- 1Department of Biology and Environmental Science, University of New Haven, West Haven, CT, United States
- 2Saha Cardiovascular Research Center, University of Kentucky College of Medicine, Lexington, KY, United States
Heart disease is the leading cause of death worldwide. Myocarditis, or inflammation of the cardiac muscle, is estimated to cause up to 1.5 million cases annually, with viral infection being the most common disease culprit. Past studies have shown that Parvovirus B19 is routinely detected in endomyocardial biopsies. This virus has been linked to acute heart inflammation, which can cause cardiac muscle damage. However, because Parvovirus B19 can be found in the heart tissues in the absence of disease symptoms, it is unclear if the long-term presence of the virus contributes to, or initiates, heart disease. Here, we utilized a PCR-based detection assay to assess the presence of the B19V genome and its mRNA intermediates in human heart tissues. The analysis was carried out in three heart layers derived from one individual: epicardium, endocardium and myocardium. We showed the Parvovirus B19 genome presence variability in different heart layers. Similarly, viral transcriptional activity, assessed by the mRNA presence, was detected only in a few of the analyzed samples. Our results suggest that localized sites of Parvovirus B19 infection may exist within individual heart layers, which may have implication for the cardiac muscle inflammation.
Introduction
Parvovirus B19 (B19V) is a known cause of Erythema infectiosum, or fifth disease, in humans. This small, non-enveloped virus of approximately 5.6 kb in length belongs to the Parvoviridae family. The viral genome consists of a coding region, flanked by identical terminal repeats (ITRs) on both sides (1). The coding region is translated into a nonstructural protein (NS1), 2 capsid proteins (VP1 and VP2), and also two nonstructural proteins (7.5kDa and 11kDa) produced during B19V infection (2). More than 50% of adolescents have antiparvovirus B19 antibodies, which makes the virus a common childhood infection (3). Adults can also come down with fifth disease, and it is estimated that most people are seropositive by the time they reach 50 years of age. B19V causes a variety of symptoms, such as rash that first appears on a face, and later spreads to the trunk and extremities, fever, headache, nausea or joint pain (4). The majority of immunocompetent individuals recover from the disease on their own. Nonetheless, people with a weakened immune system may experience anemia, and some preexisting conditions, such as sickle-cell anemia, may cause individuals infected with B19V to develop a transient aplastic crisis, or pure red blood cell aplasia (5, 6). Exposure to fifth disease during pregnancy can lead to a transmission of the virus to the fetus, which may result in hydrops fetalis and pregnancy complications (3). Interestingly, the virus is commonly found in the human heart while performing endomyocardial biopsies, suggesting that it can persist in the cardiac tissue after the initial infection (7). The B19V genome has been previously detected in patients suffering from heart disease, as well as in healthy individuals (8–10), although the role the virus may play in the development or the progression of heart disease is not well understood.
The heart is composed of three layers: epicardium (outer), myocardium (middle) and endocardium (inner), each with different cellular composition. Epicardium, which has a protective function, contains mesothelial cells (11), whereas myocardium is composed of cardiomyocytes responsible for the cardiac contractile force (12). Endothelial cells and connective tissues are characteristic of endocardium, which protects the inner heart tissue (13). It is thought that non-cardiomyocytes, such as fibroblasts, endothelial cells and smooth muscle cells also play a role in the heart’s physiological function and the response to injury (12, 14, 15). B19V does not infect cardiomyocytes, but it has been previously shown to be able to infect the vascular endothelial cells due to the presence of P-antigen/α5β1-integrin on these cells (16). Typically, the virus is unable to complete its full replication cycle in these cells. Interestingly, replication of B19V in the endothelial cells have been reported in patients suffering from acute viral myocarditis (7).
The first case of B19V-induced heart disease was described in 1993, but it took another decade of research to suggest that B19V may be a causative agent of cardiac inflammation (17, 18). Numerous studies have reported B19V as a cause of acute and fatal myocarditis (19–21), and shed light on cardiac dysfunction associated with both acute and chronic infection with this virus. In situ hybridization experiments detected the presence of the B19V genome in the endothelial cells of myocardium, and in small arteries and arterioles in individuals suffering from acute inflammatory cardiomyopathy (8, 17, 22). It was suggested that the subsequent damage to the heart could be a result of cytopathic effect or immune system activation and inflammation (16, 23). Past studies have reported myocyte damage after inflammatory cell recruitment (24), as manifested by the presence of inflammatory infiltrates in the heart muscle fibers (25), or myocyte vacuolization (26). The infection of cardiac cells by B19V can cause diminished coronary microcirculation and cardiac myocyte necrosis, which are hallmarks of cardiac pathology (8, 24). Studies also reported that chronic cardiac inflammation can be associated with the presence of B19V in the capillary system of the myocardium (22). In both acute and chronic infection of B19V-induced cardiac pathogenesis, high levels of cytokines: interferon γ, tumor necrosis factor α, as well as interleukins 6 and 8 are thought to mediate cardiac inflammation and necrosis (27). B19V is also the most frequently detected viral genome in patients suffering from dilated cardiomyopathy (DCM) (28). On the other hand, the B19V genome has been found in the hearts of healthy individuals (8, 29). For example, studies performed by Dennert et al. determined that 100 out of 125 patients with DCM, and 15 out of 20 healthy individuals had detectable levels of B19V in their heart tissues (9). Currently, it is not understood if the long-term B19V presence in the human heart tissue may be associated with negative cardiovascular outcomes, or if it could exacerbate already existing heart issues.
