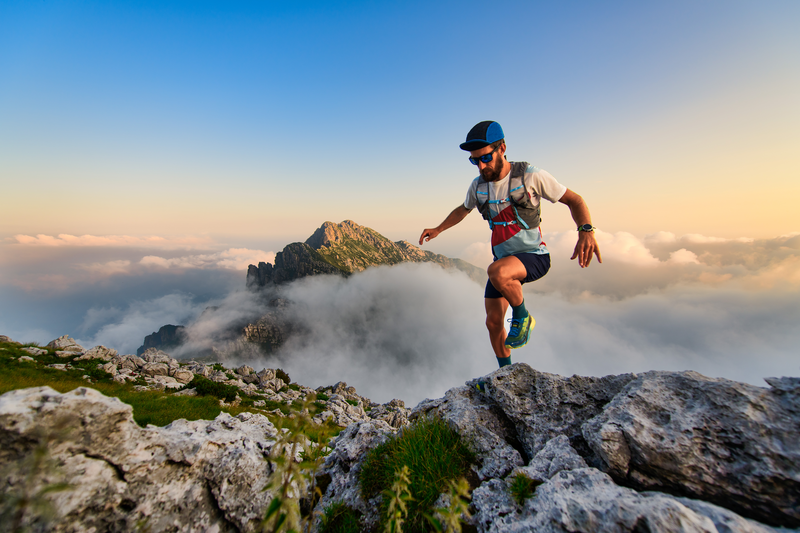
94% of researchers rate our articles as excellent or good
Learn more about the work of our research integrity team to safeguard the quality of each article we publish.
Find out more
ORIGINAL RESEARCH article
Front. Virtual Real. , 09 January 2023
Sec. Virtual Reality and Human Behaviour
Volume 3 - 2022 | https://doi.org/10.3389/frvir.2022.1059971
This paper examines the relationship between time and motion perception in virtual environments. Previous work has shown that the perception of motion can affect the perception of time. We developed a virtual environment that simulates motion in a tunnel and measured its effects on the estimation of the duration of time, the speed at which perceived time passes, and the illusion of self-motion, also known as vection. When large areas of the visual field move in the same direction, vection can occur; observers often perceive this as self-motion rather than motion of the environment. To generate different levels of vection and investigate its effects on time perception, we developed an abstract procedural tunnel generator. The generator can simulate different speeds and densities of tunnel sections (visibly distinguishable sections that form the virtual tunnel), as well as the degree of embodiment of the user avatar (with or without virtual hands). We exposed participants to various tunnel simulations with different durations, speeds, and densities in a remote desktop and a virtual reality (VR) laboratory study. Time passed subjectively faster under high-speed and high-density conditions in both studies. The experience of self-motion was also stronger under high-speed and high-density conditions. Both studies revealed a significant correlation between the perceived passage of time and perceived self-motion. Subjects in the virtual reality study reported a stronger self-motion experience, a faster perceived passage of time, and shorter time estimates than subjects in the desktop study. Our results suggest that a virtual tunnel simulation can manipulate time perception in virtual reality. We will explore these results for the development of virtual reality applications for therapeutic approaches in our future work. This could be particularly useful in treating disorders like depression, autism, and schizophrenia, which are known to be associated with distortions in time perception. For example, the tunnel could be therapeutically applied by resetting patients’ time perceptions by exposing them to the tunnel under different conditions, such as increasing or decreasing perceived time.
Psychological research has identified various factors and effects that influence our space-time perception. Vierordt (1868) was one of the first to establish a law of time perception that relates perceived duration to actual duration over various time intervals and as a function of task complexity. The phenomenon of motion is crucial to any description of relativity. When an object moves through space-time, its motion properties can, to some extent, determine the way the object is perceived. Although controversial, it has been reported that the motion of an object itself causes distortions in the perception of that object from the perspective of an observer, who is in a different frame of reference (Caelli et al., 1982). Psychological relativity can be viewed as the effect of space on temporal perception and vice versa. One example of psychological relativity was named the kappa effect by Abe (1935): A shorter temporal interval can appear longer by increasing the spatial distances between successive flashes. Several studies have shown that time perception is prolonged for fast-moving stimuli compared to slower or stationary stimuli, a phenomenon called (subjective) time dilation (Roelofs and Zeeman, 1951; Goldstone and Lhamon, 1974; Brown, 1995). Time perception in VR can be passively affected by the medium itself as a distraction (Schneider and Hood, 2007; Schneider et al., 2011) or actively by time influencers, so-called ‘zeitgebers’ (e.g., the speed of the Sun’s path) (Schatzschneider et al., 2016), or the illusion of self-motion, also known as ‘vection’ (Dichgans and Brandt, 1978). Previous research revealed how the size of the field of view and visual elements moving in synchrony with visual motion patterns contribute to this illusion (Brandt et al., 1973; Dichgans and Brandt, 1978; Palmisano et al., 2015). VR provides an interesting design space with several dimensions to investigate and manipulate time perception in humans. It could be applied in therapeutic interventions to treat mental disorders known to be related to distorted time perception. An improved understanding of the underlying time experience, as well as the utility and potential impacts of the digital media, is required to develop new diagnostic and therapeutic VR applications. We must understand if and how media-related effects alter time perception if we want to use such tools in real-world applications. Potential effects can be psychological, such as attention, flow, and distraction; and object-related, such as the duration and location of events, the number of these events, their relativity in space and time. Typical VR-related effects include the illusions of self-motion, virtual body ownership, or spatial presence.
This article investigates the relationship between the illusion of self-motion (vection) and subjective time perception in virtual reality. The developed virtual tunnel enables several vection-related simulation characteristics (density and velocity of spatial markers, and exposure duration) to be modified. Two experiments compared the resulting effects of different tunnel settings on time perception between a remote desktop and a laboratory-controlled VR exposure. In the VR experiment there was a condition with virtual hands and one without. Time appeared to have passed subjectively faster when exposed to fast-speed, high-density virtual objects in both studies. Specifically, in the VR study, fast-speed resulted in a significantly stronger vection experience when owning virtual hands and exposed to low-density. Subjects in the immersive-VR study reported higher levels of vection, a faster subjective passage of time, and shorter time estimates compared to the remote desktop study. The overall results contribute to a better understanding of VR effects on time perception to guide the development of diagnostic and therapeutic interventions.
Physical time is understood as the time given and measured using clocks in the real world. Psychological time is often described as being subjective, referring to experienced temporal dimensions of duration, speed, and the order of perceived events (Zakay, 2014). Here we refer to the passage of time as the speed at which time subjectively passes when perceiving events and duration estimation when estimating time frames retrospectively. How we subjectively experience temporal moments can be extremely variable. An influencing factor on this dynamic scale is attention, where splitting the focus between a task and time can lead to a subjective acceleration or deceleration of time. This has been described in psychology with the ‘attentional gate model’ (AGM) (Zakay and Block, 1997), which “…holds that a person may divide attentional resources between attending to external events and attending to time. Attending to time opens the attentional gate, thereby allowing pulses to pass through to the cognitive counter…“, where the accumulated pulses give an estimate of how much time has passed. The number of pulses may increase, depending on factors such as arousal (strong emotional response), which can accelerate the pacemaker’s rate leading to more pulses, and conversely, the subjective experience of time dilation (Alvarez Igarzábal et al., 2021). An ‘optimal experience’ is one in which attention is placed solely on the task, and time appears to pass much faster. This creates a state characterized by a loss of the sense of time and self, and deep concentration on the task at hand (Wittmann, 2015). This altered time perception is a mental state known as ‘flow,’ coined by Csikszentmihalyi (1990). For example, participants in a VR video-game study who experienced higher levels of flow not only performed better in the game, but also experienced time passing more quickly (Rutrecht et al., 2021). The estimation of time is a fundamental feature of interpreting ‘cause and effect,’ meaning that it is imperative to our own feelings of agency (Haggard, 2017), the idea that we as individuals are able to interact with the world and maintain the feeling that it is we who are causing an effect. Both the sense of agency and perceptions of time are present in altered forms in pathological mental conditions, such as depression, autism, and schizophrenia (Allman, 2015; Kühn et al., 2018; Martin et al., 2019). How time passes has been the subject of much research, particularly on how one can modulate the subjective experience. Promising results have been found by applying modern technological advancements from the realm of gaming and virtual environments. For example, Kühn et al. (2018) were able to modulate the subjective time experience as a therapeutic approach. In addition to the mediation of perceived time through attention, psychological research has already identified factors and effects that influence our relativity of space-time perception. Probably the first to demonstrate psychological relativity was Benussi and Winter (1913). He demonstrated that judgments of spatial distances could depend on the time scales in which those distances are presented. Helson and King (1931) extended these findings into the tactile domain. They named this phenomenon, in which longer time intervals appear to take place over larger spatial distances, the ‘tau effect.’ Abe (1935) found that a longer temporal interval could be made to appear shorter by decreasing the spatial distance between successive flashes, and vice versa; i.e., a shorter temporal interval could be made to appear longer by increasing the spatial distance between successive flashes. Cohen et al. (1953) replicated Abe’s work, calling it the ‘kappa effect.’ Brown (1995) investigated the effects of motion stimulus on time perception (number of stimuli and different speeds from no movement at all to very fast). Faster speeds lengthened perceived time to a greater degree than slower speeds, and intervals associated with more changes were perceived to be longer than intervals with fewer changes. Kaneko and Murakami (2009) investigated further how this illusion occurs and they systematically manipulated temporal frequency, spatial frequency, and the speed of the stimulus to identify the determining factor. Their results showed that the speed of the stimulus described the manipulation better than either the spatial or temporal frequency. This could be part of higher-level, motion-processing stages in the brain. We accordingly manipulated the speed of external zeitgebers to indirectly manipulate observers‘ attention and time experiences.
