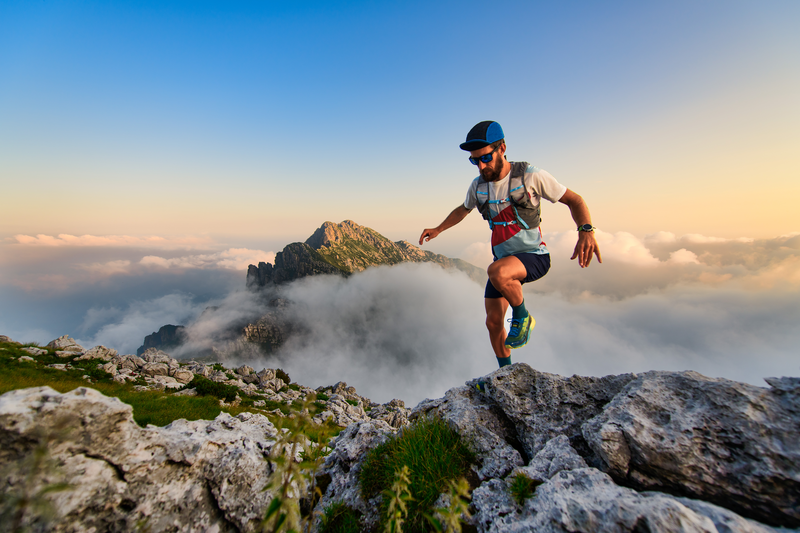
95% of researchers rate our articles as excellent or good
Learn more about the work of our research integrity team to safeguard the quality of each article we publish.
Find out more
ORIGINAL RESEARCH article
Front. Virtual Real. , 30 June 2021
Sec. Virtual Reality in Medicine
Volume 2 - 2021 | https://doi.org/10.3389/frvir.2021.681034
This article is part of the Research Topic Novel Applications of Virtual and Mixed Reality in Pain Research and Treatment View all 6 articles
People with fibromyalgia (FM) have movement-related fear impacting on daily activities. While virtual reality has been used as a distractor to promote exercise, it can be used to manipulate visual feedback (VF) about movement, potentially influencing pain and movement. Objectives: A. To determine whether altered VF modulates pain during movement; B. To compare adaptation to an altered VF between FM participants and healthy controls (HC); C. To explore relationships between adaptation, limb position sense, kinesiophobia and pain. 20 FM participants and 20 HC performed a reaching task during two sessions in a KINARM exoskeleton including a virtual reality interface allowing to replace their arm with a virtual arm. In one session, VF was altered to show GREATER movements while in the other it showed SMALLER movements (randomized order). Pain was assessed periodically using a numerical rating scale. Movement amplitude was assessed during exposure to altered VF (adaptation) and pre-/post-exposure (without VF; after-effects). Limb position sense was assessed with a KINARM task, and kinesiophobia was assessed with the Tampa Scale for Kinesiophobia (TSK-11). Pain intensity increased slightly with movement repetitions (p < 0.001), but did not differ between the VF conditions (GREATER vs. SMALLER). Both groups exhibited visuomotor adaptation, as shown by VF-dependent changes in movement amplitude and speed during exposure to altered VF, and by the presence of VF-dependent after-effects (p < 0.001 for all variables). However, no differences were observed across groups for any of these variables, despite the fact that FM had significantly more difficulty to correctly detect VF conditions than HC (p = 0.046). No clear limb position sense deficits were observed in FM participants, and no significant relationships were found between TSK-11 scores and changes in pain intensity during exposure to altered VF. Altering VF did not influence pain during a reaching task in the FM group. Surprisingly, both groups adapted similarly to altered VF. Visuomotor adaptation is therefore preserved in FM, despite impairments in sensory perception and the poor ability to detect VF alterations in the present study. Further research is warranted to clarify the relationship between sensory perceptions and motor control in FM.
Fibromyalgia (FM) syndrome is a chronic widespread pain condition (Wolfe et al., 2016) affecting 2.7% of the population (Queiroz, 2013), mainly women (McNally et al., 2006), and requiring a combination of pharmacological and non-pharmacological treatments (Goldenberg et al., 2004). Among non-pharmacological interventions, exercise has been shown beneficial (Mannerkorpi and Iversen, 2003; Busch et al., 2008; Mcloughlin et al., 2011). However, FM is often associated with kinesiophobia, i.e. pain-related fear of movement (Turk et al., 2004), that can lead to activity avoidance and disengagement in daily participation, which may in turn contribute to maintaining and aggravating disability in FM patients (Vlaeyen et al., 1995; Asmundson et al., 1999; Turk et al., 2004; Karsdorp and Vlaeyen, 2009). Thus, targeting interventions that promote movement while also reducing kinesiophobia ought to be a promising avenue of treatment for FM patients.
Virtual reality (VR) in the management of pain is known to provide distraction from pain and to increase motivation to move (Keefe et al., 2012; Wiederhold et al., 2014; Jones et al., 2016). An alternative way of using VR consists of providing altered visual feedback (VF) about movements. Introducing a visual rotation or manipulating the visual gain induces trajectory or amplitude modifications, which can extend beyond the duration of the exposure to the manipulation (after-effects) and can occur without a conscious detection by the participant (Ghahramani et al., 1996; Krakauer et al., 2000; Heuer and Hegele, 2008; Bagce et al., 2013; Cressman and Henriques, 2015; Hayashi et al., 2016; Bourdin et al., 2019).
A recent study shows that altering VF to create the perception of smaller movement amplitude in people with chronic neck pain increases actual movement amplitude despite the fact that some participants did not notice the mismatch between the actual and the displayed movement (Chen et al., 2017). Moreover, manipulating the perceived neck rotation via a head-mounted display influenced the onset of pain (Harvie et al., 2015), although there is mitigated evidence on this matter (Kragting et al., 2020). Thus, a VR intervention based on altered VF for people with FM could potentially promote greater movement amplitude and alleviate pain intensity, as participants would perform greater movements without necessarily noticing it.
The general aim of the study was to assess whether altered VF about movement influenced pain intensity and movement kinematics in FM. The specific aims were: A—to determine whether altered VF (GREATER or SMALLER movement) modulates pain during movements; and B—to compare adaptation to an altered VF between FM and healthy controls (HC), both upon (B.1) and after (B.2; after-effects) exposure to altered VF. We hypothesized that: A—SMALLER VF would induce less pain than GREATER VF during reaching movements, since SMALLER VF would make the reaching movement seem less threatening, thus provoking less pain compared to a GREATER VF about that same movement; and that B— both FM and HC participants would adapt their movement amplitude and average speed upon (B.1) and after (B.2) exposure to altered VF, but that FM would adapt to a lesser extent because patients with chronic pain have a less accurate detection of movement manipulation compared to healthy participants (Roosink et al., 2015; Brun et al., 2018b). This, combined with proprioceptive deficits that are frequently reported in chronic pain populations (Lewis et al., 2010; Claeys et al., 2011; Bank et al., 2013; Brun et al., 2018b), could affect their ability to adapt to altered VF. Finally, a secondary aim (aim C) was to explore relationships between adaptation upon exposure to altered VF, limb position sense, kinesiophobia and pain.
Participants with FM and age and sex matched HC participants were recruited. Inclusion criteria specific to the FM group were: adults (>18 years old) with a confirmed diagnosis of FM. Inclusion criteria specific to the HC group were: adults with no neurological or musculoskeletal disorders, including chronic pain. Exclusion criteria for both groups were: visual, motor or neurological impairment that could affect task performance, and having a body mass index over 30 (as this is usually incompatible with the robotic system employed in the study). Overall, 20 participants with FM and 20 age and sex matched HC participants were included in this study. They were recruited from Quebec City Fibromyalgia Association and from Laval University.