In this study, we analyzed human heart tissue samples obtained from patients suffering from heart disease (heart transplants or ventricular assist device – VAD implants), as well as cardiac tissues derived from individuals who had no reported cardiovascular issues at the time of death (controls). We assessed the presence of B19V DNA in three layers of the heart: epicardium, endocardium and myocardium, derived from the hearts of individual patients. Furthermore, we differentiated the latent and active B19V infection in the analyzed samples by detecting the VP1 mRNA intermediates.
Materials and methods
Heart tissue sample processing
The human heart tissue samples were obtained from the Gill Heart & Vascular Institute, KY. The tissues were derived from heart transplants or VAD implants, as previously described (30). The heart tissues were also obtained from tissue donors, who had no reported heart disease at the time of death (Figure 1). 50 mg +/- 2 mg of epicardium, endocardium, or myocardium, obtained from each individual was dissected using a sterile scalpel before a nucleic acid extraction procedure. PRO Scientific Bio-Gen homogenizer was used to dissociate the heart tissue samples, and DNA and RNA were extracted using a Quick-DNA/RNA Miniprep Plus Kit (Zymo Research, D7003). RNA samples were treated with DNAse I at the time of the procedure to eliminate DNA contamination according to manufacturer’s instructions (31). The absence of residual DNA was independently verified in a PCR reaction to ensure no contamination in the RNA samples. The quantity of DNA and RNA was assessed using NanoDrop One spectrophotometry (Thermo Fisher Scientific), and the material quality was evaluated using 1% agarose gel electrophoresis. Following the extraction procedure, all samples were stored at -80°C.
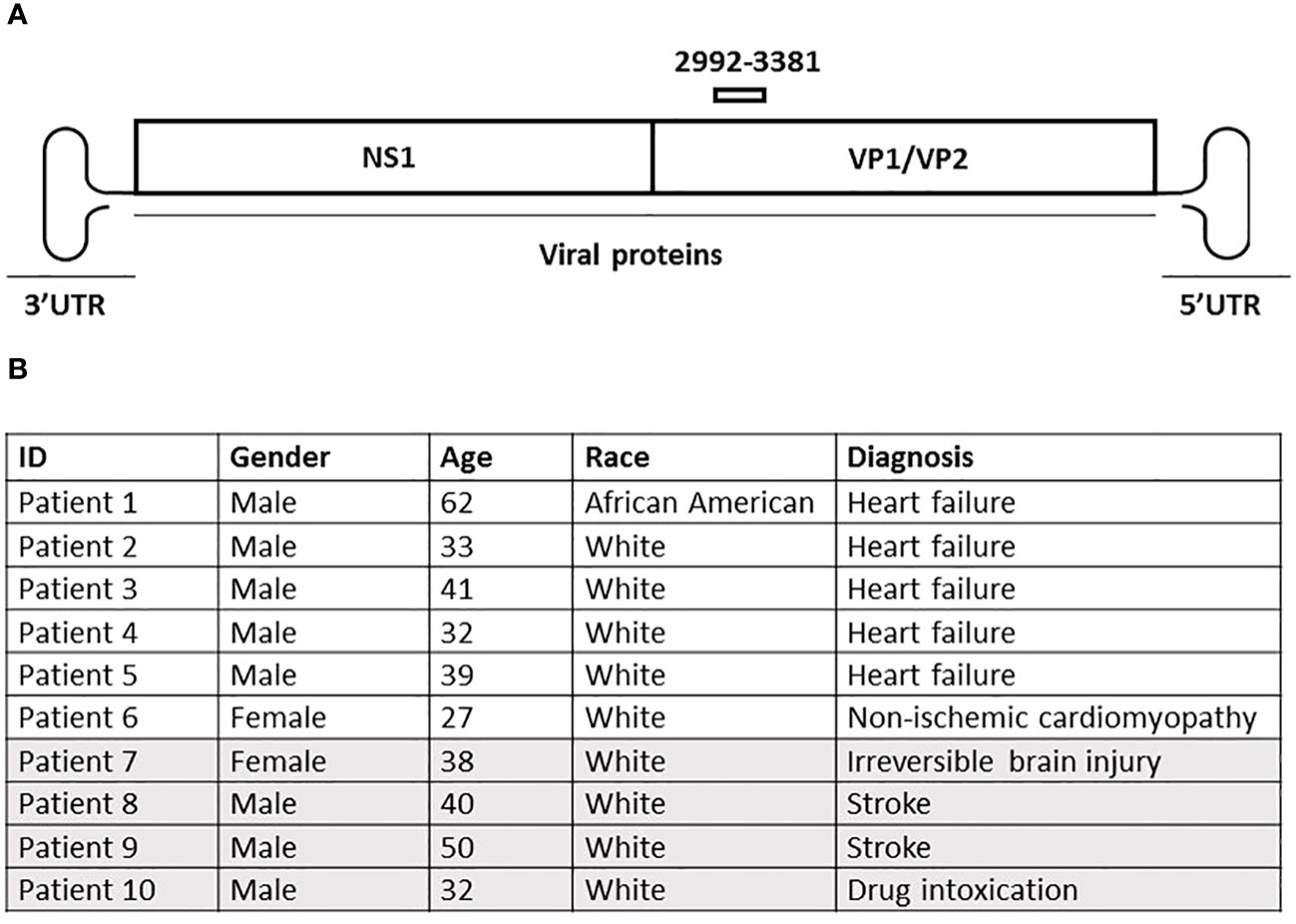
Figure 1 (A) Schematic representation of the human parvovirus B19 (B19V) genome. Viral proteins are shown as boxes, with the 3′ and 5′ UTRS on each end. The primer pair used to assess the presence of the viral DNA is marked above the viral protein region. (B) Clinical data of sampled patients. Patients 1-6 (white) had a history of cardiovascular disease at the time of death or heart transplant, whereas patients 7-10 (grey) had no reported heart disease at the time of death. Epicardium, endocardium and myocardium were collected from each individual patient.
