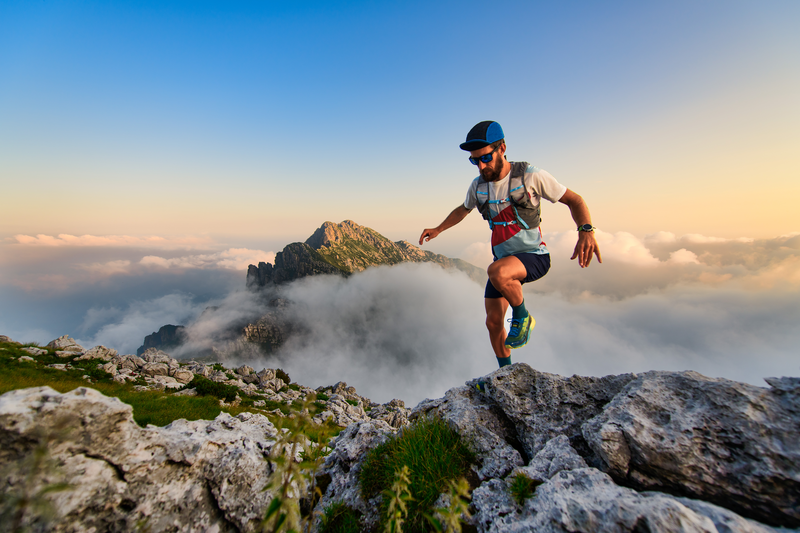
94% of researchers rate our articles as excellent or good
Learn more about the work of our research integrity team to safeguard the quality of each article we publish.
Find out more
ORIGINAL RESEARCH article
Front. Virtual Real. , 24 November 2020
Sec. Virtual Reality and Human Behaviour
Volume 1 - 2020 | https://doi.org/10.3389/frvir.2020.582095
This article is part of the Research Topic Cybersickness in Virtual Reality Versus Augmented Reality View all 13 articles
Visually induced motion sickness is an unpleasant but common side-effect of many simulations and VR-applications. We investigated whether an earth-fixed reference frame provided in the simulation is able to reduce motion sickness. To do so, we created a moving starfield that did not contain any indicators of the spatial orientation of the observer. As the observer was simulated to move through the randomly oscillating starfield, a time-to-contact task had to be carried out. Two colored stars on collision course with each other had to be spotted, then they disappeared and the time of their collision had to be judged. Eye-movements, task performance, and motion sickness were recorded. This condition without visual reference to the observer's upright was supplemented with three conditions containing either an earth-fixed fixation cross, an earth-fixed horizon line, or a line that was yoked to the head. Results show that only the earth-fixed horizon was able to significantly reduce visually induced motion sickness. Thus, a mere earth-stationary anchor does not suffice, a clear indication of earth horizontal seems necessary to reap a modest benefit.
Motion sickness (MS) is a common physiological and psychological response to unfamiliar motion patterns and a frequent side-effect induced by provocative motion environments, as associated with many forms of transportation, such as ships, aircraft, and automobiles. Motion sickness can also occur during Virtual Reality (VR) applications (e.g., driving or flight simulators), typically labeled as visually induced motion sickness (VIMS). VIMS is a special case of MS, which is primarily caused by stimulation of the visual system in the absence of real, physical movement (Keshavarz et al., 2014).
The nomenclature regarding VIMS is highly inconsistent and often dependent on the technology being employed, with studies referring to VIMS as cybersickness (e.g., McCauley and Sharkey, 1992; Davis et al., 2014), simulator sickness (e.g., Kennedy et al., 1992; Hettinger and Haas, 2003), gaming sickness (e.g., Chen et al., 2016; Oldenburg, 2018), and virtual reality sickness (e.g., Guna et al., 2019; Saredakis et al., 2020). However, we will use the term VIMS, as it includes all of the above-mentioned types of MS (Keshavarz et al., 2014).
Several theories have been proposed to explain VIMS, including the role of postural control (Riccio and Stoffregen, 1991) or eye movements (Ebenholtz et al., 1994), but the true ethiopathogeny and the biological mechanisms underlying VIMS still remain elusive. Claremont (1931) originally suggested the theory that sea sickness is caused by an inter-sensory conflict, which was later refined by Steele (1961), Guedry (1965), and Reason (1969). Reason and Brand (1975) later formalized and distilled the previous research into the framework of sensory conflict theory, which is probably the most commonly accepted notion for the development of VIMS today. It states that VIMS is caused (a) if conflicting information is provided by the visual, vestibular, and somatosensory senses; (b) if the configuration sensed among these three modalities does not match what would be expected based on previous experience. For instance, the visual stimulation in an immersive but stationary driving simulator may suggest apparent self-motion of the driver (known as vection), whereas the vestibular and somatosensory senses signal stasis. This visual-vestibular conflict can, under certain circumstances (e.g., lack of adaptation mechanisms) result in the sensation of VIMS.
In the context of traditional MS (i.e., sea sickness), anecdotal evidence suggests that leaving the cabin and finding a spot on the deck of the ship may help to reduce feelings of MS. Fresh air might contribute to subdue the symptoms (D'Amour et al., 2017), but, at the same time, the horizon becomes visible when on the ship's deck, which reduces the intersensory conflict between the visual and vestibular senses (Hill, 1936; Bruner, 1955; Rolnick and Bles, 1989; Turner and Griffin, 1995; Tal et al., 2012; Keshavarz et al., 2014). Interestingly, Mayo et al. (2011) found a reduction in body-sway when viewing the horizon on deck of a ship, which supports the idea that being able to see the horizon helps to reduce MS, as increased body sway has been associated with elevated levels of MS and VIMS in the past (Stoffregen and Smart, 1998; Smart et al., 2002).
