- 1International Centre for Genetic Engineering and Biotechnology (ICGEB), Cape Town, South Africa
- 2Department of Biology & Chemistry, Faculty of Basic Sciences and Engineering, Universidad de los Llanos, Villavicencio, Colombia
- 3Institute of Infectious Disease and Molecular Medicine (IDM), Faculty of Health Sciences, University of Cape Town, Cape Town, South Africa
- 4Department of Integrative Biomedical Sciences, Faculty of Health Sciences, University of Cape Town, Cape Town, South Africa
- 5Wellcome Centre for Infectious Disease Research in Africa, University of Cape Town, Cape Town, South Africa
- 6Division of Medical Virology, Department of Pathology, University of Cape Town, Cape Town, South Africa
- 7Department of Infectious Diseases, Imperial College London, London, United Kingdom
- 8The Francis Crick Institute, London, United Kingdom
- 9Desmond Tutu Health Foundation, Cape Town, South Africa
- 10Department of Medicine, Faculty of Health Sciences, University of Cape Town, Cape Town, South Africa
- 11School of Animal Sciences, Faculty of Agricultural Sciences and Natural Resources, Universidad de los Llanos, Villavicencio, Colombia
Background: Globally, the most significant risk factors for adverse COVID-19 outcome are increasing age and cardiometabolic comorbidities. However, underlying coinfections may modulate COVID-19 morbidity and mortality, particularly in regions with high prevalence of infectious diseases.
Methods: We retrospectively analyzed serum samples for IgG antibodies against the common circulating coronaviruses HCoV-NL63, HCoV-229E, HCoV-OC43 and HCoV-HKU1 from non-hospitalized and hospitalized confirmed COVID-19 patients recruited during the first (June-August 2020) and second (October 2020-June 2021) COVID-19 wave in Cape Town, South Africa. Patients were grouped according to COVID-19 disease severity: Group 1: previously SARS-CoV-2 infected with positive serology and no symptoms (n=94); Group 2: acutely SARS-CoV-2 infected, hospitalized for COVID-19 and severe symptoms (n=92).
Results: The overall anti-HCoV IgG seroprevalence in the entire patient cohort was 60.8% (95% CI: 53.7 – 67.8), with 37.1% HCoV-NL63 (95% CI: 30 – 44), 30.6% HCoV-229E (95% CI: 24 – 37.3), 22.6% HCoV-HKU1 (95% CI: 16.6 – 28.6), and 21.0% HCoV-OC43 (95% CI: 15.1 – 26.8). We observed a significantly higher overall HCoV presence (72.3% versus 48.9%) and coinfection frequency (43.6% versus 19.6%) in group 1 compared to group 2 patients with significantly higher presentation of HCoV-NL63 (67.0% versus 6.6%) and HCoV-HKU1 (31.1% versus 14.1%). However, only antibody titers for HCoV-NL63 were significantly higher in group 1 compared to group 2 patients (p< 0.0001, 1.90 [95% CI: 0.62 – 2.45] versus 1.32 [95% CI: 0.30 – 2.01]) which was independent of the participants’ HIV status. Logistic regression analysis revealed significantly protective effects by previous exposure to HCoV-NL63 [p< 0.001, adjusted OR = 0.0176 (95% CI: 0.0039 – 0.0786)], while previous HCoV-229E exposure was associated with increased COVID-19 severity [p = 0.0051, adjusted OR = 7.3239 (95% CI: 1.8195–29.4800)].
Conclusion: We conclude that previous exposure to multiple common coronaviruses, and particularly HCoV-NL63, might protect against severe COVID-19, while no previous HCoV exposure or single infection with HCoV-229E might enhance the risk for severe COVID-19. To our knowledge, this is the first report on HCoV seroprevalence in South Africa and its possible association with cross-protection against COVID-19 severity.
