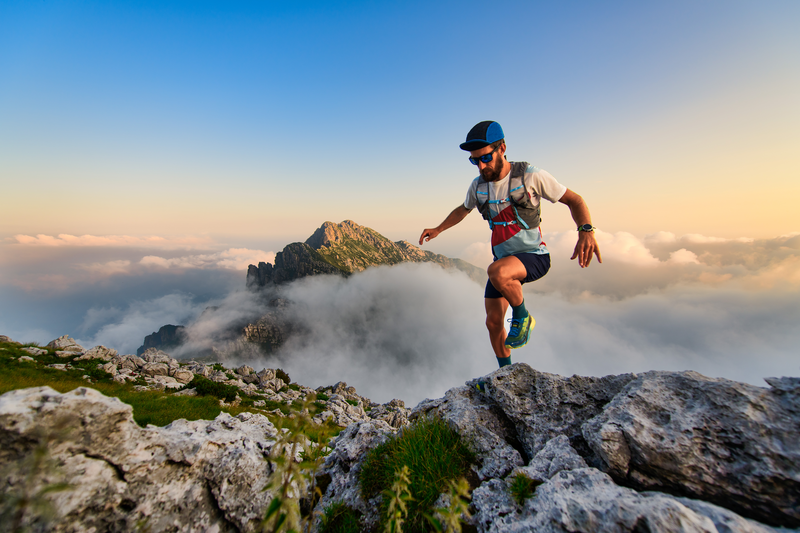
94% of researchers rate our articles as excellent or good
Learn more about the work of our research integrity team to safeguard the quality of each article we publish.
Find out more
BRIEF RESEARCH REPORT article
Front. Virol. , 08 January 2024
Sec. Emerging and Reemerging Viruses
Volume 3 - 2023 | https://doi.org/10.3389/fviro.2023.1325282
Mayaro (MAYV) and Una (UNAV) are emerging alphaviruses circulating in the Americas. Earlier reports have revealed that MAYV infects different human cell lines, including synovial and dermal fibroblasts, chondrocytes, osteoblasts, astrocytes and pericytes, as well as neural progenitor cells. In this study we evaluated the susceptibility of immortalized human microglia HMC3 cells and brain microvascular endothelial HBEC-5i cells to MAYV and UNAV infection. Cytopathic effects, cell viability, viral progeny yields, and the presence of E1 and nsP1 proteins in HMC3 and HBEC-5i cells infected with several MAYV or UNAV strains were assessed using an inverted microscope, MTT assay, plaque-forming assays, and immunofluorescence or Western blot, respectively. Finally, the expression of immune response genes was analyzed using RT-qPCR. MAYV and UNAV demonstrated strong cytopathic effects and significantly reduced cell viability in HMC3 cells. Moreover, the HMC3 cells were efficiently infected regardless of the virus strain tested, and E1 and nsP1 viral proteins were detected. In contrast, only MAYV appeared to infect HBEC-5i cells, and minimal effects on cell morphology or viability were observed. Furthermore, the MAYV titer and viral protein levels were substantially lower in the infected HBEC-5i cells when compared to those of the infected microglia cells. Finally, unlike UNAV, MAYV elicited a strong expression of specific interferon-stimulated genes in microglia cells, along with pro-inflammatory cytokines implicated in the immune response. Collectively, these findings demonstrate that MAYV and UNAV are capable of infecting relevant human brain cells.
Mayaro (MAYV) and Una (UNAV) viruses are emerging and neglected New World alphaviruses within the Togaviridae family (1). MAYV is the etiologic agent responsible for Mayaro fever, a disease with unspecific symptoms, including fever, headache, rash, leukopenia, diarrhea, myalgia, retroorbital pain and some cases, a severe polyarthralgia that can last for month to years (1, 2). In contrast, there is only serologic evidence of UNAV infection in human or non-human primates, and the symptoms associated with this infection are unknown (3–5). MAYV is actively circulating in different countries of Central and South America, mostly through an enzootic cycle in which the primary vectors are sylvatic mosquitoes Haemagogus janthinomys and the main hosts are non-human primates (6, 7). Nevertheless, laboratory and field studies suggest that urban vectors, such as Aedes aegypti or Aedes albopictus, have the capacity to transmit this virus (8, 9). On the other hand, several strains of UNAV have been isolated from Psorophora ferox and Psorophora albipes mosquitoes in different Latin American countries (10–12).
Although MAYV infections represent a potential public health threat, this virus is a poorly characterized pathogen and obtaining new information regarding viral pathogenesis and cell tropism remains fundamental. The cellular factors involved in MAYV or UNAV’s cell entry are not well understood and are largely inferred based on information from other related alphaviruses. However, the cell adhesion protein Mxra8 was recently reported as a putative receptor for Chikungunya (CHIKV), Ross River, MAYV and O’nyong nyong viruses (13). In knockout Mxra8 cells, infection was significantly reduced; however, all these viruses were able to replicate, indicating that additional factors mediate cell entry. On the other hand, Mxra8 was not implicated in UNAV’s entry (13). Interestingly, Mxra8 protein was found to be is expressed in mouse brain, specifically in astroglia end feet in the perivascular region and appears functionally associated with the blood-brain barrier (14).