All participants provided their written informed consent prior to their participation to the study. The experiment was performed in accordance with the tenets of the Declaration of Helsinki and the study protocol was approved by the local ethical review board (Institut de réadaptation en déficience physique de Québec, Canada, no 2014-395).
Participants from both groups took part in two experimental sessions separated by 7.9 ± 2.1 days. For the FM group, the first experimental session comprised an interview to gather clinical characteristics, including kinesiophobia (see Secondary Outcome Measures for details). Both groups were also required to complete a bilateral Limb position sense Task at the beginning of the first session. As for the rest of the two experimental sessions, they were identical for both groups and consisted of a visuomotor adaptation task comprising a series of target reaching movements with participants’ self-reported dominant upper limb (UL) where a virtual UL replacing the actual UL displayed either a GREATER or SMALLER VF on movement. Participants were also required to complete a series of target reaching movements without VF (i.e. not seeing either their actual or virtual UL) before (PRE-) and after (POST-) exposure to altered VF (Figure 1). One VF condition (GREATER or SMALLER) was tested per session in a counterbalanced order for each group. Pain intensity was verbally assessed throughout the sessions (FM group only). For the FM group, the first experimental session lasted 90 min and the second session lasted 60 min. For the HC group, both sessions lasted 60 min.
FIGURE 1. Study Design. First session began with the Limb position sense Task. Sessions 1 and 2 comprised: the Familiarization task, the Pre-exposure task with no visual feedback (VF) comprising nine targets, the Exposure to altered VF (GREATER or SMALLER) task comprising four blocks of ten targets, the Post-exposure task with no VF comprising six blocks of three of the nine initial targets, pseudo-randomly displayed every 2 min over a period of 10 min, and a Wash-out task. Pain Intensity was assessed verbally with a numerical pain rating scale (NPRS) ranging from 0 to 10 during the Familiarization task (Baseline), as well as after the first (Expo-T1) and last block (Expo-T4) of Exposure to altered VF. Kinematics measures (Movement Amplitude and Average Speed) were acquired during the reaching task in the Pre-Exposure, Exposure (Expo-T1 and Expo-T4) and Post-Exposure blocks.
The limb position sense Task and the Target Reaching Task were completed using the KINARM Exoskeleton Lab™ (KINARM, Kingston ON, Canada). The KINARM is a robotized bilateral exoskeleton allowing robot- or participant-driven movements of the elbow and shoulder joints in the horizontal plane. The robot is interfaced with a 2D virtual environment (Dexterit-E software, version 3.6) which samples participant’s elbow and shoulder joints angles at a 1000 Hz frequency, allowing the display of a real-time feedback on movement with a virtual UL replacing the participant’s real UL (Figure 2). For the present study, actual ULs were obstructed from view in both tasks (Target Reaching Task and Limb position sense Task). For the Target Reaching Task, participants were provided with different types of VF on movement performed by a virtual UL: no VF, veridical VF, GREATER VF or SMALLER VF. This experimental set-up allowed for an immersive virtual experience. Since participants had control over the virtual ULs, which moved in accordance with their actual movements while their real ULs were concealed from view, it enabled a sense of presence which is required in order to achieve immersion (Witmer and Singer, 1998).
FIGURE 2. KINARM set-up and virtual upper limb (UL). The mirror allows the virtual UL to be displayed at a proper depth so that it replaces one’s real UL. During the experiment, a bib was worn to prevent participants from seeing their proximal UL (not shown in the picture).
This task was performed using a KINARM Standard Task [Arm position matching task, version 3.6 (Dukelow et al., 2010)]. The robot randomly moved one of the participant’s UL into one of four predefined positions, and the participant was required to mirror-match the position with the opposite UL. No VF was provided to the participant. Upon completion of the first round (24 trials), the opposite UL was tested. This task was used in previous studies to assess limb position sense in various clinical populations, including stroke (Dukelow et al., 2010) and a chronic pain populations (Brun et al., 2018b; Brun et al., 2020).
After a calibration procedure, participants performed a familiarization task consisting in six reaching movements toward virtual targets using a virtual UL displaying their own movements with a veridical VF on movement. This was achieved to familiarize the participants with the exoskeleton as well as with the reaching task. This task was completed again at the end of each session (wash-out) to minimize potential carry-over effects of altered VF.
Participants were required to reach toward a series of ten randomly displayed targets (presented one by one) with their self-reported dominant hand, with a virtual UL displaying an altered VF either GREATER or SMALLER than one’s own movements. Targets had a 2 cm diameter and were presented either at ±20° from midline, requiring a reaching movement of 8, 10, 12, 14 or 16 cm from the starting position, thus involving an average movement amplitude of 12 ± 3.2 cm (Figure 3A). The altered VF corresponded to a 1.5 magnification (GREATER VF—Figure 3D) or a corresponding minimization (0.67, SMALLER VF—Figure 3E) of the index fingertip position; the displayed fingertip position was scaled with respect to the starting position. Visual target display was also scaled so that the actual movement required was similar between conditions. The distance between the fingertip position and the starting position was first scaled with the desired factor (1.5 or 0.67). Then, using available code from the manufacturer, this scaled position of the fingertip position was used to retrieve angles at shoulder and elbow. These “scaled” angles were then used to display the virtual arm according to the selected scaling factor. It is important to note that only the VF differed between conditions; the actual movement needed to reach the targets being identical for both VF conditions. Participants were instructed to reach as precisely as possible toward the center of each target with the virtual index fingertip and to move at a comfortable pace. At the beginning of the first trial, the UL was passively moved by the robot to the starting position target. At the beginning of each trial, the starting position target was displayed for 2 s (Figure 3A), after which one of the ten targets would appear. Participant had to reach to the target with their index fingertip and maintain this position for 2 s. Target then disappeared while the starting position target reappeared, which marked the beginning of the next trial. Participants completed a total of 40 trials, separated in four blocks (Expo-T1, Expo-T2, Expo-T3, Expo-T4). Only one target was displayed at any given time (either the starting position target or one of the ten targets).
FIGURE 3. Upper panel: Location of targets during (A) PRE- and POST-Exposure to altered visual feedback (VF) and (B) Exposure to altered VF. Lower Panel: Types of VF: PRE- and POST-exposure task, no VF was provided to the participants (C); during Exposure, virtual UL displayed GREATER (D) or SMALLER (E) movements than participant’s actual movements. Blue bars and red dots represent participant’s real UL and were only visible to the experimenter.
Participants had to complete a series of target reaching movements with no VF on movement (Figure 3C). Targets had a 2 cm diameter and were presented either at midline or at ±40° from midline, and at a distance of 8, 12, or 16 cm from the starting position, thus requiring an average movement amplitude of 12 ± 4 cm (Figure 3B). At the beginning of the first trial, the UL was passively moved by the robot to a starting position and a visual cue was provided to the participants once this was achieved. Once the starting position had been maintained for 2 s, targets were randomly displayed one by one. Note that the position of these targets was slightly different from the ones used during the exposure to altered VF in order to assess whether the adaptation to altered VF would generalize to various movements. Participants were instructed to reach as precisely as possible toward the center of each target, moving at a comfortable pace, and to give a verbal cue once they had reached the target. Once this was achieved, participants had to go back to the starting position in order to launch the next trial.
PRE-Exposure: Prior to Exposure, participants reached once toward each of the nine targets (randomly displayed).
POST-Exposure: This task comprised the same nine targets as the Pre-Exposure task, but split into three blocks (average required movement amplitude for each block: 12 cm) which were presented in a pseudo-random order every 2 min over a 10 min period. Overall, each block was repeated twice.
