- Human-Computer Interaction Group, University of Würzburg, Würzburg, Germany
Psycho-pathological conditions, such as depression or schizophrenia, are often accompanied by a distorted perception of time. People suffering from this conditions often report that the passage of time slows down considerably and that they are “stuck in time.” Virtual Reality (VR) could potentially help to diagnose and maybe treat such mental conditions. However, the conditions in which a VR simulation could correctly diagnose a time perception deviation are still unknown. In this paper, we present an experiment investigating the difference in time experience with and without a virtual body in VR, also known as avatar. The process of substituting a person’s body with a virtual body is called avatar embodiment. Numerous studies demonstrated interesting perceptual, emotional, behavioral, and psychological effects caused by avatar embodiment. However, the relations between time perception and avatar embodiment are still unclear. Whether or not the presence or absence of an avatar is already influencing time perception is still open to question. Therefore, we conducted a between-subjects design with and without avatar embodiment as well as a real condition (avatar vs. no-avatar vs. real). A group of 105 healthy subjects had to wait for seven and a half minutes in a room without any distractors (e.g., no window, magazine, people, decoration) or time indicators (e.g., clocks, sunlight). The virtual environment replicates the real physical environment. Participants were unaware that they will be asked to estimate their waiting time duration as well as describing their experience of the passage of time at a later stage. Our main finding shows that the presence of an avatar is leading to a significantly faster perceived passage of time. It seems to be promising to integrate avatar embodiment in future VR time-based therapy applications as they potentially could modulate a user’s perception of the passage of time. We also found no significant difference in time perception between the real and the VR conditions (avatar, no-avatar), but further research is needed to better understand this outcome.
1 Introduction
Deviations of time perception can indicate important mental health problems. Psycho-pathological conditions, like depression or schizophrenia, are commonly associated with a disturbance of the sense of time, often described by the feeling of being “stuck in time” (Vogeley and Kupke, 2007). People with schizophrenia have difficulties estimating time intervals (Vogeley and Kupke, 2007) and a disturbance in the order of events (Giersch and Mishara, 2017). The succession of events and the relationships between events, aka time event-structure, which contributes to the sense of time continuity, is actually distorted for persons suffering from schizophrenia. Typically, information that occurs within windows of 20–50 ms is judged to be simultaneous, and such temporal simultaneity can help to group information together (Javitt and Freedman, 2015). Asynchrony helps us to distinguish successive events from one another, and to order them. However, patients with schizophrenia have difficulties in distinguishing sensory stimuli in time (Giersch and Mishara, 2017). Basic temporal structure is maintained by people with depression, but they report a slowing-down of the experienced passage of time (Vogeley and Kupke, 2007; Fuchs, 2013). Persons affected by such disorders are severely handicapped, since the feeling of time contributes to our well-being (Meissner and Wittmann, 2011; Droit-Volet et al., 2013; Wittmann, 2013). Although these psycho-pathological conditions can be detected, the diagnoses and therapies targeting a distorted time perception are difficult to realize.
Virtual Reality (VR) therapy has been proven to be efficient in treating a variety of specific psychological disorders, e.g., specific anxieties or post-traumatic stress disorders (Wallach et al., 2009; Gonçalves et al., 2012; Lindner, 2020). Most approaches regarding people suffering from schizophrenia or depression do not focus on the disturbed perception of time (Freeman et al., 2017). Patients in group therapies prefer the possibility of anonymization through an avatar (Dilgul et al., 2021). Hence it seems worthwhile to investigate the applicability of VR for the development of diagnostic and therapeutic methods based on time perception as depicted in Figure 1.
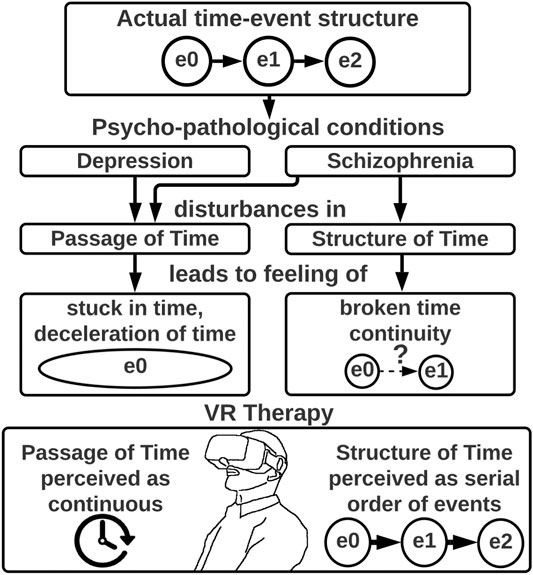
FIGURE 1. Psycho-pathological conditions effects on time perception and overall objective of a VR therapy for disturbed time perception.
However, such an envisioned usage of VR needs to first sanitize VR with respect to any potential impact on time perception per se and on healthy subjects. Prior work confirmed the existence of a perceived distance compression in VR (Grechkin et al., 2010). Since space and time are interdependent phenomena in human perception (Helson and King, 1931; Jones and Huang, 1982; Sarrazin et al., 2004), it is not unreasonable to hypothesize similar distortions to exist for the perceived time in VR, i.e., time compression or extension. It was also shown that the perception of time in VR can be influenced passively via the medium as a distraction (Schneider and Hood, 2007; Schneider et al., 2011) or actively by means of time influencers, or so-called zeitgebers (e.g., velocity of the course of the Sun) (Schatzschneider et al., 2016). “Zeitgebers are cues that help to locate oneself in time (of day) or to mark the passing of time, i.e., the speed of time” (Schatzschneider et al., 2016).
Substituting a person’s body with a virtual body significantly impacts the experience of perception in VR. Numerous studies demonstrated interesting perceptual, emotional, behavioral and psychological effects caused by embodiment (Peña et al., 2009; Spanlang et al., 2014). In addition, VR therapeutic applications tend to develop environments using different forms of embodiment (Shahnewaz et al., 2016; Hamzeheinejad et al., 2019; Kern et al., 2019).
Achieving plausible and believable virtual embodiment requires specific technical skills and expensive infrastructure, usually requiring full-body tracking and possibly haptic feedback (Spanlang et al., 2014). The use of advanced VR systems used in avatar embodiment studies is neither conceivable nor recommended in a medical practice with medical personnel who are not trained in the operation of the required technology. Technical problems can quickly render a VR experience detrimental. This should be avoided at all costs, especially for patients suffering from psychosis. Low-cost VR consumer systems such as the Oculus Quest™ seem to ease the technical complexity and hence operation of VR systems. Such systems and their head and hand tracking lack sufficient full-body tracking for a faithful avatar embodiment. Alternative approaches based on inverse kinematics do not yet solve this problem, as they are often still prone to incorrect movement predictions, which can lead to undesirable biases in perceptual, emotional, behavioral and psychological effects. At the moment, there is no simple or cost-aware approach to faithful movement reproduction. The actual need to support avatar embodiment is an important question to answer for future time-based VR therapy tools to determine whether it is worth investing research and development time in (extended) embodiment.
Lugrin et al. (2019) measured the estimation and judgement of a short waiting time in VR with or without avatar embodiment, and with different approaches to replicate an existing room (i.e., using a 360°-panoramic picture or rendered 3D models). The results showed that waiting time in VR can be perceived differently than in reality. Only differences in the perceived time between a real waiting situation and a waiting situation in VR without an avatar could be determined. This first results suggests a possible relation between avatar embodiment and time perception. However, no measures such as embodiment or presence were taken in the course of this study and only 15 participants per condition were evaluated. Taking into account previous work and its identified limitations this paper reports on a study focusing on the influence of embodiment on time perception. The approach focuses on the specific manipulation of embodiment as an independent variable, the collection of a substantial sample size and the use of a sufficiently long duration during VR exposure.
The next sections will summarize our contributions and main findings before presenting the related work and our approach. The experiment carried out is then described in detail, while the last part will discuss the overall results and limitations before concluding with our future work.
2 Contributions
We present a novel study investigating the influence of VR with and without embodiment on time perception of healthy people. We replicated and extended the experiment from Lugrin et al. (2019). We added additional measures related to presence and avatar embodiment and collected a larger sample. The experiment is reproducing a waiting task scenario, which is typical for time perception experiments (Osuna, 1985; Taylor, 1994; Zakay, 2014). However, we evaluated this task in a real and in a virtual condition. We let people wait in the real physical environment or, in VR inside a virtual environment replicating the real one. Our main finding is that the presence of an avatar in VR leads to a significantly faster perceived passage of time, whereby the participants felt the time going faster. Integrating avatar embodiment into future time-based VR therapy applications therefore seems promising, as it could potentially modulate the user’s perception of the passage of time. There was no significant difference in terms of time perception between the real and the VR conditions (avatar, no-avatar). This result contradicts the previous findings of Lugrin et al. (2019) therefore future work is needed to examine potential underlying factors.
3 Related Work
The definition of time is multifaceted and varies across many research areas, e.g., in physics, biology, philosophy and psychology. Physical Time and Psychological Time are two distinct concepts that are essential in understanding time perception. Physical Time is defined as a “non-spatial continuum in which events occur in apparently irreversible succession from the past through the present to the future” (The free dictionary, 2020). The Physical Time could then be understood as the time given and measured using clocks in the real world and is considered to be objective. The Psychological Time, in contrast, is often described as being subjective: “It refers to temporal dimensions such as duration, pace and the order of perceived and internal events” (Zakay, 2014). This sense of time co-constitutes our subjectivity (Kant, 1990) which we can experience as “stream of consciousness”—a continuous whole that passes over time (James et al., 1890). In this paper, we refer to this as “passage of time.” Time also structures the serial order of events enabling us to differentiate memory contents (past) from actual sensory experiences (present) to prepare goal-directed actions (future). We refer to this as “structure of time.”
