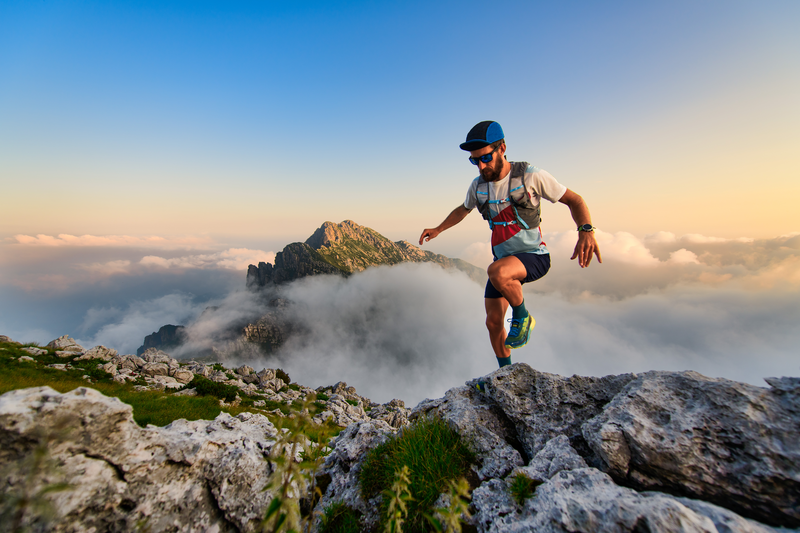
94% of researchers rate our articles as excellent or good
Learn more about the work of our research integrity team to safeguard the quality of each article we publish.
Find out more
ORIGINAL RESEARCH article
Front. Virol. , 24 January 2025
Sec. Viral Disease Investigation
Volume 4 - 2024 | https://doi.org/10.3389/fviro.2024.1542963
Background: Research from multiple regions indicates that Coronavirus disease of 2019 (COVID-19) pandemic has altered the epidemiological characteristics of respiratory viruses.
Methods: This single-center retrospective study included children hospitalized for lower respiratory tract infections (LRTIs) from 2017 to mid-2024. Data on respiratory syncytial virus (RSV), adenovirus (ADV), influenza A virus (IAV), influenza B virus (IBV), and parainfluenza virus (PIV)—were collected and analyzed across pre-pandemic, pandemic, and post-pandemic periods.
Results: A total of 59173 children were included. Detection rates were 34.97% (8553/24459) pre-pandemic, 21.93% (4621/21067) during the pandemic, and 21.64% (2953/13647) post-pandemic. Mixed infections rates significantly decreased during the pandemic (0.78%) and post-pandemic (0.78%) compared to pre-pandemic levels (1.63%) (P < 0.001). RSV was the most prevalent virus across all periods (P < 0.001). Following non-pharmaceutical interventions (NPIs) in 2020, detection rates declined significantly. After restrictions eased in 2021, RSV, PIV, and IBV surged, while IAV continued to decline until its resurgence in spring 2023 (P < 0.001). Certain viruses showed altered seasonal patterns: during the spring and summer of 2021, RSV detection rates exceeded those of previous years, while the expected winter 2022 peak was delayed by approximately six months. IAV peaks disappeared during winters of 2020 and 2021, peaking in spring 2023. IBV peaked in autumn 2021 and winter 2023. PIV had an unusual rise in summer 2020, peaking in autumn, delayed by about five months. The peak in the first half of 2024 returned to its normal timing. ADV appeared to be unaffected, with increased detection in 2022, and decreased in 2023 and 2024.
Conclusion: Epidemiological patterns of respiratory viruses have been altered by multiple influencing factors. The significance of vaccination, hygiene practices, public health interventions and etc. was emphasized in addressing pandemics. Additionally, ongoing dynamic monitoring is essential due to the evolving epidemiology.
Respiratory infections are recognized as one of the leading causes of morbidity and mortality among children worldwide (1). COVID-19 is caused by the severe acute respiratory syndrome coronavirus 2 (SARS-CoV-2). After its initial report in Wuhan at the end of 2019 (2), it rapidly spread worldwide, resulting in millions of hospitalizations and deaths. Various countries implemented a series of stringent NPIs, including, but not limited to, mask-wearing, hand hygiene, social distancing, school closures, travel restrictions, and lockdowns (3). From March 2020 to March 2021, up to one-quarter of countries enforced strict NPIs (4). While these measures successfully mitigated the transmission of COVID-19, they also suppressed the circulation of other pathogens transmitted via airborne or fecal-oral routes, leading to altered seasonal patterns and atypical epidemic peaks (5–8). However, studies have indicated that some characteristics of respiratory virus epidemiology exhibited new variations instead of previous patterns, including changes in infection severity, age distribution, subtypes, and overall trends (9–11). The potential for these changes to result in more severe epidemics in the future remains unknown.
This single-center retrospective study was conducted at the Department of Respiratory Medicine, Children’s Hospital of Chongqing Medical University. Hospitalized children (<18 years) with LRTIs from January 1st, 2017, to June 1st, 2024, were included. Exclusion criteria were: 1) severe deformities such as large atrial or ventricular septal defects, bronchopulmonary dysplasia, dextrocardia, and neuromuscular diseases; 2) malignant tumors, primary immunodeficiencies, or immunosuppressive therapy during hospitalization. Children with severe congenital cardiopulmonary deformities may exhibit increased susceptibility to viral infections due to anatomical abnormalities. Additionally, children with malignant tumors, immune deficiencies, or those receiving immunosuppressive therapy may experience immune dysfunction, resulting in infection patterns, clinical presentations, and disease severity that differ significantly from the general population. Excluding these special cases ensures the scientific rigor, reliability, and broader applicability of the results, thereby contributing to more accurate and meaningful conclusions.
Children were grouped by age (<1, 1-3, 3-6, >6 years). Study period was divided into: pre-pandemic (January 1st, 2017, to December 31st, 2019), pandemic (January 1st, 2020, to December 31st, 2021) and post-pandemic (January 1st, 2022, to May 31st, 2024), based on the implementation and relaxation of restrictions. Seasons were classified by Chinese climatic conditions: spring (March-May), summer (June-August), autumn (September-November), and winter (December-February).