Cavalheiro and colleagues demonstrated that MAYV were able to infect mouse primary macrophages and mouse macrophage-derived cell lines in a time-dependent manner (15). In these cells, MAYV infection induced the expression of tumor necrosis factor alpha and the production of reactive oxygen species (ROS), contributing to cell death (15). In another study using human hepatic HepG2 cell lines, the authors also found that MAYV infection promoted ROS production, and consequently provoked oxidative stress in these cells (16). It has been reported that MAYV induces a persistent polyarthralgia in some infected patients (2). With this in mind, Bengue and coworkers evaluated the susceptibility of human chondrocytes, fibroblast-like synoviocytes and osteoblasts, the main cell types implicated in osteoarthritis, to MAYV. Their results revealed that all these cell lines could be infected with MAYV (17). Moreover, several arthritis-related genes were upregulated in infected human chondrocytes, such as matrix metalloproteinases (MMP), including MMP1, MMP7, MMP8, MMP10, MMP13, MMP14 and MMP15 (17). Our group found that primary human dermal fibroblasts are susceptible to MAYV infection and elicit a strong expression of interferon-stimulated genes (ISGs), interferon, and cytokines, promoting an antiviral state (18). To the best of our knowledge, there are no previous studies analyzing the cell tropism of UNAV.
While MAYV infection has not been associated with neurological sequelae, recent reports indicate that MAYV efficiently infect human neural progenitor cells, pericytes and astrocytes in vitro (19, 20). Moreover, in astrocytes MAYV infection induces a strong antiviral response and the production of inflammatory mediators, which could contribute to neuroinflammation (19). Nevertheless, whether MAYV or UNAV can infect other key brain cells remains unexplored. Microglia are immune cells in the central nervous system (CNS) that play an important role during viral infection and in inflammatory diseases (21). In addition, brain microvascular endothelial cells are fundamental elements in the blood-brain barrier, a highly selective and semipermeable barrier that prevents the free passage of solutes or blood into the CNS (22). In the present study we evaluate the susceptibility of human microglia HMC3 cells and brain microvascular endothelial HBEC-5i cells to MAYV and UNAV infection.
Human microglia HMC3 cells (CRL-3304), human brain microvascular endothelial HBEC-5i cells (CRL-3245) and Vero-E6 cells (CRL-1586) were obtained from American Type Culture Collection (ATCC, Manassas, VA, USA) and grown in Eagle’s Minimum Essential Medium (EMEM), Dulbecco’s Modified Eagle’s Medium (DMEM)/Kaighn’s Modification of Ham’s F-12 Medium (F-12K), and Minimal Essential Medium (MEM), respectively. All media were supplemented with 10% fetal bovine serum (FBS), a 1% penicillin-streptomycin antibiotic solution, and 2mM of L-Glutamine (all reagents were obtained from Gibco, Waltham, MA, USA). For the endothelial cells, the medium was supplemented with 100 µg/ml of Endothelial Cell Growth Supplement (Millipore, Temecula, CA, USA). Cell lines were incubated at 37°C under a 5% CO2 atmosphere. The Mayaro (MAYV, AVR 0565, Peru; MAYV, Guyane, French Gianna; and MAYV, TRVL 4675, Trinidad and Tobago) and Una (UNAV, BT 1495-3, Panama; UNAV, 788382, Trinidad and Tobago; and UNAV, CoAr 2518, Colombia) strains used in this work were obtained from the World Reference Center for Emerging Viruses and Arboviruses (WRCEVA) at University of Texas Medical Branch (UTMB, USA) and kindly provided by Dr. Scott Weaver. All virus strains were produced in Vero-E6 cells and then titrated, aliquoted and stored as previously reported (23).
HMC3 and HBEC-5i cells were grown in 12-well or 96-well plates and then infected with MAYV or UNAV at a multiplicity of infection (MOI) of 1 for a period of 24 to 72 h. After that, images were captured with an inverted microscope to analyze cell morphology. For the cell viability experiments, we used the MTT method as previously described (23). After 24, 48 or 72 h of infection, 5 mg/ml of 3-(4,5-Dimethyl-2-thiazolyl)-2,5-diphenyltetrazolium bromide (MTT, Sigma-Aldrich, St. Louis, MI, USA) solution was added to the cells and incubated for an additional 4 h. Formazan crystals were dissolved with DMSO, and absorbance was determined at 570 nm using a microplate reader spectrophotometer (BioTeK, Winooski, VT, USA). Results are shown as the percentage of viable cells relative to untreated control cells.
HMC3 and HBEC-5i cells were infected with MAYV and UNAV at an MOI of 1 or 10. After 1 h of virus adsorption, the inoculum was removed and fresh medium was added to the cells; at the indicated times, 900 µl of cell supernatants were collected and virus titers were evaluated using plaque-forming assay.