3.1 Time Estimation and Time Perception
In the area of psychological time research, many models have been developed that attempt to describe the underlying processes of time perception. One of the most prominent models today is the Attentional-Gate Model (AGM) (Zakay and Block, 1995; Block and Zakay, 1996; Zakay and Block, 1997). The AGM describes a so-called pacemaker, which emits pulses continuously and at a fixed rate. This rate varies depending on the arousal level of a person. The number of pulses within a time interval are counted by the cognitive counter. However, the cognitive counter only registers impulses when attention is focused on time. The total number of impulses is then passed on to the working memory, which compares it with values in the retrospective memory already known from the past for certain periods of time. Consequently, the AGM implies that the resources of attention must always be shared between the focus on time itself and other events. The AGM is mainly used due to its heuristic value to describe the subjectively perceived time (Jokic et al., 2018).
In the area of psychological time research two methods exist to estimate time subjectively. One can let people estimate a certain interval of time, either prospectively or retrospectively. When estimating time prospectively, the person knows in advance that after a task or activity the elapsed time should be estimated. According to the AGM, the accuracy of the assessment is then mainly dependent on the attention given to time itself (Zakay and Block, 1995; Block and Zakay, 1996; Zakay and Block, 1997). In contrast, when estimating time retrospectively, people do not know in advance that they will be asked to estimate time at a later stage. Therefore, the estimation depends strongly on the information stored in memory (Ornstein, 1969; Block and Reed, 1978; Block, 1982; Block and Zakay, 1996). Two of the best known models that attempt to explain retrospective time estimation are the contextual-change model (Block and Reed, 1978; Block, 1982) and the Ornstein’s storage size hypothesis (Ornstein, 1969).
The contextual-change model states that the subjective passed time depends on the number of contextual changes stored in memory and the amount of information available at the moment of estimation (Block and Reed, 1978; Block, 1982). According to Ornstein (1969), the subjectively estimated time of a period of time depends on how much memory storage space is used for the events occurring in that period. It is generally assumed that depending on whether time is estimated prospectively or retrospectively, different cognitive processes are involved (Hicks et al., 1976; Block, 1992; Zakay, 1993). Especially the influence of attention on time differs between the two types of estimation (Zakay, 1993). In waiting situations one often unintentionally focuses on the time itself and therefore a time duration estimation of the interval is always prospective (Zakay, 2015). In the case of a longer waiting period, it is likely that in addition to the prospective mechanisms, the retrospective memory components will also influence the time duration judgement (Jokic et al., 2018). In general, the sense of time can change considerably in waiting situations.
This is often due to the sensation of wasting time (Osuna, 1985) or the unknown waiting duration (Taylor, 1994). These feelings usually have a negative connotation and are related to the perception of a slow passing time (Sackett et al., 2010). In this case persons tend to overestimate the actual waiting time, usually referred to as boredom (Zakay, 2014). If distractors like magazines are not available, people not only get more bored, but they also focus more on time itself, which leads to the experience of an even more slowed waiting time (Zakay and Block, 1997).
3.2 Time Studies in Virtual Reality
VR can be defined as “an approach that uses displays, tracking, and other technologies to immerse the user in a virtual environment” (LaViola, 2017). Such a VE is “a synthetic, spatial (usually 3D) world seen from a first-person point of view. The view in a virtual environment is under the real-time control of the user” (LaViola, 2017). Immersive VR embeds users in a three-dimensional environment and can elicit a sense of presence in this particular constructed world (Steuer, 1992; Bryson, 2013; Lelyveld and Entertainment, 2015). Often the sense of presence is also defined (at least partially) as the feeling of “being there” (Witmer and Singer, 1998; Lelyveld and Entertainment, 2015). Some contributions modify or extend this concept (Sanchez-Vives and Slater, 2005; Slater, 2009). They refer to the previously defined “presence” as place illusion, the illusion of being in the place depicted by the VE. The reality of the events taking place there is then defined as plausibility illusion.
A study provided a proof-of-concept by showing the influence on cognitive functions and time judgments of virtual Sun movements (Schatzschneider et al., 2016). It has been suggested that time could be scaled to a reference time, e.g., by accelerating or decelerating the time of visible clocks or increasing or decreasing the duration of a virtual day (Schatzschneider et al., 2016). A framework exploring ways to intentionally manipulate the perception of time in VR using zeitgebers was presented Landeck et al. (2020). Time travel illusion and its effects on people was also investigated, showing to have an impact on regretting bad decisions (Friedman et al., 2014; Pizarro et al., 2015). In this case, the time travel illusion is based on repeating the same scenario several times. You see your older self and its actions. One then has the possibility to manipulate the overall result based on the previously acquired knowledge. It was also shown, that fear does not affect time estimation in VR (Kitajima et al., 2020).
van der Ham et al. (2019) recently investigated the estimation of time duration by letting people watch video clips in VR and in the real world. They found no significant difference in the estimated time duration between VR and the real world. In addition, Bruder and Steinicke (2014) conducted a pilot study to investigate whether the time perception in the real world differs from that in VR. It was shown that VR including a walking task does not change the subjective time by itself. However, the surveyed ten persons only had to estimate time intervals between 2 and 5 s. They were also aware that they had to estimate time. In such prospective experimental designs, the experienced duration depends heavily on the amount of attention to time (Fleisig et al., 2009). If all attention resources are devoted to a task, factors such as boredom do not matter (Zakay, 2014). In other situations, such as a prolonged waiting situation, the results would probably be different. In such a situation, due to a lack of a task or distraction, attention would always be focused on the time itself and boredom could occur. Consequently, the results are not generally transferable. Lugrin et al. (2019) investigated such a waiting situation in which they made people wait 7.5 min in a room without distractors or a task. The results showed that people rate the waiting time duration in VR without embodiment higher than when waiting in reality. The absence of a virtual body could produce a deviation in time perception possibly related to reduced plausibility of the virtual environment.
If the quality of the simulation shows inconsistencies, the interest of the participants in the environment may increase (Latoschik et al., 2019) and could lead to an expanded perception of time. It is unclear why there was no difference between the VR with embodiment and VR without embodiment conditions, which should also differ in terms of plausibility. The experiment is limited, however, especially in the collection of important measures and the size of the sample. Neither embodiment nor presence was examined and only 15 subjects per condition were surveyed. Landau et al. (2020) showed that young adults who were embodied in a virtual body of a child using 180° stereoscopic video significantly overestimated the duration of the VR experience compared with a control group embodied in a virtual body of their own age. Furthermore, after exposing participants to a VR experience, Bansal et al. (2019) observed a novel relationship between action and event speed that resulted in a recalibration of time perception in a psychophysical task.
3.3 Avatars and Embodiment
The term “avatar embodiment” describes the process of substituting a person’s body with a virtual body in VR (Spanlang et al., 2014). This requires motion capturing of a user’s body that is used to animate an avatar representing oneself and that can be visualized in first-person perspective via a Head-Mounted Display (HMD) providing synchronized visuomotor feedback to the user (Gall and Latoschik, 2018). Research has clearly confirmed that embodiment substantially promotes immersion (Slater and Steed, 2000; Waltemate et al., 2018) and that typical psychophysical effects of embodied VEs (including presence, body ownership, emotional response, etc.,) are emphasized by high immersion and photo-realistic and personalized avatars (Achenbach et al., 2017; Latoschik et al., 2017; Waltemate et al., 2018). Avatar embodiment can also create a so-called Proteus effect (changing the way a person acts, thinks or feels) (Peña et al., 2009), e.g., by acting like a child (Banakou et al., 2013). Embodied VEs provide a promising and safe way to manipulate the perceived time duration, and can provide an important tool for a better understanding of human cognition. While full-body embodiment allows more experimental control, it may also work as a distractor itself. If avatar embodiment functions as a distractor, this could in turn lead to an underestimation of time. To enhance the overall sense of embodiment, according to Kilteni et al. (2012) the influencing subcomponents (feeling of self-localization, sense of agency, sense of body ownership) have to be addressed. The feeling of self-localization is improved by matching movements and a first-person perspective in relation to the artificial body. The sense of agency is enhanced in VR when motion is mapped to the virtual body in real-time or almost real-time. This is done by tracking the participant’s movements and transferring them to the movement of the virtual avatar. By using an inverse kinematics method (Yuan and Steed, 2010) only certain parts of the body have to be tracked. Several factors are known to increase the sense of body ownership, e.g., bottom-up factors like synchronous sensory information of the physical biological and the seen virtual avatar’s body are strong promoters. This can be achieved by visuotactile (e.g., haptics) or visuoproprioceptive (e.g., matching movements of the virtual avatar) feedback (Gonzalez-Franco et al., 2010). In addition, late research also identified the positive effect of realism and similarity on body ownership as top-down factors using photo-realistic and personalized avatars (Achenbach et al., 2017; Latoschik et al., 2017; Waltemate et al., 2018).
The close connection between the sense of time and self-consciousness is underpinned by studies showing a linkage to the sense of the internal state of the body, so-called interoception (Meissner and Wittmann, 2011; Droit-Volet et al., 2013; Wittmann, 2013; Pollatos et al., 2014). The temporal organization of perception and action, ranging from the processing of milliseconds of duration up to daily rhythms and beyond, has been studied extensively (Buhusi and Meck, 2005; Wittmann, 2016). With increasing time intervals affective and interoceptive bodily states play an important role (Craig, 2009; Droit-Volet et al., 2013; Wittmann, 2013).
