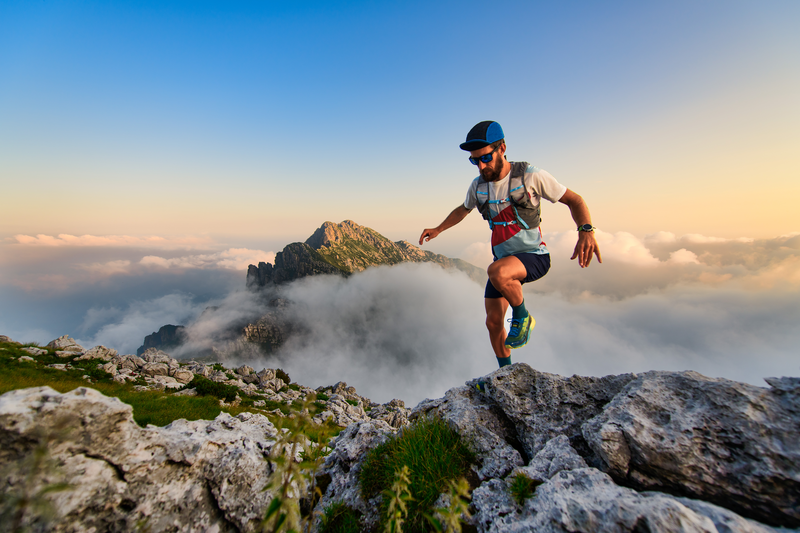
95% of researchers rate our articles as excellent or good
Learn more about the work of our research integrity team to safeguard the quality of each article we publish.
Find out more
ORIGINAL RESEARCH article
Front. Virol. , 08 November 2024
Sec. Virus and Host Immunity
Volume 4 - 2024 | https://doi.org/10.3389/fviro.2024.1479645
This article is part of the Research Topic Innate and Adaptive Immune Responses to Viral Infection View all 8 articles
Introduction: Seroprevalence of anti-severe acute respiratory syndrome coronavirus 2 (anti-SARS-CoV-2) antibodies in the postvaccination period in Kenya remains to be elucidated. This study aimed to determine the seroprevalence of anti-SARS-CoV-2 IgM and IgG and evaluate Covid-19 vaccination uptake in a university setting in Nairobi.
Methods: This cross-sectional study assayed serum anti-SARS-CoV-2 IgM and IgG levels using enzyme-linked immunosorbent assays. A structured questionnaire was used to determine vaccine uptake, vaccine hesitancy and reasons for hesitancy.
Results: A total of 189 participants were enrolled (median age, 21 years; female, 50.8%). The seroprevalence of anti-SARS-CoV-2 was 12.7% for IgM and 87.8% for IgG. Anti-SARS-CoV-2 IgG titers were higher among the vaccinated vs. non-vaccinated individuals (p < 0.001, U = 2817.5), females vs. males (p = 0.024, U = 3616), and those vaccinated ≤ 6 months before the study vs. those vaccinated >1 year earlier (p = 0.002, H = 12.359). The vaccination hesitancy rate was 43.4% and the underlying reasons included mistrust (22.4%), health concerns (19.7%), and lack of information (18.4%).
Discussion: The high seroprevalence of anti-SARS-CoV-2 IgG is an indication of high exposure to SARS-CoV-2 either through natural infection or through vaccination. The high vaccine hesitancy noted necessitates community engagement, and public education to dispel myths and misinformation prior to roll out of new vaccines and other health interventions.
Coronavirus disease 2019 (COVID-19), caused by severe acute respiratory syndrome coronavirus 2 (SARS-CoV-2), remains a public health problem, even though the pandemic has been controlled effectively across the world. Since the first case of COVID-19 was reported in Kenya in March 13, 2020, a total of 344,130 COVID-19 cases, 5,689 deaths, and 337,309 recoveries have been confirmed, with approximately 1,132 ongoing infections reported as of April 12, 2024 (1). Researchers have acknowledged that the reported numbers are a gross underestimation of the actual figures (2). To inform infection control strategies, such as vaccination, studying the seroprevalence of anti-SARS-CoV-2 IgG and IgM could help to better define the epidemiology of COVID-19 (3). Anti-SARS-CoV-2 IgM antibodies are produced in the early phase of the immune response and are indicators of current or recent infection (4). In contrast, the IgG antibodies emerge later after IgM antibodies in a primary infection or vaccination and persist in the body for months or years. Therefore, the anti-SASR-CoV-2 IgG and IgM antibodies can provide important insights necessary for understanding immunity and progression of COVID-19.