Demographic characteristics (name, gender, age, admission date and clinical diagnosis) and laboratory test results of the enrolled patients was extracted. Specimens of nasopharyngeal aspirate or bronchoalveolar lavage fluid were collected by trained nurses within 24 hours of hospitalization, following standard operating procedures. Patients for tracheal aspiration were positioned semi-recumbent after finishing oral hygiene. Suction catheters and negative pressure (80-120 mmHg) were selected based on age. After a test suction, the catheter was inserted into the trachea to collect deep secretions. BALF collection adhered to the Guideline of pediatric flexible bronchoscopy in China (12). Saline (37°C, 1 ml/kg per instillation, ≤20 ml per session, total ≤5-10 ml/kg) is sequentially infused through the bronchoscope’s working channel, followed by aspiration with a negative pressure of 100-200 mmHg (ensuring bronchial patency) to obtain BALF. The reabsorption rate of each lavage fluid was ≥40%. Samples were immediately transported to the laboratory and processed stringently following standardized experimental protocols. Virus antigens were detected using a multiplex direct immunofluorescence assay (M-DIFA) kit or a multiplex real-time quantitative polymerase chain reaction (MRT-PCR) targeting viral nucleic acid fragments. Both methods have been demonstrated in numerous studies to exhibit high sensitivity (typically 85-95%) and specificity (>95%) for viral detection, and are widely used for detecting various viruses (13). During the study, minor adjustments were made to some methods. However, these changes did not significantly impact the virus detection rate. Previous studies have also shown no statistical difference in the positive detection rates between the two methods (14). RSV, ADV, IAV, IBV and PIV are routinely tested for at the Department of Respiratory Medicine, Children’s Hospital of Chongqing Medical University, whereas other viruses are not included in the standard screening of all admitted children. To ensure data completeness, only these five viruses were included in our study. All experimental procedures were strictly adhered to according to the hospital’s standard operating protocols to ensure consistency in the detection methods across different years.
The study protocol was approved by the Ethics Committee of Children’s Hospital of Chongqing Medical University (File No. (2023)263). As this study is an observational retrospective study, and respiratory pathogen testing is a standard routine examination for children admitted due to respiratory infections, written informed consent was not required.
Detection rates of the five viruses, were compared before, during, and after the COVID-19 pandemic. Trends in viral detection rates over time and correlations between viral detection rates and age were analyzed to determine the seasonal patterns and age-specific differences in viral infections.
Categorical variables were expressed as numbers and percentages (n, %), while continuous variables were summarized as median and interquartile range (IQR) [M (P25, P75)]. Continuous variables were analyzed using Kruskal-Wallis test and categorical variables were compared using either the Pearson chi-square test or Fisher’s exact test. Monte Carlo method was used for categorical variables whose theoretical number less than 5 and account for more than 20%. P < 0.05 was considered statistically significant. Statistical analyses were performed using SPSS version 27.0 (IBM, New York, USA), while Microsoft Excel 2021 and R 4.2.1 were utilized for data visualization.
According to the inclusion and exclusion criteria, a total of 59173 children were included, Among them, 24881 were younger than 1 year, 14773 were aged 1-3 years, 11475 were aged 3-6 years, and 8044 were older than 6 years. Overall, children under 3 years of age accounted for the majority of hospitalizations due to LRTIs, representing 77.4% pre-pandemic, 66% during the pandemic and 50% post-pandemic. The positive cases in each period accounted for the largest proportion of < 1 year old, accounting for more than half. Statistically significant differences across age groups were noted among virus-positive cases (P < 0.001), which were not observed in gender (P = 0.052, Table 1).
A total of 8,553 (34.97%), 4,621 (21.93%), and 2,953 (21.64%) cases were identified with respiratory viruses during the pre-pandemic, pandemic and post-pandemic, respectively. Significant statistical differences in detection rates were noted among the viruses across three periods (P < 0.001, Table 2). Specifically, RSV was detected in 9585 cases (16.20%), PIV in 4211 cases (7.12%), ADV in 1852 cases (3.13%), IAV in 888 cases (1.50%), and IBV in 265 cases (0.45%). RSV had the highest rates in all periods, accounting for 54.71%, 62.28%, and 68.68%, respectively.
Overall detection rates were the highest during pre-pandemic and slightly elevated during the post-pandemic relative to the pandemic period, with the exception of ADV, which exhibited a significantly lower detection rate during post-pandemic (0.25%) compared to the pandemic (1.36%). During the pre-pandemic, IAV was the predominant subtype, whereas IBV showed a slightly higher detection rate during the pandemic. A total of 670 co-infection cases (≥2 viruses detected) were observed, with detection numbers and detection rates of 399 (1.63%), 164 (0.78%), and 107 (0.78%) across the three periods, showing significant statistical differences (P < 0.001, Table 2).
The detection rates and counts of viruses in same seasons during the three periods were statistically different (P < 0.05, Table 3). Following the restrictions’ relaxation, an increase in RSV detection rates was noted during summer and autumn, while a decrease occurred in autumn and winter, contrasting with the trends observed for PIV. Additionally, increased detection rates of influenza viruses were found in spring and autumn.
RSV was identified as the most prevalent virus across all periods in <3 years group, followed by PIV (Figure 1). In >3 years group, ADV exhibited the highest detection rates during the pre-pandemic and pandemic, while RSV predominated during the post-pandemic. ADV constituted a low percentage (<5%) of detected viruses during the post-pandemic across all age groups.
Figure 1. Proportion of detected viruses. Numbers in parentheses indicate the total number of detections for each age group across three periods.
The overall detection rate of viruses was highest in the <1 year group, with rates of 46.52%, 31.45%, and 40.73% during three periods, respectively, showing a decline with increasing age (Figure 2A). Overall, the detection rates remained relatively low during pandemic. Detection rates were comparable to or slightly higher during post-pandemic than those during pandemic (Figures 2B, C, E, F). However, the detection rate of ADV significantly decreased after the relaxation of restrictions (Figure 2D).