3.4 Summary
So far, many factors have been identified that affect perception of time in real life, especially in waiting scenarios. In contrast, there is little evidence on the influence of embodiment on time perception in VR. Since embodiment can significantly influence the VR experience, as well as the cost and time of development and systems needed (i.e., requiring full body tracking or not), it is important to further investigate its possible impact on time perception.
4 Materials and Methods
To use VR as a potential diagnosis and therapy tool for people with a distorted sense of time, we want to address the following question: “Does immersive VR with the absence of an avatar already provoke any time perception deviation, e.g., a compression or extension, for healthy users?” For this purpose, we explore the impact of the Illusion of Virtual Body Ownership (IVBO) by omission or inclusion of an avatar on time perception. Our design follows the approach of Lugrin et al. (2019). We let people wait for 7.5 min in a room without any distractors or time indicators (Figure 2). We performed a between-subjects design with three conditions: 1) letting people wait in VR with an avatar (avatar) 2) letting people wait in VR without an avatar (no-avatar) 3) letting people wait in the real physical environment without the use of VR (real). We tackled prior limitations of Lugrin et al. (2019) by collecting additional measures and a larger sample. We also added a virtual mirror in the scene allowing participants to see a reflection of their avatar (Figure 3). A virtual mirror allows participant to view the reflection of their virtual human body moving as they move. Synchronous mirror reflection tends to elicit a higher subjective sense of ownership over the avatar (Gonzalez-Franco et al., 2010; Spanlang et al., 2014). Supplementary Video S1 shows both VR conditions.
It is important to understand that in the waiting situation where you wait for an unknown amount of time without any possible distractors or interaction, all conditions should elicit a slower perception of time (Osuna, 1985; Taylor, 1994), also referred to as boredom (Zakay, 2014).
However, we believe the absence of a virtual body will strongly alter the time perception during the waiting phase. The lack of a virtual body should significantly decrease the illusion of plausibility and place (Slater and Steed, 2000), as well as increase boredom (Osuna, 1985; Taylor, 1994; Zakay, 2014) and could therefore be connected to more discomfort and negative emotions resulting in a longer perceived waiting time (Angrilli et al., 1997; Droit-Volet et al., 2004).
Slater and Steed (2000) showed a positive relationship between presence and avatar embodiment in an immersive VE. The lack of an avatar should reduce presence. A result also confirmed by Gall and Latoschik (2018), in which participants experienced breaks in presence when not receiving synchronized visuomotor feedback regarding their body movement. A lower presence is also associated to a lower enjoyment (Sylaiou et al., 2010), meaning that participant without avatar should have a higher feeling of boredom. In addition, the lack of virtual body will prevent participants to interact with the simulation. In the avatar condition, participants at least have the possibility to control their virtual body and see it move as they move.
Moreover, the lack of a virtual body should increase discomfort and even eeriness because it creates an unusual and unnatural situation. In particular, because there is a conflict between proprioceptive and visual feedback. The participants immersed in VR can feel their real body, but they cannot see it (Gall and Latoschik, 2018). Previous work (Schwind et al., 2017) revealed that realistic human hands with missing fingers have negative effects such as fear of amputation, limb loss or feeling of losing body control. It also has been shown that the lack of a body makes correct estimations of space and distance/depth more difficult (Ries et al., 2008; Phillips et al., 2010)
The existence of a perceived distance compression in VR has already been verified in earlier work (Grechkin et al., 2010). Since time and space are considered to be interdependent phenomena in human perception, (Helson and King, 1931; Jones and Huang, 1982; Sarrazin et al., 2004), it is reasonable to assume that subjectively perceived time distortions could exist in VR. These could manifest themselves in the form of a subjectively perceived extension or compression of time. Therefore, we believe that the time perception in the real condition will differ from the VR conditions (avatar, no-avatar). On the one hand, it was already shown that VR as a medium can passively lead to an underestimation of time (Schneider and Hood, 2007; Schneider et al., 2011). On the other hand, in a waiting situation discomfort could become more apparent to the participants. Wearing a HMD can result in digital eye strain or symptoms related to its ergonomics, e.g., neck pain (Hirzle et al., 2021). This would result in negative emotions, which are related to the perception of a slow passing time (Angrilli et al., 1997; Droit-Volet et al., 2004). People would also potentially focus more on time itself and tend to overestimate the waiting time (Zakay and Block, 1997). Time perception could additionally be influenced by other factors such as the field of view, the refresh rate of the HMD and simulation frequency or the colors and the resolution of the images shown on the display (Brown, 1995; Kanai et al., 2006; Thönes et al., 2018).
In the case of time experiments in the range of minutes, both a time duration estimation and an assessment of the passage of time are recorded (Jokic et al., 2018; Lugrin et al., 2019), therefore we formulated the following hypotheses:
(H1) The estimation of the waiting duration will be longer in the no-avatar condition compared to the avatar condition.
(H2) The passage of time will be perceived slower in the no-avatar condition compared to the avatar condition.
(H3) The estimation of the waiting duration as well as the passage of time in the real condition will differ from the VR conditions (avatar, no-avatar).
4.1 Measures
All collected dependent variables are displayed in Table 1.
4.1.1 Simulator Sickness Questionnaire
Simulator sickness was assessed with the Simulator Sickness Questionnaire (SSQ) (Kennedy et al., 1993) before and after the experiment. It was used to check the quality of the VR simulation and to determine any negative effects that might have affected the results.
4.1.2 Inventory on Subjective Time, Self, Space
We also used the Inventory on Subjective Time, Self and Space (STSS) (Pfeifer et al., 2016; Jokic et al., 2018). Jokic et al. (2018) used a time arrow covering a range from 0 to 30 min to let participants estimate the waiting time. However, we slightly modified this question to make it independent of a time frame provided by an arrow and to avoid a central tendency bias. The adapted question is now based on Tobin et al. (2010): “Intuitively (without further thinking), how long do you think the waiting time lasted (in minutes and seconds)?.” To measure the intensity of consciousness of the physical self and space, two non-verbal image scales were used, containing response categories from 0 to 6.
We used the same questions as Jokic et al. (2018): “How intensively did you experience your body most of the time?” and “How intensively did you experience the surrounding space most of the time?.” Higher values imply a better consciousness of body and space.
In addition, three 100 mm-line visual analogue scales were presented to the participants, 1) “How often did you think about time?” (anchor points: not at all—extremely often), 2) “How fast did time pass for you?” (extremely slow—extremely fast), 3) “How much boredom did you experience most of the time?” (no boredom at all—extreme boredom)
4.1.3 Virtual Embodiment Questionnaire
The VEQ (Roth and Latoschik, 2019, 2020) is used to assess embodiment and is a further development of the Alpha-IVBO (Roth et al., 2017) and the original IVBO. It consists of the subscales “Ownership,” “Agency” and “Change.” Participants had to answer the corresponding questions at the end of the experiment in the avatar and no-avatar conditions. Each subscale has four items that are rated on a seven-point Likert scale (1–7).
4.1.4 Igroup Presence Questionnaire
Since presence and embodiment correlate with each other, we also assessed presence using the Igroup Presence Questionnaire (IPQ) (Schubert et al., 2001; Regenbrecht and Schubert, 2002; Schubert, 2003). after the waiting period. The IPQ consists of the subscales “General Presence,” “Spatial Presence,” “Involvement” and “Experienced Realism.” The items of the subscales are rated on a seven-point Likert scale (0–6). Due to the IPQ’s questions about the virtual environment (e.g., “Somehow I felt that the virtual world surrounded me.” or “I did not feel present in the virtual space.”), it was only collected for the avatar and no-avatar conditions and not for the real condition.
4.2 Design
We delimited the tracking area of our lab by curtains to create a real waiting room. There was only a carpet and a chair. This real waiting room environment was remodeled using 3D models. Since we wanted to create visual-haptic coherence when using an avatar, participants had to be able to touch the chair. To adjust the 3D model, we tracked the position of the real chair and adjusted the model’s size to the real chair’s dimensions. The provided avatar was selected depending on the gender of the participant and its size was scaled accordingly. In general, the subjects received a relatively simple human avatar (Figure 4). To match the avatar’s position and movements with those of the participant, feet, hips, hands and head were tracked. Based on the positions of these, the positions of the remaining body parts were calculated using inverse kinematics. Neither an avatar nor the tracking devices were displayed in the no-avatar condition. During first tests, some participants reported that they sometimes closed their eyes. Since closing the eyes for a longer duration could influence the manipulation of embodiment, we used the VIVE Pro Eye to add another sanity check. The integrated eye tracker allows for a recording of the time frames during which the participants closed their eyes.
4.3 Procedure
Participants were recruited for a VR experiment. None of them were aware of the real goal of this experiment. Figure 5 visualizes the structure of a 13-stage long experimental session. First, participants were welcomed by the experimenter and received a consent form. Once they signed it, they filled a demographic questionnaire and the SSQ. Pupil distance was measured and set on the headset. We ensured that no distractors such as cell phones or watches were available. For ensuring comparability between the conditions, the VR equipment was also attached in the real condition. Once all VR devices were attached, the participant was asked to stand still for the calibration of the avatar. After the calibration, the participants had to sit on the chair behind them. For the avatar and no-avatar conditions the corresponding VE was started. In the real condition the HMD was removed. Then the experimenter pretended that something was not working properly and needed the help of a colleague. While the colleague is called in, the participants should hold the controllers in their hands and remain seated on the chair. The experimenter left the room and waited 7.5 min in an adjacent room. The experimenter observed the subject through a camera to be able to stop the experiment if it appeared that the participant was suffering from any discomfort. After 7.5 min the experimenter came back and apologized for the inconvenience. First, the HMD and the controllers were removed from the test person. In the avatar and no-avatar conditions the participants then filled the modified STSS, the SSQ, the VEQ and the IPQ during the “Post Questionnaires” phase. In the real condition, they only filled in the modified STSS and SSQ, as most of the IPQ and VEQ questions cannot be answered adequately without having been in the VR before. To avoid revealing the end of the study, the trackers remained attached. After the questionnaires were completed, we removed the trackers. Finally, participants got the information about the real aim of the experiment and were asked about general feedback. On average, each experimental session lasted 25 min.