Virtual Reality (VR) can be used to create controlled environments that still preserve external validity and allow one to investigate behavior and measure the responses to many questions in a less dangerous environment. LaViola (2017) defined VR as “…an approach that uses displays, tracking, and other technologies to immerse the user in a virtual environment.” This virtual environment can be seen from a first-person perspective through a display device that is under the user’s real-time control. When users are more convinced they are inside the virtual environment, it is traditionally called the ‘sense of presence’ (Steuer, 1992; Bryson, 2013; Lelyveld and Entertainment, 2015). Presence is also often extended to the concept of a place illusion. This refers to the virtual place, and consequently the plausibility of events occurring in this virtual environment is often referred to as a ‘plausibility illusion’ (Sanchez-Vives and Slater, 2005; Slater, 2009). Schneider and Hood (2007); Schneider et al. (2011) already demonstrated that by immersing patients inside a virtual environment (VE), time perception can be passively influenced by the medium as a distraction itself. Malpica et al. (2022) provided evidence that larger visual changes (traditional media vs. VR) shorten perceived time. Schatzschneider et al. (2016) proposed that subjective time perception could be scaled inside a VE and manipulated by time influencers, ‘zeitgebers.’ “Zeitgebers are cues that help to locate oneself in time or to mark the passing of time. i.e., the speed of time” (Schatzschneider et al., 2016). This concept was extended in work by Landeck et al. (2020). They proposed a framework consisting of virtual zeitgebers that can perform in three dimensions that seem to be important to investigate the influence on our subjective experience of time: 1) velocity, 2) density, and 3) synchronicity. They did not investigate whether the perceived velocity of objects can contribute to the feeling of self-motion if large parts of the visual field are moving consistently. Weber et al. (2020) were the first to test the illusion of self-motion on prospective time judgements in a VR simulation. Fast driving led to a significant overestimation of time; vection led to an overestimation of short intervals. The amount of presence did not significantly influence the accuracy of the time judgements. Because very short time durations were chosen, it was likely that time spans were too short to really induce an illusion of self movement. Vection has been shown to increase with exposure time (Seno et al., 2018). Seno et al. (2011) had previously shown that the illusion of self-motion affects duration estimates. They focused only on the estimated time of a return trip, hypothesizing that these are estimated to be shorter than outward journeys. This effect could only be observed in combination with a feeling of self-motion perception. In both, the work by Weber et al. (2020) and Seno et al. (2011), there was no embodiment condition and no measurement of time passage or boredom. Friedman et al. (2014); Pizarro et al. (2015) focused more on seeing how participants change their behavior when given the opportunity to turn back (virtual) time. Unfortunately, measurements of the subjective perception of time while experiencing a time-travel illusion were not taken. This remains an interesting approach to the investigation of temporal subjectivity in VR. Kitajima et al. (2020) also investigated whether fear of height influences on subjects‘ time perception in VR, but were unable to derive a significant effect; Bruder and Steinicke (2014) showed that VR did not change subjective time in a walking task compared to a walking task without VR. However, only ten participants took part and time spans between two and 5 s were surveyed, so this may only apply to some specific situations. According to the AGM, time is mediated by the focus on time itself, and resources are devoted either to a task or to raw time, and factors like boredom do not matter (Zakay, 2014). In other situations where no task is present and a so-called waiting situation occurs, boredom and a focus on time do matter. Lugrin et al. (2019) investigated such a waiting-room task in which participants had to wait 7.5 min and had no tasks or distractors available, apart from VR itself as a distractor. The results showed significantly higher retrospective time-duration judgements than an embodied VR or waiting in reality. A possible break in plausibility was also discussed by Unruh et al. (2021): “The absence of a virtual body could produce a deviation in time perception possibly related to reduced plausibility of the virtual environment.” Latoschik and Wienrich (2022) proposed “…making congruence and plausibility the central conditions in a novel theoretical model for describing VR experiences and effects.” Congruent and plausible spatial cues in relation to our own bodies are thus important for the study and manipulation of illusions in VR. More evidence for an influence on time perception in embodied VR simulations was found by Landau et al. (2020). Participants were embodied in virtual child bodies using a 180° stereoscopic video, which significantly contributed to an overestimation of time duration. An interesting approach was chosen by Bansal et al. (2019). They investigated a novel relationship between action and sensory reaction, where they introduced a so-called “movement contingent time-flow” condition in which their real movements were linked to the flow of time in VR. They reported that this relationship affected continuous motor time-perception performance. After an exposure in VR where participant movements were coupled to the time flow in the simulation, participants underestimated the duration of a probe-movement interval by half in a time reproduction task with a continuous-motor method. This supports the idea and concept of recalibrating, the sustainable manipulation of time perception or virtual time perception in VR, and is transferable and meaningful in the context of time-perception-based VR therapy. It also highlights that embodied factors, like the sense of agency, really are important and provide clues about the relationship between body perception and time perception in general.
When large areas of the visual field are moving coherently, observers often tend to perceive this as self-movement rather than movement of the surroundings. This type of illusion is traditionally called ‘visual illusion of self-motion,’ or simply vection (Brandt et al., 1973). Palmisano et al. (2015) conducted a literature overview and comprehensively summarized vection research with it is challenges, definitions, and measures. This work examined vection not only in the visual illusory domain, but also summarized approaches that vection is multifaceted and also includes the vestibular system, the somatosensory system, the proprioceptive system of muscle and joint receptors, and the auditory system. These sensory systems receive signals that register self-movement not only from optical flow. Any one of these senses contributes to the feeling of self-movement, but vision has already proven to play a major role (Brandt et al., 1973). In the literature the term vection is often referred to as illusion of self-motion, visually mediated self-motion, and the subjective experience of self-motion. The main difference between these terms is which sensory manipulation was chosen. Visual or vestibular sensory information contributes to an experience of self-motion mediated through more complex sensory input or controlled and focused on individual channels. When we refer to vection, we refer to the visual illusion of self-motion (Dichgans and Brandt, 1978). According to Palmisano et al. (2015), this still is the most common definition in the literature. Dichgans and Brandt (1978) further differentiated between circular and linear vection. The term vection was already used by Fischer and Wodak (1924) when they wrote about sensations of self-motion induced by optokinetic stimulation and called this Vektionen (German). There is an ongoing discussion in the research community about the functional significance of vection and whether conscious experiences of self-motion are merely intriguing epiphenomena with little or no behavioral relevance. Riecke and Feuereissen (2012) demonstrated that spatial orientation tasks can be significantly improved by the illusion of self-motion. They believe it helped participants to update internal representations of their position and orientation in a virtual environment, suggesting that this might also apply to the real environment. They provided a strong argument against the above-mentioned criticism. To assess the subjective experience of self-motion, previous research often relied on subjective assessments of the onset and end of vection and on subjective ratings of the experienced vection intensity during or after stimulus presentation. Possible promising objective measurements proposed to assess vection include: 1) eye-movements, 2) electroencephalography (EEG), and 3) postural responses. These deserve further investigation, especially since eye-movements and postural data can be easily obtained in a VR study. Vection is closely related to motion sickness, which appears to be triggered by visually induced illusions of motion and missing proprioception information about motion. To exclude such possible interference, we decided to use the Simulator Sickness Questionnaire (SSQ) before and after VR exposure (Kennedy et al., 1993). One of our main contributions is the investigation of the relationship between time perception and the experience of self-motion.