Figure 2. The detection rates of each virus across three time periods (pre-pandemic, pandemic, post-pandemic) and four age groups (<1y, 1-3y, 3-6y, >6y). Blue represents pre-pandemic, red represents during the pandemic, and green represents post-pandemic. (A) Overall detection rates of viruses across three time periods and four age groups. (B) Changes in the detection rate of RSV across three time periods and four age groups. (C) Changes in the detection rate of PIV across three time periods and four age groups. (D) Changes in the detection rate of ADV across three time periods and four age groups. (E) Changes in the detection rate of IVA across three time periods and four age groups. (F) Changes in the detection rate of IVB across three time periods and four age groups.
Following NPIs in 2020, detection rates of five viruses declined significantly and seasonal patterns have changed (Figure 3A).
Figure 3. The temporal variation in virus detection rates from 2017.1.1-2024.6.1. Note. Horizontal axis represents time, with a label displayed every three months to indicate each season. Vertical axis indicates detection rate. (A) The fitting curves for the detection rates of different viruses. Different colors represent the detection rates of various viruses: yellow for ADV, red for IVA, orange for IVB, blue for PIV, and purple for RSV. The y-axis (detection rate) values are 0.0, 0.2, 0.4, 0.6, and 0.8. (B) The daily detection rate of RSV. The y-axis values are 0.0, 0.2, 0.4, 0.6, and 0.8. (C) The daily detection rate of ADV. The y-axis (detection rate) values are 0.0, 0.1, 0.2, 0.3, and 0.4. (D) The daily detection rate of PIV. The y-axis (detection rate) values are 0.0, 0.1, 0.2, 0.3, and 0.4. (E) The daily detection rate of IVA. The y-axis (detection rate) values are 0.0, 0.1, 0.2, and 0.3. (F) The daily detection rate of IVB. The y-axis (detection rate) values are 0.0, 0.05, and 0.15.
From 2017 to 2020, RSV epidemic peaks regularly occurred between November and February. Detection rates significantly declined from 36.00% in January to 12.85% in February 2020, remaining below 4% for the subsequent seven months, while the winter peak of 43.84% wasn’t lower than previous years. In spring and summer 2021, detection rates remained above 10%, exceeding those of previous years, while a decline was noted in spring and summer 2022 with an average of 1.0%(95% CI, 0.72-1.37%). The expected epidemic peak for autumn and winter 2022 was delayed until April and May 2023, with summer and autumn detection rates significantly higher than in previous years. A small peak in February 2024 reached only half of the levels of previous years (22.74%) (Figure 3B).
ADV exhibits a distinct seasonal pattern, peaking in summer and declining by March every four years (15). ADV was prevalent throughout 2019 [monthly average 8.69% (95% CI, 8.16-9.46%)], and showed a significant peak in summer 2019 (16.19%) and a notable decrease in spring 2020 [monthly average 0.72% (95% CI, 0.33-1.37%)], with no distinct peak. Detection rates increased in 2022 [monthly average 5.8% (95% CI, 5.22-6.35%)] and a summer peak was noted (12.5%). Throughout 2023, detection rates significantly declined [monthly average 0.20% (95% CI, 0.12-0.31%)], with recovery not observed until spring 2024 (Figure 3C).
The epidemic peaks of PIV from 2017 to 2019 regularly showed in spring and summer. Detection rates significantly declined in spring 2020 and resurged in August 2020, culminating in a peak in the autumn, approximately five months later. Following the dual peaks in July (12.23%) and October (11.6%) 2021, a marked decrease was observed. Furthermore, it peaked in summer 2023 at 16.97% and increased in May 2024(Figure 3D).
A significant decline in both IAV and IBV detection was observed in February 2020, with IAV almost undetectable throughout the pandemic. The seasonal pattern of IAV completely disappeared during the winters of 2020 and 2021, and the peak wasn’t observed until March 2023 (10.16%). In contrast, IBV detection rate increased in autumn 2021 and winter 2023 and slightly increased in the winter 2024, while remained at an almost negligible level at other time. (Figures 3E, F).
Previous studies have indicated that the prevalence of RSV starts from late summer in tropical regions, reaching temperate areas during winter, with the peak typically occurring between December and March in the Northern Hemisphere (16). The prevalence of influenza is about 0-3 months later than RSV, usually peaks in spring and winter. Additionally, ADV showed higher detection rates in North China during winter and spring, while southern regions exhibited peaks in summer (17) Furthermore, the common PIV 3 in China are usually prevalent from late spring to summer, with lower detection rates in winter (18, 19).The data in this study reveal that the epidemiological trends of the five respiratory viruses were distinct and regular before the pandemic. Specifically, the prevalence of RSV was observed from November to February, similar to influenza viruses. An overall high detection rate and a summer peak of ADV (16.19%) was observed during its popular year of 2019. PIV was prevalent during spring and summer.
NPIs implemented globally during 2020 significantly altered social behavior and disrupted the epidemiological patterns of respiratory viruses. Consequently, hospitalizations in children for LRTIs and respiratory virus detection rates sharply decreased in 2020. Our data showed that while the RSV winter peak in 2020 (43.84%) was not lower than previous years, the peak was absent in 2022. Researches from countries and regions, such as Asia, Europe and Australia, reported a delay of 3 to 12 months for the expected RSV peak in 2020 (5, 9, 20–28), with peaks occurring in spring to autumn 2021, exceeding previous years. The seasonal patterns of IAV and IBV were absent during the pandemic, and the disappearance in 2020 was similarly reported in the US, Italy, Australia, Europe and New Zealand (27, 29–32). The data from the Chongqing indicate that the influenza vaccination rates were 1.13% for 2017-2018, 0.67% for 2018-2019, 1.18% for 2019-2020, and 2.67% for 2020-2021. The decline in the vaccination rate during 2018-2019 was primarily attributed to the negative news, which led to a decrease in public confidence in the vaccine, resulting in a nationwide drop in influenza vaccination coverage. Notably, the substantial increase in vaccination rates in 2020-2021 may be linked to the heightened public health awareness triggered by the COVID-19 pandemic (33).In contrast, the detection rate of ADV remained stable as other non-enveloped viruses (34), peaking at 12.50% in the summer of 2022, consistent with global findings (27, 35–38). Finally, the detection rate of PIV significantly declined in 2020, with the spring-summer peak delayed by approximately five months until autumn, as observed in South Korea (39).