4.4 Software and Hardware
The VE was developed using the Unreal Engine 4™. For the correct animation and calibration of the avatar IKinema Orion and the corresponding plugin for the Unreal Engine 4™ were used. The hardware setup consisted of a stationary PC (Intel Core i7-6700k 4.0 GHz CPU, 16 GB of RAM, Nvidia GeForce GTX 1080 Graphics card). On this machine, the application runs on average with 90 fps. The end-to-end latency was measured in two ways. Once by manually counting frames. This was done using a high-speed camera that captured a 240 fps video of a 60 Hz screen, showing the movement of motion tracked controllers. We also measured the latency using a sine fitting approach (Stauffert et al., 2020). The latency was on average 48 or 42 ms depending on the method. These values are sufficient for a VR application (Carmack, 2013; Waltemate et al., 2015).
The participants were immersed in the VE using the HTC VIVE™ Pro. The corresponding HMD has a resolution of
4.5 Participants
A total of 121 participants were recruited for the study. Sixteen participants had to be excluded due to technical issues or since they did not follow the protocol. The mean age of the remaining 105 people (73 females, 32 males) was 24.14 years (SD = 7.65). 67 participants had prior experience with VR and 96 were native speakers. None of the subjects were visually impaired or had to be excluded because of simulator sickness or for closing their eyes too often.
4.6 Statistical Analysis
All STSS items as well as the IPQ ratings and VEQ ratings were analyzed on the interval measurement scale (Boone and Boone, 2012; Pfeifer et al., 2016; Jokic et al., 2018). When normality and variance equality were given, each STSS item was analyzed with a one-way Anova. Normality was verified by the Shapiro-Wilk test and variance homogeneity by the Levene’s test. Since the Anova is quite robust to violations of normality (Glass et al., 1972; Lix et al., 1996; Blanca et al., 2017), especially with a large number of subjects (n = 105), we performed an Anova, even though the assumption did not hold. In case variance equality could not be assumed, we performed a Welch Anova. The effect size is indicated by Eta squared
For each IPQ and VEQ item independent t-tests between groups were performed if normality and variance homogeneity held true. Normality was checked using the Shapiro-Wilk test and variance homogeneity applying the F-test. In case normality could not be assumed, a Mann-Whitney U test was used. If only the variance homogeneity assumption held not true, a Welch–Satterthwaite equation was applied. The effect size is indicated by Cohen’s d. The results of the IPQ and the VEQ are shown in Table 3.
Additionally, three one-sample Wilcoxon signed rank tests were performed to analyze whether either of the three conditions deviates from the objectively measured 7.5 min.
4.7 Results
4.7.1 Inventory on Subjective Time, Self, Space
The outcome of the Levene’s test indicate that variance homogeneity can be considered for all STSS items. Normality could not be assumed for any of the STSS items. The results show no significant difference between the estimated waiting time between the conditions (Figure 6). Of the three visual analogue scales of the STSS, only time passing showed a significant difference (Figure 7). In the avatar condition time was experienced to pass faster than in the no-avatar condition. Regarding the deviation from the objective time, there was no difference between the avatar condition (
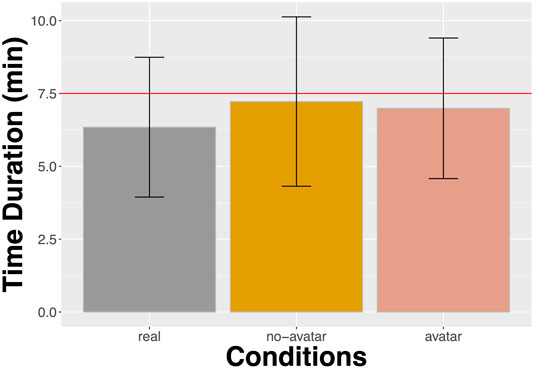
FIGURE 6. Waiting time duration estimation per condition, with standard deviation bars. Red line indicates objective waiting time.
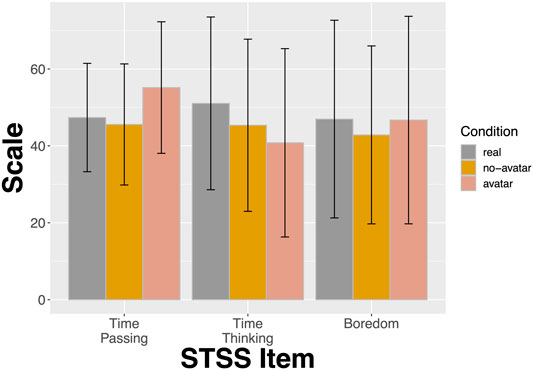
FIGURE 7. Score on the STSS items “Time Passing,” “Time Thinking” and “Boredom” in the no-avatar and avatar condition, with standard deviation bars.
4.7.2 Igroup Presence Questionnaire
The IPQ was only analyzed for differences between the avatar and no-avatar conditions. The results of the F-tests show that variance homogeneity could be assumed for all IPQ subscales but “Experienced Realism.” All but “General Presence” can be considered normally distributed. There was a significant difference in the IPQ “Spatial Presence” scores. The IPQ subscale “Involvement” scores did also significantly differ.
4.7.3 Virtual Embodiment Questionnaire
The VEQ was only analyzed for differences between the avatar and no-avatar conditions. With regard to the subscales of the VEQ, variance homogeneity can only be considered for “Ownership.” The results of the Shapiro-Wilk tests show that normality can not be assumed for any of the subscales. The “Ownership,” the “Agency” and the “Change” subscale scores were significantly higher in the avatar condition.
4.8 Discussion
We measured significantly higher IVBO values for the avatar condition compared to the no-avatar condition, thus confirming a successful manipulation check of our independent variable embodiment. The results also confirm our H2 and reject our H1.
We expected the estimation of the waiting duration to be longer in the no-avatar condition compared to the avatar condition (H1). In terms of presence, the values of the IPQ subscales “Spatial Presence” and “Involvement” were significantly higher in the avatar condition than in the no-avatar condition. “General Presence” and “Experienced Realism” did not differ significantly. Since a positive relationship between presence and body movement in an immersive VE was shown by Slater and Steed (2000), one could expect that all IPQ subscales would differentiate between the two groups. In addition to embodiment, we enhanced presence by matching visual and haptic feedback (Gall and Latoschik, 2018). This could have led to the fact that the “General Presence” subscale, which asks for the feeling of being present in the virtual world, did not differ significantly between the avatar and no-avatar condition. With regard to “Experienced Realism,” no differences between the conditions could probably be identified, as all participants were in the same VE. The significantly lower Spatial Presence and Involvement measured in the condition without an avatar seem to confirm that the illusion of place and plausibility (Sanchez-Vives and Slater, 2005; Slater, 2009) were not present. However, it seems that being able to interact with one’s avatar and see it in the mirror did not make the situation any less boring. This is supported by the STSS item boredom, which shows no significant difference between the conditions. Although presence and plausibility were lower in the no-avatar condition, no difference in time duration estimation was observed and H1 was rejected.
Regarding our H2, we expected a difference in the passage of time. Participants in the no-avatar condition would perceive a slower passage of time compared to the avatar condition (H2). The STSS item asking “How fast did time pass for you?” did reveal a significant difference between the conditions. As mentioned before presence and plausibility differed between the conditions. Even though the avatar did not make the waiting situation any less boring, H2 was confirmed. Although the people were able to move freely and received proprioceptive and haptic feedback, they did not see their own bodies. As a result, they may have become more focused on the position of their real body. The presence of the mirror might have intensified this effect, making the presence or absence of a virtual body inevitably noticeable. It could also be related to emotional discomfort, but we didn’t specifically access that kind of comfort. Our expectation that the real condition would differ from the avatar condition in terms of both time duration estimation and passage of time H3 could not be confirmed and has to be rejected.
Considering the time duration estimations, the waiting time in the real condition did not significantly differ from the VR conditions (avatar, no-avatar), but was on average more than 30 s shorter. It seems that VR as a medium itself did not act as a distractor. This is supported by the fact that there was no significant difference in terms of boredom between the conditions. The time was underestimated on average in all conditions, which is quite surprising. In particular, in the real condition, we expected duration estimations to be similar to Jokic et al. (2018) rather than Lugrin et al. (2019) due to the sample size of the studies. On average, however, people in our study estimated the waiting duration about 2 min shorter than Jokic et al. (2018) and about 50 s longer than Lugrin et al. (2019).
A possible explanation could be the nature of the waiting scenario. Similar to Lugrin et al. (2019) a technical problem was simulated, which created a kind of “accidental” waiting scenario. According to Minsky (1974) a typical waiting situation could be seen as a “frame.” A “frame” can be defined as “a data-structure for representing a stereotyped situation” (Minsky, 1974). A frame representing information about a waiting scenario probably includes some sort of a room with a door, a window, some uncomfortable chairs across the walls and a table. You also are prepared to wait for a specific event you are aware of beforehand, e.g., waiting at the doctor.