Pain Intensity was assessed verbally with a Numerical Pain Rating Scale (NPRS) ranging from 0 (no pain) to 10 (worst possible pain). This outcome was measured at rest at different time points throughout the sessions: 1- After the Familiarization task (Baseline); 2- After the first altered VF exposure block (Expo-T1) and 3- After the fourth altered VF exposure block (Expo-T4).
Movement Amplitude (cm) was calculated for each trial as the length of a vector between the starting position and the index fingertip position at the end of movement. The end of movement was defined as the x and y coordinates where participant’s reaching movement stopped (speed < 2 cm/s), before readjustments; Average Speed (cm/s) was calculated for each trial among the vector corresponding to Movement Amplitude.
Both kinematic variables were computed from the index fingertip position calculated in real-time by the Dexterit-E software using custom-made MATLAB 2013 scripts (Mathworks inc., Massachussetts).
In order to assess explicit awareness of the alteration, participants were asked to identify which VF condition they had been exposed to at the end of each session. Reported answers were either “GREATER VF,” “SMALLER VF” or “Don’t know.” Note that at the beginning of each session, participants were told that VF would either be GREATER or SMALLER. They did not know whether VF condition would be different or the same between the two sessions.
For the Limb position sense Task, the mean absolute distance errors (cm) in the x- and y-axes across trials were obtained for both ULs.
Clinical characteristics for the FM group were gathered at the beginning of the first session and included a brief medical history (time since diagnosis, comorbidities, medication, Pain intensity) as well as the completion of the TSK-11. The TSK-11 is a self-reported questionnaire assessing movement-related fear among people suffering from chronic pain. Its score ranges between 11–44, with higher scores indicating greater movement-related fear of pain (George et al., 2012). A French-Canadian version of this questionnaire has been validated (French et al., 2002).
Results are reported as mean ± standard deviation (SD). An independent t test (2-tailed) was used to compare groups for age, and a Fisher’s exact test was used to compare groups for handedness.
Pain Intensity was analyzed using a 2 [VF condition (GREATER or SMALLER)] × 3 [Time (Baseline, Expo-T1, Expo-T4)] repeated-measures analyses of variance (rmANOVA). It was hypothesized that SMALLER VF would induce less pain than GREATER VF during reaching movements.
Movement Amplitude and Average Speed were analyzed for the altered VF exposure phase using 2 [Group (FM or HC)] × 2 [VF condition (GREATER or SMALLER)] × 2 [Time (Expo-T1, Expo-T4)] rmANOVAS. It was hypothesized that both FM and HC would adapt their movement amplitude and average speed, but to a lesser extend for the FM group. Adaptation should manifest as a decrease in movement amplitude and speed over time when exposed to GREATER VF (and vice-versa for SMALLER VF) to ultimately achieve similar movement amplitude and average speed for both VF conditions by the end of exposure.
To assess whether participants were aware of the visual manipulation, the proportion of correct identification of both VF conditions among Groups was assessed using chi-square test of independence.
For the PRE- and POST-Exposure phases, Movement Amplitude and Average Speed were analyzed using 2 [Group (FM or HC)] × 2 [VF condition (GREATER or SMALLER)] × 3 [Time (PRE, 0 minPOST, 10 minPOST)] rmANOVAs. It was hypothesized that both FM and HC would show after-effects, but to a lesser extend for the FM group. Expected after-effects would be a decrease in movement amplitude and average speed following exposure to a GREATER VF (and vice-versa for SMALLER VF) (Cressman and Henriques, 2015).
Two participants from the HC group and one participant from the FM had to be excluded from the Average Speed analysis as their movements were too jerky, hence it was not possible to calculate their Average Speed.
Whether FM participants showed proprioceptive deficits was first established by analyzing errors in the Limb position sense task using a 2 [Groups (FM or HC)] × 2 [Error direction (x-axis or y-axis)] rmANOVA.
The different variables of interest were assessed using Kendall’s tau correlation coefficients.
Normal distribution of the data was assessed using the Kolmogorov-Smirnov goodness-of-fit test, homoscedasticity was assessed using Levene test and sphericity was assessed with Mauchly test. Greenhouse-Geisser correction was used when the assumption of sphericity was not respected. For all comparisons, p < 0.05 (two-sided) was considered statistically significant. Post-hoc analyses were performed using SIDAK correction.
All statistical analyses were performed using IBM SPSS Statistics software version 25.0 (IBM Corp., Armonk, New York).
Participants’ demographic characteristics (age, handedness, gender) and FM participants’ clinical characteristics (time since diagnosis, average pain intensity, and TSK-11 scores) are reported in Table 1. HC and FM groups did not differ in terms of age or handedness.
No main effect of VF on Pain was observed [F (1, 19) = 0.37, p = 0.55] for the FM group. There was a main effect of Time on Pain [F (1.5, 29.1) = 17.5, p < 0.001, ηp = 0.48], which increased over the course of the experiment (Figure 4). Post-hoc analyses revealed that Pain intensity was greater at Expo-T4 compared to Expo-T1 (p = 0.031) and Baseline (p = < 0.001), and that Pain intensity was greater at Expo-T1 compared to Baseline (p = 0.001). Notably, average Pain at Expo-T4 was comparable to that reported by participants over the last 24 h, suggesting that task-induced Pain was not worse than that associated with regular daily activities. There was no interaction between Time and VF, both VF conditions leading to similar increases in Pain [F (2, 38) = 0.13, p = 0.88].
FIGURE 4. Pain Intensity at Baseline, Expo-T1 and Expo-T4 for the GREATER and SMALLER visual feedback (VF). Error bars represent the standard error of the mean.
In order to assess adaptation to altered VF upon exposure, Movement Amplitude and Average Speed changes between the beginning (Expo-T1) and the end (Expo-T4) of exposure to altered VF were analyzed.
Both groups adapted their movements to both VF conditions, as shown by changes in Movement Amplitude that were specific to the VF condition (Figure 5A). There was a significant interaction between Time and VF [F (1,38) = 20.6, p < 0.001, ηp = 0.35]. Post-hoc analyses revealed that for the SMALLER VF condition, movement amplitude increased over time during exposure (p < 0.001) whereas for the GREATER VF condition, movement amplitude decreased over time during exposure (p = 0.02). It is also of interest to note that by the end of exposure (Expo-T4), Movement Amplitude tends to be closer to 12 cm for both VF conditions (Figure 5A), which is the real average amplitude required to reach the targets. There was a main effect of VF on Movement amplitude [F (1,38) = 63.4, p < 0.001, ηp = 0.63], however there was no main effect of Time [F (1,38) = 3.72, p = 0.06] as a result of the interaction. Altogether, these results show that participants adapted their reaching movement by altering movement amplitude in a way that is consistent with the displayed VF. Importantly however, there was no main effect of Group [F (1,38) = 0.06, p = 0.81], nor any significant interaction between Group and the other variables, meaning that adaptation was similar between FM and HC.
FIGURE 5. Adaptation to altered visual feedback (VF) upon exposure across groups. (A) As expected, Movement amplitude increased over time in the SMALLER VF condition (circles), but decreased in the GREATER VF condition (triangles). These changes were similar in participants with fibromyalgia (FM; red empty symbols) and healthy controls (HC; blue filled symbols) (B) Both groups adapted their Average Speed in a way that is consistent with the displayed VF condition, but this adaptation was similar across groups. Error bars represent standard error of the mean.