It is thought that a visible horizon reduces the sensory conflict by providing a frame-of-reference that allows the visual system to synchronize with the perceived motion. In aviation, an extended horizon line or Malcolm Horizon (Malcolm, 1983) makes use of this concept by projecting an artificial horizon line across the cockpit, providing pilots with a line that is parallel to the true horizon, regardless of the aircraft's orientation with respect to the ground. The Malcolm Horizon has been shown to reduce tilt sensations in pilots (Lackner, 1990, p. 47). In fact, artificial horizons were amongst the earliest standard instruments used in aeronautical navigation (Schroer, 2003), and maintaining visual contact is recommended to reduce MS in poor viewing conditions (Burcham, 2002, p. 5).
The understanding of the mitigating effect of a horizon can be supplemented by considering rest frames. As suggested by Prothero (1998) and Prothero and Parker (2003), the concept of rest frames is derived from the observation that humans have a strong innate perception of stationary objects. By their definition, rest frames are particular visual frames that are perceived to be stationary and are used as a neurological comparator for spatial judgments. In contrast, reference frames define a positional reference system for spatial features, allowing comparative localization with respect to position, orientation, and motion. According to Prothero (1998), a scene can be divided into two distinct elements: (1) the content-of-interest and (2) the independent visual background. The content-of-interest is the entirety of all the foreground cues, for instance a scene in which an observer is driving a car, whereas the independent visual background is a visual object that, which is consistent with inertial cues and provides an earth-fixed reference frame (e.g., a fixation cross or a static horizon).
With regard to VIMS in virtual environments as experienced when wearing a head-mounted display (HMD), the absence or presence of visual reference information would produce different predictions for VIMS severity according to the following reasoning. According to the wide-spread conflict theory of VIMS, malaise increases as a function of the conflict between visual and vestibular/proprioceptive information (see e.g., Nooij et al., 2017). Now, how does the reference frame play into this? When the comparator pits consistent visual information against consistent but disagreeing vestibular information, the conflict, and thus VIMS, should be largest. If visual information is inconsistent, this should reduce the conflict. A stimulus devoid of reference information should be most provocative. A stimulus providing an earth-stable reference, in contrast, should minimize the conflict as it is maximally consistent with the vestibular information. Other visual references, such as a fixation dot, or a reference that is yoked to the head of the observer, such as the visible frame of the HMD, should fall somewhere in-between. Previous studies have examined the effect of an artificial horizon and found it to be beneficial in the reduction of VIMS symptoms (Rolnick and Bles, 1989; Lin et al., 2004; Tal et al., 2012, 2014). However, they have not compared degrees of visual reference frame information.
Eye-movements have been taken to indicate visual information processing and have been discussed as a potential contributor to the genesis of MS and VIMS (Ebenholtz et al., 1994) and have been linked to the occurrence of MS via stimulation of the vagal nerve. Thus, minimizing the amount of eye movements should reduce the occurrence of MS and VIMS. Providing a visual reference frame should thus reduce eye-movements and VIMS if the two are causally related. In fact, several studies successfully demonstrated that a fixation cross presented in the center of a visual scene can reduce nystagmus and VIMS at the same time (Stern et al., 1990; Flanagan et al., 2002; Webb and Griffin, 2002).
The objective of the present study was to further investigate the role of stationary rest frames and eye movements in the occurrence of VIMS; that is to determine whether different rest frames can serve as a potential countermeasure and effectively reduce VIMS. To achieve this, we exposed our participants to a potentially nauseating optic flow stimulus (starfield) in a VR-setup using an HMD. The starfield stimulus was chosen based on the theoretical and methodological considerations of Keshavarz et al. (2019), who successfully induced VIMS with this type of stimulus. To provide a meaningful stimulus, we designed a time-to-contact (TTC) task to be performed during the experiment. It fulfilled the function to direct the subject's attention to the stimulus and at the same served as a performance measure. TTC is the time remaining until a collision occurs between two objects that approach each other provided they will continue on the same trajectory at the same speed. This prediction task ensured a high level of alertness.
Four distinct experimental conditions were chosen which varied with respect to the presence of additional visual cues that were superimposed on the screen: two conditions included earth-stationary visual cues (Fixation Cross and a Fixed Horizon), one condition included a visual cue that was congruent with the camera's random motion (Moving Horizon), and one condition acted as control with no additional visual cues (No Visual Cues). Apart from self-reported VIMS scores, we measured heart-rate and eye-movements, which have been linked to the occurrence of VIMS in previous studies (Crampton, 1955; Cowings et al., 1986; Stout et al., 1995; Holmes and Griffin, 2001), considering that the majority of VIMS symptoms is related to an increase in sympathetic activity and a decrease in parasympathetic activity (Hu et al., 1991; Doweck et al., 1997; Holmes and Griffin, 2001). Although a strong relationship between heart-rate/heart-rate variability and VIMS could not be established in the past (Mullen et al., 1998), we added these measures to gather further insights into the physiological changes associated with VIMS. Perceptual measures (vection, immersion, realism) regarding the stimulus were collected following stimulus presentation and are analyzed in relationship to FMS ratings (Fast Motion Sickness Scale; Keshavarz et al., 2014, 2015) and gender (Hemmerich et al., 2019). While the relationship between vection and VIMS is not yet fully understood, the probability of experiencing VIMS increases with the occurrence of vection, making it a potential prerequisite for VIMS, given that other factors are also in place (Keshavarz et al., 2015). Similarly, correlates between VIMS and immersion (e.g., Yang et al., 2012; Duan et al., 2018), as well as realism have been reported (e.g., D'Amour et al., 2017; Pouke et al., 2018).
Thirty-three participants volunteered for this study. Eight participants (7 female) chose to terminate the experiment prematurely due to severe levels of VIMS and 3 participants were excluded because they reported medical conditions (chronic pain), resulting in a final sample of n = 22 (15 female, 7 male). Participants' age ranged from 19 to 33 years (M = 24.2 years, SD = 3.3 years). Inclusion criteria were normal or corrected-to-normal (lenses only) vision. Correction with eyeglasses was an exclusion criterion during recruitment due to the restricted space in the head-mounted display (HMD). The study was conducted in accordance with the Declaration of Helsinki. Participants received partial course credit as compensation. All participants were naïve with respect to the purpose of the study and were not familiar with the experimental task and rationale.