Looking at the study done by Jokic et al. (2018) their design fits the waiting room “frame.” People were waiting in a stereotypical room for an experiment to start. However, the “accidental” waiting scenario was neither in a typical room nor was it a typical situation. People were sitting on a comfortable chair in an area delimited by black curtains and the experiment they signed up for already started. The deviation from a typical waiting room scenario was necessary in this case due to the use of VR and the calibration of the avatar. The difference between the subjects’ expectations of a typical waiting room and the actual waiting room may have increased their awareness. They then did not focus on time itself but on the current situation, resulting in an underestimation of the waiting time duration (Zakay and Block, 1997; Zakay, 2015).
Additionally the fact that people were sitting on a comfortable chair during the waiting time could led to a rather relaxed situation. On the one hand the lowest subjectively estimated time in the real condition could then be due to the fact that for the other conditions the subjects were wearing a HMD which could have led to digital eye strain or HMD ergonomic related symptoms to be more apparent. In the real condition, on the other hand, they could rather lean back on the chair and sink into thoughts, which leads to an underestimation of time (Thönes and Wittmann, 2016). This would also explain why only the real condition did significantly differ from the objective 7.5 min.
However, the explanation could also be much simpler. Participants might embellished their time duration judgements to gloss over the delay at the start of the study for the benefit of the experimenter. When asked if the respective person had to wait too long, most participants answered that the delay was not a problem.
In terms of time passing, there was also no significant difference between the real and the VR conditions (avatar, no-avatar). However, there is a tendency that people in the real condition report a slower passage of time compared to the avatar condition. As mentioned before boredom did not significantly differ between the conditions. There was also no significant difference regarding time thinking, but you can see a tendency that people in the real condition tend to focus more on time itself compared to the avatar condition. A potential connection between the passage of time and time thinking should therefore be observed more closely in future experiments.
In summary, embodiment between the avatar and the no-avatar condition was significantly different and our hypothesis H2 that time is perceived slower in VR without an avatar could be confirmed. In contrast, time duration estimates of participants did not differ significantly between the avatar and no-avatar condition and our H1 was rejected. Furthermore, the real condition did not differ from the VR conditions (avatar, no-avatar) in terms of estimating the duration or passage of time and H3 was also rejected.
Why the avatar condition differs from the no-avatar condition with regard to the passage of time but not with regard to the time duration estimation could be due to the kind of manipulation. Our results show a significantly lower Spatial Presence and Involvement in the condition without an avatar thus seemingly confirming that the illusion of place and plausibility were not present. Wearden (2015) argues that time duration estimation and passage of time must be considered separately. While both seem to be influenced by similar factors (Jokic et al., 2018), previous studies also showed that time duration can be influenced without influencing time passage and vice versa (Wearden et al., 1998; Wearden, 2008). In particular, the lack of the two illusions could therefore be a factor that has only or at least a stronger influence on the time passage than on the time duration estimation.
With regard to the results identified by Lugrin et al. (2019), we argue that the integration of a virtual mirror to strengthen any embodiment effects, renders a direct comparison between the experiments invalid. However, both experiments show similar effects of a time perception deviation for certain conditions. Our empirical results do not allow for the reasons to be explicitly pinpointed but they generate enough results for an informed speculation about the common reason. The conditions under which the time perception deviation was detected were also characterized by different aspects that seemed to have influenced the plausibility of the environment. The affected conditions did not include an avatar which becomes even more apparent as a break in plausibility in the our experiment with the additional mirror where participants did not see themselves in the no-avatar condition. This in turn could be associated with more discomfort and negative emotions, leading to a longer perceived waiting time (Angrilli et al., 1997; Droit-Volet et al., 2004). Lugrin et al. (2019) did not identify the same deviation effect for the 360°-panoramic picture without avatar condition. It can potentially be explained by the low-realistic rendering (no real depth-cues besides head rotations, e.g., no stereo or motion parallax) which—in itself—maybe is of such a lower plausibility that the missing avatar does not really make a big difference anymore.
Even though there was no significant difference between the real condition and the VR conditions (avatar, no-avatar) in terms of the perception of time, there is still an interesting tendency. Although having an avatar in VR should be more closer to reality, the real condition was actually closer to the no-avatar condition than to the avatar condition in terms of the feeling of passage of time. Since the results are not consistent with Jokic et al. (2018) and Lugrin et al. (2019), more research is needed to better understand the underlying factors.
4.9 Limitations
Only healthy people participated and therefore our results are limited in terms of transferability to people with a disturbed sense of time. The sample also contained more women than men, which could have led to a bias. We only used a generic male and female avatar model in our studies. The passage of time could further be affected by the avatar appearance (Latoschik et al., 2016; Roth et al., 2016), e.g., providing a photo-realistic and personalized avatar (Waltemate et al., 2018). Such an avatar would not only lead to a higher avatar acceptance, but also result in an increased plausibility of the VR. This potentially leads to an even faster passage of time. To validate this assumption, a future study needs to provide personalized avatars and analyze their effects on the perceived passage of time. We also did not directly measure discomfort, which should be recorded in future experiments.
5 Future Work
Overall, we believe that our results represent a first step towards successful diagnosis and treatment of psycho-pathological conditions in VR based on the measurement and manipulation of time perception. Our future work is divided in four main research directions. The first step is to replicate our experiment with a clinical population.
We also would like to extend our experiment by including a variable foreperiod task with and without virtual body as first starting point to develop a diagnostic and treatment tool for individuals with schizophrenia. This variable foreperiod task consists in measuring reaction time when one waits for a red light to turn green. At a variable interval, a target is displayed on the screen and participants must confirm its appearance by pressing a response key quickly. With increasing interval duration, the reaction times to recognize the target become faster (Niemi and Näätänen, 1981). The acceleration of reaction times at longer intervals is referred to as the “variable foreperiod effect” and reflects the increasing expectation over time. This probability increasing with time is referred to as the “hazard function” and can be used to dynamically generate and update temporal predictions. Martin et al. (2017) showed that this predictive expectation appears to be disrupted in patients with schizophrenia. Our results could then play an important role in the choice of embodiment when replicating the variable foreperiod task in VR.
As an alternative, approaches in the direction of mindfulness could be explored. Mindfulness can be defined as “the awareness that emerges through paying attention on purpose, in the present moment, and nonjudgmentally to the unfolding of experience moment by moment” (Kabat-Zinn, 2003). It has been shown that mindfulness can be associated with time perception and a higher level of mindfulness can lead to a faster passage of time (Weiner et al., 2016). There are already quite a few therapeutic approaches in VR that rely on mindfulness meditation (Döllinger et al., 2021). People slowly drift down a computer-generated river in VR (Nararro-Haro et al., 2016; Gomez et al., 2017; Navarro-Haro et al., 2019) or walk through a forest (Gromala et al., 2015). The use of an avatar in such scenarios could potentially lead to an even faster perceived passage of time and counteract the slowed perception of time reported by patients.
Our next main research direction is to investigate active manipulations of time influencers in VR, as performed by Schatzschneider et al. (2016). According to Brown (1995), faster speeds lengthened perceived time to a greater degree than slower speeds and intervals associated with more changes are perceived to be longer than intervals with fewer changes. By manipulating the velocity, frequency and amount of visual stimuli, such as the arm of a clock as an artificial zeitgeber, the manipulation could lead to a counterbalance to the feeling of time slowing down, potentially leading to improved patient well-being. We would like to extend the possibility to influence time perception with different avatar embodiment and disembodiment variation.
6 Conclusion
This article investigated the influence of avatar embodiment on time perception of healthy people in VR. Subjects had to wait seven and a half minutes 1) with an avatar in VR (avatar) 2) without an avatar in VR (no-avatar) 3) or in the real physical environment (real) in a room without distractors or time indicators. After the waiting period, participants were asked to estimate the waiting time duration and to describe their experience of the passage of time. Our main result indicates that an avatar leads to a faster perceived passage of time. There was also no significant difference in time perception between the real and the VR conditions (avatar, no-avatar), but additional work is needed to better understand the underlying factors.
Based on our results, an integration of avatar embodiment into future time-based VR therapy applications appears to be promising, as it could potentially moderate the user’s perception of the passage of time. Our future work will focus on replicating and extending our experiment with actual patients suffering from psycho-pathological conditions. To get one step closer to a concrete solution for a time-based VR therapy, a replication of the variable foreperiod task in VR or the use of a mindfulness meditation environment utilizing different forms of avatar embodiment such as no-avatar, partial-avatar, and avatar, could be a promising first starting point.
Data Availability Statement
The raw data supporting the conclusion of this article will be made available by the authors, without undue reservation.
Ethics Statement
The studies involving human participants were reviewed and approved by Ethikkommission des Institut Mensch-Computer-Medien (Universität Würzburg). The patients/participants provided their written informed consent to participate in this study.
Author Contributions
FU, ML, J-LL, and MEL contributed to conception and design of the study. FU and ML developed the software and performed the statistical analyzes. FU wrote the first draft of the manuscript. J-LL and MEL were the project administrators and supervised the work. All authors contributed to manuscript revision, read, and approved the submitted version.
Funding
This work is funded by the VIRTUALTIMES project (ID-824128) funded by the European Union under the Horizon 2020 program. This publication was supported by the Open Access Publication Fund of the University of Würzburg.
Conflict of Interest
The authors declare that the research was conducted in the absence of any commercial or financial relationships that could be construed as a potential conflict of interest.
Supplementary Material
The Supplementary Material for this article can be found online at: https://www.frontiersin.org/articles/10.3389/frvir.2021.658509/full#supplementary-material
References
Achenbach, J., Waltemate, T., Latoschik, M. E., and Botsch, M. (2017). “Fast Generation of Realistic Virtual Humans,” in Proceedings of the 23rd ACM Symposium on Virtual Reality Software and Technology, Gothenburg, Sweden, November 8–10, 2017 (ACM), 12.