COVID-19 in Kenya has shown a dual epidemiological pattern, with cities like Nairobi recording higher seroprevalence of anti-SARS-CoV-2 antibodies compared to rural areas (5). In the study conducted by Etyang et al. from December 2020 to May 2021 in Kenya, the seroprevalence of anti-SARS-CoV-2 IgG was 36%, 32.4%, and 14.5% in Kisumu (sub-urban), Nairobi (urban), and Kilifi (rural), respectively, at the beginning of the study (6). The seroprevalence rose to 42%, 50.2%, and 24.7% in the three areas at the end of the study. By the end of 2022, the seroprevalence of anti-SARS-CoV-2 IgG had risen to 92.2% in Nairobi and 77.4% in Kilifi (7). The rise in seroprevalence could be due to natural infection by SARS-CoV-2 or COVID-19 vaccination, which was actively rolled out from March 2021 to the end of 2022. On May 5, 2023, the World Health Organization (WHO) downgraded COVID-19 from a public health emergency of international concern, but maintained that a public health risk remained, especially because of the possible emergence of new variants (8).
Vaccination has been instrumental to the control of the COVID-19 pandemic worldwide. In Kenya, as of May 2022, more than 32 million individuals had received at least one dose of COVID-19 vaccine, and as of August 2022, 9.3 million adults had been fully vaccinated. Following the approval of COVID-19 vaccines, 36.5% of the population in Kenya expressed reluctance toward getting vaccinated against COVID-19 (9). This hesitancy may impede efforts to achieve herd immunity and control the spread of the virus. Additionally, the effectiveness of the vaccination drives in Kenya has been further complicated by the emergence of multiple SARS-CoV-2 variants of concern (10). This raises questions about the ability of certain populations to maintain protective levels of anti-SARS-CoV-2 antibodies and the efficacy of the rolled-out COVID-19 vaccines.
Studies on the epidemiological patterns of COVID-19 in Kenya were mainly conducted during the early days of the COVID-19 pandemic and vaccine roll out, and the seroprevalence of anti-SARS-CoV-2 antibodies post-vaccination remains unclear. With COVID-19 now establishing endemicity with seasonal outbreaks, determining the level of antibody protection is an important public health priority. Therefore, this study aimed to determine the seroprevalence of anti-SARS-CoV-2 IgM and IgG and evaluate the COVID-19 vaccination uptake in a university community in Nairobi, Kenya.
We conducted a cross-sectional, population-based study to assess the seroprevalence of anti-SARS-CoV-2 IgM and IgG antibodies among healthy volunteers from the Kenyatta University community. The study was carried out from April to July 2023. Ethical approval was obtained from the Kenyatta University Ethics Review Committee (protocol code: PKU/2379/11516; February 28, 2022). Further approval was granted by the National Commission for Science, Technology, and Innovation. All volunteers provided written informed consent to participate in this study.
A call for participation was made via posters, staff meetings, and after-class announcements. Volunteers were considered eligible if they were 1) students or staff at Kenyatta University, 2) aged ≥18 years, 3) consented to provide nasopharyngeal swab and blood specimen, and 4) consented to complete the structured questionnaire.
The study utilized a structured questionnaire to gather clinical and demographic data. The demographic data included age, sex, level of education, and occupation. Clinical data encompassed history of COVID-19 diagnosis, COVID-19 vaccination status, type of vaccine administered, administration of booster vaccine, and occurrences of breakthrough infection. In this study, a breakthrough infection was defined as positive COVID-19 diagnosis despite vaccination.
Nasopharyngeal swabs were collected from each participant and labeled with a unique identifier. The nasopharyngeal swabs underwent on-site testing for SARS-CoV-2 using a rapid COVID-19 test kit (Panbio™ COVID-19 Ag Rapid Test Device, Abbott, Jena, Germany).