In a within-subjects design, all participants were exposed to four experimental conditions: (1) Moving Horizon, (2) Fixed Horizon, (3) Fixation Cross, and (4) No Visual Cues. Note that we deliberately chose a within-subjects design over a between-subjects approach, as MS varies considerably among subjects, increasing the interindividual variability and complicating the comparison across different experimental groups. The order of conditions was randomized and presented in a single test session. The Moving Horizon was identical to the Fixed Horizon with the exception that the Moving Horizon was not fixed in virtual space but rather moved synchronously with the camera's random motion through the virtual environment. Both the Fixed Horizon and the Fixation Cross created an earth-stationary reference in VR, which was invariant to the randomly generated motion but changed its relative position in the display depending on the observer's head movements. Strictly speaking, the Horizon was a narrow rectangle, 11.10 × 0.07 relative units in size, whereas the Fixation Cross was comprised of two 2.19 × 0.07 rectangles, which were perpendicular to each other at their geometric center. All objects were positioned at the Cartesian origin in virtual space.
The optic flow stimulus consisted of a 14 min simulated flight through a virtual starfield. The visual reference condition was randomly changed every 3.5 min, such that each participant saw all 4 conditions during the 14 min flight. The stimulus was created and presented using Unity3D, a cross-platform game engine with emphasis on rendering-speed and realism. The starfield was generated using a particle system, which spawned a constant conic torrent of random particles (i.e., white spheres) toward the camera object, with ~4.000 visible particles being rendered at any given time, although, due to the vastness of the virtual space, far fewer were visible (see Figure 1). The stars grew in retinal size according to their proximity in virtual space. Random motion was produced using the plugin Jitter (Virtual Escapes, 2019). Motion profiles were randomly generated using a seeded pseudorandom number generator and, therefore, they were practically identical between subjects. A post-render bloom shader was applied to the particles to increase the overall stimulus realism and to amplify the nauseogenic effect (Bonato et al., 2015). After all, the goal was to trigger measurable VIMS without incapacitating the participants. To guarantee a high level of alertness and to evaluate participants' performance as a function of VIMS, the time-to-contact task was incorporated into the simulation.
Figure 1. The starfield stimulus used, here as seen in the Fixed Horizon condition. The yellow line marks the artificial horizon; the two red squares gravitated toward one another before disappearing (TTC-task).
In a pilot study (n = 11) prior to the actual experiment, we identified the rotational and transitional parameters controlling the motion of the attached camera objects. The plugin Jitter requires three parameters (magnitude, amplitude, and frequency) which we set to the values found in Table 1 following the pilot study. Magnitude controls the amount of noise, with larger values corresponding to an increase in the noise level; the amplitude specifies a range which defines the upper and lower bound between which random values can vary; frequency controls the speed at which the random noise varies, with larger values indicating a more abrupt transition to the next value.
The stimulus was presented stereoscopically on an HTC Vive HMD with an integrated Pupil Labs binocular eye-tracking add-on. The HTC Vive has a combined resolution of 2,160 × 1,200 px (1,080 × 1,200 px per eye) and 110° field of view horizontally, 100° field of view vertically, rendering at 90 Hz; the Pupil Labs eye-tracker has a sampling frequency of 100 Hz per eye, with a gaze accuracy of ~1.0° and a gaze precision of ~0.08°. Participants used the Vive controller in their preferred hand to respond to the TTC task. As per the recommendations of Santini et al. (2018), only samples with a confidence rating > 0.66 were included in the analysis.
At intervals of 7 s, participants were presented with two red squares, which started moving toward one another at varying speeds but disappeared before colliding. Participants were tasked to press the trigger button on the Vive controller at the moment when the objects would have collided had they continued on their trajectory. Speeds of the red squares were chosen such that collision times ranged between 600 and 1,500 ms, with 10 different speed conditions in total. The red squares were positioned slightly behind the horizon or cross and presented at three varying inclinations (−25°, 0°, 25°), pivoting at the Cartesian origin, 50 relative units apart at their starting position. This resulted in 30 unique combinations that were repeated at random for each of the four experimental conditions. Non-response trials were followed by a red flash. Absolute and constant errors in completed TTC judgments were used for statistical analysis.
Heart rate and eye movements were measured during the experiment. Heart rate was measured continuously at 1 Hz using the Covidien Nellcor PM10N and then averaged in 1 min blocks for subsequent analysis, for all correlational analysis. This way, heart rate was down-sampled to the frequency of the VIMS ratings. Eye-movements were recorded using the Pupil Labs binocular eye-tracking add-on, which integrates into the HTC Vive.
The Fast Motion Sickness Scale (FMS; Keshavarz and Hecht, 2011) was used to measure the level of VIMS during stimulus presentation. The FMS is a verbal rating scale ranging from 0 (no sickness) to 20 (severe sickness) and focuses on subjects' general discomfort, nausea, and stomach problems. The FMS has been shown to have high correlations with the Simulator Sickness Questionnaire by Kennedy et al. (1993), and its rapid administration allows for the quantification of the time course of MS. The FMS allows for unobtrusive rapid self-report while participants are engaged in the VR task and was used every 60 s during the stimulus presentation. Ratings of the subjective intensity of vection, as well as immersion and realism of the display were obtained once at the end of the session. Participants rated the maximal strength of the vection they had experienced, that is the feeling of moving forward through the starfield (as opposed to stars moving by) on a scale ranging from 1 (no vection) to 7 very strong vection. Then they indicated how often throughout the 14 min of stimulus presentation they were in a state of vection, on a similar scale from 1 (never) to 7 (pretty much the entire time). Immersion, the sophistication of the virtual world, and realism, the sense of being in the simulation, were likewise rated on 7-point scales.