As for Movement Amplitude, both groups adapted their Average Speed in a way that is consistent with the displayed VF condition (Figure 5B), but this adaptation was similar across groups. There was a significant interaction between Time and VF [F (1, 38) = 29.9, p < 0.001, ηp = 0.44]; post-hoc analyses revealed that at Expo-T1, Average Speed was greater for the GREATER VF condition compared to the SMALLER VF condition (p < 0.001), whereas there Average speed was not statistically different between GREATER and SMALLER VF at Expo-T4 (p = 0.92). Those results show that participants adapted their movement Speed to both VF conditions. There were also main effects of VF [F (1, 38) = 6.28, p = 0.02, ηp = 0.14] and Time [F (1, 38) = 38.4, p < 0.001, ηp = 0.50] on Average Speed. Importantly however, there was no main effect of Group [F (1,38) = 0.20, p = 0.66], nor any significant interaction between Group and the other variables, again indicating that adaptation was similar between FM and HC. The absence of a Group effect also indicates that even though movements were performed at a self-selected comfortable speed, FM participants produced movements that were comparable to those of HC participants.
After each session, participants were asked to identify which VF condition they had been exposed to. 20% of FM participants and 50% of HC participants correctly identified both VF conditions. Chi-square test revealed a significant relation between Group and accurate identification of altered VF [X2 (1, N = 40) = 3.95, p = 0.046].
In order to assess the presence of after-effects after exposure to altered VF, changes in Movement Amplitude and Average Speed between Pre- and Post-exposure (0 minPost-exposure and 10 minPost-exposure) phases were analyzed.
Both groups displayed after-effects for both VF conditions, that is, changes in Movement Amplitude in the Post-exposure phase compared with the Pre-exposure phase (Figure 6A). There was a significant interaction between Time and VF [F (1.7, 66.4) = 33.2, p < 0.001, ηp = 0.47]; post-hoc analyses revealed that Movement Amplitude was not different between SMALLER and GREATER VF in the Pre-exposure phase (p = 0.13), whereas at 0minPost-exposure, Movement Amplitude was greater for the SMALLER VF condition than the GREATER VF condition (p < 0.001), and this difference remained present at 10minPost-exposure (p < 0.001). Those results are consistent with the after-effects expected Post-exposure to GREATER and SMALLER VF. There were also main effects of VF [F (1, 38) = 99.4, p < 0.001, ηp = 0.72] and Time [F (1.4, 54.4) = 13.5, p < 0.001, ηp = 0.26] on Movement Amplitude; post-hoc analyses revealed that Movement Amplitude was greater in the Pre-exposure phase compared to 0minPost-Exposure (p = 0.005) and to 10minPost-exposure (p < 0.001). However, the difference between 0minPost and 10minPost was not statistically significant (p = 0.053). There was no effect of Group on Movement Amplitude [F (1,38) = 2.24, p = 0.14], and the only significant interaction involving Group did not involve VF {Group*Time interaction [F (1.4, 54.4) = 7.4, p = 0.004, ηp = 0.16], the Control Group making slightly larger movements than FM group prior to exposure (p = 0.013)}. Overall, these results suggest that both groups displayed similar after-effects Post-exposure to altered VF.
FIGURE 6. Adaptation to altered visual feedback (VF): After-effects across groups. (A) As expected, Movement amplitude increased post-exposure to SMALLER VF condition (circles), but decreased post-exposure to GREATER VF condition (triangles). These changes were similar in subjects with fibromyalgia (FM; red empty symbols) and healthy controls (HC; blue filled symbols) (B) Both groups adapted their Average Speed in a way that is consistent with the displayed VF condition, but this adaptation was similar across groups. Error bars represent standard error of the mean.
As for Movement Amplitude, participants adapted their Average Speed Post-Exposure to both altered VF conditions (Figure 6B). There was a significant interaction between Time and VF [F (1.8, 63.6) = 16.1, p < 0.001, ηp = 0.32]; post-hoc analyses revealed that Average Speed was not different between the two VF conditions prior to exposure (p = 0.42). At 0minPost-exposure, Average Speed was greater after the SMALLER VF condition than the GREATER VF condition (p < 0.001), and this pattern was maintained at 10minPost-exposure (p = 0.001). There were also main effects of VF [F (1, 35) = 30.5, p < 0.001, ηp = 0.47] and Time [F (1.6, 57.3) = 4.48, p = 0.02, ηp = 0.11] on Average Speed; post-hoc analyses revealed that Average Speed was greater in the Pre-exposure phase compared to the 0minPost-exposure phase (p = 0.04) and the 10minPost-exposure phase (p = 0.03). However, the difference between 0minPost and 10minPost was not statistically significant (p = 0.77). There was no effect of Group on Average Speed Pre- and Post-exposure to altered VF [F (1,35) = 1.88, p = 0.18], nor any significant interaction between Group and the other variables, again indicating that adaptation was similar between FM and HC.
The rmANOVA revealed no main effect of Group on mean errors [F (1,38) = 0.17, p = 0.68], both groups displaying similar errors for a specific direction (x- or y-axis), although there was a significant main effect of the Error direction, participants displaying larger errors in the x-axis than in the y-axis [F (1, 38) = 75.9, p < 0.001, ηp = 0.67]. No significant interaction was observed between Group and Error direction [F (1, 38) = 0.22, p = 0.64]. Figure 7A shows an example of representative participants from each group. Figure 7B shows the mean errors in the x- and y-axes for each group. As no deficit in Limb position sense nor deficits in adaptation were observed, associations with changes in Pain were not further assessed.
FIGURE 7. Limb position sense Task. (A) Individual data for representative participants from each group. The green squares represent the position of left and right upper limbs (UL) moved passively by the robot, and the superimposed blue dashed squares represent the matching positions with the contralateral UL. (B) Mean errors for the FM and HC groups in the x- (in black) and y-axes (in gray). Error bars represent the standard error of the mean.
Mean score for the TSK-11 was 26.6 ± 6.3. Kendall’s tau coefficient between TSK-11 scores and changes in Pain intensity between Expo-T1 and Expo-T4 pooled for both VF conditions was not statistically significant (τ= 0.048, p = 0.8). As not deficits in adaptation were observed, no further assessment of relationship between kinesiophobia and adaptation were conducted.
The general aim of this study was to assess whether altered VF influenced pain intensity and UL kinematics in FM participants. Overall, the results showed that altered VF did not impact on pain intensity and that both FM and HC adapted their movement amplitude and speed upon and after exposure to SMALLER and GREATER VF, without any differences observed between FM and HC while FM participants had more difficulty to detect the altered VF. Interestingly, this difficulty to detect the altered feedback was observed despite the absence of significant deficits in Limb position sense in the FM group. This apparent discrepancy could be explained by the fact that assessment of Limb position sense mainly depends on the processing of afferent feedback (the tested arm being positioned passively), while the detection of visuomotor mismatch is likely to rely not only on afferent proprioceptive feedback, but also on motor efferences. Interestingly, higher conflict sensitivity (i.e. sensory disturbances evoked when the visual feedback is altered in a very obvious manner) in fibromyalgia has been recently shown to occur mainly in sensory-motor conflicts rather than in sensory-sensory conflicts, which could reflect a deficit in updating predicted sensory feedback (Brun et al., 2020). Finally, no significant association was found between kinesiophobia and changes in pain intensity during exposure to altered VF.