Introduction
In Sub-Saharan Africa (SSA), the COVID-19 pandemic occurred against the background of high prevalence of communicable diseases, such as Human immunodeficiency virus (HIV), tuberculosis and malaria, as well as inadequate health care systems and numerous socioeconomic factors (1). There was substantial concern that this would exacerbate COVID-19 outcome in these already vulnerable populations. However, as seen globally, increasing age was by far the highest risk for adverse COVID-19 outcome, and a large cohort study conducted in South Africa involving >200,000 hospitalized COVID-19 patients reported advanced age as a strong predictor for in-hospital mortality with odds ratios (OR) of 11.29 (age group 60-69 years), 15.93 (age group 70-79 years) and 20.67 (age group >80 years) (2). Other risk factors included cardiometabolic comorbidities (ORs between 1.07 and 2.21), while HIV infection and past and current tuberculosis increased the risk of COVID-19 mortality only slightly (OR 1.34 and 1.48, respectively) (2). This is supported by the WHO Global COVID-19 Clinical Data Platform which identified HIV as a slight risk factor for severe critical illness at hospital admission (OR 1.13) and in-hospital mortality (OR 1.30) (3). In addition, other underlying infections have been proposed to aggravate the clinical picture of COVID-19, such as Influenza viruses (4); lytic oncogenic herpesvirus infections such as Kaposi’s sarcoma-associated herpesvirus (KSHV) (5) and Epstein-Barr virus (EBV) (6); fungal coinfections such as Aspergillus spp., Mucor spp., Rhizopus spp., and Candida spp (7). and bacteria such as Mycoplasma spp., Pseudomonas spp., Heamophilus spp. and Chlamydia spp (8). These findings highlight the importance of screening other relevant co-circulating pathogens that contribute to COVID-19 etiology (8).
While the lower numbers in Severe Acute Respiratory Syndrome Coronavirus 2 (SARS-CoV-2) infections and COVID-19 morbidity and mortality in SSA compared to Europe, North America and Asia could certainly have been affected by suboptimal reporting due to lower levels of infrastructure for diagnostics and epidemiological surveillance, other explanations were proposed such as the relatively younger age structure (9) and/or previous exposure to other circulating Human Coronaviruses (HCoV) which could have elicited cross-protective humoral and/or cellular responses against conserved epitopes (10–12). Indeed, it was recently reported that the prevalence of SARS-CoV-2 serological cross-reactivity was significantly higher in samples from SSA compared with the USA (13), suggesting that prior exposure to HCoV may have induced protective responses against SARS-CoV-2.
There are seven known HCoV that cause respiratory diseases, of which four globally circulating seasonal strains are known to cause the “common cold” with mild symptoms in majority, namely HCoV-OC43, HCoV-HKU-1, HCoV-NL63 and HCoV-229E; whereas three cause severe infections, namely Severe Acute Respiratory Syndrome Coronavirus 1 (SARS-CoV-1), Middle East Respiratory Syndrome-Related Coronavirus (MERS) and SARS-CoV-2 (14). Among the HCoV, the strains HCoV-NL63 and HCoV-229E belong to the alpha-CoV genus, while the remaining five strains belong to the beta-CoV phylogenetic cluster (15). The seasonal HCoV share partial genomic sequence homology with SARS-CoV-2 with the two beta-CoV (HCoV-OC43 and HCoV-HKU1) sharing 50.5% and 51.6% identity, respectively, followed by the alpha-HCoV-NL63 (48.7%) and HCoV-229E (47.7%) (16). Of the structural proteins Spike (S), Membrane (M) and Nucleocapsid (N) which have been reported to be involved in T cell responses (11), protein sequence homology among SARS-CoV-2 and HCoV revealed identities between 29% and 47% with HCoV-OC43 having the most similar structural proteins to SARS-CoV-2 (16). It is therefore reasonable to assume that pre-pandemic infection with seasonal HCoV might contribute to serological and/or cellular cross-protection against severity of SARS-CoV-2 infection.
To our knowledge, no serological studies on HCoV have been conducted in South Africa that assessed HCoV pre-exposure and COVID-19 outcome, and data on acute HCoV infections in South Africa have been primarily obtained from pediatric patients (17–21). To address the question whether previous exposure to HCoV might have played a protective role against COVID-19 morbidity and mortality in South Africa, we set up an in-house ELISA platform to retrospectively test convalescent patient plasma for antibodies recognizing the N protein of HCoV as most cross-reactive responses have previously been described to target SARS-CoV-2 N (13). We directly compared two cohorts of patients who were recruited during the first and second COVID-19 wave, respectively, in Cape Town, South Africa. All patients displayed positive serology for SARS-CoV-2; however, one patient group was asymptomatic at the time of recruitment without history of severe COVID-19 disease, while the other group represented acutely infected hospitalized COVID-19 patients. Assuming that pre-existing immunological memory of HCoV is unlikely to prevent infection with SARS-CoV-2 (10), we herein report that it may mitigate COVID-19 disease manifestations. In addition, this study for the first time determined seroprevalence of anti-HCoV immunoglobulins class G (IgG) in Southern Africa.