‘Avatar embodiment’ requires motion capturing of a user’s body so that it can be visualized in the first-person perspective via a Head-Mounted Display (HMD). This body substitution provides synchronized visuomotor feedback to the user (Spanlang et al., 2014; Gall and Latoschik, 2018). Embodiment not only promotes immersion (Slater and Steed, 2000; Waltemate et al., 2018), but also psychophysical effects, such as presence, body ownership, and emotional response, which are emphasized by higher immersion and photo-realistic, personalized avatars (Achenbach et al., 2017; Latoschik et al., 2017; Waltemate et al., 2018). The way a person acts, thinks, or feels can also be changed by avatar embodiment. This is called the ‘proteus effect’ (Peña et al., 2009; Banakou et al., 2013). While full-body embodiment allows for more experimental control, it may create an uncanny valley effect (Ratajczyk et al., 2019) or it can be a distractor itself. Kilteni et al. (2012) identified the ‘feeling of self-localization,’ ‘sense of agency,’ and the ‘sense of body ownership’ as the main influencing components of the illusion of virtual body ownership. Embodiment is additionally important for spatial perception. Mohler et al. (2010) showed an effect of viewing a self-avatar on distance judgments in an HMD-based virtual environment. Empirical findings in the cognitive neurosciences have also led to the hypothesis that time perception entails emotional and interoceptive states (Wittmann, 2013; Teghil et al., 2020). Accordingly, the perception of time is embodied, i.e., subjective time emerges through the existence of the bodily self across time as an enduring and embodied entity. This relation is prominently disclosed in altered states of consciousness, such as meditative states or with the flotation restricted environmental stimulation technique (flotation-REST) (Wittmann, 2015). These seem to be similar to flow states in more ordinary activities like sports or playing video games (Khoshnoud et al., 2020). These states are related to a decreased awareness of the self and a diminished awareness of time (Wittmann, 2015). Our research assumes and investigates a strong connection among the subjective experience of time, space, and the bodily self. We decided to add an embodiment condition with partial embodiment, i.e., visualization of the hands only, to test whether there is a difference in experience and to contribute to the latest findings (Lugrin et al., 2019; Unruh et al., 2021).
There now exists an extensive body of knowledge on time perception in the real physical world. Related work in the domain of VR has shown that it offers a suitable approach to manipulate time-perception-related effects. For example, virtual environments with adjusted parameters were effectively employed to alter the subjective experience of time by Kühn et al. (2018); depressed patients were successfully treated with video games. We followed a similar approach and developed an interactive virtual tunnel application to modify parameters known to evoke vection in users. The virtual tunnel simulates movements of visually distinguishable sections (objects) which form the tunnel. This generates the illusion of moving or flying through these objects comparable to a flight with a spaceship through a star field. The coordinated movements of these objects make users believe that it is not the objects that are moving relative to the observer, but that it is the user who is moving relative to the objects. We used this application to systematically modify the simulation parameters of the density and velocity of sections (objects) to change the perception of vection. See Figure 1 and Figure 2 for examples of the different tunnel section densities. We also investigated potential effects between remote desktop and an immersive VR exposure. The VR study provided an additional embodiment condition in which participants either had virtual hands or did not.
The following hypotheses were developed:
(H1) In high-density and fast-speed conditions, time passes more quickly.
(H2) In high-density and fast-speed conditions, the illusion of self-motion (vection) is more intensely perceived.
(H3) Time estimates, the passage of time and the illusion of self-motion (vection) differ between the embodiment (virtual hands and no virtual hands) conditions in virtual reality.
The Unreal Engine 4.27 Epic Games (2022) was used to develop the virtual-tunnel application used in the two presented studies. An experimenter interface was created to adjust experiment parameters, the virtual user, and the virtual-tunnel environment. The virtual-tunnel sections were placed 100 m in front of and behind the participant. The amount of tunnel sections placed within the 100 m was used to describe our independent variable density. For the low density condition (Figure 1), 5 brighter and five darker sections were placed sequentially to form the 100 m long tunnel. For the high density condition (Figure 2), 20 sections (10 darker, 10 lighter) were placed accordingly. For the slow speed condition, the tunnel sections moved at a speed of 200 cm/s. For the fast speed condition, the tunnel sections moved at a speed of 1000 cm/s. These parameters were previously investigated using a pilot study. The focus was on successfully creating the illusion of self-motion while still ensuring a high level of comfort for the participants. The participant was placed exactly in the middle from the beginning. The tunnel creates and deletes new sections when they are far enough away from the participant. The moving-tunnel sections simulate a tunnel moving towards the viewer. A visual representation can be seen in Figure 3. The entire environment was presented in a monochrome gray scale to avoid color as a possible confounding factor (Shibasaki and Masataka, 2014; Thönes et al., 2018; Bilgili et al., 2020). An end of the tunnel was implemented using a static block constantly placed 100 m away and served as a fixed point in the distance. The application was extensively tested for performance and usability. Benchmarks enabled us to ensure a consistent frame rate that matched the frame rates of the target devices. After the trial duration was reached, the view was faded out and in vivo questionnaires appeared. The application recorded answers from the questionnaires and automatically saved them after each trial. This application was used to evaluate the study parameters. It was also used to record the videos that were played to participants in the remote desktop study.
FIGURE 3. A view of the platform where participants were placed virtually when wearing the VR headset (the diameter was 12.73, 6.365 m from the center to the tunnel sides).
The remote desktop study tested the effects of the virtual tunnel, perceived on a computer monitor. Participants were exposed to videos with variations of a tunnel and then answered questions about their subjective experience of the video’s duration, the passage of time, and the feeling of self-motion (vection) (see Table 1). The tunnels had three variables: two different speeds at which the tunnel moved (fast and slow), three different durations (20, 30, and 40 s), and the spatial density of the sections that made up the tunnel (high density and low density; see Table 2).
The online study was designed with the LimeSurvey version 4.5.0 Schmitz and Nagel (2006). Custom functionality was put in using HTML and javascript code. We used the framework Jquery to enhance functionality. A start button was chosen to direct the focus to the center of the screen. Displaying the video in full-screen mode, as well as hiding and displaying the questions, was implemented using custom Javascript code. Results were exported from the university hosted LimeSurvey and analyzed using JASP v.0.14 and the programming language R.
For an overview of the dependent variables, see Table 1.
We implemented the Subjective Time, Self, and Space (STSS) questionnaire (Jokic et al., 2018) to assess the effects of the virtual tunnel, perceived on a computer monitor, on time experience and bodily perception. Two items from the STSS were adapted to assess the experience of the passage of time: 1) “Intuitively, without thinking about it, how long did the tunnel last?,”2) “How fast did time pass for you?” (based on Tobin et al. (2010)), and 3) “Did you feel like you yourself were moving?” to assess the intensity of the illusion of self-motion. The latter two questions were answered using a vertical streak on a horizontal line visual analogue scale (VAS) that ranged from 0 = extremely slowly to 100 = extremely fast on question two; and from 0 = no, not at all to 100 = yes, very intensely on question three.
Before and after the experiment participants were asked about how 1) tired they currently felt using a VAS ranging from 0 to 100. Boredom was assessed on a similar VAS only after the experiment had been completed.