Contrary to our hypothesis, altering the VF about movement amplitude and velocity during a reaching task does not impact on resting pain after each condition. This contrasts with results from previous studies in various chronic pain populations showing that various manipulations of VF induce pain reduction, such as altering the size of the limb in complex regional pain syndrome (CRPS) (Moseley et al., 2008; Matamala-Gomez et al., 2019), its transparency in peripheral nerve injury (Matamala-Gomez et al., 2019), or the perceived rotation amplitude of the neck in chronic neck pain (Harvie et al., 2015). The rationale put forward by the authors to account for these results is generally based on the presence of maladaptive reorganization within the sensorimotor cortices in these populations. For instance, functional magnetic resonance imaging and magnetoencephalography studies led with CRPS patients have shown smaller cortical representation of the affected limb within the primary somatosensory cortex (S1) contralateral to the lesion (Di Pietro et al., 2013). Similar anomalies in the somatosensory processing have also been identified in the FM population (Kim et al., 2015), although clinical pain patterns are quite different compared to the aforementioned chronic pain populations in that FM is associated with widespread pain (Wolfe et al., 1990), as opposed to regional pain, for instance in CRPS (Harden et al., 2010). In light of the aforementioned studies, it was relevant to test whether altering the VF about movement would modulate pain, as it has been shown with other types of VF alterations. However, recently published work makes it less clear whether altering VF has an impact on pain. For instance, results from Harvie et al. were not replicated in a multicenter study undergone with a larger sample (Kragting et al., 2020). Moreover, recent systematic review (Heinrich et al., 2020) and meta-analysis (Wittkopf et al., 2018) put in light mitigated results obtained from studies altering the size of painful body parts in the treatment of chronic pain populations. One factor that could account for the discrepancies between studies is whether feedback manipulations were explicitly perceived by participants. It has been shown that activation of the primary somatosensory cortex reflects the perceived rather than the physical characteristics of the stimulus (Chen et al., 2003), highlighting the influence of perception on sensorimotor cortices activation (Chen et al., 2003; McCabe et al., 2009). It is important to note that manipulation of VF was only correctly detected by 20% of FM participants in the present study. Therefore, it can be suggested that accurate perception of visual manipulations is necessary to influence pain ratings in VR environments. Moreover, considering the fact that people with pain have more difficulty to identify visual manipulations (Roosink et al., 2015; Harvie et al., 2016; Brun et al., 2018b) than pain-free individuals, larger feedback manipulations (i.e. easier to detect than in the present study) might be needed to impact on pain. Furthermore, the fact that most FM participants did not detect the VF alterations could also explain why there was no relationship found between pain and kinesiophobia. The rationale behind the hypotheses in the present study was that a SMALLER VF would make the reaching movement seem less threatening, thus provoking less pain compared to a GREATER VF about that same movement. But, without a conscious awareness of the alteration, the only thing perceived will be the visuomotor mismatch, which will likely increase Pain since FM are more sensitive to perceptual mismatches (Brun et al., 2020). More research is warranted to acquire a more thorough understanding of the role of awareness of visuomotor mismatch in pain modulation. FM adapted their movement amplitude and speed to the same extent as HC upon and after exposure to altered VF. Since FM participants had significantly more difficulty to correctly identify both VF alterations compared to HC participants, this result confirms that of from previous studies showing that explicit knowledge of the alteration is not a pre-requisite for visuomotor adaptation (Bourdin et al., 2019). On a clinical point of view, it is tempting to infer that abnormal sensory perceptions lead to altered motor control, but literature suggests that this relationship is not as straightforward. (Brun et al., 2018a; Brun et al., 2020). In a study involving CRPS and HC participants, both groups performed similarly in a target location task, despite differences in sensorimotor integration for the CRPS participants compared to HC (Bultitude and Petrini, 2021). Moreover, a recent study involving visuomotor conflicts in FM vs. HC demonstrated that while people with FM are more perceptually sensitive to visuomotor conflicts (i.e. reporting higher sensory disturbances), motor disturbances induced by conflict were similar between groups (Brun et al., 2020). Comparable results have been obtained in healthy people with experimental tonic pain (Brun et al., 2017), suggesting a dissociation between the effect of pain on perception and action.
Supporting that view, alterations in the treatment of sensory information and multisensory integration have been reported in FM and other chronic pain conditions (McDermid et al., 1996; McCabe et al., 2009; Martinez et al., 2018; Martínez et al., 2019), while transcranial magnetic studies have shown no evidence of sensorimotor integration deficits in either acute or chronic pain [measured with short afferent inhibition (Turton et al., 2007; Mercier et al., 2016; Vuralli et al., 2019)]. Studies looking specifically at the effect of pain on upper limb motor learning have produced mixed results, but overall do not reveal major upper limb motor learning deficits in the presence of pain (Ingham et al., 2011; Dancey et al., 2014; Dancey et al., 2016a; Dancey et al., 2016b; Lamothe et al., 2014; Bilodeau et al., 2016; Mavromatis et al., 2017; Parker et al., 2017; Salomoni et al., 2019). Importantly, most of these studies have been conducted in acute experimental pain models, the present study being one the few focusing on individuals with chronic pain. In a study conducted with painful arthritic hand patients and HC, motor performance in a motor skill training task was overall similar between groups, suggesting preserved motor function and skill learning among the patient group (Parker et al., 2017). Furthermore, in prismatic adaptation studies with CRPS patients, visuomotor adaptation was preserved as shown by large pointing errors in the first open-loop movements that faded with repetition (corresponding with visuomotor adaptation) and after-effects following removal of prismatic goggles (Bultitude and Rafal, 2010; Christophe et al., 2016), although these studies did not comprise a control group to compare adaptation with, making the results of the present study all the more relevant.
In order to assess the external validity of the present study, it is relevant to question whether our FM group was representative of the FM population in terms of average pain, kinesiophobia and proprioceptive deficits. Since our participants were recruited from the general public—and not from a clinical facility (e.g. Pain clinic), it is likely that they displayed on average less pain and higher levels of function compared to the FM patients seen in outpatient programs, hence making them less different from HC participants than in other studies. For instance, in a study conducted with 36 FM patients recruited from outpatient pain programs, average reported pain intensity over the last 24 h was 6.9 ± 1.9 on a 0–10 visual analog scale (Brun et al., 2018c) compared to 4.9 ± 1.7 in the present study. As for kinesiophobia, in a study involving 391 FM participants (Roelofs et al., 2007), the mean TSK-11 score was 24.5 ± 6.1, which indicates overall similar levels of kinesiophobia to that recorded in the present study (mean TSK-11 score: 26.6 ± 6.3). In terms of proprioceptive deficits, both FM and HC groups in the present study displayed similar errors in the Limb position sense. Literature supporting the presence of proprioception deficits among FM syndrome is scarce and it is unclear whether people with FM have more proprioceptive deficits than HCs. While Celenay et al. found poorer trunk position sense in FM (Toprak Celenay et al., 2019), Akyol et al. found no differences between the FM and HC group in terms of proprioceptive acuity of the knee joint (Akyol et al., 2013). Similarly, Bardal et al. found no differences between FM and HC in the shoulder joint position sense test. Most relevantly, in a previous study in our lab using the same Limb position sense Task as the present study in a different sample of participants, no differences were found between FM and HC (Brun et al., 2020). Therefore, it appears that FM participants from the present study are representative of the FM population in terms of Pain, kinesiophobia and proprioception. It is important to acknowledge however that the sample size remains limited, raising the possibility of type two errors, especially for Time*VF*Group interaction (power being between 0.07 and 0.42 for the interaction term). Therefore, while the results clearly show that participants with FM are well able to perform visuomotor adaptation, no definitive conclusion can be reached on the absence of difference from controls. Nevertheless, no trend was observed, making it unlikely that major deficits would be observed with a larger sample. A limit of the current study is that participants were asked to identify VF conditions without a two-alternative forced choice. As a result, some participants answered that it felt like the VF was true to their own movements—even though they were told that VF would be either GREATER or SMALLER than their own movements. As a result, it was only possible to identify which participants had correctly identified both VF conditions (and not the participants who had wrongly identified one or both VF conditions). In the future, it would be relevant to assess accuracy of detection with a two-alternative forced choice in order to assess whether one VF condition was more easily detected than the other, and also what is the relationship between accurate detection and Pain.