Identification of B19V genome in the human heart tissue samples
PCR analysis was used to identify the VP1 region of B19V in the three layers of the heart. 75 ng of the total DNA from each individual heart layer sample along with primers specific to VP1 (F 5’ ACAACTACCCGGTACTAACTATGT 3’ and R 5’ CTCCACATGCTTTTGACAGGATTA 3’) was used for PCR amplification using the Phusion High Fidelity DNA Polymerase kit (Thermo Fisher Scientific). The conditions of the PCR reaction were as follows: 98°C for 30 sec, followed by 38 cycles of 98°C for 10 sec, 60°C for 20 sec, 72°C for 20 sec, with final extension at 72°C for 10 min. 1% agarose gel electrophoresis was used to visualize the PCR products in the presence of 6x Loading Dye. A positive control representing the VP1 region of B19V was amplified in a PCR reaction under the same conditions and used as a reference. DNA ladder (Thermo Fisher Scientific) was used as a DNA size reference.
Detection of B19V mRNA intermediates in the heart tissue samples
2000 ng of total RNA previously treated with DNAse I was used to generate cDNA using the SuperScript III first-strand synthesis system (Thermo Fisher Scientific). The RNA fragments were primed with random hexamers, as per manufacturer’s instructions, followed by incubation for 10 min at 25°C and 50 min at 50°C in the presence of the cDNA synthesis reagents. The resulting cDNA was incubated with RNAseA (Thermo Fisher Scientific) for 20 minutes at 37°C to eliminate residual RNA. 3 µl of each processed cDNA sample was used in a PCR reaction to assess the presence of viral mRNA. Specifically, primers F 5’ ACAACTACCCGGTACTAACTATGT 3’ and R 5’ CTCCACATGCTTTTGACAGGATTA 3’ were used for PCR amplification using the Phusion High Fidelity DNA Polymerase kit (Thermo Fisher), as described above. The conditions of the PCR reaction were as follows: 98°C for 30 sec, followed by 38 cycles of 98°C for 10 sec, 60°C for 20 sec, 72°C for 20 sec, with final extension at 72°C for 10 min. The cDNA products were visualized using 1% agarose gel electrophoresis in the presence of 6x Loading Dye, and the DNA ladder was used as a size reference.
Results
Detection of B19V genome in three human heart layers (epicardium, endocardium, myocardium)
The human heart layers were processed to obtain DNA, and 75 ng of material derived from epicardium, endocardium or myocardium from each individual was assessed for the presence of the VP1 gene fragment of the B19V genome. The PCR analysis of the screened samples yielded a 389 bp band in many of the analyzed samples (Figure 2), which was sequenced to confirm the B19V identity. Two sets of independently isolated heart tissue samples from each heart layer of each patient were assessed to confirm the presence of the viral genome, with similar results. To confirm the lack of detectable PCR product in samples negative for the B19V genome, a second set of PCR reactions was performed. Briefly, 2 µl of the first PCR product were used as a template in a second round of PCR reactions, which produced no detectable amplicon. We identified the B19V amplicon in 22 out of 30 total heart layer samples. Interestingly, the B19V genome was detected in the hearts of all 10 patients, although not every single layer of the heart derived from the same patient contained the viral genome (Figures 2, 3).