Blood samples (5 ml) were collected from each participant and labeled with a unique identifier. The blood samples were centrifugated at 1000 x g for 5 min to extract serum and immediately refrigerated at −20°C until testing. As described by the manufacturer, the levels of anti-SARS-CoV-2 IgM and IgG in the serum were quantified using human SARS-CoV-2 Spike (Trimer) IgM and IgG enzyme-linked immunosorbent assays (ELISA), respectively (Thermo Fisher Invitrogen, Waltham, MA, USA). The assays were based on sandwich ELISA in which wells were precoated with a trimerized spike protein. For the respective assays, the serum was diluted 1000-fold and 10 µL of the diluted sample added to the wells. Biotin-conjugated IgM and IgG antibodies were used to detect the captured anti-SARS-CoV-2 IgM and IgG antibodies, and the absorbances were read at a wavelength of 450 nm using a spectrophotometer (RT-2100C, Rayto Life and Analytical Sciences, Guangdong, China).
The absorbance readings of the standards, controls, and test samples were obtained from the spectrophotometer. Qualitative results were presented by calculating the ratio of the test optical density (OD) to the OD of the plate medium control. Samples with OD ratios >1.3 were classified as positive for SARS-CoV-2 IgG and IgM, while those with ratios <1 were considered negative. For quantitative analysis, absorbance data were used to construct a standard curve via the Excel Curve Fitting algorithm, leveraging a four-parameter algorithm for optimal curve fitting. Unknown sample concentrations were extrapolated from the standard curve.
The obtained concentrations of anti-SARS-CoV-2 IgG and IgM underwent the Kolmogorov–Smirnov test and were found to have a non-normal distribution. As such, these data are summarized using median and interquartile range. Categorical data are expressed as counts and percentages. Descriptive statistics were used to analyze the characteristics of the participants, including age, sex, and COVID-19 vaccination status. Statistical comparisons were conducted using the Mann–Whitney test (U) for binary comparisons and the Kruskal–Wallis Test (H) for multigroup comparisons. The quantitative analyses were conducted using SPSS (version 18) software (IBM Corp, Armonk, NY), with significance set at p < 0.05.
Qualitative data on vaccine hesitancy were grouped into three categories according to the 3C model of vaccine hesitancy by the WHO’s Strategic Advisory Group of Experts on Immunization (SAGE) working group (11). The 3Cs include confidence, complacency, and convenience. Then, a thematic analysis of participants’ responses was performed based on the three categories.
A total of 189 participants (female, 50.8%; median age, 21 years) were recruited to participate in this study. Participants aged 20–29 years were the majority (n = 135, 71.4%), whereas those above 50 years were the minority (n = 5, 2.6%). The overall rate of vaccination was 56.6% (n = 107), with females (n = 61, 63.5%) having a higher vaccination rate than males (n = 46, 49.5%). All the participants had college/university education. The majority of the study participants were students (n = 170, 89.9%) while the rest were teaching (n = 10, 5.3%) and non-teaching staff (n = 9, 4.8%) (Table 1).
A total of 40 participants (21.2%) self-reported that they were positive for COVID-19 in the past based on laboratory testing or clinical diagnosis. Among these, males were 22 (55%) and females were 18 (45%) (Figure 1). A test for anti-SARS-CoV-2 antigen positivity during the study, which was performed in only 65 consenting participants, revealed a positivity rate of 4.6% (n = 3) (Figure 2). The study reported breakthrough infections in 9.3% (n = 10) of the vaccinated individuals.
Of the 189 participants, 24 were positive for anti-SARS-CoV-2 IgM (12.7%), whereas 166 were positive for anti-SARS-CoV-2 IgG (87.8%) (Table 2). Female participants had a slightly higher seroprevalence (IgM, 15.6%; IgG, 92.7%) compared to males (IgM, 9.7%; IgG, 83.8%). The anti-SARS-CoV-2 IgG levels were significantly higher in females than in males (p = 0.024, U = 3616) and in vaccinated individuals than in the non-vaccinated individuals (p < 0.001, U = 2817.5). No significant age differences in the anti-SARS-CoV-2 IgG levels were observed (Table 3).
A comparison of anti-SARS-CoV-2 IgG levels and duration since vaccination showed that participants vaccinated 6 months or less prior to the study had significantly higher anti-SARS-CoV-2 IgG levels than those vaccinated more than 1 year earlier (p = 0.002, H = 12.359) (Figure 3).
The present study found no significant differences in the levels of anti-SARS-CoV-2 IgG among the participants who received vaccines from different manufacturers (Moderna, AstraZeneca, Pfizer, and Johnson & Johnson, p = 0.170, H = 5.027) (Table 4). Similarly, no significant differences in anti-SARS CoV-2 IgG levels were observed between those who had received a booster dose compared to those who had not (p = 0.758, U = 1087).