In conclusion, although FM is associated with abnormalities in sensory processing (McDermid et al., 1996; McCabe et al., 2009) and kinesiophobia, this did not seem to interfere with participants’ capacity to adapt their movements to an altered VF during a reaching task. But while visuomotor adaptation is preserved in FM, an intervention based on adaptation to SMALLER VF not did alleviate pain, nor promote greater movement amplitude compared to GREATER VF. Further research needs to be done in order to clarify the relationship between sensory perceptions and motor control in the FM population. As studies using various VR interventions continue to be conducted with chronic pain populations, one ought to be cautious when it comes to generalizing efficacy of treatment. In fact, further research shall try to clarify the mechanisms underlying pain alleviation in VR paradigms with specific chronic pain populations and the role of awareness in the modulation of pain.
The raw data supporting the conclusion of this article will be made available by the authors, without undue reservation.
The studies involving human participants were reviewed and approved by the Comité d'éthique et de recherche du CIUSSS de la Capitale Nationale. The patients/participants provided their written informed consent to participate in this study.
CM and CB designed the study; AO and MD performed data collection; CM, CB, and MD analyzed and interpreted the data; MD drafted the paper; MD, CB, AO, and CM commented on the paper and approved the final version.
CM is supported by a salary award from Fonds de recherche Québec-Santé (FRQS) (Grant Number 251649) and by an operating grant from the Canadian Institutes of Health Research (CIHR; MOP-125869). The technical development was supported by an ENGAGE grant from Natural Sciences and Engineering Research Council of Canada (NSERC; EGP477404-14), obtained in collaboration with BKIN Technologies. MD is supported by fellowships from FRQS, CIHR, Center for Interdisciplinary Research in Rehabilitation and Social Integration and Laval University Faculty of Medicine.
The authors declare that the research was conducted in the absence of any commercial or financial relationships that could be construed as a potential conflict of interest.
We thank Nicolas Robitaille, PhD, for developing and implementing the task and Martin Gagné, PhD, for the MATLAB scripts.
Akyol, Y., Ulus, Y., Tander, B., Bilgici, A., and Kuru, O. (2013). Muscle Strength, Fatigue, Functional Capacity, and Proprioceptive Acuity in Patients With Fibromyalgia. Turkiye Fiz. Tip Ve Rehabil. Dergisi-Turkish J. Phys. Med. Rehabil. 59, 292–298. doi:10.4274/tftr.22230
Asmundson, G. J. G., Norton, P. J., and Norton, G. R. (1999). Beyond Pain. Clin. Psychol. Rev. 19, 97–119. doi:10.1016/s0272-7358(98)00034-8
Bagce, H. F., Saleh, S., Adamovich, S. V., Krakauer, J. W., and Tunik, E. (2013). Corticospinal Excitability Is Enhanced after Visuomotor Adaptation and Depends on Learning rather Than Performance or Error. J. Neurophysiol. 109, 1097–1106. doi:10.1152/jn.00304.2012
Bank, P. J. M., Peper, C. E., Marinus, J., Beek, P. J., and van Hilten, J. J. (2013). Motor Dysfunction of Complex Regional Pain Syndrome Is Related to Impaired Central Processing of Proprioceptive Information. The J. Pain 14, 1460–1474. doi:10.1016/j.jpain.2013.07.009
Bilodeau, M.-C., Roosink, M., and Mercier, C. (2016). Effect of Local versus Remote Tonic Heat Pain during Training on Acquisition and Retention of a finger-tapping Sequence Task. Exp. Brain Res. 234, 475–482. doi:10.1007/s00221-015-4478-3
Bourdin, P., Martini, M., and Sanchez-Vives, M. V. (2019). Altered Visual Feedback from an Embodied Avatar Unconsciously Influences Movement Amplitude and Muscle Activity. Sci. Rep. 9, 1–9. doi:10.1038/s41598-019-56034-5
Brun, C., Gagné, M., McCabe, C. S., and Mercier, C. (2017). Sensory Disturbances, but Not Motor Disturbances, Induced by Sensorimotor Conflicts Are Increased in the Presence of Acute Pain. Front. Integr. Neurosci. 11, 1–13. doi:10.3389/fnint.2017.00014
Brun, C., Giorgi, N., Pinard, A.-M., Gagné, M., McCabe, C. S., and Mercier, C. (2019a). Exploring the Relationships between Altered Body Perception, Limb Position Sense, and Limb Movement Sense in Complex Regional Pain Syndrome. J. Pain 20, 17–27. doi:10.1016/j.jpain.2018.07.008
Brun, C., Giorgi, N., Pinard, A.-M., Gagné, M., McCabe, C. S., and Mercier, C. (2019b). Exploring the Relationships Between Altered Body Perception, Limb Position Sense, and Limb Movement Sense in Complex Regional Pain Syndrome. J. Pain 20, 17–27. doi:10.1016/j.jpain.2018.07.008
Brun, C., McCabe, C. S., and Mercier, C. (2020). The Contribution of Motor Commands to the Perturbations Induced by Sensorimotor Conflicts in Fibromyalgia. Neuroscience 434, 55–65. doi:10.1016/j.neuroscience.2020.03.017
Brun, C., Mercier, C., Grieve, S., Palmer, S., Bailey, J., and McCabe, C. S. (2018c). Sensory Disturbances Induced by Sensorimotor Conflicts Are Higher in Complex Regional Pain Syndrome and Fibromyalgia Compared to Arthritis and Healthy People, and Positively Relate to Pain Intensity. Eur. J. Pain 23, 483–494. doi:10.1002/ejp.1322
Bultitude, J. H., and Petrini, K. (2021). Altered Visuomotor Integration in Complex Regional Pain Syndrome. Behav. Brain Res. 397, 112922. doi:10.1016/j.bbr.2020.112922
Bultitude, J. H., and Rafal, R. D. (2010). Derangement of Body Representation in Complex Regional Pain Syndrome: Report of a Case Treated with Mirror and Prisms. Exp. Brain Res. 204, 409–418. doi:10.1007/s00221-009-2107-8
Busch, A. J., Schachter, C. L., Overend, T. J., Peloso, P. M., and Barber, K. A. (2008). Exercise for Fibromyalgia: A Systematic Review. J. Rheumatol. 35, 1130–1144.