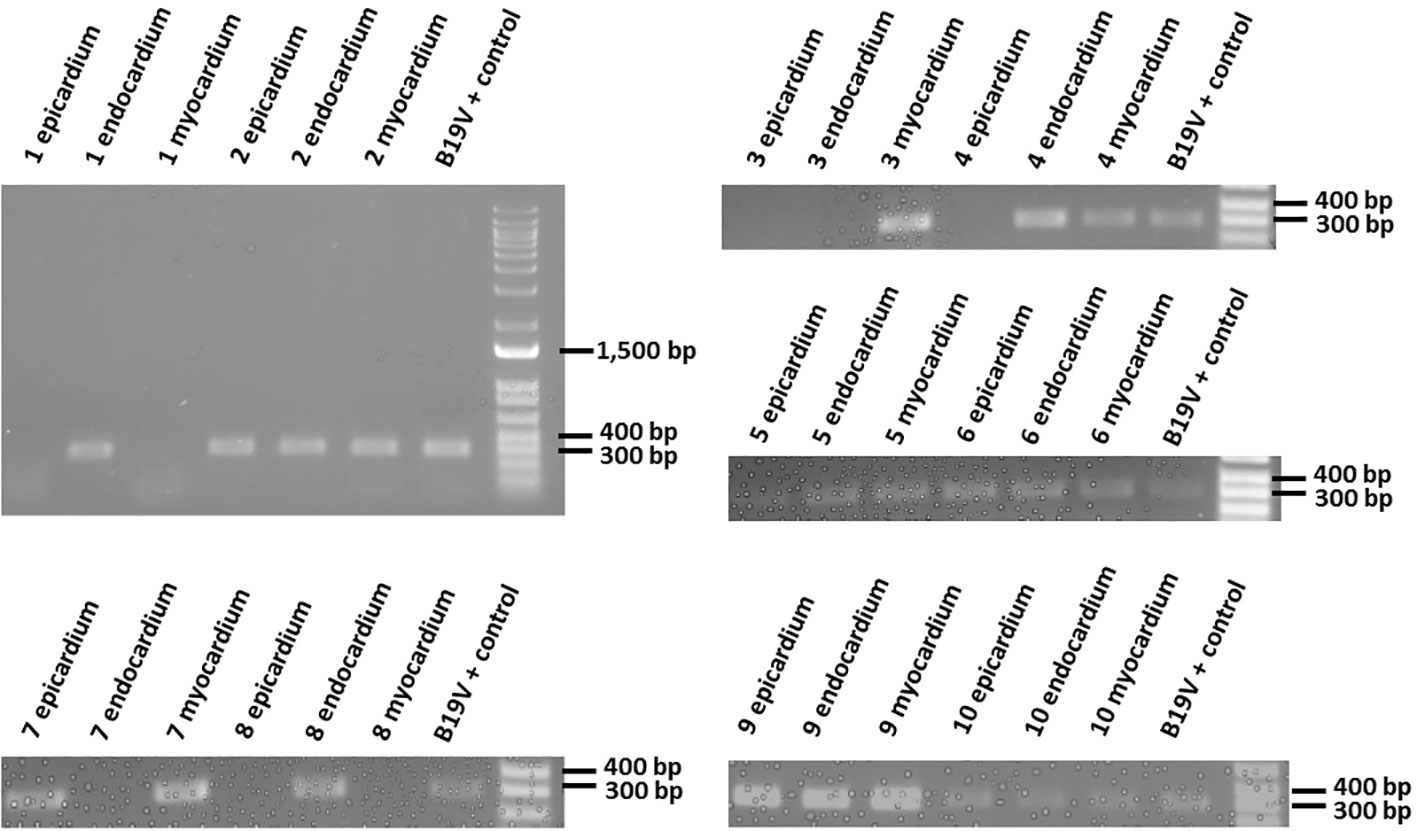
Figure 2 Amplification of the VP1 region of B19V in extracted DNA samples. Epicardium, endocardium and myocardium heart samples derived from each patient were processed to obtain DNA, and amplified in a PCR reaction with VP1 specific primers. PCR products (389bp) were visualized using 1% agarose gel electrophoresis. The last sample in each gel is a positive control for the VP1 region of the B19V genome and it is used as a reference.