Online access to the study was achieved through a participant recruitment system where students and external users could view the list and sign up. The desktop study consisted of 33 pages and a detailed structure can be found here: Table 3. Within the 33 pages there were either buttons, text inputs, or visual-analogue scales that participants had to answer. There was an automatic video playback embedded in the condition pages after which it disappeared, and questions for time-duration judgments, passage of time and vection appeared. This was tested on several browser and monitor configurations to ensure comparability.
Participants were recruited through an online university participant portal. On the welcome page, participants were greeted and informed about privacy and anonymity. Consent to the privacy policy was mandatory to proceed to the next page. The investigator’s contact address, general information, and the need for a fast internet connection were also mentioned. Participants were then asked general demographic questions about gender, age, education, and vision. This was followed by a separate section on gaming experience and optional follow-up questions when previous gaming experience was indicated. The next page contained general health questions about addiction and possible past experience with epileptic seizures. A question about the current fatigue level was included. On the following page, instructions were displayed to be read carefully, and turning to the next page was blocked for 20 s. Information was given about the virtual-tunnel videos and participants were requested to remove all distractions, such as watches or smartphones, and not to listen to music. The instruction was to focus on the videos and not to try to develop counting strategies. Since the subjects were watching multiple tunnel videos, we decided to tell them in advance that we were assessing their time experience and not their counting ability. At the end of the instructions, participants were requested to set their browser window to full screen. The first training session started on the following page. The training session was similar in its flow logic in each condition; a page with a button was loaded, and once the button was clicked, the tunnel video was played in maximum browser window size. After the video had played, it was hidden, and three questions were displayed (time estimate, time passage and vection). Training session 2 was displayed on the next page. After the completion of the two training sessions, participants were informed about the start of the actual study. All 12 conditions were displayed in random order. Each condition had the same sequence as described for the training videos. They differed only in the combination of independent variables corresponding to the conditions (duration, speed and density); see Table 2. After the completion of block A with all conditions, they were repeated in randomized order in block B. After completion of the last condition in block B, two final questions were asked about general fatigue and boredom level. The final page was then loaded, and participants were given the opportunity to leave a comment in a text box. The subjects were thanked and directed back to the participant recruitment portal.
We had a total of 137 respondents, of which five were excluded (two reported having an addiction, two used their mobile phones to do the online survey, and one took 50 min to finish; the mean completion time was 20.12 min, SD = 5.85). The final sample was N = 132. 100 men and 32 women with an average age of 25.2 years participated in this study.
We conducted repeated-measures ANOVAs to test which effects the independent variables of density, speed, and duration had on the estimation of duration, the subjective passage of time, and vection. Time-duration estimations, passage of time and vection ratings were analyzed using the interval measurement scale (Pfeifer et al., 2016; Jokic et al., 2018). Normality was tested with the Shapiro-Wilk Test. The assumption of sphericity was checked using the Mauchly test, and sphericity corrections were made with the Greenhouse-Geisser correction when necessary. We used JASP v.0.16.4 (JASP Team, 2022) for the analyses. We conducted Pearson’s correlations between all three dependent variables of duration estimation, passage of time, and vection for each tunnel condition.
We conducted t-tests comparing duration estimation, passage of time, and vection between block A (first pass of the 12 conditions) and block B (second pass of the same 12 conditions). For a full description of the conditions, see Table 2. We found no significant differences between the two blocks for any of the above-mentioned variables in any of the conditions. This shows that there was no significant effect of fatigue or learning in the participants’ responses. Therefore, we averaged each of the 12 conditions in block A with their corresponding conditions in block B and conducted the following analyses with the mean values.
For this dependent variable variance homogeneity, but not normality, were assumed. Although the Anova is quite robust to violations of normality, we still performed it with log transformed duration estimates. We used the transformed time estimates in the following tests. With respect to the dependent variable of duration estimation (judgement in seconds), the duration of the tunnel (F1.6,209.5 = 497.53, p < .001) had a significant effect. The density and speed of tunnel segments had no significant effect.
The dependent variable of time passage was significantly affected by all three independent variables, density (F1,128 = 54.56, p < .001), speed (F1,128 = 243.73, p < .001), and duration (F2,256 = 113.13, p < .001). There was also a significant interaction between density and speed (F1,128 = 9.76, p = .002). A post hoc analysis showed that the combination of high density and fast speed had the strongest effect of all on the subjective passage of time.
Considering vection, the main effects of density (F1,128 = 32.6, p < .001) and speed (F1,128 = 142.6, p < .001) were significant. There was also a significant interaction between speed and duration (F2,256 = 3.05, p = .049).
We found that passage of time and vection correlated highly and positively in each condition. The faster the subjective passage of time, the stronger the feeling of vection and vice versa. A detailed overview of the correlations between vection and passage of time can be found here Table 4. Duration estimation and vection did not correlate under any condition.
We gave clear instructions on how to use the browser in full-screen mode after the demographic questionnaire and the previous assessments of fatigue and boredom. We also requested participants to provide feedback on whether they managed to use the browser in the full-screen mode. We instructed participants at the outset not to use mobile devices, to keep all distracting elements away from the computer screen, and to focus on the tunnel as best they could. Potential distractions could not be completely ruled out and remain as a limitation for this study design. The size of the screens and the distances participants sat in front of their screens could not be recorded.
In study 2, the virtual environment was extended so that it could be viewed on a VR headset. This series of experiments was conducted as laboratory controlled study on site (for an example see Figure 4). The experimental setup included the between-group virtual hands vs. no virtual hands conditions. Participants were assigned to either the virtual hands or no virtual hands group. For the virtual hands condition, virtual hands were added to the participants’ viewpoint and replicated the movements of the participants’ hands while holding the VR controllers. This was included to examine the influence of embodiment in the VR environment and its subsequent effects on the independent variables.
FIGURE 4. The lab where the VR study was conducted; the table and chair were matched in size and location in VR. This example shows the condition with partial embodiment (virtual hands). The participant view is mirrored on the monitor on the right.
Participants experienced the VR environment with the Oculus Rift S head-mounted display (HMD) with a resolution of 1280 × 1440 pixels per eye and an 80 Hz refresh rate. Two controllers were employed to respond to presented questions following a trial. The PC specifications were as follows: Intel i7-9700K processor, 16 GB RAM, GeForce RTX 2080Ti.
The measurements in Study 2 differed only by the addition of the following questionnaires: Simulator Sickness Questionnaire (SSQ) and Igroup Presence Questionnaire (IPQ).
Because the illusion of self-movement is considered to be a main contributor to simulator sickness or motion sickness, we attempted to assess possible negative effects of the VR simulation with the Simulator Sickness Questionnaire (SSQ) (Kennedy et al., 1993). It was evaluated before and after the experiment. This was done to assess the comfort of the participants, which may have influenced the results. Because participants were seated the entire time during the experiment and were provided with a visual reference of the floor, table, and chair that also corresponded to their real-world counterparts, we expected the effects of visually induced motion sickness to be attenuated (Cao et al., 2018). Chang et al. (2020) pointed out in a review that greater discomfort was present with rotational movements compared to translational movements. Because the visual display contained only constant-velocity motion, there should be little or no sustained conflict between the visual and vestibular inputs (Keshavarz et al., 2015).
We also evaluated the feeling of presence, or how present participants really felt being inside the virtual environment, with the Igroup Presence Questionnaire (IPQ). Presence and embodiment correlated with each other, so we validated the ratings for the embodiment conditions (Schubert et al., 2001; Regenbrecht and Schubert, 2002). Especially the comparison between the conditions virtual hands and no virtual hands could contribute to interesting insights. The IPQ’s subscales were: 1) General Presence, 2) Spatial Presence, 3) Involvement, and 4) Experienced Realism. A seven-point Likert Scale was also used for the item assessment.
The tunnel generator supported additional parameters for the VR simulation: a virtual floor, a virtual table, and a virtual chair. Participants were asked to leave their controllers with their hands on the real table to match the position of the objects in the real world. The application was responsible for setting the appropriate values for the positions of the virtual table, chair, and floor. A physical table and chair were present in the experimental room, to provide a haptic experience corresponding to the visual one. The virtual representations of the floor, chair, and the table served dual purposes. One was to generate haptic feedback, which was expected to increase presence even if no virtual avatar was present. It also served as a frame of reference to keep portions of the virtual environment as a reference to the user’s position and to provide contrast to the moving tunnel. This and the participant’s seated position were expected to reduce discomfort due to visually induced motion sickness (Cao et al., 2018).