A total of 82 (43.4%) participants were not vaccinated against COVID-19. Of these, 10 did not indicate the reasons for non-vaccination. The rate of vaccine hesitancy was higher in males than in females (50.5% vs. 36.5%, p = 0.05). An analysis for the reasons for non-vaccination was conducted on the remaining 72 participants. Under the confidence category, the identified themes, which comprise the reasons for non-vaccination, were mistrust in the vaccine effectiveness and production process (22.4%), health concerns/side effects (19.7%), lack of information about the vaccines (18.4%), and religious and cultural reasons (3.9%). The complacency category comprised no reason (14.5%), lack of interest (11.8%), and procrastination (2.6%). In addition, the convenience category included vaccine unavailability (2.6%) and lack of time (3.9%) (Table 5).
COVID-19 morbidity and mortality remain significant public health concerns, necessitating robust serosurveillance to elucidate disease epidemiology and evaluate the efficacy of control strategies. This study found an overall seroprevalence of 12.7% for anti-SARS-CoV-2 IgM and 87.8% for anti-SARS-CoV-2 IgG. We observed significantly higher levels of anti-SARS-CoV-2 IgG in females than in males (p = 0.024), among the vaccinated than among the non-vaccinated individuals (p < 0.001), and among those vaccinated 6 months or less prior to the study compared to those vaccinated more than 1 year earlier (p = 0.002). However, no significant differences in anti-SARS-CoV-2 IgG levels were observed across the various brands of vaccines and after the administration of booster doses. The present study showed a vaccine hesitancy rate of 43.4%, and the primary reasons for non-vaccination were mistrust in the vaccine effectiveness and production process, health concerns/side effects, and lack of information about the vaccines.
The reduced burden of COVID-19 in the community was evident in the present study, as indicated by the low seroprevalence of anti-SARS-CoV-2 IgM (12.7%), an indication of current or recent infection. Also, among the 65 participants tested for COVID-19, only three cases were positive (4.6%). The low rate of current SARS-CoV-2 infection was also reported in Central African Republic, in which only 3.6% of the surveyed population tested positive for anti-SARS-CoV-2 IgM in May 2022 (12). Similarly, in Cameroon, the seroprevalence of antiSARS-CoV-2 IgM in September 2022 was 6.8% (13). Therefore, the results of our study and those of similar surveys corroborate the decision by the WHO to downgrade COVID-19 from a public health emergency to an ongoing health issue.
The high seroprevalence of anti-SARS-CoV-2 IgG in the present study (87.8%) is consistent with that reported by Kagucia et al. among randomly selected, age-stratified population samples from the Health and Demographic Surveillance System in Nairobi (92.2%) (7). Similarly, a hospital-based study in a rural region in Kenya conducted from January 2022 to December 2022 reported a seroprevalence of anti-SARS-CoV-2 IgG of 97.8% (14). These findings allude to widespread exposure to SARS-CoV-2 through natural infection or vaccination.
Among the factors affecting the seroprevalence of anti-SARS-CoV-2 IgG, sex and vaccination status were significantly associated with the titers of anti-SARS-CoV-2 IgG. Females had significantly higher titers of anti-SARS-CoV-2 IgG than males, likely because of the higher rate of vaccination, which was approaching statistical significance. Vaccination is an effective strategy of strengthening the immunity against COVID-19. In the present study, 56.6% of the participants (female, 63.5%; male, 49.5%) were vaccinated against COVID-19 and had higher anti-SARS-CoV-2 IgG titers compared to the non-vaccinated individuals. Similarly, an increase in anti-SARS-CoV-2 IgG titers among the vaccinated individuals has been reported in previous studies (15, 16). However, the occurrence of breakthrough infections has been acknowledged, as the vaccines do not necessarily prevent infection, but are mainly beneficial for protection against severe disease. In addition, the vaccines may not offer protection against new variants of SARS-CoV-2.