Written consent was obtained from the participants prior to the experiment. Participants were instructed in the use of the HMD, the TTC task, and the FMS scale but remained naïve as to the objective of the study until the end of the experiment. Once participants were comfortable and all pending questions had been answered, testing began with the connection and setup of the HMD and the pulse oximeter, followed by a calibration of the eye-tracker. Participants' FMS baseline readings were taken and the stimulus was started. Participants were not given any specific instructions as to what position to remain in during stimulus presentation and they were free to move their head within the virtual environment, should they choose to do so. Note that the TTC-task required them to move the head in order to spot both targets at their initial positions. FMS readings were taken in 1 min intervals for the duration of the stimulus. Upon completion, participants were asked to fill in a questionnaire asking for the overall ratings of vection, immersion, and realism. Then they were debriefed about the background of the study.
Statistical analyses were performed using SPSS 26, JASP 0.13, and R 3.6.3. We determined within-subject correlations using the rmcorr package (Bakdash and Marusich, 2017). First, we performed a general assessment to verify that VIMS had indeed been elicited. Accordingly, a paired t-test with baseline and peak FMS scores showed a significant increase and, therefore, a successful manipulation, t(21) = −5.827, p < 0.001, d = −1.242.
It is common to use peak FMS-scores for analysis purposes (see the original validation study by Keshavarz and Hecht (2011) which used peak FMS scores in a between-subjects design. However, this was no longer possible in our within-subjects design, as VIMS accumulates over the course of the entire session. Thus, we used average FMS scores per condition instead. As the relationship between FMS-scores over time was cumulative and best modeled by a quadratic function, FMS values were averaged using the geometric mean (Streiner, 2000; De Muth, 2006; Pal and Bharati, 2019).
The average FMS-scores per condition are plotted in Figure 2. A repeated measures ANOVA including the within-subjects factor experimental condition (Moving Horizon, Fixed Horizon, Fixation Cross, and No Visual Cues) was calculated for the averaged FMS scores. Sphericity was assumed, Mauchly-Test p > 0.05. Results showed a significant main effect of experimental condition, F(3, 63) = 2.867, p = 0.043, = 0.120. Planned contrasts revealed significant differences between the fixed horizon and all other conditions: Fixed Horizon vs. Moving Horizon, t(63) = −2.165, p = 0.034, Fixed Horizon vs. Fixation Cross, t(63) = −2.492, p = 0.015, Fixed Horizon vs. No visual cues, t(63) = −2.471, p = 0.016, Fixed Horizon vs. all other conditions (equally weighted), t(63) = −2.905, p = 0.005, but not between any other conditions (p's > 0.744).
Figure 2. Average FMS scores for each condition. Averages represent the geometric mean. Error bars indicate the SEM.
To provide an impression for the distribution of VIMS among subjects, we have also computed the peak FMS scores across all subjects, as well as the peak scores associated with each condition disregarding the cumulative effects. Figure 3 shows these distributions as a box-plot, illustrating that VIMS varied considerably among subjects.
Figure 3. Box-plots of overall peak FMS across all conditions and peak FMS scores for each condition. The line in the middle of the box indicates the median value. The cross represents the mean.
The means of the other measures per condition are given in Table 2. Added to this table are the results of an rmANOVA for the factor condition and each secondary measure. Heart rate did not, but absolute TTC-error did vary significantly among conditions.
Note that the scanpath length of all eye-movements was calculated by computing and summing the Euclidean distances between consecutive eye-fixation data points. The dispersion of scanpath length is the standard deviation of these distances.
Furthermore, repeated measures correlations were calculated among FMS, heart-rate, TTC-judgments, and scanpath length. For these correlations, data were averaged over 1 min intervals to make them compatible with the respective corresponding FMS values taken at the end of the same intervals (see Table 3).
Table 4 shows the correlations between peak FMS scores (i.e., the highest FMS rating during the entire 14 min stimulus presentation), gender, and post-stimulus ratings for vection, immersion, and realism.
The purpose of this paper was to further investigate if an artificial earth-reference within a virtual environment is able to reduce the degree of VIMS, which is a common and serious side-effect of VR-displays. Participants had to perform a TTC-estimation task in a virtual starfield that did not provide any orientation cues except for the experimental references (earth-fixed fixation cross, earth-fixed horizon line, head-fixed horizon line, control). An earth-fixed reference is thought to reduce the sensory conflict by providing a frame-of-reference, allowing for the synchronization of the visual system with the perceived motion (Harm et al., 1998; Prothero et al., 1999). We found that VIMS occurred in all experimental conditions, to the extent that many participants chose to abort the experiment. Among those who were able to finish the experiment, the fixed horizon line caused less VIMS than did the other conditions.
Both this horizon and the fixation cross were fixed with respect to true earth horizontal, that is, they visualized a reference consistent with the vestibular information of the horizontal. The horizon-line effect is in line with previous studies, which also found reports of lower MS with an artificial or real visible horizon (Bruner, 1955; Rolnick and Bles, 1989; Turner and Griffin, 1995; Tal et al., 2014). Interestingly, participants only experienced reduced VIMS when viewing the fixed horizon line, but not when provided with the fixation cross. This is striking, as both provide a stationary region within the visual field, which should—in theory—minimize the sensory conflict or rather provide a rest-frame congruent with inertial cues.
A possible explanation for this difference may be the narrow attentional focus when looking at the cross. The short cross-hairs of this fix-point provided an orientation cue but only when foveated. However, participants were required to focus the target stars in order to perform the TTC task. The horizon line extended into their peripheral visual field, providing a constant source of spatial orientation but the orientation of the cross was not discernable in peripheral vision. This would be consistent with previous evidence regarding the Malcolm Horizon (Malcolm, 1983; Comstock et al., 2003).
