping automatically in the HoloLens 2 application. Experiencing control over stressful situations can diminish distress, although studies manipulating level of control during exposure experience have not been able to replicate this finding (Maier & Watkins, 2005; Healey et al., 2017). Nevertheless, participants with greater control during a computerized exposure task were able to approach closer towards a spider (Healey et al., 2017). Perceptual control theory suggests that exposure is more effective when clients have increased control over the process, for example in self-guided virtual reality exposure therapy (Healey et al., 2017; Premkumar et al., 2021).
In contrast to our hypothesis, modalities did not differ in experienced realism. While higher technological embodiment could increase immersion in VR applications (Orús et al., 2021), qualitative data suggest that transparency of the spider was a disadvantage for the HoloLens 2 in the current study. Nevertheless, higher experienced realism was associated with increased anxiety within the HoloLens 2 condition but not in the smartphone condition, contrary to our previous work (De Witte et al., 2020). While reviews and meta-analyses emphasize the importance of realism to attain effective exposure in XR, empirical research into perceived realism in ARET is lacking (Powers & Emmelkamp, 2008; Baus & Bouchard, 2014). We also need better instruments to measure realism in AR since the IPQ is not tailored an AR context and internal consistency was suboptimal in the current sample.
Participants’ preferences regarding modality were mixed. The continuous handsfree integration of the spider in the physical surrounding resulted in approximately half the sample preferring the HoloLens 2 headset. However, the translucency of the spider in this modality made the remainder of the sample prefer the more realistic representation of the spider in the smartphone application. In practice, the optimal ARET modality should therefore depend on the specific patient’s preference. However, the future of XR lies in merging VR and AR functionalities in one headset allowing for passthrough VR (Hillmann, 2021), a new technology that could accommodate the drawbacks of both modalities. Passthrough VR refers to the implementation of a VR headset which captures the physical surroundings through a camera and superimposes the virtual object. Therefore, the spider could look like the current smartphone application but be presented through a continuous HMD experience. Other potential future avenues for designers of XR exposure are the integration of haptic feedback, embedding it in a self-help application, personalization (based on physiological responses), and gamification (Wiederhold & Bouchard, 2014; Kurscheidt et al., 2019; Mahmoudi-Nejad et al., 2021; Dibbets, & Schruers, 2022; Toffolo et al., 2022).
The current study has several limitations. Some participants went through the experimental procedure more quickly than expected, which reduced physiology settling in periods and resulted in exclusion of some participants from physiological analyses to guarantee a stable measurement signal. While cybersickness can also be present in AR (yet generally to a lesser extent than VR, especially with short exposure times) and lead to increased SC, effects have mostly been found on forehead SC measurements (Gavgani et al., 2017; Hughes et al., 2020). Peripheral measures, such as the current one, are generally linked to task-based arousal. Using the same virtual spider in both sequentially administered BAT tasks could attenuate anxiety levels in the second condition, but counterbalancing the order prevented bias in the overall findings. Findings in a general population cannot be directly transferred to phobic individuals. There is no gender parity in the sample. Future controlled research (RCT) should provide insight into the active ingredients promoting the anxiety-inducing potential of animals presented through different AR modalities and fully harmonize applications running on different modalities.
The current study assessed whether virtual spiders can induce anxiety and could consequently be a good candidate for inclusion in ARET in line with existing theory (Foa & Kozak, 1986; Craske et al., 2014). Virtual spiders induced equivalent levels of subjective anxiety in an AR smartphone application or HMD. Perceived realism did not differ between conditions and preferences regarding the technology of choice varied strongly. As it stands, the AR smartphone application has a larger clinical potential since smartphones are more ubiquitous and affordable, while the HoloLens 2 is uncommon and situated in a higher price range. Future research should further clarify the role of perceived realism and assess whether new XR modalities and features could promote the therapeutic potential of ARET.
Data availability statement
The datasets presented in this study can be found in online repositories. The names of the repository/repositories and accession number(s) can be found below: The data from this study are available in the OSF repository through this link: https://doi.org/10.17605/OSF.IO/7RJF2.
Ethics statement
The studies involving human participants were reviewed and approved by Social and Societal Ethics Committee of KU Leuven University. The patients/participants provided their written informed consent to participate in this study.
Author contributions
TVD and NDW designed the study and conducted the experiments. BB and GD processed the physiological parameters and FB performed the statistical analyses. WS designed the HoloLens 2 application with support of FT. NDW wrote the first draft of the paper. All authors contributed to the article and approved the submitted version.
Conflict of interest
FT is the developer of PHOBOS AR.