Virus progeny production in cell supernatants was determined using plaque-forming assay as previously performed (23). For this end, 10-fold serial dilutions of MAYV- or UNAV-infected samples were used to infected confluent Vero-E6 cells grown in 6-well plates. Next, the inoculum was eliminated, and the cells were overlaid with a solution of 1% agar in MEM supplemented with 2% FBS; then, the cells were incubated at 37°C for 3 days. Subsequently, the agar was removed, and the cells were fixed with 4% formaldehyde solution in PBS and stained with 2% crystal violet dissolved in 30% methanol solution. Lastly, the number of plaques was counted, and the virus titers were reported as plaque-forming units per milliliter (PFU/ml).
HCM3 or HBEC-5i cells grown on glass coverslips in 24-well plates were infected with MAYV or UNAV at an MOI of 1 for 48 h. Next, the cells were fixed, permeabilized and blocked as previously reported (23). After that, the cells were stained overnight at 4°C with E1 or nsP1 primary rabbit antibodies (both antibodies previously validated in our laboratory) (24), followed by AlexaFlour 568 goat anti-rabbit secondary antibody (Invitrogen, Carlsbad, CA, USA). Lastly, coverslips were mounted on slides with Prolong Diamond Antifade Mountant with DAPI (Invitrogen, Carlsbad, CA, USA), and images were obtained with an FV1000 confocal microscope (Olympus, Lombard, IL, USA). The images were analyzed with ImageJ software (National Institute of Health, Bethesda, MD, USA).
Viral protein levels were evaluated by Western blot as previously described (23). Protein extracts were obtained from MAYV- or UNAV-infected HMC3 or HBEC-5i cells in Laemmli buffer with 10% dithiothreitol (Bio-Rad, Hercules, CA, USA). Proteins were fractionated in SDS-PAGE, transferred to nitrocellulose membranes, and blocked with a solution of 5% non-fat milk in T-TBS buffer for 30 min. After, the membranes were incubated with the following primary antibodies: rabbit polyclonal anti-E1, rabbit polyclonal anti-nsP1, and mouse monoclonal anti-β-actin (Cat # VMA00048, Bio-Rad, Hercules, CA, USA). Next, the membranes were washed three times with T-TBS buffer and incubated with HRP-conjugated goat anti-rabbit (Cat. # 926-80011) or goat anti-mouse (Cat. # 926-80010) secondary antibodies (LI-COR, Lincoln, NE, USA) for 1 h at room temperature. Finally, the membranes were incubated with SignalFire™ ECL Reagent (Cell Signaling Technology, Danvers, MA, USA) for 5 min, and the chemiluminescent signal was detected with a C-Digit scanner (Li-COR, Lincoln, NE, USA).
Total RNA from mock- or infected-HMC3 cells were obtained using an RNeasy Kit (Qiagen, Valencia, CA, USA) following the manufacturer’s instructions. cDNA was synthesized from 1 µg of RNA using a High-Capacity cDNA Reverse Transcription Kit, and quantitative RT-qPCR was performed using Power SYBR Green PCR Master Mix in a QuantStudio™ 5 thermocycler (Applied Biosystems, Foster City, CA, USA). The following immune response genes were detected: interferon induced with helicase C domain 1 (IFIH1), 2’-5’-oligoadenylate synthetase 2 (OAS2), MX dynamin like GTP 1 (MX1), ISG15 ubiquitin like modifier (ISG15), absent in melanoma 2 (AIM2), RNA sensor RIG-I (RIGI), interferon alpha 1 (IFNA1), interferon beta 1 (IFNB1), tumor necrosis factor (TNF), interleukin 6 (IL6), interleukin 1 beta (IL1B), C-X-C motif chemokine ligand 8 (CXCL8), C-C motif chemokine ligand 5 (CCL5), interferon regulatory factor 3 (IRF3), interferon regulatory factor 7 (IRF7), toll like receptor 3 (TLR3), and toll like receptor 7 (TLR7). All genes were previously validated (25–30) and are listed in Supplementary Table 1. Relative mRNA expression was determined using the β-actin gene for normalization according to the ΔΔ CT method (31).
All data were analyzed with the One-Way ANOVA test followed by Dunnett’s post hoc test or the Mann & Whitney test. Data analysis was performed and graphics were created with GraphPad Prism software version 10.0.3 for Mac. All experiments were performed at least 2 or 3 times with 3 replicates. For each assay, the mean and standard deviation are shown. A p value < 0.05 was considered statistically significant.