Another explanation may be that due to the TTC task, subjects performed more medio-lateral head-movements to keep both targets discernable within their field-of-view. The earth-fixed horizon line remained more or less stable when making medio-latera head turns, and was thus not obtrusive while still providing a predictably motionless area. In contrast, the vertical bar of the fixation cross—as soon as it was no longer foveated—produced the impression that it moved, sometimes causing apparent motion while scanning along the azimuth. This may have substantially reduced its ability to serve as an earth-fixed reference point.
The peak FMS scores did not explain any variance in the ratings of vection, immersion, and realism: none of the correlations were significant. The correlations between immersion, realism, and vection frequency can be explained by the conceptual overlap among these measures. The failure of perceived vection to partially explain VIMS is not uncommon. Findings here have been inconsistent across studies (for a full review, see Keshavarz et al., 2014, 2015). We did not find a significant main effect of gender, probably due to the small number of male subjects. The positive correlation of FMS and gender indicates that women tended to experience higher FMS scores than men, which is often the case in larger samples (but see Hemmerich et al., 2019).
The relationship between VIMS and eye-movements and heart-rate is tenuous at best. We found a small, but significant correlation of FMS ratings with heart-rate, which is consistent with some previous studies (Crampton, 1955; Cowings et al., 1986; Stout et al., 1995; Holmes and Griffin, 2001), but has not been found by others (Graybiel and Lackner, 1980; Mullen et al., 1998). While the exact physiological effects of VIMS on heart rate require further investigation, it is most commonly assumed that a shared process in the autonomic nervous system is responsible for the association, consistent with a stress response (Harm, 2002; Keshavarz et al., 2014). We could not detect any differential effects of our experimental conditions on such a response, that is, the slight elevation of heart rate with increasing VIMS was the same for all visual reference conditions.
Furthermore, we observed a small but significant positive correlation between eye movements and VIMS ratings. While the correlational analysis did reveal a general relationship between FMS scores and scanpath length and -dispersion, this association was not modulated by the experimental condition. Many studies have investigated the role of nystagmus and MS (e.g., Quarck et al., 2000; Flanagan et al., 2002; Gupta, 2005). However, our objective was not to specifically investigate nystagmus, as it was dictated more or less by the TTC-task, but rather to look into general metrics of ocular motion. Webb and Griffin (2002) observed lower MS ratings when participants were asked to fixate in order to reduce their eye movements. Elbin et al. (2019) found that subjects with a higher susceptibility to MS also exhibited more saccadic eye movements. Ebenholtz et al. (1994) proposed that MS may be elicited by nystagmus in such a manner that the corresponding afferent signals stimulating the nervus vagus conjointly affect the adjacent nuclei vestibulares. This spill-over may hold an explanation of the interactions responsible for this association. Clearly, further research is required to understand the contribution of eye movements to VIMS.
This is the first study that investigates the relationship between visual TTC judgments and VIMS. We determined both the average absolute and constant errors when making TTC judgments. Participants performed the task very well. They were on average only about 70 ms too late in their judgments of the collision. This is remarkable given the unusual environment. For instance, Gray and Regan (2000) found larger errors in a non-provocative visual environment. This suggests that the VR-setup and the associated level of VIMS did not interfere with the TTC-task. Notwithstanding, there was a significant tendency to underestimate TTC a little more with increased VIMS. This result is compatible with studies showing that the emotional valence of the stimulus modulates TTC response times. Threatening stimuli caused TTC to be underestimated (Brendel et al., 2012) and Vagnoni et al. (2012), speculating that MS may have its evolutionary roots in the response to ingested toxins which constitutes a response to a threatening stimulus (Treisman, 1977).
Note, however, that the absolute TTC errors, which indicate variability of the TTC-judgments, were uncorrelated with VIMS.
All four conditions were presented subsequently in one session in a within-subjects design. As with every within-subjects design, carry-over effects are a considerable disadvantage; however, we presented the experimental conditions in different orders to reduce such carry-over effects while minimizing the number of drop-outs. The total exposure to the stimulus was limited to 14 min to make the experimental sessions tolerable, resulting in 3.5 min per condition. We acknowledge that this rather short stimulus duration per condition may have resulted in the overall low VIMS scores. We recommend for future studies to prolong the stimulus presentation to maximize the likelihood of experiencing stronger levels of VIMS with each experimental condition administered on different days. However, we believe that it is unlikely but conceivable that longer exposures would prompt participants to make better use of the earth-stable reference cues.
After extensive piloting, we had decided on a stimulus that was just provocative enough so subjects were likely to finish the experiment. Nonetheless, eight participants reported severe simulator sickness and terminated the experiment prematurely and were excluded from the data analysis. Consequently, only those who were able to finish all four experimental conditions were included in the data analysis, which likely explains the low sickness scores found in this study. This may limit the generalizability of our findings to mildly provocative stimulations. In other words, we acknowledge that these results are limited by low overall FMS ratings (MPeakFMS = 4.64) and should accordingly be interpreted with care. Future research could explore the possibility of an adaptive stimulus, whereby the frequency and amplitude of the random motion is altered according to participants' current FMS ratings, thereby increasing or decreasing motion to meet a target FMS-value. Analysis could incorporate this parameter as a time-varying covariate.
Would participants be able to benefit more from an earth-fixed reference cue when the task allows them to fixate this cue at all times? This may well be the case, but it is exceedingly difficult to provide such a cue and at the same time ensure that the provocative stimulus is not ignored. Our results do only speak to the case where the reference is provided next to a main task that draws most of the attentional resources.
This study adds to and qualifies the growing body of literature suggesting a beneficial effect of a visible horizon in the reduction of MS. We observed that the presence of a stationary earth-fixed horizon while performing a time-to-contact task in VR, significantly lowers VIMS, as measured by FMS-ratings. While the absolute difference in VIMS ratings between experimental groups was small, this effect could not be found for an earth-stationary fixation point, neither for a head-fixed horizontal line. Only the artificial horizontal line sufficed to provide sufficient rest-frame information. These results can be utilized when designing virtual environments.