Chen, K. B., Sesto, M. E., Ponto, K., Leonard, J., Mason, A., Vanderheiden, G., et al. (2017). Use of Virtual Reality Feedback for Patients with Chronic Neck Pain and Kinesiophobia. IEEE Trans. Neural Syst. Rehabil. Eng. 25, 1240–1248. doi:10.1109/TNSRE.2016.2621886
Chen, L. M., Friedman, R. M., and Roe, A. W. (2003). Optical Imaging of a Tactile Illusion in Area 3b of the Primary Somatosensory Cortex. Science 302, 881–885. doi:10.1126/science.1087846
Christophe, L., Chabanat, E., Delporte, L., Revol, P., Volckmann, P., Jacquin-Courtois, S., et al. (2016). Prisms to Shift Pain Away: Pathophysiological and Therapeutic Exploration of CRPS with Prism Adaptation. Neural Plasticity 2016, 1–21. doi:10.1155/2016/1694256
Claeys, K., Brumagne, S., Dankaerts, W., Kiers, H., and Janssens, L. (2011). Decreased Variability in Postural Control Strategies in Young People with Non-specific Low Back Pain Is Associated with Altered Proprioceptive Reweighting. Eur. J. Appl. Physiol. 111, 115–123. doi:10.1007/s00421-010-1637-x
Cressman, E. K., and Henriques, D. Y. P. (2015). Generalization Patterns for Reach Adaptation and Proprioceptive Recalibration Differ after Visuomotor Learning. J. Neurophysiol. 114, 354–365. doi:10.1152/jn.00415.2014
Dancey, E., Murphy, B. A., Andrew, D., and Yielder, P. (2016a). The Effect of Local vs Remote Experimental Pain on Motor Learning and Sensorimotor Integration Using a Complex Typing Task. Pain 157, 1682–1695. doi:10.1097/j.pain.0000000000000570
Dancey, E., Murphy, B., Andrew, D., and Yielder, P. (2016b). Interactive Effect of Acute Pain and Motor Learning Acquisition on Sensorimotor Integration and Motor Learning Outcomes. J. Neurophysiol. 116, 2210–2220. doi:10.1152/jn.00337.2016
Dancey, E., Murphy, B., Srbely, J., and Yielder, P. (2014). The Effect of Experimental Pain on Motor Training Performance and Sensorimotor Integration. Exp. Brain Res. 232, 2879–2889. doi:10.1007/s00221-014-3966-1
Di Pietro, F., McAuley, J. H., Parkitny, L., Lotze, M., Wand, B. M., Moseley, G. L., et al. (2013). Primary Somatosensory Cortex Function in Complex Regional Pain Syndrome: A Systematic Review and Meta-Analysis. J. Pain 14, 1001–1018. doi:10.1016/j.jpain.2013.04.001
Dukelow, S. P., Herter, T. M., Moore, K. D., Demers, M. J., Glasgow, J. I., Bagg, S. D., et al. (2010). Quantitative Assessment of Limb Position Sense Following Stroke. Neurorehabil. Neural Repair 24, 178–187. doi:10.1177/1545968309345267
French, D. J., Roach, P. J., and Mayes, S. (2002). Peur du mouvement chez des accidentés du travail: L'Échelle de Kinésiophobie de Tampa (EKT). Can. J. Behav. Sci. 34, 28–33. doi:10.1037/h0087152
George, S. Z., Lentz, T. A., Zeppieri, G., Lee, D., and Chmielewski, T. L. (2012). Analysis of Shortened Versions of the Tampa Scale for Kinesiophobia and Pain Catastrophizing Scale for Patients After Anterior Cruciate Ligament Reconstruction. Clin. J. Pain 28, 73–80. doi:10.1097/AJP.0b013e31822363f4.Analysis
Ghahramani, Z., Wolpert, D. M., and Jordan, M. I. (1996). Generalization to Local Remappings of the Visuomotor Coordinate Transformation. J. Neurosci. 16, 7085–7096. doi:10.1523/jneurosci.16-21-07085.1996
Goldenberg, D. L., Burckhardt, C., and Crofford, L. (2004). Management of Fibromyalgia Syndrome. JAMA 292, 2388–2395. doi:10.1001/jama.292.19.2388
Harden, N. R., Bruehl, S., Perez, R. S. G. M., Birklein, F., Marinus, J., Maihofner, C., et al. (2010). Validation of Proposed Diagnostic Criteria (The “Budapest Criteria”) for Complex Regional Pain Syndrome. Pain 150, 268–274. doi:10.1016/j.pain.2010.04.030
Harvie, D. S., Broecker, M., Smith, R. T., Meulders, A., Madden, V. J., and Moseley, G. L. (2015). Bogus Visual Feedback Alters Onset of Movement-Evoked Pain in People With Neck Pain. Psychol. Sci. 26, 385–392. doi:10.1177/0956797614563339
Harvie, D. S., Hillier, S., Madden, V. J., Smith, R. T., Broecker, M., Meulders, A., et al. (2016). Neck Pain and Proprioception Revisited Using the Proprioception Incongruence Detection Test. Detect. Test 96, 671–678. doi:10.2522/ptj.20150210
Hayashi, T., Yokoi, A., Hirashima, M., and Nozaki, D. (2016). Visuomotor Map Determines How Visually Guided Reaching Movements Are Corrected Within and Across Trials. eNeuro 3, 1–13. doi:10.1523/ENEURO.0032-16.2016
Heinrich, M., Steiner, S., and Bauer, C. M. (2020). The Effect of Visual Feedback on People Suffering from Chronic Back and Neck Pain - a Systematic Review. Physiother. Theor. Pract. 36, 1220–1231. doi:10.1080/09593985.2019.1571140
Heuer, H., and Hegele, M. (2008). Adaptation to a Nonlinear Visuomotor Amplitude Transformation With Continuous and Terminal Visual Feedback. J. Mot. Behav. 40, 368–379. doi:10.3200/jmbr.40.5.368-379
Ingham, D., Tucker, K. J., Tsao, H., and Hodges, P. W. (2011). The Effect of Pain on Training-Induced Plasticity of the Corticomotor System. Eur. J. Pain 15, 1028–1034. doi:10.1016/j.ejpain.2011.04.006
Jones, T., Moore, T., and Choo, J. (2016). The Impact of Virtual Reality on Chronic Pain. PLoS One 11, e0167523. doi:10.1371/journal.pone.0167523
Karsdorp, P. A., and Vlaeyen, J. W. S. (2009). Active Avoidance but Not Activity Pacing Is Associated with Disability in Fibromyalgia. Pain 147, 29–35. doi:10.1016/j.pain.2009.07.019
Keefe, F. J., Huling, D. A., Coggins, M. J., Keefe, D. F., Rosenthal, Z. M., Herr, N. R., et al. (2012). Virtual Reality for Persistent Pain: A New Direction for Behavioral Pain Management. Pain 153, 2163–2166. doi:10.1016/j.pain.2012.05.030
Kim, J., Loggia, M. L., Cahalan, C. M., Harris, R. E., Beissner, F., Garcia, R. G., et al. (2015). The Somatosensory Link in Fibromyalgia: Functional Connectivity of the Primary Somatosensory Cortex Is Altered by Sustained Pain and Is Associated with Clinical/autonomic Dysfunction. Arthritis Rheumatol. 67, 1395–1405. doi:10.1002/art.39043
Kragting, M., Schuiling, S. F., Voogt, L., Pool‐Goudzwaard, A. L., and Coppieters, M. W. (2020). Using Visual Feedback Manipulation in Virtual Reality to Influence Pain‐Free Range of Motion in People with Nonspecific Neck Pain. Pain Pract. 21, 428–437. doi:10.1111/papr.12971
Krakauer, J. W., Pine, Z. M., Ghilardi, M.-F., and Ghez, C. (2000). Learning of Visuomotor Transformations for Vectorial Planning of Reaching Trajectories. J. Neurosci. 20, 8916–8924. doi:10.1523/JNEUROSCI.20-23-08916.2000
Lamothe, M., Roy, J.-S., Bouffard, J., Gagné, M., Bouyer, L. J., and Mercier, C. (2014). Effect of Tonic Pain on Motor Acquisition and Retention while Learning to Reach in a Force Field. PLoS One 9, e99159. doi:10.1371/journal.pone.0099159
Lewis, J. S., Kersten, P., McPherson, K. M., Taylor, G. J., Harris, N., McCabe, C. S., et al. (2010). Wherever Is My Arm? Impaired Upper Limb Position Accuracy in Complex Regional Pain Syndrome. Pain 149, 463–469. doi:10.1016/j.pain.2010.02.007
Mannerkorpi, K., and Iversen, M. D. (2003). Physical Exercise in Fibromyalgia and Related Syndromes. Best Pract. Res. Clin. Rheumatol. 17, 629–647. doi:10.1016/S1521-6942(03)00038-X
Martinez, E., Aira, Z., Buesa, I., Aizpurua, I., Rada, D., and Jatsu Azkue, J. (2018). Embodied Pain in Fibromyalgia: Disturbed Somatorepresentations and Increased Plasticity of the Body Schema 13, e0194534.doi:10.1371/journal.pone.0194534
Martínez, E., Guillen, V., Buesa, I., and Azkue, J. J. (2019). A Distorted Body Schema and Susceptibility to Experiencing Anomalous Somatosensory Sensations in Fibromyalgia Syndrome. Clin. J. Pain 35, 887–893. doi:10.1097/AJP.0000000000000754
Matamala-Gomez, M., Diaz Gonzalez, A. M., Slater, M., and Sanchez-Vives, M. V. (2019). Decreasing Pain Ratings in Chronic Arm Pain Through Changing a Virtual Body: Different Strategies for Different Pain Types. J. Pain 20, 685–697. doi:10.1016/j.jpain.2018.12.001
Mavromatis, N., Neige, C., Gagné, M., Reilly, K., and Mercier, C. (2017). Effect of Experimental Hand Pain on Training-Induced Changes in Motor Performance and Corticospinal Excitability. Brain Sci. 7, 15. doi:10.3390/brainsci7020015
McCabe, C. S., Cohen, H., Hall, J., Lewis, J., Rodham, K., and Harris, N. (2009). Somatosensory Conflicts in Complex Regional Pain Syndrome Type 1 and Fibromyalgia Syndrome. Curr. Rheumatol. Rep. 11, 461–465. doi:10.1007/s11926-009-0067-4
McDermid, A. J., Rollman, G. B., and McCain, G. A. (1996). Generalized Hypervigilance in Fibromyalgia: Evidence of Perceptual Amplification. Pain 66, 133–144. doi:10.1016/0304-3959(96)03059-X
Mcloughlin, M. J., Stegner, A. J., and Cook, D. B. (2011). The Relationship Between Physical Activity and Brain Responses to Pain in Fibromyalgia. J. Pain 12, 640–651. doi:10.1016/j.jpain.2010.12.004
McNally, J. D., Matheson, D. A., and Bakowsky, V. S. (2006). The Epidemiology of Self-Reported Fibromyalgia in Canada. Chronic Dis. Can. 27, 9–16.