Participants were informed about the details of the study and asked to sign an informed consent form. A demographic questionnaire and a questionnaire to assess their current wellbeing were given the subjects to determine whether they were appropriate to participate in the experiment. Participants were then asked to complete the SSQ. The experimenter explained the basics of the experiment as well as the VR headset and how the controller devices worked. Participants were seated at a table and asked to perform the following tasks and report any problems aloud: 1) move their head to visually explore the virtual environment, 2) move their hands, 3) touch the virtual desk with their hands. They were then shown two training sessions consisting of fixed variables that were different from the variables used in the study. This acclimated the subjects to the virtual environment and showed them how to answer the questions that appeared at the end of each trial. These were the same as in the actual run. The variables allowed for a combination of 12 different runs. See Table 2 for the configurations set, and Figure 5 for an overview of the procedure. The trials were randomized at the beginning of the experimental series for 100 participants using a pseudo-randomizer (Haahr, 1998) to prevent an unintended influence of a particular combination on the subjective experience of time. It was thus completely up to chance which set of trials was presented to the participants. Participants were asked to complete the SSQ again after the experimental session, as well as the IPQ and the boredom rating for the first time. This completed the study, and participants were dismissed.
Forty-three participants between the ages of 18 and 36 years were recruited. One participant was excluded in the final analysis due to a screening error. The final participant count was n = 42. 22 women and 20 men with an average age of 26.6 years. The vast majority were students from the University, recruited via an online platform, flyers, word-of-mouth, and other studies at the Institute. Prospective participants were screened over the telephone to determine if they fulfilled the requirements to take part in the experiment (sex, age, eyesight, hearing, educational status, mental health, and medication, as well as their gaming experience). In accordance with the changing regulations during the COVID-19 pandemic, participants completed a verbal checklist of symptoms and presented a negative antigen test or vaccination certificate the day of the trial. Each participant was given ten Euros for their participation. This study was approved by the local Ethics Committee of the Institute.
The analyses were conducted with the programming language R using RStudio as development environment. Time-duration estimations, passage of time and vection ratings were analyzed using the interval measurement scale (Pfeifer et al., 2016; Jokic et al., 2018). We applied three within-subject variables: density, speed, and duration, as well as one between-subject variable: virtual hands. We conducted a 4-way mixed ANOVA each to investigate the effects and interactions of the four independent variables on the three dependent variables, time-duration estimation, passage of time, and vection. Normality was tested with the Shapiro-Wilk Test and variance homogeneity, with the Levene Test. The assumption of sphericity was checked using the Mauchly test, and sphericity corrections were made when necessary. Independent t-tests were performed for the IPQ items. Normality assumption was checked with the Shapiro-Wilk test. Variance homogeneity was checked using the F-test. The A total score and the following subscales were calculated for the SSQ: Nausea, Ocular Motor Activity, and Disorientation. The means of the total score and of the subscales were used to interpret symptom severity and the source of potential problems.
We conducted Pearson’s correlations between all three dependent variables of duration estimation, passage of time, and vection for each tunnel condition.
Similar to the analysis of Study 1, we compared blocks A and B with a t-test (within-variables overview see Table 2). We found no significant differences between the two blocks. This indicates that there appeared to be no significant effect of fatigue or learning in the participants’ responses. We then averaged each of the 12 conditions in block A with their corresponding conditions in block B and conducted the analyses with the mean values.
For the dependent variable of the duration estimate (assessment in seconds), variance homogeneity, but not normality, were assumed. We applied a logarithmic-data-transformation approach for the duration estimates because heteroscedasticity was not an issue (Manning, 1998). We used the transformed time estimates in the following tests. We found significant effects for the duration (F (2, 80) = 168.328, p < .0001) and speed (F (1, 40) = 10.352, p < .01) of the tunnel. We also found a significant two-way interaction between speed and density (F (1, 40) = 6.289, p = .016). Time estimations being consistently judged at about half the actual duration of the trial (Trial20sec: M = 11.9, sd = 5.6; Trial30sec: M = 14, sd = 6.6; Trial40sec: M = 17, sd = 8).
The mixed ANOVA results yielded a strong statistical significance for all three within-subjects variables: duration (F1.73,69.01 = 27.62, p < .001), density (F1,40 = 23.12, p < .001), and speed (F1,40 = 98.6, p < .001). The effects of the dependent variables are shown in Figure 6. There was a clear difference in the response to speed; the reported passage of time was higher at faster speeds (Speedslow: M = 49.5, sd = 11.4, Speedfast: M = 58, sd = 12.3). We additionally found a significant two-way interaction between density and duration at (F2,80 = 3.63, p = .031) and a significant three-way interaction among virtual hands, speed and duration at (F2,80 = 3.43, p = .037).
For the variable vection, the mixed ANOVA reported a strong statistical significance in the variable speed (F1,40 = 20.91, p < .001). The effects of the dependent variable can be clearly seen in Figure 7, where the lower reported illusion of self-movement at slower speeds can be detected. We also found a significant two-way interaction between speed and density at (F1,40 = 4.55, p = .039). A post hoc pairwise comparison t-test (Bonferroni adjusted) revealed consistently low densities that were perceived in the embodiment condition (visualized with two virtual hands) and regardless of time durations, vection was perceived to be significantly different between fast and slow speeds. Fast speeds resulted in significantly higher illusions of self-movement with virtual hands and exposed to low densities.
In most of the tunnel conditions we found significant correlations between passage of time and vection ratings, see Table 5.
Normality and variance homogeneity could not be assumed for the SSQ scores. We therefore chose the Wilcoxon Rank Sum Test to analyze whether there was a significant difference between the scores before and after VR exposure. There was a significant difference between the SSQ total score and all subscales of nausea, disorientation, and oculomotor. The SSQ total pre score (M = 5.25, sd = 5.29) was significantly different from the SSQ total post score (M = 16.8, sd = 14.53). According to a meta-analysis by Saredakis et al. (2020), the SSQ values for VR experiments of 55 reviewed papers were categorized into content type, detail, interactions, sensory feedback, and environment. The overall range of SSQ mean scores ranged from 14.30 to 35.27. The SSQ total scores reported here can thus be considered low. Notably, because VR applications in which optical flow was involved and exposure time was greater than 10 min had higher mean SSQ scores (Saredakis et al., 2020). No participant complained or asked for a break during or after the experiment. There were no participant dropouts.
Normality and variance homogeneity could be assumed for all IPQ items: involvement, spatial presence, experienced realism and general presence. Independent t-tests were performed and no significant difference could be found between the embodied and the not-embodied group.
The limitation that vection promotes motion sickness symptoms has already been pointed out, but we found comparatively low levels of severity. We still consider it important to mention this as a possible limitation, even if it is considered minor. The difference between the virtual hands conditions was too small. The two studies may not have been perceived in exactly the same way. In the VR study, the virtual environment was enriched by a virtual floor, table, and chair, which were not present in the online study. The tunnel application used was identical; the same parameters were chosen, and participants were able to experience the same virtual tunnel despite the different viewpoint sizes and distances. The chair and table were added to increase user comfort and make the setup more plausible and comparable to the online study.