Angrilli, A., Cherubini, P., Pavese, A., and Manfredini, S. (1997). The Influence of Affective Factors on Time Perception. Perception & Psychophysics 59, 972–982. doi:10.3758/bf03205512
Banakou, D., Groten, R., and Slater, M. (2013). Illusory Ownership of a Virtual Child Body Causes Overestimation of Object Sizes and Implicit Attitude Changes. Proc. Natl. Acad. Sci. 110, 12846–12851. doi:10.1073/pnas.1306779110
Bansal, A., Weech, S., and Barnett-Cowan, M. (2019). Movement-contingent Time Flow in Virtual Reality Causes Temporal Recalibration. Sci. Rep. 9, 4378–4413. doi:10.1038/s41598-019-40870-6
Blanca, M. J., Alarcón, R., Arnau, J., Bono, R., and Bendayan, R. (2017). Non-normal Data: Is Anova Still a Valid Option?. Psicothema 29, 552–557. doi:10.7334/psicothema2016.383
Block, R. A. (1992). “Prospective and Retrospective Duration Judgment: The Role of Information Processing and Memory,” in Time, Action and Cognition (Dordrecht: Springer), 141–152. doi:10.1007/978-94-017-3536-0_16
Block, R. A., and Reed, M. A. (1978). Remembered Duration: Evidence for a Contextual-Change Hypothesis. J. Exp. Psychol. Hum. Learn. Mem. 4, 656–665. doi:10.1037/0278-7393.4.6.656
Block, R. A. (1982). Temporal Judgments and Contextual Change. J. Exp. Psychol. Learn. Mem. Cogn. 8, 530–544. doi:10.1037/0278-7393.8.6.530
Block, R. A., and Zakay, D. (1996). Models of Psychological Time Revisited. Time and mind 33, 171–195.
Brown, S. W. (1995). Time, Change, and Motion: The Effects of Stimulus Movement on Temporal Perception. Perception & Psychophysics 57, 105–116. doi:10.3758/bf03211853
Bruder, G., and Steinicke, F. (2014). “Time Perception during Walking in Virtual Environments,” in 2014 IEEE Virtual Reality (VR), Minneapolis, MN, March 29–April 2, 2014 (IEEE), 67–68.
Bryson, S. (2013). Virtual Reality: A Definition History-A Personal Essay. arXiv. preprint (arXiv:1312.4322).
Buhusi, C. V., and Meck, W. H. (2005). What Makes Us Tick? Functional and Neural Mechanisms of Interval Timing. Nat. Rev. Neurosci. 6, 755–765. doi:10.1038/nrn1764
Carmack, J. (2013). Latency Mitigation Strategies. Available at: https://danluu.com/latency-mitigation/.
Craig, A. D. (2009). Emotional Moments across Time: a Possible Neural Basis for Time Perception in the Anterior Insula. Phil. Trans. R. Soc. B 364, 1933–1942. doi:10.1098/rstb.2009.0008
Dilgul, M., Hickling, L. M., Antonie, D., Priebe, S., and Bird, V. J. (2021). Virtual Reality Group Therapy for the Treatment of Depression: A Qualitative Study on Stakeholder Perspectives. Front. Virtual Real. 1, 42. doi:10.3389/frvir.2020.609545
Döllinger, N., Wienrich, C., and Latoschik, M. E. (2021). Challenges and Opportunities of Immersive Technologies for Mindfulness Meditation: A Systematic Review. Front. Virtual Reality 2, 29. doi:10.3389/frvir.2021.644683
Droit-Volet, S., Brunot, S., and Niedenthal, P. (2004). Brief Report Perception of the Duration of Emotional Events. Cogn. Emot. 18, 849–858. doi:10.1080/02699930341000194
Droit-Volet, S., Fayolle, S., Lamotte, M., and Gil, S. (2013). Time, Emotion and the Embodiment of Timing. Timing Time Percept 1, 99–126. doi:10.1163/22134468-00002004
Fleisig, D., Ginzburg, K., and Zakay, D. (2009). A Model of Waiting’s Duration Judgment. NeuroQuantology 7, 58–65. doi:10.14704/nq.2009.7.1.207
Freeman, D., Reeve, S., Robinson, A., Ehlers, A., Clark, D., Spanlang, B., et al. (2017). Virtual Reality in the Assessment, Understanding, and Treatment of Mental Health Disorders. Psychol. Med. 47, 2393–2400. doi:10.1017/s003329171700040x
Friedman, D., Pizarro, R., Or-Berkers, K., Neyret, S. n., Pan, X., and Slater, M. (2014). A Method for Generating an Illusion of Backwards Time Travel Using Immersive Virtual Reality—an Exploratory Study. Front. Psychol. 5, 943. doi:10.3389/fpsyg.2014.00943
Fuchs, T. (2013). Temporality and Psychopathology. Phenom Cogn. Sci. 12, 75–104. doi:10.1007/s11097-010-9189-4
Gall, D., and Latoschik, M. E. (2018). “The Effect of Haptic Prediction Accuracy on Presence,” in 2018 IEEE Conference on Virtual Reality and 3D User Interfaces, Tuebingen/Reutlingen, Germany, March 18–22, 2018 (IEEE), 73–80.
Giersch, A., and Mishara, A. L. (2017). Is Schizophrenia a Disorder of Consciousness? Experimental and Phenomenological Support for Anomalous Unconscious Processing. Front. Psychol. 8, 1659. doi:10.3389/fpsyg.2017.01659
Glass, G. V., Peckham, P. D., and Sanders, J. R. (1972). Consequences of Failure to Meet Assumptions Underlying the Fixed Effects Analyses of Variance and Covariance. Rev. Educ. Res. 42, 237–288. doi:10.3102/00346543042003237
Gomez, J., Hoffman, H. G., Bistricky, S. L., Gonzalez, M., Rosenberg, L., Sampaio, M., et al. (2017). The Use of Virtual Reality Facilitates Dialectical Behavior Therapy "Observing Sounds and Visuals" Mindfulness Skills Training Exercises for a Latino Patient with Severe Burns: A Case Study. Front. Psychol. 8, 1611. doi:10.3389/fpsyg.2017.01611
Gonzalez-Franco, M., Perez-Marcos, D., Spanlang, B., and Slater, M. (2010). “The Contribution of Real-Time Mirror Reflections of Motor Actions on Virtual Body Ownership in an Immersive Virtual Environment,” in 2010 IEEE virtual reality conference (VR), Boston, MA, March 20–24, 2010 (IEEE), 111–114.
Gonçalves, R., Pedrozo, A. L., Coutinho, E. S. F., Figueira, I., and Ventura, P. (2012). Efficacy of Virtual Reality Exposure Therapy in the Treatment of Ptsd: a Systematic Review. PloS one 7, e48469. doi:10.1371/journal.pone.0048469
Grechkin, T. Y., Nguyen, T. D., Plumert, J. M., Cremer, J. F., and Kearney, J. K. (2010). How Does Presentation Method and Measurement Protocol Affect Distance Estimation in Real and Virtual Environments?. ACM Trans. Appl. Perception 7, 26. doi:10.1145/1823738.1823744
Gromala, D., Tong, X., Choo, A., Karamnejad, M., and Shaw, C. D. (2015). “The Virtual Meditative Walk: Virtual Reality Therapy for Chronic Pain Management,” in Proceedings of the 33rd Annual ACM Conference on Human Factors in Computing Systems, Seoul, Republic of Korea, April 18–23, 2015, 521–524.
Hamzeheinejad, N., Roth, D., Götz, D., Weilbach, F., and Latoschik, M. E. (2019). “Physiological Effectivity and User Experience of Immersive Gait Rehabilitation,” in The First IEEE VR Workshop on Applied VR for Enhanced Healthcare, Osaka, Japan, March 23–27, 2019 (IEEE), 1421–1429.
Helson, H., and King, S. M. (1931). The Tau Effect: an Example of Psychological Relativity. J. Exp. Psychol. 14, 202–217. doi:10.1037/h0071164
Hicks, R. E., Miller, G. W., and Kinsbourne, M. (1976). Prospective and Retrospective Judgments of Time as a Function of Amount of Information Processed. Am. J. Psychol. 89, 719–730. doi:10.2307/1421469
Hirzle, T., Cordts, M., Rukzio, E., Gugenheimer, J., and Bulling, A. (2021). “A Critical Assessment of the Use of Ssq as a Measure of General Discomfort in Vr Head-Mounted Displays,” in Proceedings of the 2021 ACM CHI Virtual Conference on Human Factors in Computing Systems-CHI, Yokohama, Japan, May 8–13, 2021, 8–13.
James, W., Burkhardt, F., Bowers, F., and Skrupskelis, I. K. (1890). The Principles of Psychology, 1. Macmillan London.
Javitt, D. C., and Freedman, R. (2015). Sensory Processing Dysfunction in the Personal Experience and Neuronal Machinery of Schizophrenia. Ajp 172, 17–31. doi:10.1176/appi.ajp.2014.13121691
Jokic, T., Zakay, D., and Wittmann, M. (2018). Individual Differences in Self-Rated Impulsivity Modulate the Estimation of Time in a Real Waiting Situation. Timing & Time Perception 6, 71–89. doi:10.1163/22134468-00002101
Jones, B., and Huang, Y. L. (1982). Space-time Dependencies in Psychophysical Judgment of Extent and Duration: Algebraic Models of the Tau and Kappa Effects. Psychol. Bull. 91, 128–142. doi:10.1037/0033-2909.91.1.128
Kabat-Zinn, J. (2003). Mindfulness-based Interventions in Context: Past, Present, and Future. Clin. Psychol. Sci. Pract. 10, 144–156. doi:10.1093/clipsy/bpg016
Kanai, R., Paffen, C. L. E., Hogendoorn, H., and Verstraten, F. A. J. (2006). Time Dilation in Dynamic Visual Display. J. Vis. 6, 8. doi:10.1167/6.12.8
Kant, I. (1990). Critique of Pure Reason. Buffalo, NY: Prometheus Books. (Original work published 1781).