Circulation patterns of certain virus did not return to the previous and remained atypical. RSV’s abnormal peaks persisted, with debates on infection severity—some studies report worsened (10, 40), alleviated (9, 41, 42), or unchanged (43–45) symptoms, likely due to host differences. In children over 3, the dominant virus shifted from ADV to RSV, in line with Australian reports of increased RSV in older children (11, 46). IAV had a significant peak in March 2023, while IBV remained nearly absent, slightly increasing in winter 2024. Influenza infection rates are returning to pre-pandemic levels, with H3N2 dominant in 2022, replaced by H1N1 in early 2023 (35, 39, 47, 48). PIV detection remained low until it peaked at 16.97% in summer 2023, now returning to pre-pandemic trends (29).
Epidemiology changes of respiratory viruses may result from combined effects of the implementation of NPIs, population immune responses, viral interactions, viral mutations and etc. First, NPIs impact respiratory viruses (49), with non-enveloped viruses (e.g., ADV, rhinoviruses) being more resistant to inactivation by alcohol-based sanitizers (50, 51) and masks (52), than enveloped viruses like RSV and influenza. Second, stringent restrictions have prevented the population from getting natural infections, resulting in a lack of immune memory against pathogens, referred to as “immunological debt”, causing abnormal viral prevalence after restrictions were eased (53). The mechanisms underlying immune memory may include: immune evasion and viral mutation (such as SARS-CoV-2, which can evade immune detection by altering surface antigen structures or suppressing immune responses, as well as reducing host immune system recognition through antigen drift and antigenic recombination) (54); immune tolerance and chronic infection (for instance, immune debt may lead to long-term, low-level persistent infections, extending the duration of outbreaks, or it may induce immune tolerance in the host) (53); a decline in the herd immunity threshold (when herd immunity is not sufficiently stimulated to reach the threshold, leading to the vigorous spread of a new wave of the virus) (55, 56); delayed recovery of the immune system (i.e., when host symptoms resolve, the immune system may not have fully recovered, resulting in a weakened immune response to other viruses) (57); and the interaction between vaccination and immune debt (in regions with low vaccination coverage, immune debt may contribute to more frequent viral transmission). Third, viral interactions have been observed in both vivo and vitro (58). Influenza can suppress SARS-CoV-2 replication (58–60), while SARS-CoV-2 can affect other virus patterns. Competition mechanisms may include direct competition for host resources, superinfection exclusion (SIE), which refers to the phenomenon in which host cells initially infected by one pathogen block further infection by the same or other viruses (particularly similar viruses) for a period of time (61), and the suppression of other viruses by producing specific immune-suppressive molecules or modulating the host’s immune response (58). Homologous viruses may inhibit other subtypes or strains through cross-reactive immune responses (62), while heterologous viruses (e.g., RSV, coronaviruses, influenza) may restrict others via interferon production (63). Fourthly, continuous mutations alter viral traits, transmissibility and epidemiological patterns (64), explaining changes like delayed RSV peaks and the disappearance of influenza (65–71). These complex factors complicate future respiratory infection predictions in children. Vaccination not only enhances immune memory, providing immediate protection, but also contributes to the maintenance of long-term immunity, thereby reducing the burden of viral infections. Thus, increasing immunity levels, boosting vaccination rates, and prioritizing the protection of high-risk populations may effectively mitigate the public health risks associated with immune debt. Additionally, the integration of other public health interventions will further strengthen the immune barrier, reducing disease burden and the risk of epidemic resurgence. Vaccination represents the core strategy for addressing immune debt (72, 73). In addition, continuous dynamic monitoring is also vitally important.
This study is the first to systematically collect and analyze epidemiological data on common respiratory viruses among children in Chongqing, while also summarizing findings from similar global research. However, several limitations were identified. First, as a single-center retrospective study conducted at the Children’s Hospital of Chongqing Medical University, the results may not be generalizable to a broader population. Large-scale multicenter studies are needed to provide more comprehensive insights for the prevention and control of respiratory viral infections. Second, the study only included the five most common viruses identified in the hospital’s respiratory department, limiting its scope. Future research should aim to include a wider range of viruses and subtypes for a more complete analysis.
This study systematically collected and analyzed epidemiological data on common respiratory viruses among children in Chongqing, summarizing global research findings. However, limitations include being a single-center retrospective study, which may not represent a larger population. Large-scale multicenter studies are needed for broader insights. Additionally, only the five most common viruses at the hospital’s respiratory department were included, so future research should analyze more viruses and subtypes.
This study included 59,173 hospitalized children with LRTIs from 2017 to mid-2024, analyzing the impact of COVID-19 pandemic on pediatric respiratory infection epidemiology in combination with relevant global studies. NPIs were found to affect the seasonal patterns of viruses like RSV and influenza. Potential explanations for atypical epidemics after the relaxation of restrictions were discussed. Whether previous epidemic patterns will return or new patterns will be formed requires further study. The importance of vaccination, hygiene practices, and public health interventions was emphasized. Additionally, continuous dynamic monitoring is crucial to address evolving viral epidemiology.
All data generated or analyzed in this study are included in the article, further inquiries can be directed to the corresponding author.
The studies involving humans were approved by the Ethics Committee of Children’s Hospital of Chongqing Medical University (File No. (2023)263). The studies were conducted in accordance with the local legislation and institutional requirements. As this study is an observational retrospective study, and respiratory pathogen testing is a standard routine examination for children admitted due to respiratory infections, written informed consent was not required.
TG: Conceptualization, Data curation, Writing – original draft. FH: Conceptualization, Data curation, Writing – original draft. YZ: Conceptualization, Data curation, Writing – original draft. SL: Conceptualization, Data curation, Writing – original draft. DT: Conceptualization, Data curation, Supervision, Writing – review & editing.