As anticipated, the actual duration of the tunnel had a strong influence on the subsequent estimated duration. Longer durations led to longer estimations in both studies. This is unsurprising, but it serves to confirm the validity of the results. For the VR study only, fast speeds also led to longer estimations. In addition, we found an interaction between speed and density for the VR study. The spatial markers in the virtual environment seem to be emphasized in VR, which leads to the assumption that the availability of depth information plays an important role when estimating time retrospectively. This seems to be irrelevant for the remote desktop study, as a monitor does not present the degree of depth information that VR technology does. In both studies participants experienced a faster subjective passage of time for shorter durations, faster speeds, and higher densities. Therefore, the passage of time was significantly and strongly affected by fast speed and high density. This leads to the acceptance of H1. Under high-density and fast-speed conditions, the passage of time is perceived as faster. In study 1, we found a significant interaction between speed and density, whereas in study 2 we found a significant interaction between duration and density. These are interesting results that require further research. As of now, we can only speculate about their meaning. One possibility is that the frequency of movement on a computer monitor contributes more to the subjective feeling of the passage of time in comparison to VR, where the exposure time and the amount of spatial markers seem to play a bigger role. In study 1, density and speed had significant effects on vection. Vection was reported to be more intense in fast-speed conditions than in slow-speed conditions and in high-density conditions than in low-density conditions. In study 2, only speed had a significant effect on vection. In VR, vection was reported to be more intense in fast-speed conditions than in slow-speed conditions. H2 can be accepted because high density and fast speed had significant effects on vection. We also found significant positive correlations between vection and the passage of time in both studies. We found this correlation in every tunnel condition of study 1 and in nearly all tunnel conditions of study 2 (excluding conditions: 01, 02, 10, see Table 5). There appears to be a linear relationship between vection and the passage of time that should be further investigated. Speed had significant effects on both vection and passage of time ratings and might contribute to this correlation. Virtual reality appears to be superior for experiencing the illusion of self-motion, which is consistent with previous results. A larger field of view, depth information, and user ability to dynamically adjust the view with head movements seemed to have promoted this illusion. Since not only the estimation of duration (underestimation), which has been demonstrated in previous work, but also the passage of time (an acceleration) was significantly affected, this could be called the illusion of time compression. In the VR study, estimated times were shorter than those estimated in the online study and, on average, trends showed an underestimation of nearly 50% compared to the actual time elapsed. The single item boredom, which was queried following both studies, also showed a drastic difference between the two studies - a mean value of 74.8 in the desktop study compared to 41.1 in VR. From this one could further speculate about a connection between passage of time, boredom, and vection. In particular, the observed interplay between the experienced passage of time and vection constitutes a novel contribution to the study of self-motion. We plan to explore this connection further. Concerning embodiment, we only found partial significant differences between the virtual hand conditions in the VR study. For the dependent variable passage of time, a significant three-way interaction among virtual hands, speed, and duration was found. In addition, fast velocities led to a significantly more intense illusion of self-motion when participants had virtual hands and were exposed to a low density tunnel. For this reason, we cannot fully accept H3, as these were ‘partial’ effects between the virtual hands conditions. Still, if further research supports this, it could be an interesting contribution to our knowledge about motion perception, time perception, and body perception. A possible interplay of spatial markers in relation to visual body markers could enhance the strength of the illusion of self-motion, because it seemed more coherent for participants. An explanation why this occurred only for low densities could be that our high density condition was probably less distinguishable in terms of perceiving single moving markers and their speed in relation to the virtual hands. According to Unruh et al. (2021), having a virtual body in their VR waiting-room setup made a difference. The waiting situation was very different from the situation participants experienced in our study. This could explain the different effects. Since the waiting room study compared no virtual body to a full virtual-body representation, it could be that virtual hands are not sufficient to reveal similar pronounced differences for the perceived passage of time and time estimation. Kanai et al. (2006) suggested that “…the temporal frequency of a stimulus serves as a ‘clock’ for perceived duration.” Our results show similar relationships between the density of spatial markers and their speed, and support the concept that the internal clock that controls time perception has its basis in early stages of processing. Moreover, the presence of virtual body parts also seem to have a subtle influence on the perception of the passage of time and the illusion of self-motion.
Overall, the virtual tunnel appeared to be well suited to manipulate experienced time. In a direct manipulation approach, a combination of the density of spatial markers and the speed at which they moved seemed to enhance virtual time (illusions); this was particularly evident in the VR study. Sufficient information about this relationship may enable us to automatically adjust the passage of time for observers in the future. For future therapeutic approaches, the combinations of comfortable densities and speeds need to be further investigated in relation to virtual time illusions. If the intensity of the experienced self-motion can be controlled, we can also imagine controlling the perceived passage of time. This may prove particularly useful for time-based VR therapies in the future. Linking perceived velocity to perceived time and self-motion is important for verifying and using objective measures such as eye-tracking and posture data in VR. Virtual whole-body representations should also be evaluated in a next step. Especially in combination with an interactive task, time perception could be influenced even more.
The raw data supporting the conclusion of this article will be made available by the authors, without undue reservation.
The studies involving human participants were reviewed and approved by the local Ethics Committee of the Institute for Frontier Areas of Psychology and Mental Health (IGPP_2021_03). The patients/participants provided their written informed consent to participate in this study.
ML and FA share first authorship. ML, FA, FU, SK, MW, J-LL, and MEL contributed to the conception and design of the study. ML and FU developed the software. ML, FA, HH, and SK recruited participants and acquired the data. ML and HH performed the statistical analyses. ML wrote the first draft of the manuscript. J-LL, MW, and MEL were the project administrators and supervised the work. All authors contributed to manuscript revision, read, and approved the submitted version.
This work is funded by the VIRTUALTIMES project (ID-824128) funded by the European Union under the Horizon 2020 program. This publication was supported by the Open Access Publication Fund of the University of Wuerzburg.
The authors wish to thank Moritz Pischel and Lena Mohnke for help and data acquisition.
The authors declare that the research was conducted in the absence of any commercial or financial relationships that could be construed as a potential conflict of interest.
All claims expressed in this article are solely those of the authors and do not necessarily represent those of their affiliated organizations, or those of the publisher, the editors and the reviewers. Any product that may be evaluated in this article, or claim that may be made by its manufacturer, is not guaranteed or endorsed by the publisher.
The Supplementary Material for this article can be found online at: https://www.frontiersin.org/articles/10.3389/frvir.2022.1059971/full#supplementary-material
Abe, S. (1935). Experimental study of the corelation between time and space. Tohoku Psychol. Folia 3, 53–68.
Achenbach, J., Waltemate, T., Latoschik, M. E., and Botsch, M. (2017). Fast generation of realistic virtual humans proceedings of the 23rd ACM symposium on virtual reality software and technology. ACM 12, 1. doi:10.1145/3139131.3139154
Allman, M. J. (2015). Abnormal timing and time perception in autism spectrum disorder? A review of the evidence. Time distortions mind, 37–56. doi:10.1163/9789004230699_003
Alvarez Igarzábal, F., Hruby, H., Witowska, J., Khoshnoud, S., and Wittmann, M. (2021). What happens while waiting in virtual reality? A comparison between a virtual and a real waiting situation concerning boredom, self-regulation, and the experience of time. Technol. Mind, Behav. 2. doi:10.1037/tmb0000038
Banakou, D., Groten, R., and Slater, M. (2013). Illusory ownership of a virtual child body causes overestimation of object sizes and implicit attitude changes. Proc. Natl. Acad. Sci. 110, 12846–12851. doi:10.1073/pnas.1306779110
Bansal, A., Weech, S., and Barnett-Cowan, M. (2019). Movement-contingent time flow in virtual reality causes temporal recalibration. Sci. Rep. 9, 4378–4413. doi:10.1038/s41598-019-40870-6
Benussi, V., and Winter, C. (1913). Psychologie der zeitauffassung. Heidelberg, Germany: C. Winter, 6.
Bilgili, B., Ozkul, E., and Koc, E. (2020). The influence of colour of lighting on customers’ waiting time perceptions. Total Qual. Manag. Bus. Excell. 31, 1098–1111. doi:10.1080/14783363.2018.1465814
Brandt, T., Dichgans, J., and Koenig, E. (1973). Differential effects of central versus peripheral vision on egocentric and exocentric motion perception. Exp. Brain Res. 16, 476–491. doi:10.1007/bf00234474
Brown, S. W. (1995). Time, change, and motion: The effects of stimulus movement on temporal perception. Percept. Psychophys. 57, 105–116. doi:10.3758/bf03211853
Bruder, G., and Steinicke, F. (2014). “Time perception during walking in virtual environments,” in 2014 IEEE Virtual Reality, 2014 IEEE Virtual Reality, March 29–April 02, 2014 IEEE, 67. doi:10.1109/VR.2014.6802054
Bryson, S. (2013). Virtual reality: A definition history-a personal essay. arXiv preprint arXiv:1312.4322.