The raw data supporting the conclusions of this article will be made available by the authors, without undue reservation.
Ethical review and approval was not required for the study on human participants in accordance with the local legislation and institutional requirements. The patients/participants provided their written informed consent to participate in this study.
WH: manuscript and data analysis. BK and HH: manuscript. All authors contributed to the article and approved the submitted version.
The authors declare that the research was conducted in the absence of any commercial or financial relationships that could be construed as a potential conflict of interest.
Bakdash, J. Z., and Marusich, L. R. (2017). Repeated measures correlation. Front. Psychol. 8:456. doi: 10.3389/fpsyg.2017.00456
Bonato, F., Bubka, A., and Thornton, W. (2015). Visual blur and motion sickness in an optokinetic drum. Aerospace Med. Hum. Perform. 86, 440–444. doi: 10.3357/AMHP.4105.2015
Brendel, E., DeLucia, P. R., Hecht, H., Stacy, R. L., and Larsen, J. T. (2012). Threatening pictures induce shortened time-to-contact estimates. Atten. Percept. Psychophys. 74, 979–987. doi: 10.3758/s13414-012-0285-0
Bruner, J. M. (1955). Seasickness in a destroyer escort squadron. US. Armed. Forces Med. J. 6, 469–490.
Burcham, P. M. (2002). Motion Sickness Literature Search (No. ARL-MR-504). Aberdeen Proving Ground, Maryland. Retrieved from: Army Research Laboratory website: https://apps.dtic.mil/sti/pdfs/ADA402963.pdf (accessed May 15, 2020).
Chen, D. J., Bao, B., Zhao, Y., and So, R. H. Y. (2016). Visually induced motion sickness when viewing visual oscillations of different frequencies along the fore-and-aft axis: keeping velocity versus amplitude constant. Ergonomics 59, 582–590. doi: 10.1080/00140139.2015.1078501
Comstock, J. R., Jones, L. C., and Pope, A. T. (2003). The effectiveness of various attitude indicator display sizes and extended horizon lines on attitude maintenance in a part-task simulation. Proc. Hum. Factors Ergon. Soc. Annu. Meet. 47, 144–148. doi: 10.1177/154193120304700130
Cowings, P. S., Suter, S., Toscano, W. B., Kamiya, J., and Naifeh, K. (1986). General autonomic components of motion sickness. Psychophysiology 23, 542–551. doi: 10.1111/j.1469-8986.1986.tb00671.x
Crampton, G. H. (1955). Studies of motion sickness. Xvii. Physiological changes accompanying sickness in man. J. Appl. Physiol. 7, 501–507. doi: 10.1152/jappl.1955.7.5.501
D'Amour, S., Bos, J. E., and Keshavarz, B. (2017). The efficacy of airflow and seat vibration on reducing visually induced motion sickness. Exp. Brain Res. 235, 2811–2820. doi: 10.1007/s00221-017-5009-1
Davis, S., Nesbitt, K., and Nalivaiko, E. (2014). “A systematic review of cybersickness,” in Proceedings of the 2014 Conference on Interactive Entertainment - IE2014, eds. K. Nesbitt, K. Blackmore, and S. P. Smith (New York, NY: ACM Press), 1–9.
De Muth, J. E. (2006). Basic Statistics and Pharmaceutical Statistical Applications, 2nd Edn. Biostatistics, Vol. 16. Boca Raton, FL: Chapman & Hall/CRC.
Doweck, I., Gordon, C. R., Shlitner, A., Spitzer, O., Gonen, A., Binah, O., et al. (1997). Alterations in R-R variability associated with experimental motion sickness. J. Auton. Nerv. Syst. 67, 31–37. doi: 10.1016/S0165-1838(97)00090-8
Duan, H., Zhai, G., Min, X., Zhu, Y., Sun, W., and Yang, X. (2018). “Assessment of visually induced motion sickness in immersive videos,” in Lecture Notes in Computer Science. Advances in Multimedia Information Processing – PCM 2017 Vol. 10735, eds. B. Zeng, Q. Huang, A. El Saddik, H. Li, S. Jiang, and X. Fan (Cham: Springer International Publishing), 662–672.
Ebenholtz, S. M., Cohen, M. M., and Linder, B. J. (1994). The possible role of nystagmus in motion sickness: a hypothesis. Aviat. Space Environ. Med. 65, 1032–1035.
Elbin, R. J., Kontos, A. P., Sufrinko, A., McElroy, M., Stephenson-Brown, K., Mohler, S., et al. (2019). Motion sickness susceptibility and baseline vestibular and ocular-motor performance in adolescent athletes. J. Athl. Train 54, 939–944. doi: 10.4085/1062-6050-347-18
Flanagan, M. B., May, J. G., and Dobie, T. G. (2002). Optokinetic nystagmus, vection, and motion sickness. Aviat. Space Environ. Med. 73, 1067–1073.
Gray, R., and Regan, D. (2000). Estimating the time to collision with a rotating nonspherical object. Vis. Res. 40, 49–63. doi: 10.1016/S0042-6989(99)00157-1
Graybiel, A., and Lackner, J. R. (1980). Evaluation of the relationship between motion sickness symptomatology and blood pressure, heart rate, and body temperature. Aviat. Space Environ. Med. 51, 211–214.
Guedry, F. E. (1965). Psychophysical studies of vestibular function. Contrip Sens. Physiol. 14, 63–135. doi: 10.1016/B978-1-4831-6746-6.50008-0
Guna, J., Geršak, G., Humar, I., Song, J., Drnovšek, J., and Pogačnik, M. (2019). Influence of video content type on users' virtual reality sickness perception and physiological response. Fut. Gener. Comput. Syst. 91, 263–276. doi: 10.1016/j.future.2018.08.049
Gupta, V. K. (2005). Motion sickness is linked to nystagmus-related trigeminal brain stem input: a new hypothesis. Med. Hypotheses 64, 1177–1181. doi: 10.1016/j.mehy.2004.11.031
Harm, D. L. (2002). “Motion sickness neurophysiology, physiological correlates, and treatment,” in Human Factors and Ergonomics. Handbook of Virtual Environments: Design, Implementation, and Applications, ed K. M. Stanney (Mahwah, NJ: Lawrence Erlbaum Associates), 637–662.