The remaining authors declare that the research was conducted in the absence of any commercial or financial relationships that could be construed as a potential conflict of interest.
Publisher’s note
All claims expressed in this article are solely those of the authors and do not necessarily represent those of their affiliated organizations, or those of the publisher, the editors and the reviewers. Any product that may be evaluated in this article, or claim that may be made by its manufacturer, is not guaranteed or endorsed by the publisher.
Supplementary material
The Supplementary Material for this article can be found online at: https://www.frontiersin.org/articles/10.3389/frvir.2022.1066996/full#supplementary-material
References
Albakri, G., Bouaziz, R., Alharthi, W., Kammoun, S., Al-Sarem, M., Saeed, F., et al. (2022). Phobia exposure therapy using virtual and augmented reality: A systematic review. Appl. Sci. 12, 1672. doi:10.3390/app12031672
Bandarian-Balooch, S., Neumann, D. L., and Boschen, M. J. (2015). Exposure treatment in multiple contexts attenuates return of fear via renewal in high spider fearful individuals. Journal of Behavior Therapy and Experimental Psychiatry 47, 138–144. doi:10.1016/j.jbtep.2014.12.006
Baus, O., and Bouchard, S. (2014). Moving from virtual reality exposure-based therapy to augmented reality exposure-based therapy: A review. Frontiers in Human Neuroscience 8, 1–15. doi:10.3389/fnhum.2014.00112
Botella, C., Breton-López, J., Quero, S., Baños, R. M., García-Palacios, A., Zaragoza, I., et al. (2011). Treating cockroach phobia using a serious game on a mobile phone and augmented reality exposure: A single case study. Computers in Human Behavior, 27, 217–227. doi:10.1016/j.chb.2010.07.043
Botella, C. M., Juan, M. C., Baños, R. M., Alcañiz, M., Guillén, V., and Rey, B. (2005). Mixing realities? An application of augmented reality for the treatment of cockroach phobia. Cyberpsychology, Behavior 8, 162–171. doi:10.1089/cpb.2005.8.162
Botella, Cristina, Fernández-Álvarez, J., Guillén, V., García-Palacios, A., and Baños, R. (2017). Recent progress in virtual reality exposure therapy for phobias: A systematic review. Curr. Psychiatry Rep. 19. doi:10.1007/s11920-017-0788-4
Bretón-López, J., Quero, S., Botella, C., García-Palacios, A., Baños, R. M., and Alcañiz, M. (2010). An augmented reality system validation for the treatment of cockroach phobia. Cyberpsychology, Behavior, and Social Networking, 13, 705–710. doi:10.1089/cyber.2009.0170
Choy, Y., Fyer, A. J., and Lipsitz, J. D. (2007). Treatment of specific phobia in adults. Clinical Psychology Review, 27, 266–286. doi:10.1016/j.cpr.2006.10.002
Craske, M., Treanor, M., Conway, C., Zbozinek, T., and Vervliet, B. (2014), Maximizing exposure therapy: An inhibitory learning approach, Behaviour Research and Therapy, 58. 10–23. doi:10.1016/j.brat.2014.04.006
De Witte, N. A. J., Joris, S., Van Assche, E., and Van Daele, T. (2021). Technological and digital interventions for mental health and wellbeing: An overview of systematic reviews. Front. Digit. Health 3, 1–10. doi:10.3389/fdgth.2021.754337
De Witte, N. A. J., Scheveneels, S., Sels, R., Debard, G., Hermans, D., and Van Daele, T. (2020). Augmenting exposure therapy: Mobile augmented reality for specific phobia. Front. Virtual Real. 1, 1–12. doi:10.3389/frvir.2020.00008
Dibbets, P., and Schruers, K. (2022). An online spider game: Overcome your fear, exposure is near. Computers in human behavior reports, 6, 100201. doi:10.1016/j.chbr.2022.100201
Diemer, J., Mühlberger, A., Pauli, P., and Zwanzger, P. (2014). Virtual reality exposure in anxiety disorders: Impact on psychophysiological reactivity. World Journal of Biological Psychiatry, 15, 427–442. doi:10.3109/15622975.2014.892632
Flavián, C., Ibáñez-Sánchez, S., and Orús, C. (2019). The impact of virtual, augmented and mixed reality technologies on the customer experience. Journal of Business Research, 100, 547–560. doi:10.1016/j.jbusres.2018.10.050
Foa, E. B., and Kozak, M. J. (1986). Emotional processing of fear. Exposure to corrective information. Psychological Bulletin, 99, 20–35. doi:10.1037/0033-2909.99.1.20
Gadermann, A. M., Alonso, J., Vilagut, G., Zaslavsky, A. M., and Kessler, R. C. (2012). Comorbidity and disease burden in the national comorbidity survey replication (NCS-R). Depression and Anxiety, 29, 797–806. doi:10.1002/da.21924
Garcia-Palacios, A., Botella, C., Hoffman, H., and Fabregat, S. (2007). Comparing acceptance and refusal rates of virtual reality exposure vs. in vivo exposure by patients with specific phobias. Cyberpsychology, Behavior, 10, 722–724. doi:10.1089/cpb.2007.9962
Garcia-Palacios, A., Hoffman, H. G., See, S. K., Tsai, A., and Botella, C. (2001). Redefining therapeutic success with virtual reality exposure therapy. Cyberpsychology, Behavior, 4, 341–348.