In addition, the duration since vaccination significantly affected the anti-SARS-CoV-2 IgG titers. The individuals vaccinated 6 months or less prior to the study had significantly higher levels of anti-SARS-CoV-2 IgG than those who were vaccinated more than 1 year before the study. Consistent with previous studies, the anti-SARS-CoV-2 IgG titers peak within 6 months after vaccination, followed by a rapid decline (17, 18). The administration of first, second, or third booster doses is reported to prolong the high titers of anti-SARS-CoV-2 IgG. Matsumoto et al. found a longitudinal increase in anti-SARS-CoV-2 levels with administration of each booster dose (19). However, in the present study, no difference in anti-SARS-CoV-2 IgG titers was found between individuals who received a booster dose and those who did not. The necessity for booster doses in augmenting vaccine efficacy and strengthening immune response has been demonstrated by reduced rates of COVID-19-related hospitalization and mortality risk in previous studies (20, 21). The lack of a significant difference in antibody titers according to the administration of booster vaccines in the present study might be due to the low number of booster dose recipients (29 out of 107), which precludes definitive conclusions regarding the association between booster administration and enhanced antibody response.
The present study also found no significant difference in anti-SARS-CoV-2 IgG titers according to the type of vaccine. This finding contrasts that of Karl et al. who found higher levels of anti-SARS-CoV-2 in individuals who received two doses of the Comirnaty® vaccine and one dose of the Spikevax® vaccine compared to those who received three doses of the Spikevax® vaccine (15). Another study found significantly higher titers of anti-SARS-CoV-2 IgG in those who received three doses of the Pfizer/BioNTech® vaccine compared to those who received two doses of the AstraZeneca® vaccine and a third dose of the Pfizer/BioNTech® vaccine (22).
A relatively high vaccine hesitancy was observed in the present study. Despite the 87.8% seroprevalence of anti-SARS-CoV-2 IgG, 43.4% of the participants did not receive the COVID-19 vaccine, which is higher than that reported in an earlier study in Kenya, in which 19% of the participants were reluctant to get vaccinated (23). Our study comprised mainly young people, and the high vaccine hesitancy in young adults has been reported previously. Rajshekhar et al. reported that 59.5% of young adults in informal settlements in Kenya showed COVID-19 vaccine hesitancy (24). In the United States, 37.2% of young adults aged 18–24 years were not vaccinated against COVID-19 (25). These findings show a high rate of vaccine hesitancy in the youthful and well-educated population of mostly university students in the case of the current study, which is suggested to be due to the distrust of the government and pharmaceutical companies and fear of adverse health effects of the vaccines (26). Similarly, in the present study, most non-vaccinated individuals cited mistrust in the vaccine effectiveness and production process, health concerns/side effects, and lack of information about the vaccines. According to the 3Cs model by SAGE, these factors relate to a lack of confidence in the vaccine (11). The quick development of the COVID-19 vaccines for emergency use may have heightened suspicion and eroded the confidence that people had in the pharmaceutical industry, given that vaccines usually undergo many years of basic research and clinical trials for safety and efficacy before regulatory approval. In addition, the lack of adequate knowledge about the vaccine was also a major barrier to enhanced vaccination. For instance, some participants thought that the COVID-19 vaccine was meant for only those with a previous infection. Given the public health need at the height of the pandemic, less effort was made to engage the public on the new vaccines, compounding the effect of widespread misinformation and conspiracy theories. Knowledge enables people to make informed decisions about the need for vaccination and helps address unfounded fears about vaccines (27).
Further, complacency was an important driver of vaccine hesitancy in the present study, as indicated by participants who were not interested in vaccination and those who reported no reason for non-vaccination. This complacent attitude is reported to arise from low perceived risk of COVID-19 among young adults, who mainly had mild disease (24). The other factor is convenience, which was a concern for only a few participants, suggesting that vaccine availability was not a major problem. As emphasized by the WHO, these factors need to be considered when formulating vaccination programs in the population.
This study has a few limitations. First, the study comprised a limited demographic, of mainly young adults aged 18–29 years, and hence comparison of anti-SARS-CoV-2 seroprevalence across different age groups could not be made. The study findings may also not be generalized to other age groups. Nevertheless, the inclusion of a homogenously young and educated population has helped characterize the seroprevalence of this population and understand the reasons for vaccination hesitancy specific to this group that may also apply to the general population, In future, a study including a diverse and representative age range may provide richer community-wide data on the seroprevalence of anti-SARS-CoV-2 antibodies and vaccination trends. Second, the majority of participants were not tested for COVID-19 during the study, and hence the prevalence of COVID-19 based on self-reporting is inaccurate. Nonetheless, the assay for IgM helped identify possible cases of ongoing infection. For past infection, reporting by the study cohort, made up of majorly young participants who are likely to recall their Covid-19 diagnosis history, and given the anxiety and public awareness associated with Covid-19 diagnosis then, is most likely to be accurate.