Recently it was reported that MAYV efficiently infects different human brain cells, including neural progenitor cells, pericytes and astrocytes (19). To explore whether MAYV and its closest-related alphavirus, UNAV, have the capacity to infect other human brain cells, we evaluated microglia HMC3 cells and brain microvascular endothelial HBEC-5i cells. Previous studies have revealed that MAYV can induce a potent cytopathic effect in different cell lines, including Vero cells and primary human dermal fibroblasts (18, 32). Thus, we infected HMC3 and HBEC-5i cells with MAYV or UNAV at an MOI of 1, and at different times of infection, we analyzed the cell morphology using an inverted microscope. In this experiment, we found that both MAYV and UNAV promoted substantial cytopathic effects in HMC3 cells in a time-dependent manner (Supplementary Figure 1). In contrast, we observed minor cytopathic effects in HBEC-5i cells infected with MAYV, and these cells appeared to be unaffected by UNAV infection (Supplementary Figure 1). To evaluate the impact of the MAYV or UNAV infection on the cellular integrity of HMC3 and HBEC-5i cells, we performed a kinetic infection experiment; at specific time points, cell viability was measured using the MTT method. As shown in the Figure 1, MAYV and UNAV infection provoked a significant reduction in cell viability among the HMC3 cells at 72 hours post infection (hpi) (Figure 1A). However, we only observed a decrease in cell viability among MAYV-infected HBEC-5i cells (Figure 1B). Together, these results indicate that MAYV and UNAV infection promote extensive cell damage in human microglia HMC3 cells.
Figure 1 Mayaro and Una viruses efficiently infect human microglia HMC3 cells. HMC3 (A) and HBEC-5i (B) cells were infected with MAYV (AVR0565 strain) or UNAV (BT 1495-3 strain) at an MOI of 1. At different time points, cell viability was assessed using the MTT method. HMC3 (C, D) or HBEC-5i (E) cells were infected with MAYV or UNAV at an MOI of 1 or 10 for 1h. Next, the inoculum was removed, and the cells were incubated for the indicated times. Viral progeny production in cell supernatants was quantified using plaque-forming assays. Viral titers are reported as plaque-forming units per milliliter (PFU/ml). The mean and standard deviation of three independent experiments in triplicate are shown, and the data were analyzed using the One-way ANOVA test followed by Dunnett’s post hoc test, and the Mann & Whitney test. Statistically significant differences are denoted as follows: * p < 0.05; ** p < 0.01; *** p < 0.001; **** p < 0.0001; ns: non-significant.
To evaluate whether MAYV and UNAV can replicate in HMC3 or HBEC-5i cells, we performed a kinetic infection experiment in which both cell lines were infected with MAYV or UNAV at a MOI of 1 or 10; at different time points, the viral progeny yields in cell supernatants were assessed using plaque-forming assay. In this experiment, we found increasing viral titers in the supernatants of MAYV- and UNAV-infected microglia HMC3 cells in a time- and MOI-dependent manner (Figures 1C, D). On the contrary, while MAYV demonstrated efficacy in infecting brain microvascular endothelial HBEC-5i cells, although at a low level, UNAV did not effectively infect these cells (Figure 1E). To verify these previous findings, we analyzed the expression of the E1 and nsP1 viral proteins in HMC3 or HBEC-5i cells. We performed an immunofluorescence assay in both cell lines infected with MAYV or UNAV. In this experiment, we observed strong expression of both viral proteins in MAYV- or UNAV-infected microglia HMC3 cells at 48 hpi (Figure 2A). In the case of the brain endothelial HBEC-5i cells, we noted a low expression of both proteins in MAYV-infected cells, but no expression was observed in UNAV-infected cells (Figure 2A). To further corroborate the results, we evaluated the levels of both viral proteins in MAYV- or UNAV-infected HMC3 or HBEC-5i cells using Western blot. This assay revealed that there were high levels of the E1 and nsP1 proteins for both viruses at 48 hpi in microglia HMC3 cells (Figures 2B, C). In contrast, we did not detect any viral proteins in MAYV- or UNAV-infected HBEC-5i cells (Figures 2D, E). Taken together, these results demonstrate that MAYV and UNAV competently infect human microglia HMC3 cells.
Figure 2 Detection of viral E1 and nsP1 proteins in MAYV- or UNAV-infected HMC3 and HBEC-5i cells. (A) HMC3 and HBEC-5i cells were infected with MAYV (AVR 0565 strain) or UNAV (BT 1495-3 strain) as previously performed. Following 48h of infection, the viral E1 and nsP1 proteins were assessed by immunofluorescence. A representative image of at least 10 fields is shown. Kinetic infection assay in HMC3 and HBEC-5i cells infected with MAYV (B, D) or UNAV (C, E) showing the E1 and nsP1 protein levels using Western blot. A representative blot of three independent experiments is shown. β-actin antibody was used as a loading control. WB, Western blot; MW, molecular weight; kDa, kilodaltons.
To validate our preceding results, we assessed the capacity of other MAYV and UNAV strains to infect HMC3 and HBEC-5i cells. We infected both cell lines with the MAYV Guyane and TRVL 4675 or UNAV 788382 and CoAr 2518 strains, all isolated from different Latin American countries. As we observed in our previous experiments, all the MAYV and UNAV strains tested were able to efficiently infect human microglia HMC3 cells in a time-dependent manner (Figures 3A, B). MAYV strains showed similar kinetic infection profiles in HMC3 cells (Figure 3A). However, we noted lower infection capacity with the UNAV COAR-2518 strain (Figure 3B). Again, only the MAYV strains infected the brain endothelial HBEC-5i cells, and with less efficiency (Figure 3C). These results confirm that MAYV and UNAV competently infect human microglia HMC3 cells.