Harm, D. L., Parker, D. E., Reschke, M. F., and Skinner, N. C. (1998). Relationship between selected orientation rest frame, circular vection and space motion sickness. Brain Res. Bull. 47, 497–501. doi: 10.1016/S0361-9230(98)00096-3
Hemmerich, W. A., Shahal, A., and Hecht, H. (2019). Predictors of visually induced motion sickness in women. Displays 58, 27–32. doi: 10.1016/j.displa.2018.11.005
Holmes, S. R., and Griffin, M. J. (2001). Correlation between heart rate and the severity of motion sickness caused by optokinetic stimulation. J. Psychophysiol. 15, 35–42. doi: 10.1027//0269-8803.15.1.35
Hu, S., Grant, W. F., Stern, R. M., and Koch, K. L. (1991). Motion sickness severity and physiological correlates during repeated exposures to a rotating optokinetic drum. Aviat. Space Environ. Med. 62, 308–314.
Kennedy, R. S., Lane, N. E., Berbaum, K. S., and Lilienthal, M. G. (1993). Simulator sickness questionnaire: an enhanced method for quantifying simulator sickness. Int. J. Aviat. Psychol. 3, 203–220. doi: 10.1207/s15327108ijap0303_3
Kennedy, R. S., Lane, N. E., Lilienthal, M. G., Berbaum, K. S., and Hettinger, L. J. (1992). Profile analysis of simulator sickness symptoms: application to virtual environment systems. Presence 1, 295–301. doi: 10.1162/pres.1992.1.3.295
Keshavarz, B., and Hecht, H. (2011). Validating an efficient method to quantify motion sickness. Hum. Factors 53, 415–426. doi: 10.1177/0018720811403736
Keshavarz, B., Hecht, H., and Lawson, B. D. (2014). “Visually induced motion sickness: characteristics, causes, and countermeasures,” in Human Factors and Ergonomics Series. Handbook of Virtual Environments: Design, Implementation, and Applications, eds. K. S. Hale and K. M. Stanney (Boca Raton, FL: CRC Press), 648–697.
Keshavarz, B., Philipp-Muller, A. E., Hemmerich, W., Riecke, B. E., and Campos, J. L. (2019). The effect of visual motion stimulus characteristics on vection and visually induced motion sickness. Displays 58, 71–81. doi: 10.1016/j.displa.2018.07.005
Keshavarz, B., Riecke, B. E., Hettinger, L. J., and Campos, J. L. (2015). Vection and visually induced motion sickness: how are they related? Front. Psychol. 6:472. doi: 10.3389/fpsyg.2015.00472
Lackner, J. (1990). “Human orientation, adaptation, and movement control,” in Motion Sickness, Visual Displays, and Armored Vehicle Design: Proceedings of a Conference (Aberdeen Proving Ground, MD: Ballistic Research Laboratory).
Lin, J. J. W., Abi-Rached, H., and Lahav, M. (2004). “Virtual guiding avatar,” in Proceedings of the 2004 Conference on Human Factors in Computing Systems - CHI '04, eds. E. Dykstra-Erickson and M. Tscheligi (New York, NY: ACM Press), 719–726.
Malcolm, R. (1983). “The malcolm horizon – history and future,” in Proceedings of the Peripheral Vision Horizon Display (PVHD) Conference, ed R. S. Kellogg (Edwards, CA: NASA Conference Publication).
Mayo, A. M., Wade, M. G., and Stoffregen, T. A. (2011). Postural effects of the horizon on land and at sea. Psychol. Sci. 22, 118–124. doi: 10.1177/0956797610392927
McCauley, M. E., and Sharkey, T. J. (1992). Cybersickness: perception of self-motion in virtual environments. Presence 1, 311–318. doi: 10.1162/pres.1992.1.3.311
Mullen, T. J., Berger, R. D., Oman, C. M., and Cohen, R. J. (1998). Human heart rate variability relation is unchanged during motion sickness. J. Vestibular Res. 8, 95–105. doi: 10.3233/VES-1998-8113
Nooij, S. A. E., Pretto, P., Oberfeld, D., Hecht, H., and Bülthoff, H. H. (2017). Vection is the main contributor to motion sickness induced by visual yaw rotation: implications for conflict and eye movement theories. PLoS ONE 12:e0175305. doi: 10.1371/journal.pone.0175305
Oldenburg, M. (2018). “Kinetose und reisediarrhoe,” in Reisemedizin und Impfen: Empfehlungen für Ärzte, Betriebe und Beruflich Reisende, eds V. Harth, D.-M. Rose, S. Letzel, and D. Nowak (Landsberg am Lech: Ecomed Medizin), 96–106.
Pouke, M., Tiiro, A., LaValle, S. M., and Ojala, T. (2018). “Effects of visual realism and moving detail on cybersickness,” in 2018 IEEE Conference on Virtual Reality and 3D User Interfaces (VR), (IEEE), 665–666.
Prothero, J. D. (1998). The role of rest frames in vection, presence and motion sickness [Doctoral thesis]. University of Washington: Seattle, WA, United States.
Prothero, J. D., Draper, M. H., Furness, T. A., Parker, D. E., and Wells, M. J. (1999). The use of an independent visual background to reduce simulator side-effects. Aviation Space Environ. Med. 70(Pt .1), 277–283.
Prothero, J. D., and Parker, D. (2003). “A unified approach to presence and motion sickness,” in Virtual and Adaptive Environments, eds. L. Hettinger and M. Haas (CRC Press), 47–66.