Caelli, T., Flanagan, P., and Green, S. (1982). On the limits of perceptual complementarity in the kinetic depth effect. Percept. Psychophys. 31, 437–445. doi:10.3758/bf03204853
Cao, Z., Jerald, J., and Kopper, R. (2018). “Visually-induced motion sickness reduction via static and dynamic rest frames,” in IEEE conference on virtual reality and 3D user interfaces (VR), Germany, 18-22 March 2018 (IEEE), 105.
Chang, E., Kim, H. T., and Yoo, B. (2020). Virtual reality sickness: A review of causes and measurements. Int. J. Human–Computer Interact. 36, 1658–1682. doi:10.1080/10447318.2020.1778351
Cohen, J., Hansel, C. E. M., and Sylvester, J. D. (1953). A new phenomenon in time judgment. Nature 172, 901. doi:10.1038/172901a0
Dichgans, J., and Brandt, T. (1978). Visual-vestibular interaction: Effects on self-motion perception and postural control. Perception 8, 755–804. doi:10.1007/978-3-642-46354-9_25
Epic Games (2022). Unreal engine. Available at: https://www.unrealengine.com.
Fischer, M. H., and Wodak, E. (1924). Beiträge zur physiologie des menschlichen vestibularapparates. Pflüger’s Arch. für gesamte Physiol. Menschen Tiere 202, 523–552. doi:10.1007/bf01723519
Friedman, D., Pizarro, R., Or-Berkers, K., Neyret, S., Pan, X., and Slater, M. (2014). A method for generating an illusion of backwards time travel using immersive virtual reality-an exploratory study. Front. Psychol. 5, 943. doi:10.3389/fpsyg.2014.00943
Gall, D., and Latoschik, M. E. (2018). The effect of haptic prediction accuracy on presence, IEEE Conference on Virtual Reality and 3D User Interfaces, Germany, 18-22 March 2018, IEEE, 73–80.
Goldstone, S., and Lhamon, W. T. (1974). Studies of auditory-visual differences in human time judgment: 1. Sounds are judged longer than lights. Percept. Mot. Ski. 39, 63–82. doi:10.2466/pms.1974.39.1.63
Haggard, P. (2017). Sense of agency in the human brain. Nat. Rev. Neurosci. 18, 196–207. doi:10.1038/nrn.2017.14
Helson, H., and King, S. M. (1931). The tau effect: An example of psychological relativity. J. Exp. Psychol. 14, 202–217. doi:10.1037/h0071164
Jokic, T., Zakay, D., and Wittmann, M. (2018). Individual differences in self-rated impulsivity modulate the estimation of time in a real waiting situation. Timing & Time Percept. 6, 71–89. doi:10.1163/22134468-00002101
Kanai, R., Paffen, C. L., Hogendoorn, H., and Verstraten, F. A. (2006). Time dilation in dynamic visual display. J. Vis. 6, 8. doi:10.1167/6.12.8
Kaneko, S., and Murakami, I. (2009). Perceived duration of visual motion increases with speed. J. Vis. 9, 14. doi:10.1167/9.7.14
Kennedy, R. S., Lane, N. E., Berbaum, K. S., and Lilienthal, M. G. (1993). Simulator sickness questionnaire: An enhanced method for quantifying simulator sickness. Int. J. Aviat. Psychol. 3, 203–220. doi:10.1207/s15327108ijap0303_3
Keshavarz, B., Riecke, B. E., Hettinger, L. J., and Campos, J. L. (2015). Vection and visually induced motion sickness: How are they related? Front. Psychol. 6, 472. doi:10.3389/fpsyg.2015.00472
Khoshnoud, S., Alvarez Igarzábal, F., and Wittmann, M. (2020). Peripheral-physiological and neural correlates of the flow experience while playing video games: A comprehensive review. PeerJ 8, e10520. doi:10.7717/peerj.10520
Kilteni, K., Groten, R., and Slater, M. (2012). The sense of embodiment in virtual reality. Presence Teleoperators Virtual Environ. 21, 373–387. doi:10.1162/pres_a_00124
Kitajima, M., Kanari, K., and Sato, M. (2020). “An examination of effects of fear using vr content on time estimation,” in 26th ACM Symposium on Virtual Reality Software and Technology, Virtual Event, Canada, November 1–November 4, 2020, 1–2. doi:10.1145/3385956.3422104
Kühn, S., Berna, F., Lüdtke, T., Gallinat, J., and Moritz, S. (2018). Fighting depression: Action video game play may reduce rumination and increase subjective and objective cognition in depressed patients. Front. Psychol. 9, 129. doi:10.3389/fpsyg.2018.00129
Landau, D. H., Hasler, B. S., and Friedman, D. (2020). Virtual embodiment using 180° stereoscopic video. Front. Psychol. 11, 1229. doi:10.3389/fpsyg.2020.01229
Landeck, M., Unruh, F., Lugrin, J.-L., and Latoschik, M. E. (2020). “Metachron: A framework for time perception research in vr,” in 26th ACM Symposium on Virtual Reality Software and Technology, Virtual Event, Canada, November 1–November 4, 2020 (New York, NY, USA: Association for Computing Machinery). VRST ’20. doi:10.1145/3385956.3422111
Latoschik, M. E., Roth, D., Gall, D., Achenbach, J., Waltemate, T., and Botsch, M. (2017). “The effect of avatar realism in immersive social virtual realities,” in Proceedings of the 23rd ACM Symposium on Virtual Reality Software and Technology, Gothenburg, Sweden, November 8–November 10, 2017 (ACM), 39. doi:10.1145/3139131.3139156
Latoschik, M. E., and Wienrich, C. (2022). Congruence and plausibility, not presence?! pivotal conditions for xr experiences and effects, a novel model. provisionally Accept. 2022, 1. doi:10.3389/frvir.2022.694433
Lelyveld, P., and Entertainment, U. (2015). Virtual reality primer with an emphasis on camera-captured vr. SMPTE Motion Imaging J. 124, 78–85. doi:10.5594/j18599
Lugrin, J.-L., Unruh, F., Landeck, M., Lamour, Y., Latoschik, M. E., Vogeley, K., et al. (2019). “Experiencing waiting time in virtual reality,” in 25th ACM symposium on virtual reality software and technology, Parramatta, NSW, Australia, November 12–November 15, 2019, 1–2.