Figure 3 Different strains of Mayaro and Una viruses efficiently infect human microglia HMC3 cells. HMC3 (A, B) or HBEC-5i (C) cells were infected with MAYV Guyane and TRVL 4675 or UNAV 788382 and CoAr 2518 strains; at the indicated times points, viral progeny yields in cell supernatants were assessed using plaque-forming assays. Viral titers are reported as plaque-forming units per milliliter (PFU/ml). The mean and standard deviation of three independent experiments in triplicate are shown, and the data were analyzed using the Mann & Whitney test. Statistically significant differences are denoted as follows: * p < 0.05; ** p < 0.01; *** p < 0.001; **** p < 0.0001; ns: non-significant.
Given that human microglia HMC3 cells were highly susceptible to MAYV and UNAV infection, we focused on this cell line for a more detailed characterization of the infection process with these viruses. We analyzed the expression of immune response genes, including RNA sensors, interferon, interferon-stimulated genes, cytokines, chemokines, and transcription factors in MAYV- or UNAV-infected HMC3 cells. As shown in Figure 4, we found that MAYV infection strongly triggers the expression of the RNA sensors IFIH1 and RIGI (Figures 4A, F) and the interferon-stimulated genes OAS2, MX1, ISG15 and AIM2 (Figures 4B–E). In addition, we observed an increase in expression of the cytokines IFNA1, IFNB1, TNF, IL6, CXCL8 and CCL5 (Figures 4G, H–J, L, M), the transcription factors IRF3 and IRF7, and the toll-like receptor TLR3 and TLR7 genes (Figures 4N–Q). In the case of UNAV-infected HMC3 cells, we noted a less pronounced rise in the expression of IFIH1, OAS2, MX1, ISG15, IFNB1, TNF, IL6, CXCL8, CCL5, TLR3, and TLR7 genes (Figures 4A–D, H–J, L, M, P, Q). These findings suggest that MAYV induces a potent antiviral state in HMC3 cells.
Figure 4 MAYV potently induces the expression of immune response genes in human microglia HMC3 cells. HMC3 cells were infected with MAYV (AVR 0565 strain) or UNAV (BT 1495-3) at an MOI of 1. At 24 h post infection, expression of the following immune response genes IFH1 (A), OAS2 (B), MX1 (C), ISG15 (D), AIM2 (E), RIGI (F), IFNA1 (G), IFNB1 (H), TNF (I), IL6 (J), IL1B (K), CXCL8 (L), CCL5 (M), IRF3 (N), IRF7 (O), TLR3 (P) and TLR7 (Q), was evaluated using RT-qPCR. The graphics expresses the fold change in expression for the genes tested when comparing mock control cells vs. MAYV- or UNAV-infected cells. The mean and standard deviation of two independent experiments in triplicate are shown, and the data were analyzed using the Mann & Whitney test. Statistically significant differences are denoted as follows: * p < 0.05; ** p < 0.0001; ns: non-significant.
MAYV and UNAV are emerging and neglected alphaviruses. The poor characterization of these viruses’ pathogenesis results in limited knowledge regarding their cell tropism. However, several in vitro studies demonstrate that MAYV can infect different human cell lines, among them, chondrocytes, fibroblast-like synoviocytes, osteoblasts, and dermal fibroblasts (17, 18). Moreover, it was recently reported that MAYV can infect several types of human brain cells, including pericytes, astrocytes, and progenitor neural cells (19). Conversely, to our best knowledge, there is not information available regarding UNAV’s cell tropism.
In the present study we evaluated the susceptibility of immortalized human microglia HMC3 cells and brain microvascular endothelial HBEC-5i cells to MAYV and UNAV infection. Our results indicate that MAYV as well as UNAV promoted substantial cytopathic effects in microglia HMC3 cells in a time-dependent manner. Also, we observed a significant reduction in cell viability among the HMC3 cells infected with these viruses. In contrast, only MAYV appeared to have an effect on brain microvascular endothelial HBEC-5i cells, and this effect was less pronounced. These results are in agreement with previous studies that demonstrating that MAYV, as well as similar alphaviruses, are able to induce a potent cytopathic effect in Vero cells and human dermal fibroblasts in vitro (18, 32).
To verify if HMC3 or HBEC-5i cells might be susceptible to MAYV and UNAV infection, we performed a kinetic infection experiment; at specific time points, we quantified viral progeny production in the supernatants of both cell lines. In these assays, we found that microglia HMC3 cells were vulnerable to infection with all MAYV and UNAV strains tested. In addition, we detected a strong expression of the viral E1 and nsP1 proteins in MAYV- or UNAV-infected HMC3 cells at 48 hpi, corroborating our previous findings. Similar results were observed in microglia cells infected with CHIKV, another arthritogenic alphavirus closely related to MAYV and UNAV (33). An additional study demonstrated that CHIKV was able to infect microglia HMC3 cells (34). However, the present study demonstrated only MAYV strains were able to infect brain microvascular endothelial HBEC-5i cells, and with a very limited capacity. It has been reported that Sindbis virus, a prototype member of the Alphavirus genus, is also capable of infecting human brain endothelial cells (35).