Kennedy, R. S., Lane, N. E., Berbaum, K. S., and Lilienthal, M. G. (1993). Simulator Sickness Questionnaire: An Enhanced Method for Quantifying Simulator Sickness. Int. J. aviation Psychol. 3, 203–220. doi:10.1207/s15327108ijap0303_3
Kern, F., Winter, C., Gall, D., Käthner, I., Pauli, P., and Latoschik, M. E. (2019). “Immersive Virtual Reality and Gamification within Procedurally Generated Environments to Increase Motivation during Gait Rehabilitation,” in 2019 IEEE Conference on Virtual Reality and 3D User Interfaces (VR), Osaka, Japan, March 23–27, 2019, 500–509.
Kilteni, K., Groten, R., and Slater, M. (2012). The Sense of Embodiment in Virtual Reality. Presence: Teleoperators and Virtual Environments 21, 373–387. doi:10.1162/pres_a_00124
Kitajima, M., Kanari, K., and Sato, M. (2020). “An Examination of Effects of Fear Using Vr Content on Time Estimation,” in 26th ACM Symposium on Virtual Reality Software and Technology, Virtual Event, Canada, November 1–4, 2020.
Landau, D. H., Hasler, B. S., and Friedman, D. (2020). Virtual Embodiment Using 180° Stereoscopic Video. Front. Psychol. 11, 1229. doi:10.3389/fpsyg.2020.01229
Landeck, M., Unruh, F., Lugrin, J.-L., and Latoschik, M. E. (2020). “Metachron: A Framework for Time Perception Research in Vr,” in 26th ACM Symposium on Virtual Reality Software and Technology, Virtual Event, Canada, November 1–4, 2020 (New York, USA: Association for Computing Machinery). VRST ’20. doi:10.1145/3385956.3422111
Latoschik, M. E., Kern, F., Stauffert, J.-P., Bartl, A., Botsch, M., and Lugrin, J.-L. (2019). Not alone Here?! Scalability and User Experience of Embodied Ambient Crowds in Distributed Social Virtual Reality. IEEE Trans. Vis. Comput. Graphics 25, 2134–2144. doi:10.1109/tvcg.2019.2899250
Latoschik, M. E., Lugrin, J.-L., and Roth, D. (2016). “FakeMi: A Fake Mirror System for Avatar Embodiment Studies,” in Proceeding of ACM Symposium on Virtual Reality Software and Technology, Garching bei Mun¨chen, Germany, November 2–4, 2016.
Latoschik, M. E., Roth, D., Gall, D., Achenbach, J., Waltemate, T., and Botsch, M. (2017). “The Effect of Avatar Realism in Immersive Social Virtual Realities,” in Proceedings of the 23rd ACM Symposium on Virtual Reality Software and Technology, Gothenburg, Sweden, November 8–10, 2017 (ACM), 39.
LaViola, J. J. (2017). 3D User Interfaces: Theory and Practice. second edition edn. Boston: Addison-Wesley.
Lelyveld, P., and Entertainment, U. (2015). Virtual Reality Primer with an Emphasis on Camera-Captured Vr. SMPTE Mot. Imag. J. 124, 78–85. doi:10.5594/j18599
Lindner, P. (2020). Better, Virtually: the Past, Present, and Future of Virtual Reality Cognitive Behavior Therapy. J. Cogn. Ther., 14, 23, 46. doi:10.1007/s41811-020-00090-7
Lix, L. M., Keselman, J. C., and Keselman, H. J. (1996). Consequences of assumption Violations Revisited: A Quantitative Review of Alternatives to the One-Way Analysis of Variance F Test. Rev. Educ. Res. 66, 579–619. doi:10.3102/00346543066004579
Lugrin, J.-L., Unruh, F., Landeck, M., Lamour, Y., Latoschik, M. E., Vogeley, K., et al. (2019). “Experiencing Waiting Time in Virtual Reality,” in 25th ACM Symposium on Virtual Reality Software and Technology, Parramatta, NSW, Australia, November 12–15, 2019.
Martin, B., Franck, N., Cermolacce, M., Falco, A., Benair, A., Etienne, E., et al. (2017). Fragile Temporal Prediction in Patients with Schizophrenia Is Related to Minimal Self Disorders. Scientific Rep. 7, 1–10. doi:10.1038/s41598-017-07987-y
Meissner, K., and Wittmann, M. (2011). Body Signals, Cardiac Awareness, and the Perception of Time. Biol. Psychol. 86, 289–297. doi:10.1016/j.biopsycho.2011.01.001
Minsky, M. (1974). “A Framework for Representing Knowledge Marvin Minsky MIT-AI Laboratory Memo 306, June, 1974,” in The Psychology of Computer Vision. Editors P. Winston, J. Haugeland, C. Allan, and E. E. Smith.
Nararro-Haro, M. V., Hoffman, H. G., Garcia-Palacios, A., Sampaio, M., Alhalabi, W., Hall, K., et al. (2016). The Use of Virtual Reality to Facilitate Mindfulness Skills Training in Dialectical Behavioral Therapy for Borderline Personality Disorder: a Case Study. Front. Psychol. 7, 1573. doi:10.3389/fpsyg.2016.01573
Navarro-Haro, M. V., Modrego-Alarcón, M., Hoffman, H. G., López-Montoyo, A., Navarro-Gil, M., Montero-Marin, J., et al. (2019). Evaluation of a Mindfulness-Based Intervention with and without Virtual Reality Dialectical Behavior Therapy Mindfulness Skills Training for the Treatment of Generalized Anxiety Disorder in Primary Care: A Pilot Study. Front. Psychol. 10, 55. doi:10.3389/fpsyg.2019.00055
Niemi, P., and N??t?nen, R. (1981). Foreperiod and Simple Reaction Time. Psychol. Bull. 89, 133–162. doi:10.1037/0033-2909.89.1.133
Osuna, E. E. (1985). The Psychological Cost of Waiting. J. Math. Psychol. 29, 82–105. doi:10.1016/0022-2496(85)90020-3
Peña, J., Hancock, J. T., and Merola, N. A. (2009). The Priming Effects of Avatars in Virtual Settings. Commun. Res. 36, 838–856. doi:10.1177/0093650209346802
Pfeifer, E., Sarikaya, A., and Wittmann, M. (2016). Changes in States of Consciousness during a Period of Silence after a Session of Depth Relaxation Music Therapy (Drmt). Mmd 8, 180–186. doi:10.47513/mmd.v8i4.473
Phillips, L., Ries, B., Kaeding, M., and Interrante, V. (2010). “Avatar Self-Embodiment Enhances Distance Perception Accuracy in Non-photorealistic Immersive Virtual Environments,” in 2010 IEEE virtual reality conference (VR), Boston, MA, March 20–24, 2010 (IEEE), 115–1148.
Pizarro, R., Berkers, K.-O., Slater, M., and Friedman, D. (2015). How to Time Travel in Highly Immersive Virtual Reality. ICAT-EGVE, 117–124. doi:10.2312/egve.20151318
Pollatos, O., Laubrock, J., and Wittmann, M. (2014). Interoceptive Focus Shapes the Experience of Time. PloS one 9, e86934. doi:10.1371/journal.pone.0086934
Regenbrecht, H., and Schubert, T. (2002). Real and Illusory Interactions Enhance Presence in Virtual Environments. Presence: Tele. Virtual Environ. 11, 425–434. doi:10.1162/105474602760204318
Ries, B., Interrante, V., Kaeding, M., and Anderson, L. (2008). “The Effect of Self-Embodiment on Distance Perception in Immersive Virtual Environments,” in Proceedings of the 2008 ACM symposium on Virtual reality software and technology, Bordeaux, France, October 27–29, 2008, 167–170.
Roth, D., and Latoschik, M. (2019). Construction of a Validated Virtual Embodiment Questionnaire. arXiv.
Roth, D., and Latoschik, M. E. (2020). Construction of the Virtual Embodiment Questionnaire (Veq). IEEE Trans. Visualization Comp. Graphics 26 (12), 3546–3556. doi:10.1109/TVCG.2020.3023603
Roth, D., Lugrin, J.-L., Latoschik, M. E., and Huber, S. (2017). “Alpha Ivbo-Construction of a Scale to Measure the Illusion of Virtual Body Ownership,” in Proceedings of the 2017 CHI Conference Extended Abstracts on Human Factors in Computing Systems, Denver, CO, May 6–11, 2017 (ACM), 2875–2883.
Roth, D., Lugrin, J.-L., Galakhov, D., Hofmann, A., Bente, G., Latoschik, M. E., et al. (2016). “Avatar Realism and Social Interaction Quality in Virtual Reality,” in Proceedings of IEEE Virtual Reality, Greenville, SC, March 19–23, 2016, 277–278.