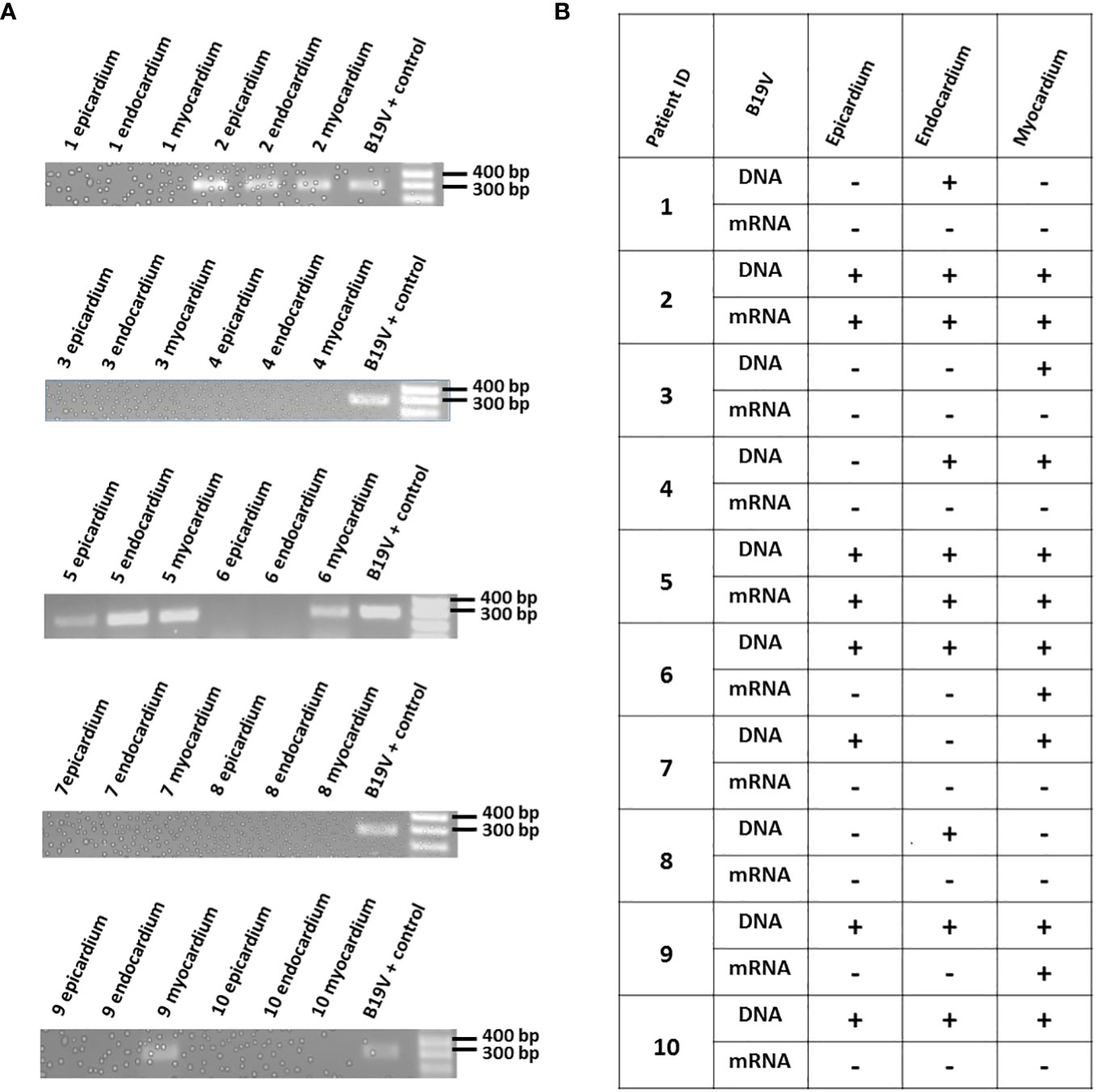
Figure 3 (A) Amplification of VP1 region in cDNA samples derived from patients' total heart tissue RNA. Epicardium, endocardium and myocardium heart samples from each patient were processed to obtain RNA, followed by reverse transcription. The resulting cDNA was amplified in a PCR reaction, and the products representing the viral mRNA were visualized using 1% agarose gel electrophoresis. A positive control for the VP1 region of B19V (DNA) was used in a PCR reaction as a reference. (B) Comparison of B19V DNA and mRNA presence in the analyzed samples. The presence or absence of the virus (DNA or mRNA) is represented as "+", or "-", respectively.
Assessment of B19V mRNA in the human heart layers
To evaluate the B19V activity in the human hearts, the total RNA extracted from all three layers of the hearts was reverse transcribed to cDNA. Prior to the procedure, all RNA samples were treated with DNAse I, purified, and the absence of the B19V PCR amplicon was confirmed using gel electrophoresis. A 389bp PCR product was detected in 8 out of 22 heart layers previously identified as positive for the presence of the B19V genome. Similar to the findings described while analyzing the viral presence in different heart layers, the B19V mRNA was not detected in every sample positive for the B19V genome (Figure 3).
Discussion
Cardiovascular disease is the leading cause of death worldwide. The heart can be susceptible to various diseases that can disrupt or weaken its natural mechanism to pump blood throughout the human body, which supplies oxygen to cells and eliminates waste products. Viruses have been previously shown to cause cardiac inflammation, which is often characterized by the onset of viral agents or the immune response to viral infection (32, 33). There is ample scientific literature that illustrates the association between B19V infection and human heart malfunction (19–21, 25–27). In this regard, B19V has been described in the context of acute myocarditis, as manifested by detectable levels of viral genome in the heart tissue, histological examination and clinical symptoms, and potential long-term outcomes (16, 17, 20, 25, 26). Past research has also proven B19V to be the leading cardiotropic virus found in patients with both DCM and cardiomyopathy (7). Even though the virus does not target cardiomyocytes, the infection of endothelial cells of the myocardial vessels drives the inflammatory responses that in turn damage the cardiac muscle (24, 34).
It has been suggested that the persistence of B19V in the cardiac tissue may lead to mitochondrial impairment, which could also contribute to the heart muscle injury over time (35). On the other hand, the causative relationship between B19V and heart disease is complicated by the fact that the viral genome can be found in endomyocardial biopsies in the absence of disease symptoms. As a result, the concept of B19V persistence in the heart tissue and its impact on cardiovascular disease remains poorly understood.