Materials and methods
Study cohort
An analytical retrospective observational cross-sectional study of South African patients exposed to SARS-CoV-2 (n=186) was conducted. Patients were grouped into two categories: 1. previously SARS-CoV-2 infected with positive serology and no symptoms; 2. acutely SARS-CoV-2 infected, hospitalized for COVID-19 and severe symptoms. The demographic and clinical characteristics of all patients are presented in Table 1.
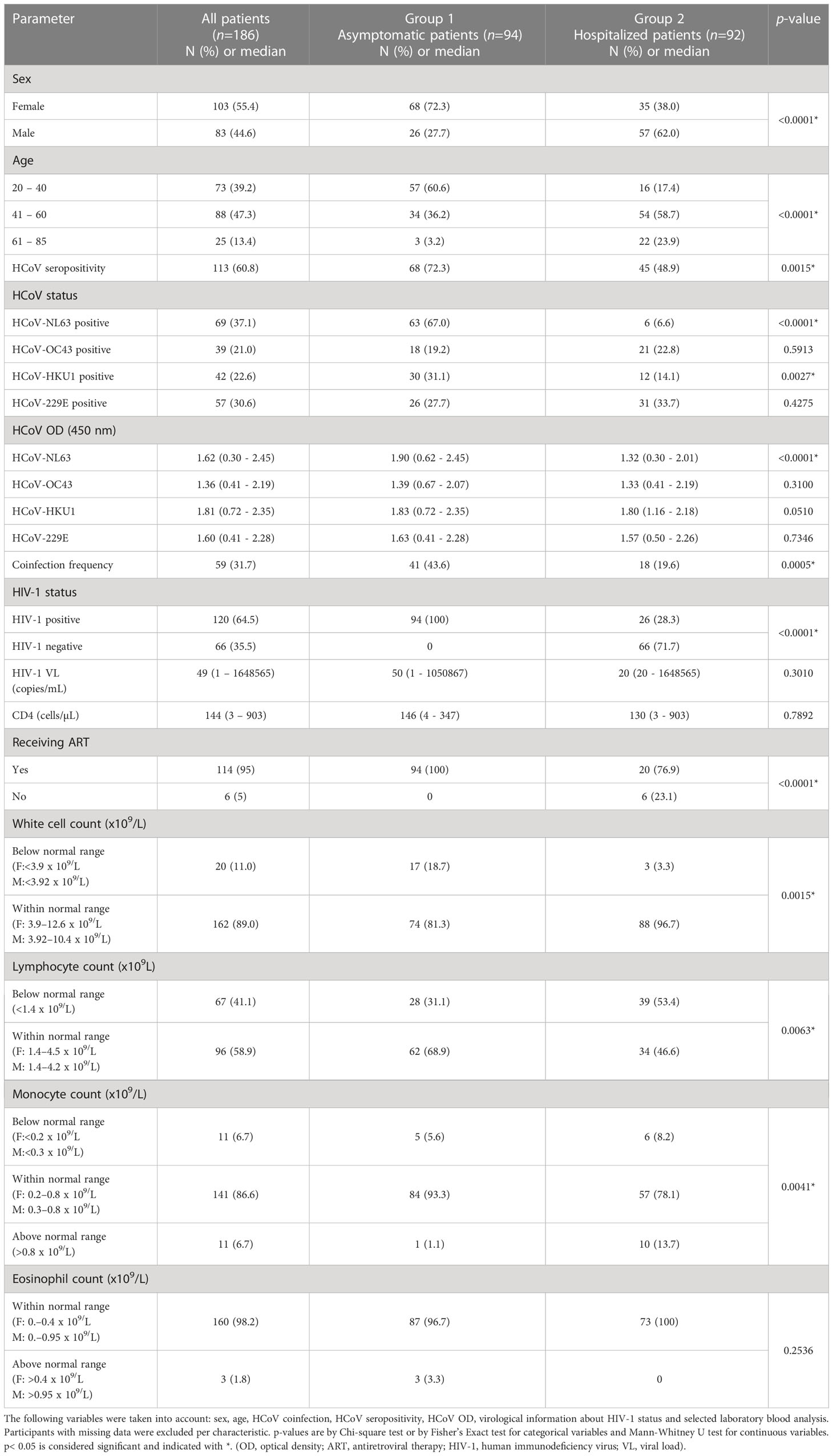
Table 1 Baseline demographic and clinical characteristics of the two patient groups (n = 186) according to COVID-19 severity (group 1: asymptomatic with positive SARS-CoV-2 serology, n = 94; group 2: hospitalized COVID-19 patients, n = 92).