The author(s) declare financial support was received for the research, authorship, and/or publication of this article. Funding for the study was provided by the National Natural Science Foundation of China (grant number: 81870012) and the National Natural Science Foundation of China (grant number: 82171712), Joint project of Chongqing Health Commission and Science and Technology Bureau, No. 2022MSXM043.
The authors declare that the research was conducted in the absence of any commercial or financial relationships that could be construed as a potential conflict of interest.
The author(s) declare that no Generative AI was used in the creation of this manuscript.
All claims expressed in this article are solely those of the authors and do not necessarily represent those of their affiliated organizations, or those of the publisher, the editors and the reviewers. Any product that may be evaluated in this article, or claim that may be made by its manufacturer, is not guaranteed or endorsed by the publisher.
1. GBD 2019 Diseases and Injuries Collaborators, Vos T, Lim SS, Abbafati C, Abbas KM, Abbasi M, et al. Global burden of 369 diseases and injuries in 204 countries and territories, 1990-2019: a systematic analysis for the Global Burden of Disease Study 2019. Lancet. (2020) 396:1204–22. doi: 10.1016/S0140-6736(20)30925-9
2. Wu F, Zhao S, Yu B, Chen YM, Wang W, Song ZG, et al. A new coronavirus associated with human respiratory disease in China. Nature. (2020) 579:265–9. doi: 10.1038/s41586-020-2008-3
3. Chow EJ, Uyeki TM, Chu HY. The effects of the COVID-19 pandemic on community respiratory virus activity. Nat Rev Microbiol. (2023) 21:195–210. doi: 10.1038/s41579-022-00807-9
4. Phillips T, Zhang Y, Petherick A. A year of living distantly: global trends in the use of stay-at-home orders over the first 12 months of the COVID-19 pandemic. Interface Focus. (2021) 11:20210041. doi: 10.1098/rsfs.2021.0041
5. Burrell R, Saravanos G, Britton PN. Unintended impacts of COVID-19 on the epidemiology and burden of paediatric respiratory infections. Paediatr Respir Rev. (2023) S1526-0542(23)00044-1. doi: 10.1016/j.prrv.2023.07.004
6. Karbuz A, İşançlı DK, Kılıçaslan Ö, Kırmacı Ç, Emre I, Beşel L, et al. The effects of measures taken during the COVID-19 pandemic on the seasonal dynamics of respiratory viruses in children. Turk J Pediatr. (2023) 65:592–602. doi: 10.24953/turkjped.2023.154
7. Galli C, Pellegrinelli L, Giardina F, Ferrari G, Uceda Renteria SC, Novazzi F, et al. On the lookout for influenza viruses in Italy during the 2021-2022 season: Along came A(H3N2) viruses with a new phylogenetic makeup of their hemagglutinin. Virus Res. (2023) 324:199033. doi: 10.1016/j.virusres.2022.199033
8. Graziani A, Bozza S, Borghi M, Mencacci A, Camilloni B. Circulation and seasonality of respiratory viruses in hospitalized patients during five consecutive years (2019-2023) in Perugia, Italy. Viruses. (2024) 16(9):1394. doi: 10.3390/v16091394
9. Jia R, Lu L, Su L, Lin Z, Gao D, Lv H, et al. Resurgence of respiratory syncytial virus infection during COVID-19 pandemic among children in shanghai, China. Front Microbiol. (2022) 13:938372. doi: 10.3389/fmicb.2022.938372
10. Nygaard U, Hartling UB, Nielsen J, Vestergaard LS, Dungu KHS, Nielsen JSA, et al. Hospital admissions and need for mechanical ventilation in children with respiratory syncytial virus before and during the COVID-19 pandemic: a Danish nationwide cohort study. Lancet Child Adolesc Health. (2023) 7:171–9. doi: 10.1016/S2352-4642(22)00371-6
11. Rice E, Oakes DB, Holland C, Moore HC, Blyth CC. Respiratory syncytial virus in children: epidemiology and clinical impact post-COVID-19. Curr Opin Infect Dis. (2023) 36:522–8. doi: 10.1097/QCO.0000000000000967
12. Experts Group of Pediatric Respiratory Endoscopy, Talent Exchange Service Center of National Health Commission, Endoscopy Committee, Pediatric Section of Chinese Medical Doctor Association, Pediatric Respiratory Endoscopy Committee, Endoscopists Section of Chinese Medical Doctor Association, et al. Guideline of pediatric flexible bronchoscopy in China(2018 version). Chin J Appl Clin Pediatr. (2018) 33:983–9. doi: 10.3760/cma.j.issn.2095-428X.2018.13.006
13. Rodrigues AC, Marcusso RMN, Souza DN, Fahl WO, Caporale GMM, Macedo CI, et al. A comparative study of direct fluorescent antibody, mouse inoculation, and tissue culture infection testing for rabies diagnoses. J Virol Methods. (2022) 300:114426. doi: 10.1016/j.jviromet.2021.114426
14. Choudhary ML, Anand SP, Tikhe SA, Walimbe AM, Potdar VA, Chadha MS, et al. Comparison of the conventional multiplex RT-PCR, real time RT-PCR and Luminex xTAG® RVP fast assay for the detection of respiratory viruses. J Med Virol. (2016) 88:51–7. doi: 10.1002/jmv.24299
15. Chen Y, Lin T, Wang CB, Liang WL, Lian GW, Zanin M, et al. Human adenovirus (HAdV) infection in children with acute respiratory tract infections in Guangzhou, China, 2010-2021: a molecular epidemiology study. World J Pediatr. (2022) 18:545–52. doi: 10.1007/s12519-022-00590-w