Here, we utilized a PCR strategy to detect the B19V genome in 3 heart layers of 10 individuals. Patients 1-6 had a history of heart disease at the time of death or heart transplant, whereas patients 7-10 had no history of cardiac issues at the time of death. We were able to verify the presence of the B19V genome in the heart tissues, which had been previously shown in other studies. Interestingly, we could detect the B19V genome in all of the 10 patients, albeit not in the same heart layer. For instance, the B19V genome was found in the endocardium, but not in either epicardium or myocardium obtained from Patient #1. The differential pattern of the B19V genome presence in the three heart layers derived from the same individual was also observed in Patients 3, 4, 7 & 8. On the other hand, Patients 2, 5, 6, 9 & 10 had detectable B19V genome in all three layers of their hearts.
The persistence of B19V genome has been reported in a number of studies; yet, the reactivation of the B19V virus in such cases is poorly understood. Bock et al. reported that patients suffering from myocarditis or dilated cardiomyopathy were more likely to have the B19V genome detected in their hearts than the control individuals (23). The B19V RNA replicative intermediates were found only in the heart samples derived from the inflamed hearts, and not in the control patients who had no cardiac inflammation. Whether such viral reactivation may contribute to, or exacerbate already existing heart tissue inflammation, remains to be elucidated. More recently, B19V mRNA was also detected in the endomyocardial biopsies of individuals suffering from unexplained heart failure (36). In our own analysis, the transcriptional activity of B19V was assessed by analyzing the mRNA levels. The mRNA transcripts were present in 8 out of 22 heart layer samples previously shown to be positive for the presence of the B19V genome. Interestingly, Patients # 2 & 5 were positive for the viral genome and viral mRNA in all three layers of the heart. On the other hand, Patients # 6 & 9 had detectable levels of viral mRNA in only 1 layer of the heart, even though the B19V genome was present in all three layers. These results indicate that the B19V reactivation events can occur independently in different heart layers derived from the same patient. Furthermore, B19V mRNA was detected in a single heart layer derived from an individual with no reported heart disease. In the future, it will be interesting to investigate if such reactivation leads to a localized increase in inflammation, or whether it could initiate site-specific cardiac damage.
The reliable assessment of viral reactivation is a consistent problem in the field of cardiovascular health. While an active infection is marked by a significant viral load production, a persistent type of an infection is characterized by a low viral load, which could impact the detection of viral transcripts. In the context of the heart tissue, limited sample availability presents another challenge for evaluating the impact of reactivated viral species on patients’ outcomes. Finally, heart tissues from healthy donors are not always included in the studies as controls due to ethical reasons, which could influence the interpretation of the experimental results in the context of disease. Our results present evidence for differential B19V genome presence in human heart layers derived from a single patient. Similarly, the virus can be selectively activated in different regions of the heart, as shown by the mRNA assessment. We detected the mRNA intermediates in patients who had a history of heart disease, as well as in a control, but future large-scale analyses are needed to establish how these intermediates may play a role in cardiac damage. Importantly, given the lability of the mRNA molecule, as well as the absence of the splicing products, it will be imperative to employ additional methods, such as RNA-Seq or single-cell RNA sequencing, to assess the B19V transcriptional activity. Many questions regarding the role of B19V persistence and reactivation in the human heart remain, and future studies will likely provide insights into the mechanisms that may guide B19V mediated heart disease.
Data availability statement
The original contributions presented in the study are included in the article/supplementary material. Further inquiries can be directed to the corresponding author.
Ethics statement
The studies involving humans were approved by The University of New Haven IRB. The studies were conducted in accordance with the local legislation and institutional requirements. The human samples used in this study were gifted from another research group. Written informed consent for participation was not required from the participants or the participants’ legal guardians/ next of kin in accordance with the national legislation and institutional requirements.
Author contributions
AB: Data curation, Investigation, Methodology, Writing – review & editing. AG: Investigation, Methodology, Validation, Writing – review & editing. SP: Investigation, Methodology, Writing – review & editing. KC: Resources, Writing – review & editing. AK: Data curation, Conceptualization, Writing – original draft, Writing – review & editing.
Funding
The author(s) declare financial support was received for the research, authorship, and/or publication of this article. This research was supported by the Faculty Research Fund from the University of New Haven (AK).
Acknowledgments
We thank Ahmed Abdel-Latif (University of Michigan), Maya Guglin (Indiana University School of Medicine), Mary Sheppard (University of Kentucky College of Medicine) and the UK Gill Heart & Vascular Institute, KY, for providing the human heart tissue samples. We thank the laboratory members, and our collaborators, for helpful discussions and suggestions.
Conflict of interest
The authors declare that the research was conducted in the absence of any commercial or financial relationships that could be construed as a potential conflict of interest.
Publisher’s note
All claims expressed in this article are solely those of the authors and do not necessarily represent those of their affiliated organizations, or those of the publisher, the editors and the reviewers. Any product that may be evaluated in this article, or claim that may be made by its manufacturer, is not guaranteed or endorsed by the publisher.