To further characterize the effect of MAYV and UNAV infection in microglia HMC3 cells, we assessed the expression of immune response genes using RT-qPCR. Our data indicate that unlike UNAV, MAYV infection potently induced the expression of RNA sensors, interferon, interferon-stimulated genes, and pro-inflammatory cytokines, promoting a general antiviral state. Similar results have been observed in astrocytes and human dermal fibroblasts infected with MAYV (18, 19).
Although MAYV has not been associated with neurological sequelae clinically, our findings indicate that MAYV is able to infect, at least in vitro, vital brain cells, such as microglia and endothelial cells. It is important to highlight that CHIKV has been associated with neurological complications in human infection, including encephalopathy, encephalitis, encephalomyelopathy, myeloneuropathy, acute disseminate encephalomyelitis, and Guillain-Barré syndrome, among others (36). Thus, studying the capacity of MAYV or UNAV to infect relevant human brain cells and their potential ability to produce neurological complications is of particular interest. In this context, it would be valuable to investigate if these viruses can infect neurons, given that at least MAYV has the capacity to infect brain endothelial cells. Thus, our results should be evaluated in animal models of MAYV or UNAV infection to explore the possible roles of these viruses in neuropathogenesis.
The original contributions presented in the study are included in the article/Supplementary Material. Further inquiries can be directed to the corresponding author.
DC: Conceptualization, Formal analysis, Investigation, Methodology, Supervision, Validation, Writing – review & editing. MS-S: Investigation, Methodology, Writing – review & editing. PV-T: Investigation, Methodology, Writing – review & editing. PEG-J: Investigation, Methodology, Writing – review & editing. DZ: Investigation, Methodology, Writing – review & editing. JG-S: Conceptualization, Formal analysis, Funding acquisition, Investigation, Methodology, Project administration, Resources, Supervision, Validation, Writing – original draft, Writing – review & editing.
The author(s) declare financial support was received for the research, authorship, and/or publication of this article. This research was funded by the Ministerio de Economía y Finanzas de Panamá (MEF), grant number 19911.012 (JG-S) and partially supported by the Sistema Nacional de Investigación (SNI) from Secretaría Nacional de Ciencia, Tecnología e Innovación de Panamá (SENACYT), grant number 23-2021 (JG-S). PV-T and DZ were supported by a Master of Science fellowship from SENACYT and Universidad de Panamá, grant number 014-2021.
We thank Scott Weaver (WRCEVA, UTMB, USA) for providing the Mayaro and Una virus strains. We also give thanks to Rodolfo Contreras and Nicanor Obaldía for their support with laboratory and equipment facilities. Finally, we express our gratitude to Jorge Ceballos and the Smithsonian Tropical Research Institute for their assistance and granting access to the confocal microscope.
The authors declare that the research was conducted in the absence of any commercial or financial relationships that could be construed as a potential conflict of interest.
All claims expressed in this article are solely those of the authors and do not necessarily represent those of their affiliated organizations, or those of the publisher, the editors and the reviewers. Any product that may be evaluated in this article, or claim that may be made by its manufacturer, is not guaranteed or endorsed by the publisher.
The Supplementary Material for this article can be found online at: https://www.frontiersin.org/articles/10.3389/fviro.2023.1325282/full#supplementary-material
1. Acosta-Ampudia Y, Monsalve DM, Rodriguez Y, Pacheco Y, Anaya JM, Ramirez-Santana C. Mayaro: an emerging viral threat? Emerg Microbes Infect (2018) 7:163. doi: 10.1038/s41426-018-0163-5
2. Arenivar C, Rodriguez Y, Rodriguez-Morales AJ, Anaya JM. Osteoarticular manifestations of Mayaro virus infection. Curr Opin Rheumatol (2019) 31:512–6. doi: 10.1097/BOR.0000000000000635
3. Diaz LA, Spinsanti LI, Almiron WR, Contigiani MS. UNA virus: first report of human infection in Argentina. Rev Inst Med Trop Sao Paulo (2003) 45:109–10. doi: 10.1590/S0036-46652003000200012
4. Diaz LA, Diaz Mdel P, Almiron WR, Contigiani MS. Infection by UNA virus (Alphavirus; Togaviridae) and risk factor analysis in black howler monkeys (Alouatta caraya) from Paraguay and Argentina. Trans R Soc Trop Med Hyg (2007) 101:1039–41. doi: 10.1016/j.trstmh.2007.04.009
5. Perez JG, Carrera JP, Serrano E, Pitti Y, Maguina JL, Mentaberre G, et al. Serologic evidence of zoonotic alphaviruses in humans from an indigenous community in the Peruvian amazon. Am J Trop Med Hyg (2019) 101:1212–8. doi: 10.4269/ajtmh.18-0850
6. Diagne CT, Bengue M, Choumet V, Hamel R, Pompon J, Misse D. Mayaro virus pathogenesis and transmission mechanisms. Pathogens (2020) 9:738. doi: 10.3390/pathogens9090738
7. Ganjian N, Riviere-Cinnamond A. Mayaro virus in latin america and the caribbean. Rev Panam Salud Publica (2020) 44:e14. doi: 10.26633/RPSP.2020.14
8. Pereira TN, Carvalho FD, De Mendonca SF, Rocha MN, Moreira LA. Vector competence of Aedes aEgypti, Aedes albopictus, and Culex quinquefasciatus mosquitoes for Mayaro virus. PloS Negl Trop Dis (2020) 14:e0007518. doi: 10.1371/journal.pntd.0007518
9. de Curcio JS, Salem-Izacc SM, Pereira Neto LM, Nunes EB, Anunciacao CE, Silveira-Lacerda EP. Detection of Mayaro virus in Aedes aEgypti mosquitoes circulating in Goiania-Goias-Brazil. Microbes Infect (2022) 24:104948. doi: 10.1016/j.micinf.2022.104948
10. Causey OR, Casals J, Shope RE, Udomsakdi S. Aura and una, two new group a arthropod-borne viruses. Am J Trop Med Hyg (1963) 12:777–81. doi: 10.4269/ajtmh.1963.12.777