16. Li Y, Reeves RM, Wang X, Bassat Q, Brooks WA, Cohen C, et al. Global patterns in monthly activity of influenza virus, respiratory syncytial virus, parainfluenza virus, and metapneumovirus: a systematic analysis. Lancet Glob Health. (2019) 7:e1031–45. doi: 10.1016/S2214-109X(19)30264-5
17. Liu MC, Xu Q, Li TT, Wang T, Jiang BG, Lv CL. Prevalence of human infection with respiratory adenovirus in China: A systematic review and meta-analysis. PloS Negl Trop Dis. (2023) 17:e0011151. doi: 10.1371/journal.pntd.0011151
18. Xiao NG, Duan ZJ, Xie ZP, Zhong LL, Zeng SZ, Huang H, et al. Human parainfluenza virus types 1-4 in hospitalized children with acute lower respiratory infections in China. J Med Virol. (2016) 88:2085–91. doi: 10.1002/jmv.v88.12
19. Ren L, Gonzalez R, Xie Z, Xiong Z, Liu C, Xiang Z, et al. Human parainfluenza virus type 4 infection in Chinese children with lower respiratory tract infections: a comparison study. J Clin Virol. (2011) 51:209–12. doi: 10.1016/j.jcv.2011.05.001
20. Olsen SJ, Winn AK, Budd AP, Prill MM, Steel J, Midgley CM, et al. Changes in influenza and other respiratory virus activity during the COVID-19 pandemic-United States, 2020-2021. Am J Transplant. (2021) 21:3481–6. doi: 10.1111/ajt.16049
21. Van Brusselen D, De Troeyer K, Ter Haar E, Vander Auwera A, Poschet K, Van Nuijs S, et al. Bronchiolitis in COVID-19 times: a nearly absent disease? Eur J Pediatr. (2021) 180:1969–73. doi: 10.1007/s00431-021-03968-6
22. Maison N, Peck A, Illi S, Meyer-Buehn M, von Mutius E, Hübner J, et al. The rising of old foes: impact of lockdown periods on “non-SARS-CoV-2” viral respiratory and gastrointestinal infections. Infection. (2022) 50:519–24. doi: 10.1007/s15010-022-01756-4
23. Hönemann M, Thiem S, Bergs S, Berthold T, Propach C, Siekmeyer M, et al. In-Depth Analysis of the Re-Emergence of Respiratory Syncytial Virus at a Tertiary Care Hospital in Germany in the Summer of 2021 after the Alleviation of Non-Pharmaceutical Interventions Due to the SARS-CoV-2 Pandemic. Viruses. (2023) 15(4):877. doi: 10.3390/v15040877
24. Bardsley M, Morbey RA, Hughes HE, Beck CR, Watson CH, Zhao H, et al. Epidemiology of respiratory syncytial virus in children younger than 5 years in England during the COVID-19 pandemic, measured by laboratory, clinical, and syndromic surveillance: a retrospective observational study. Lancet Infect Dis. (2023) 23:56–66. doi: 10.1016/S1473-3099(22)00525-4
25. Bennet R, Rinder MR, George E, Hertting O, Luthander J, Åkefeldt SO, et al. Pre-admission virus detection during the COVID-19 pandemic in children with and without symptoms of infection. Acta Paediatr. (2024) 113:1679–84. doi: 10.1111/apa.v113.7
26. Casalegno JS, Ploin D, Cantais A, Masson E, Bard E, Valette M, et al. Characteristics of the delayed respiratory syncytial virus epidemic, 2020/2021, Rhône Loire, France. Euro Surveill. (2021) 26(29):2100630. doi: 10.2807/1560-7917.ES.2021.26.29.2100630
27. Shen DP, Vermeulen F, Debeer A, Lagrou K, Smits A. Impact of COVID-19 on viral respiratory infection epidemiology in young children: A single-center analysis. Front Public Health. (2022) 10:931242. doi: 10.3389/fpubh.2022.931242
28. Anglemyer A, et al. Unusual Interseasonal RSV Activity in the Southern and Northern Hemispheres. J Infect Dis. (2022) 225(9):1680–2. doi: 10.1093/infdis/jiab620
29. Olsen SJ, Winn AK, Budd AP, Prill MM, Steel J, Midgley CM. Changes in influenza and other respiratory virus activity during the COVID-19 pandemic - United States, 2020-2021. MMWR Morb Mortal Wkly Rep. (2021) 70:1013–9. doi: 10.15585/mmwr.mm7029a1
30. Hills T, Kearns N, Kearns C, Beasley R. Influenza control during the COVID-19 pandemic. Lancet. (2020) 396:1633–4. doi: 10.1016/S0140-6736(20)32166-8
31. Soo RJJ, Chiew CJ, Ma S, Pung R, Lee V. Decreased influenza incidence under COVID-19 control measures, Singapore. Emerg Infect Dis. (2020) 26:1933–5. doi: 10.3201/eid2608.201229
32. Melidou As, Pereyaslov D, Hungnes O, Prosenc K, Alm E, Adlhoch C, et al. Virological surveillance of influenza viruses in the WHO European Region in 2019/20 - impact of the COVID-19 pandemic. Euro Surveill. (2020) 25(46):2001822. doi: 10.2807/1560-7917.ES.2020.25.46.2001822
33. Zhu L, Luo T, Yuan Y, Yang S, Niu C, Gong T, et al. Epidemiological characteristics of respiratory viruses in hospitalized children during the COVID-19 pandemic in southwestern China. Front Cell Infect Microbiol. (2023) 13:1142199. doi: 10.3389/fcimb.2023.1142199
34. Kim HM, Lee EJ, Lee NJ, Woo SH, Kim JM, Rhee JE, et al. Impact of coronavirus disease 2019 on respiratory surveillance and explanation of high detection rate of human rhinovirus during the pandemic in the Republic of Korea. Influenza Other Respir Viruses. (2021) 15:721–31. doi: 10.1111/irv.12894
35. Cho HJ, Rhee JE, Kang D, Choi EH, Lee NJ, Woo S, et al. Epidemiology of respiratory viruses in korean children before and after the COVID-19 pandemic: A prospective study from national surveillance system. J Korean Med Sci. (2024) 39:e171. doi: 10.13048/jkm.24002
36. Hirotsu Y, Nagakubo Y, Maejima M, Shibusawa M, Hosaka K, Sueki H, et al. Changes in viral dynamics following the legal relaxation of COVID-19 mitigation measures in Japan from children to adults: A single center study, 2020-2023. Influenza Other Respir Viruses. (2024) 18:e13278. doi: 10.1111/irv.13278