Gavgani, A. M., Nesbitt, K. V., Blackmore, K. L., and Nalivaiko, E. (2017). Profiling subjective symptoms and autonomic changes associated with cybersickness. Autonomic Neuroscience, 203, 41–50. doi:10.1016/j.autneu.2016.12.004
Healey, A., Mansell, W., and Tai, S. (2017). An experimental test of the role of control in spider fear. Journal of Anxiety Disorders, 49, 12–20. doi:10.1016/j.janxdis.2017.03.005
Hentati, A., Forsell, E., Ljótsson, B., Kaldo, V., Lindefors, N., and Kraepelien, M. (2021). The effect of user interface on treatment engagement in a self-guided digital problem-solving intervention: A randomized controlled trial. Internet Interventions, 26, 100448. doi:10.1016/j.invent.2021.100448
Hillmann, C. (2021). UX for XR. Apress Berkeley. Springer, Berlin, Germany, doi:10.1007/978-1-4842-7020-2
Hughes, C. L., Fidopiastis, C., Stanney, K. M., Bailey, P. S., and Ruiz, E. (2020). The psychometrics of cybersickness in augmented reality. Frontiers in Virtual Reality, 1, 1–12. doi:10.3389/frvir.2020.602954
Juan, M. C., Alcañiz, M., Monserrat, C., Botella, C., Baños, R. M., and Guerrero, B. (2005). Using augmented reality to treat phobias. IEEE Computer Graphics and Applications, 25, 31–37. doi:10.1109/MCG.2005.143
Kurscheidt, M., Ostapchuck, V., Sottek, F., Rauh, S. F., and Meixner, G. (June 2019). Augmented reality exposure therapy with tactile feedback for small animal phobia : Hardware concept and user study design. Proceedings of the 2019 IEEE International Conference on Healthcare Informatics (ICHI), Xi'an, China, IEEE, 1–7. doi:10.1109/ICHI.2019.8904800
Mahmoudi-Nejad, A., Guzdial, M., and Boulanger, P. (October 2021). Arachnophobia exposure therapy using experience-driven procedural content generation via reinforcement learning (EDPCGRL). Proceedings of the. Seventeenth AAAI Conference. Artificial Intelligence Digital EntertainmentAIIDE'21), IEEE, 9, 164–171.
Maier, S. F., and Watkins, L. R. (2005). Stressor controllability and learned helplessness: The roles of the dorsal raphe nucleus, serotonin, and corticotropin-releasing factor. Neuroscience & Biobehavioral Reviews, 29, 829–841. doi:10.1016/j.neubiorev.2005.03.021
Muris, P., and Merckelbach, H. (1996). A comparison of two spider fear questionnaires. Journal of Behavior Therapy and Experimental Psychiatry, 27, 241–244.
Oosterink, F. M. D., De Jongh, A., and Hoogstraten, J. (2009). Prevalence of dental fear and phobia relative to other fear and phobia subtypes. European Journal of Oral Sciences, 117, 135–143. doi:10.1111/j.1600-0722.2008.00602.x
Orús, C., Ibáñez-Sánchez, S., and Flavián, C. (2021). Enhancing the customer experience with virtual and augmented reality: The impact of content and device type. International Journal of Hospitality Management, 98, 103019. doi:10.1016/j.ijhm.2021.103019
Öst, L. G. (1989). One-session treatment for specific phobias. Behaviour Research and Therapy, 27, 1–7.