Mercier, C., Gagné, M., Reilly, K., and Bouyer, L. (2016). Effect of Experimental Cutaneous Hand Pain on Corticospinal Excitability and Short Afferent Inhibition. Brain Sci. 6, 45. doi:10.3390/brainsci6040045
Moseley, G. L., Parsons, T. J., and Spence, C. (2008). Visual Distortion of a Limb Modulates the Pain and Swelling Evoked by Movement. Curr. Biol. 18, R1047–R1048. doi:10.1016/j.cub.2008.09.031
Parker, R. S., Lewis, G. N., Rice, D. A., and McNair, P. J. (2017). The Association Between Corticomotor Excitability and Motor Skill Learning in People With Painful Hand Arthritis. Clin. J. Pain 33, 222–230. doi:10.1097/AJP.0000000000000392
Queiroz, L. P. (2013). Worldwide Epidemiology of Fibromyalgia. Curr. Pain Headache Rep. 17, 356. doi:10.1007/s11916-013-0356-5
Roelofs, J., Sluiter, J. K., Frings-Dresen, M. H. W., Goossens, M., Thibault, P., Boersma, K., et al. (2007). Fear of Movement and (Re)injury in Chronic Musculoskeletal Pain: Evidence for an Invariant Two-Factor Model of the Tampa Scale for Kinesiophobia across Pain Diagnoses and Dutch, Swedish, and Canadian Samples. Pain 131, 181–190. doi:10.1016/j.pain.2007.01.008
Roosink, M., McFadyen, B. J., Hébert, L. J., Jackson, P. L., Bouyer, L. J., and Mercier, C. (2015). Assessing the Perception of Trunk Movements in Military Personnel with Chronic Non-specific Low Back Pain Using a Virtual Mirror. PLoS One 10, e0120251–14. doi:10.1371/journal.pone.0120251
Salomoni, S. E., Marinovic, W., Carroll, T. J., and Hodges, P. W. (2019). Motor Strategies Learned during Pain Are Sustained upon Pain-free Reexposure to Task. Med. Sci. Sports Exerc. 51, 2334–2343. doi:10.1249/MSS.0000000000002059
Toprak Celenay, S., Mete, O., Coban, O., Oskay, D., and Erten, S. (2019). Trunk Position Sense, Postural Stability, and Spine Posture in Fibromyalgia. Rheumatol. Int. 39, 2087–2094. doi:10.1007/s00296-019-04399-1
Turk, D. C., Robinson, J. P., and Burwinkle, T. (2004). Prevalence of Fear of Pain and Activity in Patients with Fibromyalgia Syndrome. J. Pain 5, 483–490. doi:10.1016/J.JPAIN.2004.08.002
Turton, A. J., McCabe, C. S., Harris, N., and Filipovic, S. R. (2007). Sensorimotor Integration in Complex Regional Pain Syndrome: A Transcranial Magnetic Stimulation Study. Pain 127, 270–275. doi:10.1016/j.pain.2006.08.021
Vlaeyen, J. W. S., Kole-Snijders, A. M. J., Rotteveel, A. M., Ruesink, R., and Heuts, P. H. T. G. (1995). The Role of Fear of Movement/(re)injury in Pain Disability. J. Occup. Rehab 5, 235–252. doi:10.1007/BF02109988
Vuralli, D., Ayyildiz, T., Bozdag, Y., Cengiz, B., and Gunendi, Z. (2019). P44-S Sensorimotor Integration in Fibromyalgia. Clin. Neurophysiol. 130, e107. doi:10.1016/j.clinph.2019.04.580
Wiederhold, B. K., Gao, K., Sulea, C., and Wiederhold, M. D. (2014). Virtual Reality as a Distraction Technique in Chronic Pain Patients. Cyberpsychology, Behav. Soc. Networking 17, 346–352. doi:10.1089/cyber.2014.0207
Witmer, B. G., and Singer, M. J. (1998). Measuring Presence in Virtual Environments: A Presence Questionnaire. Presence 7, 225–240. doi:10.1162/105474698565686
Wittkopf, P. G., Lloyd, D. M., and Johnson, M. I. (2018). The Effect of Visual Feedback of Body Parts on Pain Perception: A Systematic Review of Clinical and Experimental Studies. Eur. J. Pain 22, 647–662. doi:10.1002/ejp.1162
Wolfe, F., Clauw, D. J., Fitzcharles, M.-A., Goldenberg, D. L., Häuser, W., Katz, R. L., et al. (2016). 2016 Revisions to the 2010/2011 Fibromyalgia Diagnostic Criteria. Semin. Arthritis Rheum. 46, 319–329. doi:10.1016/j.semarthrit.2016.08.012
Keywords: upper limb, motor adaptation, altered feedback, chronic pain, kinesiophobia
Citation: Dagenais M, Brun C, Ohayon A and Mercier C (2021) Virtual Reality in Fibromyalgia: Does Altering Visual Feedback Impact on Pain and Movement During Reaching?. Front. Virtual Real. 2:681034. doi: 10.3389/frvir.2021.681034
Received: 15 March 2021; Accepted: 18 June 2021;
Published: 30 June 2021.
Edited by:
Ross Travers Smith, University of South Australia, AustraliaReviewed by:
Sheila A. M. Rauch, Emory University, United StatesCopyright © 2021 Dagenais, Brun, Ohayon and Mercier. This is an open-access article distributed under the terms of the Creative Commons Attribution License (CC BY). The use, distribution or reproduction in other forums is permitted, provided the original author(s) and the copyright owner(s) are credited and that the original publication in this journal is cited, in accordance with accepted academic practice. No use, distribution or reproduction is permitted which does not comply with these terms.
*Correspondence: Catherine Mercier, Y2F0aGVyaW5lLm1lcmNpZXJAcmVhLnVsYXZhbC5jYQ==
Disclaimer: All claims expressed in this article are solely those of the authors and do not necessarily represent those of their affiliated organizations, or those of the publisher, the editors and the reviewers. Any product that may be evaluated in this article or claim that may be made by its manufacturer is not guaranteed or endorsed by the publisher.
Research integrity at Frontiers
Learn more about the work of our research integrity team to safeguard the quality of each article we publish.