37. Wu Y, Zhou J, Shu T, Li W, Shang S, Du L, et al. Epidemiological study of post-pandemic pediatric common respiratory pathogens using multiplex detection. Virol J. (2024) 21:168. doi: 10.1186/s12985-024-02441-8
38. Presti S, Manti S, Gambilonghi F, Parisi GF, Papale M, Leonardi S. Comparative analysis of pediatric hospitalizations during two consecutive influenza and respiratory virus seasons post-pandemic. Viruses. (2023) 15(9):1825. doi: 10.3390/v15091825
39. Yum S, Hong K, Sohn S, Kim J, Chun BC. Trends in viral respiratory infections during COVID-19 pandemic, South Korea. Emerg Infect Dis. (2021) 27:1685–8. doi: 10.3201/eid2706.210135
40. Ide N, Tabata K, Tokuma N, Murano Y, Yoneoka D, Nakazawa T, et al. Clinical Features of RS Virus Infection before, during, and after COVID-19 Pandemic. Children (Basel). (2024) 11(8):922. doi: 10.3390/children11080922
41. Fourgeaud J, Toubiana J, Chappuy H, Delacourt C, Moulin F, Parize P, et al. Impact of public health measures on the post-COVID-19 respiratory syncytial virus epidemics in France. Eur J Clin Microbiol Infect Dis. (2021) 40:2389–95. doi: 10.1007/s10096-021-04323-1
42. Ozeki S, Kawada JI, Yamashita D, Yasufuku C, Akano T, Kato M, et al. Impact of the coronavirus disease 2019 pandemic on the clinical features of pediatric respiratory syncytial virus infection in Japan. Open Forum Infect Dis. (2022) 9:ofac562. doi: 10.1093/ofid/ofac562
43. Abushahin A, Toma H, Alnaimi A, Abu-Hasan M, Alneirab A, Alzoubi H, et al. Impact of COVID−19 pandemic restrictions and subsequent relaxation on the prevalence of respiratory virus hospitalizations in children. BMC Pediatr. (2024) 24:91. doi: 10.1186/s12887-024-04566-9
44. Stera G, Pierantoni L, Masetti R, Leardini D, Biagi C, Buonsenso D, et al. Impact of SARS-coV-2 pandemic on bronchiolitis hospitalizations: the experience of an italian tertiary center. Children (Basel). (2021) 8(7):556. doi: 10.3390/children8070556
45. Camporesi A, Morello R, Ferro V, Pierantoni L, Rocca A, Lanari M, et al. Epidemiology, microbiology and severity of bronchiolitis in the first post-lockdown cold season in three different geographical areas in Italy: A prospective, observational study. Children (Basel). (2022) 9(4):491. doi: 10.3390/children9040491
46. Rao S, Armistead I, Messacar K, Alden NB, Schmoll E, Austin E, et al. Shifting epidemiology and severity of respiratory syncytial virus in children during the COVID-19 pandemic. JAMA Pediatr. (2023) 177:730–2. doi: 10.1001/jamapediatrics.2023.1088
47. Kandeel A, Fahim M, Deghedy O, Roshdy WH, Khalifa MK, Shesheny RE, et al. Resurgence of influenza and respiratory syncytial virus in Egypt following two years of decline during the COVID-19 pandemic: outpatient clinic survey of infants and children, October 2022. BMC Public Health. (2023) 23:1067. doi: 10.1186/s12889-023-15880-9
48. Luštrek M, Cesar Z, Suljič A, Kogoj R, Knap N, Virant MJ, et al. Influenza A, Influenza B, human respiratory syncytial virus and SARSCoV-2 molecular diagnostics and epidemiology in the post COVID-19 era. Respir Res. (2024) 25:234. doi: 10.1186/s12931-024-02862-7
49. Leung NHL. Transmissibility and transmission of respiratory viruses. Nat Rev Microbiol. (2021) 19:528–45. doi: 10.1038/s41579-021-00535-6
50. Chen AP, Chu IY, Yeh ML, Chen YY, Lee CL, Lin HH, et al. Differentiating impacts of non-pharmaceutical interventions on non-coronavirus disease-2019 respiratory viral infections: Hospital-based retrospective observational study in Taiwan. Influenza Other Respir Viruses. (2021) 15:478–87. doi: 10.1111/irv.12858
51. Howie R, Alfa MJ, Coombs K. Survival of enveloped and non-enveloped viruses on surfaces compared with other micro-organisms and impact of suboptimal disinfectant exposure. J Hosp Infect. (2008) 69:368–76. doi: 10.1016/j.jhin.2008.04.024
52. Leung NHL, Chu DKW, Shiu EYC, Chan KH, McDevitt JJ, Hau BJP, et al. Respiratory virus shedding in exhaled breath and efficacy of face masks. Nat Med. (2020) 26:676–80. doi: 10.1038/s41591-020-0843-2
53. Munro AP, Jones CE. Immunity debt and unseasonal childhood respiratory viruses. Br J Hosp Med (Lond). (2022) 83:1–3. doi: 10.12968/hmed.2022.0349
54. Minkoff JM, tenOever B. Innate immune evasion strategies of SARS-CoV-2. Nat Rev Microbiol. (2023) 21:178–94. doi: 10.1038/s41579-022-00839-1
55. Cohen R, Levy C, Rybak A, Angoulvant F, Ouldali N, Grimprel E, et al. Immune debt: Recrudescence of disease and confirmation of a contested concept. Infect Dis Now. (2023) 53:104638. doi: 10.1016/j.idnow.2022.12.003
56. Buchwald AG, Graham BS, Traore A, Haidara FC, Chen M, Morabito K, et al. Respiratory syncytial virus (RSV) neutralizing antibodies at birth predict protection from RSV illness in infants in the first 3 months of life. Clin Infect Dis. (2021) 73:e4421–7. doi: 10.1093/cid/ciaa648
57. Cohen R, Ashman M, Taha MK, Varon E, Angoulvant F, Levy C, et al. Pediatric Infectious Disease Group (GPIP) position paper on the immune debt of the COVID-19 pandemic in childhood, how can we fill the immunity gap? Infect Dis Now. (2021) 51:418–23. doi: 10.1016/j.idnow.2021.05.004