Group 1 consisted of a total of 94 non-hospitalized HIV-infected adults with positive serology for SARS-CoV-2 presenting for routine HIV treatment at the Gugulethu Community Health Centre Antiretroviral clinic (Desmond Tutu Centre) between October 2020 and June 2021. The patient cohort has been described previously (22). Group 2 was comprised of 92 hospitalized HIV-positive and negative adult patients with RT-qPCR-proven SARS-CoV-2 infection recruited from Groote Schuur Hospital between June and August 2020 as described before (23). Patients’ recruitment coincided with the first wave (group 2) and second wave (group 1) of COVID-19 disease in Cape Town, South Africa. None of the patients were vaccinated against SARS-CoV-2.
The study was conducted according to the declaration of Helsinki, conformed to South African Good Clinical Practice guidelines. The recruitment of both patient groups was approved by the University of Cape Town’s Health Sciences Research Ethical Committee (HREC 134/2020 and HREC 207/2020). Written informed consent was obtained from all participants. For patients in group 2, relatives provided proxy consent for participants without capacity to consent for themselves.
Sample size
The sample size was estimated with the calculation used for classical analytical cross-sectional studies in epidemiology: n = [DEFF * Np (1 – p)]/[(d2/Z2 1- α/2*(N-1) + p * (1 – p)], with DEFF (design effect), N (population size), p (anticipated frequency), d (confidence limits as % of 100) and Z (level of confidence according to the standard normal distribution). For this estimation the OpenEpi software (Open-Source Epidemiologic Statistics for Public Health) v. 3.01 from the US Centers for Disease Control and Prevention (CDC) (http://www.openepi.com/SampleSize/SSPropor.htm) was used. Since serological data from South Africa were lacking, the expected proportion (p) of patients previously infected with a common coronavirus was established as 3.7% according to the epidemiological study of acute respiratory virus infections detected among hospitalized patients in South Africa (18) who found the following acute HCoV prevalences: HCoV-229E (3.7%) and other HCoV (< 2%). Since 3.7% was the highest prevalence among the four human coronaviruses, this value was taken as reference for the sample size estimation. The study population (N) corresponded to 322444 people (i.e. confirmed COVID-19 cases in the Western Cape, South Africa as of June 30, 2021, https://sacoronavirus.co.za/2021/06/30/update-on-covid-19-30-june-2021/). For this study, the confidence interval (CI) of 95% was used and a total of 186 serums samples was analyzed.
Clinical data
Clinical data of the asymptomatic patients (group 1) was collected at enrollment, including any self-reported symptoms. Routine clinical analyses of peripheral blood samples taken at enrolment were performed by the National Health Laboratory Services (NHLS). Absolute CD4 count was determined using the Aquios PLG panel (CD45-FITC/CD4 PE monoclonal antibodies), while HIV-1 Viral Load (VL) was measured by the ALINITY mHIV-1 assay (Abbott Molecular Inc., Des Plaines, IL, USA). Full blood count and differential cell count were also performed. Previous exposure to SARS-CoV-2 was determined by in-house ELISA against SARS-CoV-2 IgG antibodies using RBD and S1 proteins as previously described (22). Only SARS-CoV-2 seropositive patient samples (n=94) were used in the present study.
Patients hospitalized with COVID-19 (group 2) were tested for SARS-CoV-2 by diagnostic RT-qPCR (Seegene, Roche or Gene Xpert) on the day of enrollment using nasopharyngeal or oropharyngeal aspirates. Full blood count, differential cell count and HIV-1 VL was also determined upon enrolment. All tests were performed by NHLS. Absolute CD4 count (for HIV-infected patients) and white cell counts (WCC) were obtained from patients’ medical files. Detailed clinical data not relevant to this study have been reported before (23). All data are reported using NHLS defined thresholds and ranges as previously determined to be applicable for the general population of South Africa. Clinical, demographic and experimental data were recorded and stored on an electronic REDCap database (24), hosted by the University of Cape Town.