Malpica, S., Masia, B., Herman, L., Wetzstein, G., Eagleman, D. M., Gutierrez, D., et al. (2022). Larger visual changes compress time: The inverted effect of asemantic visual features on interval time perception. PloS one 17, e0265591. doi:10.1371/journal.pone.0265591
Manning, W. G. (1998). The logged dependent variable, heteroscedasticity, and the retransformation problem. J. Health Econ. 17, 283–295. doi:10.1016/S0167-6296(98)00025-3
Martin, B., Franck, N., and Giersch, A. (2019). A reflection upon methods to explore timing in patients with schizophrenia. PsyCh J. 8, 82–89. doi:10.1002/pchj.268
Mohler, B. J., Creem-Regehr, S. H., Thompson, W. B., and Bülthoff, H. H. (2010). The effect of viewing a self-avatar on distance judgments in an hmd-based virtual environment. Presence 19, 230–242. doi:10.1162/pres.19.3.230
Palmisano, S., Allison, R. S., Schira, M. M., and Barry, R. J. (2015). Future challenges for vection research: Definitions, functional significance, measures, and neural bases. Front. Psychol. 6, 193. doi:10.3389/fpsyg.2015.00193
Peña, J., Hancock, J. T., and Merola, N. A. (2009). The priming effects of avatars in virtual settings. Commun. Res. 36, 838–856. doi:10.1177/0093650209346802
Pfeifer, E., Sarikaya, A., and Wittmann, M. (2016). Changes in states of consciousness during a period of silence after a session of depth relaxation music therapy (drmt). Music Med. 8, 180–186. doi:10.47513/mmd.v8i4.473
Pizarro, R., Berkers, K.-O., Slater, M., and Friedman, D. (2015). How to time travel in highly immersive virtual reality. ICAT-EGVE, 117–124. doi:10.5555/2852313.2852329
Ratajczyk, D., Jukiewicz, M., and Lupkowski, P. (2019). Evaluation of the uncanny valley hypothesis based on declared emotional response and psychophysiological reaction. Bio-Algorithms Med-Systems 15. doi:10.1515/bams-2019-0008
Regenbrecht, H., and Schubert, T. (2002). Real and illusory interactions enhance presence in virtual environments. Presence Teleoperators Virtual Environ. 11, 425–434. doi:10.1162/105474602760204318
Riecke, B. E., and Feuereissen, D. (2012). “To move or not to move: Can active control and user-driven motion cueing enhance self-motion perception (”vection”) in virtual reality?,” in Proceedings of the ACM Symposium on Applied Perception, Los Angeles, August 2012 (New York, NY, USA: Association for Computing Machinery), 17–24. doi:10.1145/2338676.2338680
Roelofs, C. O., and Zeeman, W. (1951). Influence of different sequences of optical stimuli on the estimation of duration of a given interval of time. Acta Psychol. 8, 89–128. doi:10.1016/0001-6918(51)90007-8
Rutrecht, H., Wittmann, M., Khoshnoud, S., and Alvarez Igarzábal, F. (2021). Time speeds up during flow states: A study in virtual reality with the video game thumper. Timing & Time Percept. 9, 353–376. doi:10.1163/22134468-bja10033
Sanchez-Vives, M. V., and Slater, M. (2005). From presence to consciousness through virtual reality. Nat. Rev. Neurosci. 6, 332–339. doi:10.1038/nrn1651
Saredakis, D., Szpak, A., Birckhead, B., Keage, H. A., Rizzo, A., and Loetscher, T. (2020). Factors associated with virtual reality sickness in head-mounted displays: A systematic review and meta-analysis. Front. Hum. Neurosci. 14, 96. doi:10.3389/fnhum.2020.00096
Schatzschneider, C., Bruder, G., and Steinicke, F. (2016). Who turned the clock? Effects of manipulated zeitgebers, cognitive load and immersion on time estimation. IEEE Trans. Vis. Comput. Graph. 22, 1387–1395. doi:10.1109/tvcg.2016.2518137
Schneider, S. M., and Hood, L. E. (2007). Virtual reality: A distraction intervention for chemotherapy. Oncol. Nurs. forum (NIH Public Access. 34, 39–46. doi:10.1188/07.onf.39-46
Schneider, S. M., Kisby, C. K., and Flint, E. P. (2011). Effect of virtual reality on time perception in patients receiving chemotherapy. Support. Care Cancer 19, 555–564. doi:10.1007/s00520-010-0852-7
Schubert, T., Friedmann, F., and Regenbrecht, H. (2001). The experience of presence: Factor analytic insights. Presence Teleoperators Virtual Environ. 10, 266–281. doi:10.1162/105474601300343603
Seno, T., Ito, H., and Sunaga, S. (2011). Self-motion perception compresses time experienced in return travel. Perception 40, 497–499. doi:10.1068/p6885
Seno, T., Murata, K., Fujii, Y., Kanaya, H., Ogawa, M., Tokunaga, K., et al. (2018). Vection is enhanced by increased exposure to optic flow. i-Perception 9, 204166951877406. doi:10.1177/2041669518774069
Shibasaki, M., and Masataka, N. (2014). The color red distorts time perception for men, but not for women. Sci. Rep. 4, 5899. doi:10.1038/srep05899
Slater, M. (2009). Place illusion and plausibility can lead to realistic behaviour in immersive virtual environments. Philosophical Trans. R. Soc. B Biol. Sci. 364, 3549–3557. doi:10.1098/rstb.2009.0138
Slater, M., and Steed, A. (2000). A virtual presence counter. Presence Teleoperators Virtual Environ. 9, 413–434. doi:10.1162/105474600566925
Spanlang, B., Normand, J.-M., Borland, D., Kilteni, K., Giannopoulos, E., Pomés, A., et al. (2014). How to build an embodiment lab: Achieving body representation illusions in virtual reality. Front. Robotics AI 1, 9. doi:10.3389/frobt.2014.00009
Steuer, J. (1992). Defining virtual reality: Dimensions determining telepresence. J. Commun. 42, 73–93. doi:10.1111/j.1460-2466.1992.tb00812.x
Teghil, A., Boccia, M., Nocera, L., Pietranelli, V., and Guariglia, C. (2020). Interoceptive awareness selectively predicts timing accuracy in irregular contexts. Behav. Brain Res. 377, 112242. doi:10.1016/j.bbr.2019.112242
Thönes, S., Von Castell, C., Iflinger, J., and Oberfeld, D. (2018). Color and time perception: Evidence for temporal overestimation of blue stimuli. Sci. Rep. 8, 1688–8. doi:10.1038/s41598-018-19892-z
Tobin, S., Bisson, N., and Grondin, S. (2010). An ecological approach to prospective and retrospective timing of long durations: A study involving gamers. PloS one 5, e9271. doi:10.1371/journal.pone.0009271
Unruh, F., Landeck, M., Oberdörfer, S., Lugrin, J.-L., and Latoschik, M. E. (2021). Vr time-based psychosis therapy: The influence of avatar embodiment on time perception. Front. Virtual Real. 2, 71. doi:10.3389/frvir.2021.658509
Waltemate, T., Gall, D., Roth, D., Botsch, M., and Latoschik, M. E. (2018). The impact of avatar personalization and immersion on virtual body ownership, presence, and emotional response. IEEE Trans. Vis. Comput. Graph. 24, 1643–1652. doi:10.1109/tvcg.2018.2794629
Weber, S., Weibel, D., and Mast, F. W. (2020). How self-motion in virtual reality affects the subjective perception of time. Timing & Time Percept. 8, 119–136. doi:10.1163/22134468-20191152
Wittmann, M. (2015). Modulations of the experience of self and time. Conscious. Cognition 38, 172–181. doi:10.1016/j.concog.2015.06.008
Wittmann, M. (2013). The inner sense of time: How the brain creates a representation of duration. Nat. Rev. Neurosci. 14, 217–223. doi:10.1038/nrn3452
Zakay, D., and Block, R. A. (1997). Temporal cognition. Curr. Dir. Psychol. Sci. 6, 12–16. doi:10.1111/1467-8721.ep11512604
Keywords: passage of time, illusion of self-motion, vection, virtual tunnel, therapeutic application, virtual reality, extended reality (XR)
Citation: Landeck M, Alvarez Igarzábal F, Unruh F, Habenicht H, Khoshnoud S, Wittmann M, Lugrin J-L and Latoschik ME (2023) Journey through a virtual tunnel: Simulated motion and its effects on the experience of time. Front. Virtual Real. 3:1059971. doi: 10.3389/frvir.2022.1059971
Received: 04 October 2022; Accepted: 19 December 2022;
Published: 09 January 2023.
Edited by:
Maria Limniou, University of Liverpool, United KingdomReviewed by:
Bruno Herbelin, Swiss Federal Institute of Technology Lausanne, SwitzerlandCopyright © 2023 Landeck, Alvarez Igarzábal, Unruh, Habenicht, Khoshnoud, Wittmann, Lugrin and Latoschik. This is an open-access article distributed under the terms of the Creative Commons Attribution License (CC BY). The use, distribution or reproduction in other forums is permitted, provided the original author(s) and the copyright owner(s) are credited and that the original publication in this journal is cited, in accordance with accepted academic practice. No use, distribution or reproduction is permitted which does not comply with these terms.
*Correspondence: Maximilian Landeck, bWF4aW1pbGlhbi5sYW5kZWNrQHVuaS13dWVyemJ1cmcuZGU=
†These authors have contributed equally to this work and share first authorship
Disclaimer: All claims expressed in this article are solely those of the authors and do not necessarily represent those of their affiliated organizations, or those of the publisher, the editors and the reviewers. Any product that may be evaluated in this article or claim that may be made by its manufacturer is not guaranteed or endorsed by the publisher.
Research integrity at Frontiers
Learn more about the work of our research integrity team to safeguard the quality of each article we publish.