The current study revealed widespread exposure to SARS-CoV-2 through vaccination or natural infection, as indicated by the high seroprevalence of anti-SARS-CoV-2 IgG. IgG antibody responses were more robust in vaccinated compared to non-vaccinated participants, underscoring the importance of vaccination in controlling the spread of COVID-19. The high vaccine hesitancy rate highlights the need for intensified community engagement and educational efforts to bolster vaccine uptake rates within Kenyan communities. These findings underscore the importance of understanding the interplay between vaccination status, immune response, and seroprevalence rates in shaping effective public health strategies post-COVID-19 pandemic.
The raw data supporting the conclusions of this article will be made available by the authors, without undue reservation.
The studies involving humans were approved by Ethics Review Committee, Kenyatta University. The studies were conducted in accordance with the local legislation and institutional requirements. The participants provided their written informed consent to participate in this study.
AO: Data curation, Formal analysis, Investigation, Methodology, Visualization, Writing – original draft, Writing – review & editing. EN: Conceptualization, Data curation, Formal analysis, Funding acquisition, Investigation, Methodology, Project administration, Resources, Supervision, Validation, Visualization, Writing – original draft, Writing – review & editing. MT: Investigation, Methodology, Validation, Writing – review & editing. MM: Supervision, Writing – review & editing. PT: Conceptualization, Funding acquisition, Investigation, Methodology, Writing – review & editing. JO: Conceptualization, Funding acquisition, Investigation, Methodology, Writing – review & editing. LO: Conceptualization, Funding acquisition, Writing – review & editing. JM: Funding acquisition, Writing – review & editing. VO: Funding acquisition, Writing – review & editing. SG: Conceptualization, Funding acquisition, Writing – review & editing. GM: Conceptualization, Funding acquisition, Project administration, Resources, Writing – review & editing. PO: Conceptualization, Funding acquisition, Project administration, Resources, Writing – review & editing.
The author(s) declare that financial support was received for the research, authorship, and/or publication of this article. This work was supported by the National Research Foundation (NRF), South Africa, under grant COV19200617532972.
We express our gratitude to the research sponsors and the study team for their assistance in administrative and technical matters. We acknowledge the support provided by the Kenyatta University staff throughout the study procedures. Also, we are thankful to the study participants for actively taking part in the research.
The authors declare that the research was conducted in the absence of any commercial or financial relationships that could be construed as a potential conflict of interest.
All claims expressed in this article are solely those of the authors and do not necessarily represent those of their affiliated organizations, or those of the publisher, the editors and the reviewers. Any product that may be evaluated in this article, or claim that may be made by its manufacturer, is not guaranteed or endorsed by the publisher.
1. Worldometer. Kenya COVID – coronavirus statistics – Worldometer (2024). Available online at: https://www.worldometers.info/coronavirus/country/Kenya/. (Accessed July 5, 2024).
2. Bwire G, Ario AR, Eyu P, Ocom F, Wamala JF, Kusi KA, et al. The COVID-19 pandemic in the African continent. BMC Med. (2022) 20:167. doi: 10.1186/s12916-022-02367-4
3. Mohammad Hossein ZJ, Mehran D. Seroprevalence of anti-SARS-coV-2 igG and igM antibodies among government employees in Iran. Arch Razi Inst. (2023) 78:1413–20. doi: 10.32592/ARI.2023.78.5.1413
4. Li Q, Chen L, Li F, He A. Long-term evaluation of the seroprevalence of SARS-CoV-2 IgG and IgM antibodies in recovered patients: a meta-analysis. BMC Infect Dis. (2023) 23:444. doi: 10.1186/s12879-023-08425-3
5. Adetifa IMO, Uyoga S, Gitonga JN, Mugo D, Otiende M, Nyagwange J, et al. Temporal trends of SARS-CoV-2 seroprevalence during the first wave of the COVID-19 epidemic in Kenya. Nat Commun. (2021) 12:3966. doi: 10.1038/s41467-021-24062-3
6. Etyang AO, Adetifa I, Omore R, Misore T, Ziraba AK, Ng’oda MA, et al. SARS-CoV-2 seroprevalence in three Kenyan health and demographic surveillance sites, December 2020-May 2021. PloS Glob Public Health. (2022) 2:e0000883. doi: 10.1371/journal.pgph.0000883
7. Kagucia EW, Ziraba AK, Nyagwange J, Kutima B, Kimani M, Akech D, et al. SARS-CoV-2 seroprevalence and implications for population immunity: Evidence from two Health and Demographic Surveillance System sites in Kenya, February-December 2022. Influenza Other Respir Viruses. (2023) 17:e13173. doi: 10.1111/irv.13173
8. World Health Organization. Statement on the fifteenth meeting of the IHR (2005) Emergency Committee on the COVID-19 pandemic (2023). Available online at: https://www.who.int/news/item/05-05-2023-statement-on-the-fifteenth-meeting-of-the-international-health-regulations-(2005)-emergency-committee-regarding-the-coronavirus-disease-(covid-19)-pandemic. (Accessed July 5, 2024).