Sackett, A. M., Meyvis, T., Nelson, L. D., Converse, B. A., and Sackett, A. L. (2010). You're Having Fun when Time Flies. Psychol. Sci. 21, 111–117. doi:10.1177/0956797609354832
Sanchez-Vives, M. V., and Slater, M. (2005). From Presence to Consciousness through Virtual Reality. Nat. Rev. Neurosci. 6, 332–339. doi:10.1038/nrn1651
Sarrazin, J.-C., Giraudo, M.-D., Pailhous, J., and Bootsma, R. J. (2004). Dynamics of Balancing Space and Time in Memory: Tau and Kappa Effects Revisited. J. Exp. Psychol. Hum. Perception Perform. 30, 411–430. doi:10.1037/0096-1523.30.3.411
Schatzschneider, C., Bruder, G., and Steinicke, F. (2016). Who Turned the Clock? Effects of Manipulated Zeitgebers, Cognitive Load and Immersion on Time Estimation. IEEE Trans. Vis. Comput. Graphics 22, 1387–1395. doi:10.1109/tvcg.2016.2518137
Schneider, S. M., and Hood, L. E. (2007). Virtual Reality: a Distraction Intervention for Chemotherapy. Oncol. Nurs. Forum 34, 39–46. doi:10.1188/07.onf.39-46
Schneider, S. M., Kisby, C. K., and Flint, E. P. (2011). Effect of Virtual Reality on Time Perception in Patients Receiving Chemotherapy. Support Care Cancer 19, 555–564. doi:10.1007/s00520-010-0852-7
Schubert, T., Friedmann, F., and Regenbrecht, H. (2001). The Experience of Presence: Factor Analytic Insights. Presence: Teleoperators & Virtual Environments 10, 266–281. doi:10.1162/105474601300343603
Schubert, T. W. (2003). The Sense of Presence in Virtual Environments:. Z. für Medienpsychologie 15, 69–71. doi:10.1026//1617-6383.15.2.69
Schwind, V., Knierim, P., Chuang, L., and Henze, N. (2017). “Where’s Pinky?” the Effects of a Reduced Number of Fingers in Virtual Reality,” in Proceedings of the Annual Symposium on Computer-Human Interaction in Play, Amsterdam, Netherlands, October 15–18, 2017, 507–515.
Shahnewaz, S., Afarat, I., Gayani, T. I., Mikael, S., Labbe, D., Quarles, J., et al. (2016). “Gaitzilla: A Game to Study the Effects of Virtual Embodiment in Gait Rehabilitation,” in 2016 IEEE Symposium on 3D User Interfaces (3DUI), Greenville, SC, March 19–20, 2016 (IEEE), 265–266.
Slater, M. (2009). Place Illusion and Plausibility Can lead to Realistic Behaviour in Immersive Virtual Environments. Phil. Trans. R. Soc. B 364, 3549–3557. doi:10.1098/rstb.2009.0138
Slater, M., and Steed, A. (2000). A Virtual Presence Counter. Presence: Tele. Virtual Environ. 9, 413–434. doi:10.1162/105474600566925
Spanlang, B., Normand, J.-M., Borland, D., Kilteni, K., Giannopoulos, E., Pom, A. S., et al. (2014). How to Build an Embodiment Lab: Achieving Body Representation Illusions in Virtual Reality. Front. Robot. AI 1, 9. doi:10.3389/frobt.2014.00009
Stauffert, J.-P., Niebling, F., Lugrin, J.-L., and Latoschik, M. E. (2020). “Guided Sine Fitting for Latency Estimation in Virtual Reality,” in 2020 IEEE Conference on Virtual Reality and 3D User Interfaces Abstracts and Workshops, Atlanta, GA, March 22–26, 2020 (VRW), 707–708.
Steuer, J. (1992). Defining Virtual Reality: Dimensions Determining Telepresence. J. Commun. 42, 73–93. doi:10.1111/j.1460-2466.1992.tb00812.x
Sylaiou, S., Mania, K., Karoulis, A., and White, M. (2010). Exploring the Relationship between Presence and Enjoyment in a Virtual Museum. Int. J. human-computer Stud. 68, 243–253. doi:10.1016/j.ijhcs.2009.11.002
Taylor, S. (1994). Waiting for Service: the Relationship between Delays and Evaluations of Service. J. marketing 58, 56–69. doi:10.2307/1252269
The free dictionary (2020) [Dataset]. Time Definition. Available at: https://www.thefreedictionary.com/Physical+time (accessed 11-12, 2020)
Thönes, S., Von Castell, C., Iflinger, J., and Oberfeld, D. (2018). Color and Time Perception: Evidence for Temporal Overestimation of Blue Stimuli. Sci. Rep. 8, 1688–8. doi:10.1038/s41598-018-19892-z
Thönes, S., and Wittmann, M. (2016). Time Perception in Yogic Mindfulness Meditation-Effects on Retrospective Duration Judgments and Time Passage. Psychol. Conscious. Theor. Res. Pract. 3, 316–325. doi:10.1037/cns0000088
Tobin, S., Bisson, N., and Grondin, S. (2010). An Ecological Approach to Prospective and Retrospective Timing of Long Durations: a Study Involving Gamers. PloS one 5, e9271. doi:10.1371/journal.pone.0009271
van der Ham, I. J. M., Klaassen, F., van Schie, K., and Cuperus, A. (2019). Elapsed Time Estimates in Virtual Reality and the Physical World: The Role of Arousal and Emotional Valence. Comput. Hum. Behav. 94, 77–81. doi:10.1016/j.chb.2019.01.005
Vogeley, K., and Kupke, C. (2007). Disturbances of Time Consciousness from a Phenomenological and a Neuroscientific Perspective. Schizophr Bull. 33, 157–165. doi:10.1093/schbul/sbl056
Wallach, H. S., Safir, M. P., and Bar-Zvi, M. (2009). Virtual Reality Cognitive Behavior Therapy for Public Speaking Anxiety. Behav. Modif 33, 314–338. doi:10.1177/0145445509331926
Waltemate, T., Gall, D., Roth, D., Botsch, M., and Latoschik, M. E. (2018). The Impact of Avatar Personalization and Immersion on Virtual Body Ownership, Presence, and Emotional Response. IEEE Trans. Vis. Comput. Graphics 24, 1643–1652. doi:10.1109/tvcg.2018.2794629
Waltemate, T., Hülsmann, F., Pfeiffer, T., Kopp, S., and Botsch, M. (2015). “Realizing a Low-Latency Virtual Reality Environment for Motor Learning,” in Proceedings of the 21st ACM symposium on virtual reality software and technology, Beijing, China, November 13–15, 2015, 139–147.
Wearden, J. H., Edwards, H., Fakhri, M., and Percival, A. (1998). Why”sounds Are Judged Longer Than Lights”: Application of a Model of the Internal Clock in Humans. The Q. J. Exp. Psychol. Section B 51, 97–120.
Wearden, J. H. (2015). Passage of Time Judgements. Conscious. Cogn. 38, 165–171. doi:10.1016/j.concog.2015.06.005
Wearden, J. H. (2008). The Perception of Time: Basic Research and Some Potential Links to the Study of Language. Lang. Learn. 58, 149–171. doi:10.1111/j.1467-9922.2008.00468.x
Weiner, L., Wittmann, M., Bertschy, G., and Giersch, A. (2016). Dispositional Mindfulness and Subjective Time in Healthy Individuals. Front. Psychol. 7, 786. doi:10.3389/fpsyg.2016.00786
Witmer, B. G., and Singer, M. J. (1998). Measuring Presence in Virtual Environments: A Presence Questionnaire. Presence 7, 225–240. doi:10.1162/105474698565686
Wittmann, M. (2013). The Inner Sense of Time: How the Brain Creates a Representation of Duration. Nat. Rev. Neurosci. 14, 217–223. doi:10.1038/nrn3452
Yuan, Y., and Steed, A. (2010). “Is the Rubber Hand Illusion Induced by Immersive Virtual Reality?,” in 2010 IEEE Virtual Reality Conference (VR), Boston, MA, March 20–24, 2010 (IEEE), 95–102.
Zakay, D., and Block, R. A. (1995). An Attentional-Gate Model of Prospective Time Estimation. Time dynamic Control Behav., 167–178.
Zakay, D., and Block, R. A. (1997). Temporal Cognition. Curr. Dir. Psychol. Sci. 6, 12–16. doi:10.1111/1467-8721.ep11512604
Zakay, D. (2014). Psychological Time as Information: the Case of Boredomâ€. Front. Psychol. 5, 917. doi:10.3389/fpsyg.2014.00917
Zakay, D. (1993). Relative and Absolute Duration Judgments under Prospective and Retrospective Paradigms. Perception & Psychophysics 54, 656–664. doi:10.3758/bf03211789
Keywords: virtual reality, time perception, avatar embodiment, immersion, human computer interaction (HCI)
Citation: Unruh F, Landeck M, Oberdörfer S, Lugrin J-L and Latoschik ME (2021) The Influence of Avatar Embodiment on Time Perception - Towards VR for Time-Based Therapy. Front. Virtual Real. 2:658509. doi: 10.3389/frvir.2021.658509
Received: 25 January 2021; Accepted: 18 May 2021;
Published: 19 July 2021.
Edited by:
John Naslund, Harvard Medical School, United StatesReviewed by:
Sheila A. M. Rauch, Emory University, United StatesVangelis Lympouridis, University of Southern California, United States
Copyright © 2021 Unruh, Landeck, Oberdörfer, Lugrin and Latoschik. This is an open-access article distributed under the terms of the Creative Commons Attribution License (CC BY). The use, distribution or reproduction in other forums is permitted, provided the original author(s) and the copyright owner(s) are credited and that the original publication in this journal is cited, in accordance with accepted academic practice. No use, distribution or reproduction is permitted which does not comply with these terms.
*Correspondence: Fabian Unruh, ZmFiaWFuLnVucnVoQHVuaS13dWVyemJ1cmcuZGU=