11. Haas RA, Arron-Leeuwin AE. Arboviruses isolated from mosquitos and man in Surinam. Trop Geogr Med (1975) 27:409–12.
12. Walder R, Suarez OM, Calisher CH. Arbovirus studies in southwestern Venezuela during 1973-1981. II. Isolations and further studies of Venezuelan and eastern equine encephalitis, Una, Itaqui, and Moju viruses. Am J Trop Med Hyg (1984) 33:483–91. doi: 10.4269/ajtmh.1984.33.483
13. Zhang R, Kim AS, Fox JM, Nair S, Basore K, Klimstra WB, et al. Mxra8 is a receptor for multiple arthritogenic alphaviruses. Nature (2018) 557:570–4. doi: 10.1038/s41586-018-0121-3
14. Yonezawa T, Ohtsuka A, Yoshitaka T, Hirano S, Nomoto H, Yamamoto K, et al. Limitrin, a novel immunoglobulin superfamily protein localized to glia limitans formed by astrocyte endfeet. Glia (2003) 44:190–204. doi: 10.1002/glia.10279
15. Cavalheiro MG, Costa LS, Campos HS, Alves LS, Assuncao-Miranda I, Poian AT. Macrophages as target cells for Mayaro virus infection: involvement of reactive oxygen species in the inflammatory response during virus replication. Acad Bras Cienc (2016) 88:1485–99. doi: 10.1590/0001-3765201620150685
16. Camini FC, Da Silva Caetano CC, Almeida LT, Da Costa Guerra JF, De Mello Silva B, De Queiroz Silva S, et al. Oxidative stress in Mayaro virus infection. Virus Res (2017) 236:1–8. doi: 10.1016/j.virusres.2017.04.017
17. Bengue M, Ferraris P, Baronti C, Diagne CT, Talignani L, Wichit S, et al. Mayaro virus infects human chondrocytes and induces the expression of arthritis-related genes associated with joint degradation. Viruses (2019) 11:797. doi: 10.3390/v11090797
18. Sugasti-Salazar M, Llamas-Gonzalez YY, Campos D, Gonzalez-Santamaria J. Inhibition of p38 mitogen-activated protein kinase impairs mayaro virus replication in human dermal fibroblasts and heLa cells. Viruses (2021) 13:1156. doi: 10.3390/v13061156
19. Bengue M, Ferraris P, Barthelemy J, Diagne CT, Hamel R, Liegeois F, et al. Mayaro virus infects human brain cells and induces a potent antiviral response in human astrocytes. Viruses (2021) 13:465. doi: 10.3390/v13030465
20. Geddes VEV, Brustolini OJB, Cavalcante LTF, Moreira FRR, De Castro FL, Guimaraes APC, et al. Common dysregulation of innate immunity pathways in human primary astrocytes infected with chikungunya, mayaro, oropouche, and zika viruses. Front Cell Infect Microbiol (2021) 11:641261. doi: 10.3389/fcimb.2021.641261
21. Hatton CF, Duncan CJA. Microglia are essential to protective antiviral immunity: lessons from mouse models of viral encephalitis. Front Immunol (2019) 10, 2656. doi: 10.3389/fimmu.2019.02656
22. Brandl S, Reindl M. Blood-brain barrier breakdown in neuroinflammation: current in vitro models. Int J Mol Sci (2023) 24:12699. doi: 10.3390/ijms241612699
23. Valdes-Torres P, Campos D, Bhakta M, Galan-Jurado PE, Durant-Archibold AA, Gonzalez-Santamaria J. Honokiol and alpha-mangostin inhibit mayaro virus replication through different mechanisms. Molecules (2022) 27:7362. doi: 10.3390/molecules27217362
24. Llamas-Gonzalez YY, Campos D, Pascale JM, Arbiza J, Gonzalez-Santamaria J. A functional ubiquitin-proteasome system is required for efficient replication of new world mayaro and una alphaviruses. Viruses (2019) 11:370. doi: 10.3390/v11040370
25. Bektas N, Noetzel E, Veeck J, Press MF, Kristiansen G, Naami A, et al. The ubiquitin-like molecule interferon-stimulated gene 15 (ISG15) is a potential prognostic marker in human breast cancer. Breast Cancer Res (2008) 10:R58. doi: 10.1186/bcr2117
26. Stevenson NJ, Murphy AG, Bourke NM, Keogh CA, Hegarty JE, O’farrelly C. Ribavirin enhances IFN-alpha signalling and MxA expression: a novel immune modulation mechanism during treatment of HCV. PloS One (2011) 6:e27866. doi: 10.1371/journal.pone.0027866