9. Orangi S, Mbuthia D, Chondo E, Ngunu C, Kabia E, Ojal J, et al. A qualitative inquiry on drivers of COVID-19 vaccine hesitancy among adults in Kenya. PloS Glob Public Health. (2024) 4:e0002986. doi: 10.1371/journal.pgph.0002986
10. Khamadi SA, Opanda S, Symekher SL, Limbaso SK, Langat S, Cirindi JK, et al. Whole-genome sequence analysis reveals the circulation of multiple SARS-CoV-2 variants of concern in Nairobi and neighboring counties, Kenya between March and July 2021. Virol J. (2022) 19:178. doi: 10.1186/s12985-022-01895-y
11. Gerretsen P, Kim J, Caravaggio F, Quilty L, Sanches M, Wells S, et al. Individual determinants of COVID-19 vaccine hesitancy. PloS One. (2021) 16:e0258462. doi: 10.1371/journal.pone.0258462
12. Manirakiza A, Malaka C, Mossoro-Kpinde HD, Yambiyo BM, Mossoro-Kpinde CD, Fandema E, et al. Seroprevalence of anti-SARS-CoV-2 antibodies before and after implementation of anti-COVID-19 vaccination among hospital staff in Bangui, Central African Republic. PloS Glob Public Health. (2023) 3:e0001497. doi: 10.1371/journal.pgph.0001497
13. Sorel BKD, Didiane YM, Samuel MM, Pierre FCS, Caroline DTN, Richard N, et al. Seroprevalence of SARS-CoV-2 antibodies among the university population of Ngaoundere-Cameroon. J Clin Images Med Case Rep. (2023) 4:2758. doi: 10.52768/2766-7820/2758
14. Ruttoh VK, Kipkoech B, Tonui R, et al. Seroprevalence of Anti-SARS-Cov-2 Antibodies among Patients Visiting Hospital-Based Sentinel Sites in the Rift Valley Region, Kenya (2024). Available online at: https://www.preprints.org/manuscript/202403.0923/v1. (Accessed July 5, 2024).
15. Karl T, Schuster A, Stangassinger LM, Stiboller T, Cadamuro J, Oostingh GJ. Factors Affecting SARS-CoV-2 IgG Production after Vaccination and/or Disease: A Large-Scale Seroprevalence Study. Vaccines. (2023) 11:1615. doi: 10.3390/vaccines11101615
16. Bullock JL, Hickey TE, Kemp TJ, Metz J, Loftus S, Haynesworth K, et al. Longitudinal assessment of BNT162b2- and mRNA-1273-induced anti-SARS-coV-2 spike igG levels and avidity following three doses of vaccination. Vaccines. (2024) 12:516. doi: 10.3390/vaccines12050516
17. Pegu A, O’Connell SE, Schmidt SD, O’Dell S, Talana CA, Lai L, et al. Durability of mRNA-1273 vaccine-induced antibodies against SARS-CoV-2 variants. Science. (2021) 373:1372–7. doi: 10.1126/science.abj4176
18. Collier A-RY, Yu J, McMahan K, Liu J, Chandrashekar A, Maron JS, et al. Differential kinetics of immune responses elicited by covid-19 vaccines. N Engl J Med. (2021) 385:2010–2. doi: 10.1056/NEJMc2115596
19. Matsumoto N, Sasaki A, Kadowaki T, Mitsuhashi T, Takao S, Yorifuji T. Longitudinal antibody dynamics after COVID-19 vaccine boosters based on prior infection status and booster doses. Sci Rep. (2024) 14:4564. doi: 10.1038/s41598-024-55245-9
20. Kislaya I, MaChado A, Magalhães S, Rodrigues AP, Franco R, Leite PP, et al. COVID-19 mRNA vaccine effectiveness (second and first booster dose) against hospitalisation and death during Omicron BA.5 circulation: cohort study based on electronic health records, Portugal, May to July 2022. Eurosurveillance. (2022) 27:2200697. doi: 10.2807/1560-7917.ES.2022.27.37.2200697
21. Mattiuzzi C, Lippi G. Efficacy of the second COVID-19 vaccine booster dose in the elderly. Vaccines. (2023) 11:213. doi: 10.3390/vaccines11020213