58. Piret J, Boivin G. Viral interference between respiratory viruses. Emerg Infect Dis. (2022) 28:273–81. doi: 10.3201/eid2802.211727
59. Deleveaux S, Clarke-Kregor A, Fonseca-Fuentes X, Mekhaiel E. Exploring the possible phenomenon of viral interference between the novel coronavirus and common respiratory viruses. J Patient Cent Res Rev. (2023) 10:91–7. doi: 10.17294/2330-0698.1995
60. Cheemarla NR, Watkins TA, Mihaylova VT, Foxman EF. Viral interference during influenza A-SARS-coV-2 coinfection of the human airway epithelium and reversal by oseltamivir. J Infect Dis. (2024) 229:1430–4. doi: 10.1093/infdis/jiad402
61. Adams RH, Brown DT. BHK cells expressing Sindbis virus-induced homologous interference allow the translation of nonstructural genes of superinfecting virus. J Virol. (1985) 54:351–7. doi: 10.1128/jvi.54.2.351-357.1985
62. Laurie KL, Horman W, Carolan LA, Chan KF, Layton D, Bean A, et al. Evidence for viral interference and cross-reactive protective immunity between influenza B virus lineages. J Infect Dis. (2018) 217:548–59. doi: 10.1093/infdis/jix509
63. Chan KF, Carolan LA, Korenkov D, Druce J, McCaw J, Reading PC, et al. Investigating viral interference between influenza A virus and human respiratory syncytial virus in a ferret model of infection. J Infect Dis. (2018) 218:406–17. doi: 10.1093/infdis/jiy184
64. Principi N, Autore G, Ramundo G, Esposito S. Epidemiology of respiratory infections during the COVID-19 pandemic. Viruses. (2023) 15(5):1160. doi: 10.3390/v15051160
65. Dolores A, Stephanie G, Mercedes S NJ, Érica G, Mistchenko AS, Mariana V, et al. RSV reemergence in Argentina since the SARS-CoV-2 pandemic. J Clin Virol. (2022) 149:105126. doi: 10.1016/j.jcv.2022.105126
66. Eden JS, Sikazwe C, Xie R, Deng YM, Sullivan SG, Michie A, et al. Off-season RSV epidemics in Australia after easing of COVID-19 restrictions. Nat Commun. (2022) 13:2884. doi: 10.1038/s41467-022-30485-3
67. Lee CY, Wu TH, Fang YP, Chang JC, Wang HC, Lin SJ, et al. Delayed respiratory syncytial virus outbreak in 2020 in Taiwan was correlated with two novel RSV-A genotype ON1 variants. Influenza Other Respir Viruses. (2022) 16:511–20. doi: 10.1111/irv.12951
68. Corman VM, Landt O, Kaiser M, Molenkamp R, Meijer A, Chu DK, et al. Detection of 2019 novel coronavirus (2019-nCoV) by real-time RT-PCR. Euro Surveill. (2020) 25(14). doi: 10.2807/1560-7917.ES.2020.25.3.2000045
69. Cai W, Köndgen S, Tolksdorf K, Dürrwald R, Schuler E, Biere B, et al. Atypical age distribution and high disease severity in children with RSV infections during two irregular epidemic seasons throughout the COVID-19 pandemic, Germany, 2021 to 2023. Euro Surveill. (2024) 29(13):2300465. doi: 10.2807/1560-7917.ES.2024.29.13.2300465
70. Rojo-Alba S, Martínez ZP, González-Alba JM, Boga JA, Varela CO, Álvarez MAA, et al. Respiratory syncytial virus incidence and typing in the last six seasons in the north of Spain (Asturias). Genetic characterization during the SARS-CoV-2 pandemic. J Med Virol. (2024) 96:e29499. doi: 10.1002/jmv.29499
71. Fortunato F, Campanozzi A, Maffei G, Arena F, Carri VD, Rollo T, et al. Respiratory syncytial virus-associated hospitalizations among children: an Italian retrospective observational study. Ital J Pediatr. (2024) 50:45. doi: 10.1186/s13052-024-01617-w
72. Doherty M, Buchy P, Standaert B, Giaquinto C, Prado-Cohrs D. Vaccine impact: Benefits for human health. Vaccine. (2016) 34:6707–14. doi: 10.1016/j.vaccine.2016.10.025
Keywords: COVID-19, non-pharmaceutical interventions, respiratory virus, epidemiology, pediatrics, respiratory syncytial virus
Citation: Gong T, He F, Zhao Y, Lu S and Tian D (2025) Impact of COVID-19 pandemic restrictions and relaxation on pediatric respiratory virus hospitalizations in Southwest China: a retrospective study. Front. Virol. 4:1542963. doi: 10.3389/fviro.2024.1542963
Received: 16 December 2024; Accepted: 30 December 2024;
Published: 24 January 2025.
Edited by:
Haider Al-Hello, Finnish Institute for Health and Welfare, FinlandReviewed by:
José J. Leija-Martínez, Autonomous University of San Luis Potosí, MexicoCopyright © 2025 Gong, He, Zhao, Lu and Tian. This is an open-access article distributed under the terms of the Creative Commons Attribution License (CC BY). The use, distribution or reproduction in other forums is permitted, provided the original author(s) and the copyright owner(s) are credited and that the original publication in this journal is cited, in accordance with accepted academic practice. No use, distribution or reproduction is permitted which does not comply with these terms.
*Correspondence: Daiyin Tian, dF9keUAxNjMuY29t
Disclaimer: All claims expressed in this article are solely those of the authors and do not necessarily represent those of their affiliated organizations, or those of the publisher, the editors and the reviewers. Any product that may be evaluated in this article or claim that may be made by its manufacturer is not guaranteed or endorsed by the publisher.
Research integrity at Frontiers
Learn more about the work of our research integrity team to safeguard the quality of each article we publish.