27. da Conceicao TM, Rust NM, Berbel AC, Martins NB, Do Nascimento Santos CA, Da Poian AT, et al. Essential role of RIG-I in the activation of endothelial cells by dengue virus. Virology (2013) 435:281–92. doi: 10.1016/j.virol.2012.09.038
28. Devhare PB, Chatterjee SN, Arankalle VA, Lole KS. Analysis of antiviral response in human epithelial cells infected with hepatitis E virus. PloS One (2013) 8:e63793. doi: 10.1371/journal.pone.0063793
29. Hamel R, Dejarnac O, Wichit S, Ekchariyawat P, Neyret A, Luplertlop N, et al. Biology of zika virus infection in human skin cells. J Virol (2015) 89:8880–96. doi: 10.1128/JVI.00354-15
30. Chen F, Zhang G, Yu L, Feng Y, Li X, Zhang Z, et al. High-efficiency generation of induced pluripotent mesenchymal stem cells from human dermal fibroblasts using recombinant proteins. Stem Cell Res Ther (2016) 7:99. doi: 10.1186/s13287-016-0358-4
31. Livak KJ, Schmittgen TD. Analysis of relative gene expression data using real-time quantitative PCR and the 2(-Delta Delta C(T)) Method. Methods (2001) 25:402–8. doi: 10.1006/meth.2001.1262
32. Barroso MM, Lima CS, Silva-Neto MA, Da Poian AT. Mayaro virus infection cycle relies on casein kinase 2 activity. Biochem Biophys Res Commun (2002) 296:1334–9. doi: 10.1016/S0006-291X(02)02093-4
33. Abere B, Wikan N, Ubol S, Auewarakul P, Paemanee A, Kittisenachai S, et al. Proteomic analysis of chikungunya virus infected microgial cells. PloS One (2012) 7:e34800. doi: 10.1371/journal.pone.0034800
34. Qadri SW, Kumar N, Santhoshkumar R, Desai A, Ravi V, Venkataswamy MM. Infection of human microglial cell line CHME-3 to study neuropathogenesis of chikungunya virus. J Neurovirol (2022) 28:374–82. doi: 10.1007/s13365-022-01070-7
35. Rust NM, Papa MP, Scovino AM, Da Silva MM, Calzavara-Silva CE, Marques ET Jr., et al. Bradykinin enhances Sindbis virus infection in human brain microvascular endothelial cells. Virology (2012) 422:81–91. doi: 10.1016/j.virol.2011.10.003
Keywords: Mayaro, Una, alphaviruses, human microglia HMC3 cells, human brain microvascular endothelial HBEC-5i cells, infection, susceptibility
Citation: Campos D, Sugasti-Salazar M, Valdés-Torres P, Galán-Jurado PE, Zegarra D and González-Santamaría J (2024) Differential susceptibility of human microglia HMC3 cells and brain microvascular endothelial HBEC-5i cells to Mayaro and Una virus infection. Front. Virol. 3:1325282. doi: 10.3389/fviro.2023.1325282
Received: 20 October 2023; Accepted: 19 December 2023;
Published: 08 January 2024.
Edited by:
Perumal Arumugam Desingu, Indian Institute of Science (IISc), IndiaReviewed by:
Daniel Adesse, Oswaldo Cruz Foundation (Fiocruz), BrazilCopyright © 2024 Campos, Sugasti-Salazar, Valdés-Torres, Galán-Jurado, Zegarra and González-Santamaría. This is an open-access article distributed under the terms of the Creative Commons Attribution License (CC BY). The use, distribution or reproduction in other forums is permitted, provided the original author(s) and the copyright owner(s) are credited and that the original publication in this journal is cited, in accordance with accepted academic practice. No use, distribution or reproduction is permitted which does not comply with these terms.
*Correspondence: José González-Santamaría, amdvbnphbGV6c2FudGFtYXJpYUBnb3JnYXMuZ29iLnBh
Disclaimer: All claims expressed in this article are solely those of the authors and do not necessarily represent those of their affiliated organizations, or those of the publisher, the editors and the reviewers. Any product that may be evaluated in this article or claim that may be made by its manufacturer is not guaranteed or endorsed by the publisher.
Research integrity at Frontiers
Learn more about the work of our research integrity team to safeguard the quality of each article we publish.