Quarck, G., Etard, O., Oreel, M., and Denise, P. (2000). Motion sickness occurrence does not correlate with nystagmus characteristics. Neurosci. Lett. 287, 49–52. doi: 10.1016/S0304-3940(00)01140-X
Reason, J. T. (1969). Motion sickness—some theoretical considerations. Int. J. Man Mach. Stud. 1, 21–38. doi: 10.1016/S0020-7373(69)80009-X
Riccio, G. E., and Stoffregen, T. A. (1991). An ecological theory of motion sickness and postural instability. Ecol. Psychol. 3, 195–240. doi: 10.1207/s15326969eco0303_2
Rolnick, A., and Bles, W. (1989). Performance and well-being under tilting conditions: the effects of visual reference and artificial horizon. Aviat. Space Environ. Med. 60, 779–785.
Santini, T., Fuhl, W., and Kasneci, E. (2018). PuRe: Robust pupil detection for real-time pervasive eye tracking. Comput. Vis. Image Understand. 170, 40–50. doi: 10.1016/j.cviu.2018.02.002
Saredakis, D., Szpak, A., Birckhead, B., Keage, H. A. D., Rizzo, A., and Loetscher, T. (2020). Factors associated with virtual reality sickness in head-mounted displays: a systematic review and meta-analysis. Front. Hum. Neurosci. 14:96. doi: 10.3389/fnhum.2020.00096
Schroer, R. (2003). Cockpit Instruments: A century of powered flight:1903-2003. IEEE Aerospace Electron. Syst. Mag. 18, 13–18. doi: 10.1109/MAES.2003.1226529
Smart, L. J., Stoffregen, T. A., and Bardy, B. G. (2002). Visually induced motion sickness predicted by postural instability. Human Factors, 44, 451–465. doi: 10.1518/0018720024497745
Steele, J. E. (1961). Motion sickness and spatial perception. A theoretical study. ASD technical report. United States. Air Force. Systems command. Aeronaut. Syst. Div. 61:23. doi: 10.21236/AD0273602
Stern, R. M., Hu, S., Anderson, R. B., Leibowitz, H. W., and Koch, K. L. (1990). The effects of fixation and restricted visual field on vection-induced motion sickness. Aviation, Space, and Environmental Medicine, 61, 712–715. doi: 10.1016/0022-460x(91)90512-i
Stoffregen, T. A., and Smart, L. J. (1998). Postural instability precedes motion sickness. Brain Research Bulletin, 47, 437–448. doi: 10.1016/S0361-9230(98)00102-6
Stout, C. S., Toscano, W. B., and Cowings, P. S. (1995). Reliability of psychophysiological responses across multiple motion sickness stimulation tests. J. Vestibular Res. 5, 25–33. doi: 10.1016/0957-4271(94)00018-W
Streiner, D. L. (2000). Do you see what I mean? Indices of central tendency. Can. J. Psychiatry 45, 833–836. doi: 10.1177/070674370004500908
Tal, D., Gonen, A., Wiener, G., Bar, R., Gil, A., Nachum, Z., et al. (2012). Artificial horizon effects on motion sickness and performance. Otol. Neurotol. 33, 878–885. doi: 10.1097/MAO.0b013e318255ddab
Tal, D., Wiener, G., and Shupak, A. (2014). Mal de debarquement, motion sickness and the effect of an artificial horizon. J. Vestib. Res. 24, 17–23. doi: 10.3233/VES-130505
Treisman, M. (1977). Motion sickness: an evolutionary hypothesis. Science 197, 493–495. doi: 10.1126/science.301659
Turner, M., and Griffin, M. J. (1995). Motion sickness incidence during a round-the-world yacht race. Aviat. Space Environ. Med. 66, 849–856.
Vagnoni, E., Lourenco, S. F., and Longo, M. R. (2012). Threat modulates perception of looming visual stimuli. Curr. Biol. 22, R826–R827. doi: 10.1016/j.cub.2012.07.053
Virtual Escapes (2019). Jitter [Computer Software]. Unity Technologies. Retrieved from: https://assetstore.unity.com/packages/tools/animation/jitter-136861 (accessed April 12, 2020).
Webb, N. A., and Griffin, M. J. (2002). Optokinetic stimuli: motion sickness, visual acuity, and eye movements. Aviat. Space Environ. Med. 73, 351–358.
Keywords: reference information, motion sickness, visually induced motion sickness, virtual reality, artificial horizon, performance, time-to-contact (TTC)
Citation: Hemmerich W, Keshavarz B and Hecht H (2020) Visually Induced Motion Sickness on the Horizon. Front. Virtual Real. 1:582095. doi: 10.3389/frvir.2020.582095
Received: 10 July 2020; Accepted: 27 October 2020;
Published: 24 November 2020.
Edited by:
Kay Marie Stanney, Design Interactive, United StatesReviewed by:
Benjamin Weyers, University of Trier, GermanyCopyright © 2020 Hemmerich, Keshavarz and Hecht. This is an open-access article distributed under the terms of the Creative Commons Attribution License (CC BY). The use, distribution or reproduction in other forums is permitted, provided the original author(s) and the copyright owner(s) are credited and that the original publication in this journal is cited, in accordance with accepted academic practice. No use, distribution or reproduction is permitted which does not comply with these terms.
*Correspondence: Wanja Hemmerich, d2FoZW1tZXJAc3R1ZGVudHMudW5pLW1haW56LmRl; Heiko Hecht, aGVjaHRAdW5pLW1haW56LmRl
Disclaimer: All claims expressed in this article are solely those of the authors and do not necessarily represent those of their affiliated organizations, or those of the publisher, the editors and the reviewers. Any product that may be evaluated in this article or claim that may be made by its manufacturer is not guaranteed or endorsed by the publisher.
Research integrity at Frontiers
Learn more about the work of our research integrity team to safeguard the quality of each article we publish.