22. Biedrzycka BW, Bieńkowska A, Smolińska-Fijołek E, Biedrzycki G, Dorf J. The influence of two priming doses of different anti-COVID-19 vaccines on the production of anti-SARS-coV-2 antibodies after the administration of the pfizer/bioNTech booster. Infect Drug Resist. (2022) 15:7811–21. doi: 10.2147/IDR.S390351
23. Osur J, Muinga E, Carter J, Kuria S, Hussein S, Ireri EM. COVID-19 vaccine hesitancy: Vaccination intention and attitudes of community health volunteers in Kenya. PloS Glob Public Health. (2022) 2. https://www.ncbi.nlm.nih.gov/pmc/articles/PMC10021929/.
24. Rajshekhar N, Pinchoff J, Boyer CB, Barasa E, Abuya T, Muluve E, et al. Exploring COVID-19 vaccine hesitancy and uptake in Nairobi’s urban informal settlements: an unsupervised machine learning analysis of a longitudinal prospective cohort study from 2021 to 2022. BMJ Open. (2023) 13:e071032. doi: 10.1136/bmjopen-2022-071032
25. CDC. Archive: COVID-19 vaccination and case trends by age group, United States | Data | Centers for disease control and prevention (2022). Available online at: https://data.cdc.gov/Vaccinations/Archive-COVID-19-Vaccination-and-Case-Trends-by-Ag/gxj9-t96f/about_data. (Accessed July 5, 2024).
26. Kim S, Willis E, Wehlage S, Scheffer-Wentz H, Dulitz M. COVID-19 vaccine hesitancy and short-term and long-term intentions among unvaccinated young adults: a mixed-method approach. BMC Public Health. (2022) 22:2030. doi: 10.1186/s12889-022-14448-3
Keywords: COVID-19, hesitancy, IgG, IgM, mistrust, SARS-CoV-2, seroprevalence, vaccination
Citation: Otindo AM, Ndombi EM, Theuri M, Muturi M, Thamaini P, Ogutu J, Onsongo L, Madete JK, Ofula V, Gitau S, Mwangi G and Okemo P (2024) Seroprevalence of anti-SARS-CoV-2 IgM and IgG and COVID-19 vaccine uptake in healthy volunteers in Nairobi, Kenya: a cross-sectional study. Front. Virol. 4:1479645. doi: 10.3389/fviro.2024.1479645
Received: 12 August 2024; Accepted: 15 October 2024;
Published: 08 November 2024.
Edited by:
Stefania Varchetta, IRCCS Ca ‘Granda Foundation Maggiore Policlinico Hospital, ItalyReviewed by:
Tiansheng Li, National Institutes of Health (NIH), United StatesCopyright © 2024 Otindo, Ndombi, Theuri, Muturi, Thamaini, Ogutu, Onsongo, Madete, Ofula, Gitau, Mwangi and Okemo. This is an open-access article distributed under the terms of the Creative Commons Attribution License (CC BY). The use, distribution or reproduction in other forums is permitted, provided the original author(s) and the copyright owner(s) are credited and that the original publication in this journal is cited, in accordance with accepted academic practice. No use, distribution or reproduction is permitted which does not comply with these terms.
*Correspondence: Eric M. Ndombi, ZW1ha3V0b0BnbWFpbC5jb20=; ZXJpYy5uZG9tYmlAa3UuYWMua2U=
Disclaimer: All claims expressed in this article are solely those of the authors and do not necessarily represent those of their affiliated organizations, or those of the publisher, the editors and the reviewers. Any product that may be evaluated in this article or claim that may be made by its manufacturer is not guaranteed or endorsed by the publisher.
Research integrity at Frontiers
Learn more about the work of our research integrity team to safeguard the quality of each article we publish.