References
1. Qiu Y, Zhao Z, Qiu J. Sequences of seven complete genomes of human parvovirus B19. Microbiol Resour Announc (2018) 7:e00885–18. doi: 10.1128/MRA.00885-18
2. Chen AY, Zhang EY, Guan W, Cheng F, Kleiboeker S, Yankee TM, et al. The small 11kDa nonstructural protein of human parvovirus B19 plays a key role in inducing apoptosis during B19 virus infection of primary erythroid progenitor cells. Blood (2010) 115:1070–80. doi: 10.1182/blood-2009-04-215756
4. Qiu J, Söderlund-Venermo M, Young NS. Human parvoviruses. Clin Microbiol Rev (2017) 30:43–113. doi: 10.1128/CMR.00040-16
5. Serjeant BE, Hambleton IR, Kerr S, Kilty CG, Serjeant GR. Haematological response to parvovirus B19 infection in homozygous sickle-cell disease. Lancet (2001) 358:1779–80. doi: 10.1016/S0140-6736(01)06807-6
6. Rajput R, Sehgal A, Jain D, Sen R, Gupta A. Acute parvovirus B19 infection leading to severe aplastic anemia in a previously healthy adult female. Indian J Hematol Blood Transfus (2012) 28:123–6. doi: 10.1007/s12288-011-0112-0
7. Verdonschot J, Hazebroek M, Merken J, Debing Y, Dennert R, Brunner-La Rocca H-P, et al. Relevance of cardiac parvovirus B19 in myocarditis and dilated cardiomyopathy: review of the literature: Parvovirus B19 in myocarditis and DCM. Eur J Heart Fail (2016) 18:1430–41. doi: 10.1002/ejhf.665
8. Bültmann BD, Klingel K, Näbauer M, Wallwiener D, Kandolf R. High prevalence of viral genomes and inflammation in peripartum cardiomyopathy. Am J Obstetrics Gynecology (2005) 193:363–5. doi: 10.1016/j.ajog.2005.01.022
9. Dennert R, Crijns HJ, Heymans S. Acute viral myocarditis. Eur Heart J (2008) 29:2073–82. doi: 10.1093/eurheartj/ehn296
10. Schenk T, Enders M, Pollak S, Hahn R, Huzly D. High prevalence of human parvovirus B19 DNA in myocardial autopsy samples from subjects without myocarditis or dilative cardiomyopathy. J Clin Microbiol (2009) 47:106–10. doi: 10.1128/JCM.01672-08
11. Meier AB, Zawada D, De Angelis MT, Martens LD, Santamaria G, Zengerle S, et al. Epicardioid single-cell genomics uncovers principles of human epicardium biology in heart development and disease. Nat Biotechnol (2023) 41:1787–800. doi: 10.1038/s41587-023-01718-7
12. Pinto AR, Ilinykh A, Ivey MJ, Kuwabara JT, D’Antoni ML, Debuque R, et al. Revisiting cardiac cellular composition. Circ Res (2016) 118:400–9. doi: 10.1161/CIRCRESAHA.115.307778
13. Dye B, Lincoln J. The endocardium and heart valves. Cold Spring Harb Perspect Biol (2020) 12:a036723. doi: 10.1101/cshperspect.a036723
14. Bergmann O, Zdunek S, Felker A, Salehpour M, Alkass K, Bernard S, et al. Dynamics of cell generation and turnover in the human heart. Cell (2015) 161:1566–75. doi: 10.1016/j.cell.2015.05.026
15. Dewing JM, Saunders V, O’Kelly I, Wilson DI. Defining cardiac cell populations and relative cellular composition of the early fetal human heart. PloS One (2022) 17:e0259477. doi: 10.1371/journal.pone.0259477
16. Bültmann BD, Klingel K, Sotlar K, Bock CT, Baba HA, Sauter M, et al. Fatal parvovirus B19–associated myocarditis clinically mimicking ischemic heart disease: An endothelial cell–mediated disease. Hum Pathol (2003) 34:92–5. doi: 10.1053/hupa.2003.48
17. Bock C-T. Parvovirus B19: A new Emerging Pathogenic Agent of Inflammatory Cardiomyopathy. In: Schultheiss H-P, Kapp J-F, Grötzbach G, editors. Chronic Viral and Inflammatory Cardiomyopathy, Ernst Schering Research Foundation Workshop. Springer Berlin Heidelberg (2006). p. 83–97. doi: 10.1007/3-540-30822-9_6
18. Seishima M, Shibuya Y, Watanabe K, Kato G. Pericarditis and pleuritis associated with human parvovirus B19 infection in a systemic lupus erythematosus patient. Modern Rheumatol (2010) 20:617–20. doi: 10.3109/s10165-010-0330-6
19. Schowengerdt KO, Ni J, Denfield SW, Gajarski RJ, Bowles NE, Rosenthal G, et al. Association of parvovirus B19 genome in children with myocarditis and cardiac allograft rejection: diagnosis using the polymerase chain reaction. Circulation (1997) 96:3549–54. doi: 10.1161/01.CIR.96.10.3549
20. Jain P, Jain A, Khan DN, Kumar M. Human parvovirus B19 associated dilated cardiomyopathy. Case Rep (2013) 2013:bcr2013010410–bcr2013010410. doi: 10.1136/bcr-2013-010410