Powers, M. B., and Emmelkamp, P. M. G. (2008). Virtual reality exposure therapy for anxiety disorders: A meta-analysis. Journal of Anxiety Disorders, 22, 561–569. doi:10.1016/j.janxdis.2007.04.006
Premkumar, P., Heym, N., Brown, D. J., Battersby, S., Sumich, A., Huntington, B., et al. (2021). The effectiveness of self-guided virtual-reality exposure therapy for public-speaking anxiety. Front. Psychiatry 12, 1–12. doi:10.3389/fpsyt.2021.694610
Ramtohul, I. (2015). The adoption of e-health services: Comprehensive analysis of the adoption setting from the user׳s perspective. Health Policy Technology, 4, 286–293. doi:10.1016/j.hlpt.2015.04.007
Regenbrecht, H., and Schubert, T. (2002). Real and illusory interactions enhance presence in virtual environments. Presence: Teleoperators & Virtual Environments, 11, 425–434. doi:10.1162/105474602760204318
Suso-Ribera, C., Fernández-Álvarez, J., García-Palacios, A., Hoffman, H. G., Bretón-López, J., Baños, R. M., et al. (2019). Virtual reality, augmented reality, and in vivo exposure therapy: A preliminary comparison of treatment efficacy in small animal phobia. Cyberpsychology, Behavior, and Social Networking, 22, 31–38. doi:10.1089/cyber.2017.0672
Szymanski, J., and Donohue, W. O. (1995). Fear of spiders questionnaire. Journal of Behavior Therapy and Experimental Psychiatry, 26, 31–34. doi:10.1016/0005-7916(94)00072-T
Tarnogol, F. M. (2018). Phobos AR (Version 1.3.2) [Mobile app]. Available at: Apple’s App Store (Downloaded: 19 October 2021)
Toffolo, M. B. J., Fehribach, J. R., van Klaveren, C. P. B. J., Cornelisz, I., van Straten, A., van Gelder, J-L., et al. (2022). Automated app-based augmented reality cognitive behavioral therapy for spider phobia: Study protocol for a randomized controlled trial. PLoS ONE 17, e0271175. doi:10.1371/journal.pone.0271175
van Uijen, S. L., van den Hout, M., and Engelhard, I. M. (2015). Active approach does not add to the effects of in vivo exposure. Journal of Experimental Psychology, 6, 112–125. doi:10.5127/jep.042014
Wiederhold, B., and Bouchard, S. (2014). “Arachnophobia and fear of other insects: Efficacy and lessons learned from treatment process,” in Advances in virtual reality and anxiety disorders. Series in Anxiety and Related Disorders (Boston, MA: Springer). doi:10.1007/978-1-4899-8023-6_5
Wrzesien, M., Burk hardt, J.-M., Alcañiz, M., and Botella, C. (September 2011). How technology influences the therapeutic process: A comparative field evaluation of augmented reality and in vivo exposure therapy for phobia of small animals, Proceedings of the Human-Computer Interaction - INTERACT 2011 - 13th IFIP TC 13 International Conference, 6946. IEEE, Lisbon, Portugal. doi:10.1007/978-3-642-23774-4
Zimmer, A., Wang, N., Ibach, M. K., Fehlmann, B., Schicktanz, N. S., Bentz, D., et al. (2021). Effectiveness of a smartphone-based, augmented reality exposure app to reduce fear of spiders in real-life: A randomized controlled trial. Journal of Anxiety Disorders, 82, 1–22. doi:10.1016/j.janxdis.2021.102442
Keywords: specific phobia, arachnophobia, anxiety disorders, augmented realitiy, head-mounted display (HMD), exposure therapy
Citation: De Witte NAJ, Buelens F, Debard G, Bonroy B, Standaert W, Tarnogol F and Van Daele T (2022) Handheld or head-mounted? An experimental comparison of the potential of augmented reality for animal phobia treatment using smartphone and HoloLens 2. Front. Virtual Real. 3:1066996. doi: 10.3389/frvir.2022.1066996
Received: 11 October 2022; Accepted: 28 November 2022;
Published: 16 December 2022.
Edited by:
Andrea Sanna, Politecnico di Torino, ItalyReviewed by:
Hugo Brument, Vienna University of Technology, AustriaDavide Calandra, Politecnico di Torino, Italy
Copyright © 2022 De Witte, Buelens, Debard, Bonroy, Standaert, Tarnogol and Van Daele. This is an open-access article distributed under the terms of the Creative Commons Attribution License (CC BY). The use, distribution or reproduction in other forums is permitted, provided the original author(s) and the copyright owner(s) are credited and that the original publication in this journal is cited, in accordance with accepted academic practice. No use, distribution or reproduction is permitted which does not comply with these terms.
*Correspondence: Nele A. J. De Witte, Nele.dw@thomasmore.be