21. Molina KM, Garcia X, Denfield SW, Fan Y, Morrow WR, Towbin JA, et al. Parvovirus B19 myocarditis causes significant morbidity and mortality in children. Pediatr Cardiol (2013) 34:390–7. doi: 10.1007/s00246-012-0468-4
22. Klingel K, Sauter M, Bock CT, Szalay G, Schnorr J-J, Kandolf R. Molecular pathology of inflammatory cardiomyopathy. Med Microbiol Immunol (2004) 193:101–7. doi: 10.1007/s00430-003-0190-1
23. Bock C-T, Klingel K, Kandolf R. Human parvovirus B19–associated myocarditis. N Engl J Med (2010) 362:1248–9. doi: 10.1056/NEJMc0911362
24. Kandolf R. Virusätiologie der inflammatorischen Kardiomyopathie. Dtsch Med Wochenschr (2004) 129:2187–92. doi: 10.1055/s-2004-831863
25. Ackermann M, Wagner WL, Rellecke P, Akhyari P, Boeken U, Reinecke P. Parvovirus B19-induced angiogenesis in fulminant myocarditis. Eur Heart J (2020) 41:1309–9. doi: 10.1093/eurheartj/ehaa092
26. Alberti L, Loffi M, Fragasso G, Spoladore R, Ballarotto C, Margonato A. Acute heart failure caused by parvovirus B-19 myocarditis treated with human immunoglobulin. Case Rep Cardiol (2012) 2012:1–2. doi: 10.1155/2012/180871
27. Nigro G, Bastianon V, Colloridi V, Ventriglia F, Gallo P, D’Amati G, et al. Human parvovirus B19 infection in infancy associated with acute and chronic lymphocytic myocarditis and high cytokine levels: report of 3 cases and review. Clin Infect Dis (2000) 31:65–9. doi: 10.1086/313929
28. Aravindh R, Saikia UN, Mishra B, Kumari V, Sarkar S, Sharma M, et al. Persistence of human parvovirus B19 in tissues from adult individuals: a comparison with serostatus and its clinical utility. Arch Virol (2014) 159:2371–6. doi: 10.1007/s00705-014-2065-8
29. Badrinath A, Bhatta S, Kloc A. Persistent viral infections and their role in heart disease. Front Microbiol (2022) 13:1030440. doi: 10.3389/fmicb.2022.1030440
30. Blair CA, Haynes P, Campbell SG, Chung C, Mitov MI, Dennis D, et al. A protocol for collecting human cardiac tissue for research. VAD J (2016) 2(1):10.13023. doi: 10.13023/VAD.2016.12
31. Kloc A, Campbell KS, Espinoza YAU. Detection of Parvovirus B19 genome in human heart tissue samples. BMC Res Notes (2023) 16:239. doi: 10.1186/s13104-023-06527-4
32. Kindermann I, Kindermann M, Kandolf R, Klingel K, Bültmann B, Müller T, et al. Predictors of outcome in patients with suspected myocarditis. Circulation (2008) 118:639–48. doi: 10.1161/CIRCULATIONAHA.108.769489
33. Bachelier K, Biehl S, Schwarz V, Kindermann I, Kandolf R, Sauter M, et al. Parvovirus B19-induced vascular damage in the heart is associated with elevated circulating endothelial microparticles. PloS One (2017) 12:e0176311. doi: 10.1371/journal.pone.0176311
34. Mahrholdt H, Wagner A, Deluigi CC, Kispert E, Hager S, Meinhardt G, et al. Presentation, patterns of myocardial damage, and clinical course of viral myocarditis. Circulation (2006) 114:1581–90. doi: 10.1161/CIRCULATIONAHA.105.606509
35. Bironaitė D, Kažukauskienė I, Bogomolovas J, Daunoravičius D, Jakubauskas A, Vitkus D, et al. Molecular Mechanisms behind Persistent Presence of Parvovirus B19 in Human Dilated Myocardium. In: Turksen K, editor. Cell Biology and Translational Medicine, vol. 15 . Cham: Springer International Publishing (2022). p. 181–202. doi: 10.1007/5584_2021_702
Keywords: Parvovirus B19, heart infection, cardiac inflammation, viral persistence, viral detection
Citation: Badrinath A, Gardere A, Palermo SL, Campbell KS and Kloc A (2024) Analysis of Parvovirus B19 persistence and reactivation in human heart layers. Front. Virol. 4:1304779. doi: 10.3389/fviro.2024.1304779
Received: 30 September 2023; Accepted: 10 January 2024;
Published: 06 February 2024.
Edited by:
Akio Adachi, Tokushima University, JapanReviewed by:
Maria Fernanda Perdomo, University of Helsinki, FinlandLingtong Huang, Zhejiang University, China
Copyright © 2024 Badrinath, Gardere, Palermo, Campbell and Kloc. This is an open-access article distributed under the terms of the Creative Commons Attribution License (CC BY). The use, distribution or reproduction in other forums is permitted, provided the original author(s) and the copyright owner(s) are credited and that the original publication in this journal is cited, in accordance with accepted academic practice. No use, distribution or reproduction is permitted which does not comply with these terms.
*Correspondence: Anna Kloc, YWtsb2NAbmV3aGF2ZW4uZWR1