HCoV serology
To determine serology against the four common coronaviruses HCoV-OC43, HCoV-NL63, HCoV-HKU1 and HCoV-229E, in-house indirect ELISA assays were developed, which were based on previously published methodologies (25, 26). Briefly, 3 μg/mL of recombinant N proteins (recombinant HCoV-OC43 N His-tag Protein (Bio-techne, USA), recombinant HCoV-NL63 N protein (ab270843) (Abcam, UK), recombinant HCoV-HKU1 N protein (MyBioSource, USA), and HCoV-229E N protein (MyBioSource, USA)) in a total volume of 100 μL per well was used for coating high binding 96-well plates (Thermo Fisher, USA), followed by incubation overnight at 4 °C. The following day, plates were washed three times using 1X Phosphate Buffered Saline (PBS) (Lonza BioWhittaker®, Switzerland) with 0.05% Tween® 20 (Sigma-Aldrich, USA), followed by one wash with 1X PBS. Plates were blocked with 100 μL of blocking solution per well containing 1X PBS, 0.05% Tween® 20 and 10% Fetal Bovine Serum (FBS) (Thermo Fisher, USA) at room temperature for 1 h. Thereafter, the plates were washed three times (as before). Serum aliquots (previously stored at -20 °C) were thawed and diluted in 1X PBS containing 0.05% Tween® 20 and 2.5% FBS. Based on optimization experiments, 1:200 serum dilutions were used for HCoV-229E ELISA assays, while for the other HCoV tests, 1:500 dilutions were used. 50 μL of serum dilution per well were added to the plates and incubated at room temperature for 1 h. After the serum incubation, the plates were washed five times (as before) and incubated at room temperature for 1 h with a goat anti-human IgG antibody HRP conjugate (Sigma-Aldrich, USA) at a dilution of 1:3000 in a solution containing 1X PBS, 0.05% Tween® 20 and 2.5% FBS. Thereafter, the plates were washed five times (as before). To develop the plates, 50 μL of TMB Substrate Reagent Set (BD OptEIA™, USA) was added per well and incubated at room temperature in the dark for 30 min. The reaction was stopped with 50 μL of 2 N H2SO4 per well (Sigma-Aldrich, USA). Finally, the plates were read at 450 nm using a Multiskan™ FC Microplate Photometer (Thermo Fisher, USA). Six serum-free negative controls were included per HCoV assay. A cut-off for positivity for each HCoV was set at 2 SD above the mean OD of 12 pre-pandemic samples (27). Adjusted OD values were then normalized to cut-off which were set as 1.8 (HCoV-OC43), 1.85 (HCoV-NL63), 2.03 (HCoV-HKU1) and 1.89 (HCoV-229E).
SARS-CoV-2 serology
All patient samples from both groups were selected based on previous positive SARS-CoV-2 tests. Selected group 1 patients (n=94) were reactive to SARS-CoV-2 S protein using an in-house ELISA as previously reported (22). Group 2 patients (n=92) were determined SARS-CoV-2 positive by diagnostic RT-qPCR (23). In order to confirm seropositivity against SARS-CoV-2 N protein in both groups, an in-house ELISA was applied (27). Briefly, high binding 96-well plates were coated with 50 μL of N protein (Cape Bio Pharms, Cape Town, South Africa) at a concentration of 2 μg/mL at 4 °C overnight. The following day, plates were washed five times using 1X PBS containing 0.1% Tween® 20, and then incubated in blocking buffer (1% casein, Hammarsten bovine (Sigma-Aldrich, USA), 1% PBS-Tween) at room temperature for 1 h. Thereafter, the blocking buffer was discarded and 100 μL of 1:50 diluted serum samples (in 0.5% casein and 1% Tween® 20 in PBS) were added to the plates and incubated at room temperature for 2 h. Plates were washed five times (as before) and incubated at room temperature for 1 h with a goat anti-human IgG (Fc specific) antibody (1:5000; IgG-HRP; Sigma-Aldrich, USA). To develop the plates, 100 μL of O-phenylenediamine dihydrochloride OPD (Sigma-Aldrich, USA) per well was added at room temperature for 12 min. The reaction was stopped with 50 μL 3 M hydrochloric acid (HCl). Plates were read at 490 nm using a GloMax® microplate reader (Promega, USA). The cut-off for positivity was set at 2 SD above the mean OD of 12 pre-pandemic samples. Adjusted OD values were then normalized to cut-off which was set as 0.067.
Statistical analysis
The seroprevalence (P) of the individual HCoV-229E, HCoV-NL63, HCoV-OC43, HCoV-HKU1 as well as total HCoV, respectively, was expressed as percentage of the entire patient cohort (n = 186), using the following formula, with a 95% confidence interval (CI).
Graphical representations were performed in Prism (v 9.4.1; GraphPad Software Inc., San Diego, CA, USA; https://www.graphpad.com/scientific-software/prism/) and SPSS software version 29 (IBM Corp, New York, NY, USA; https://www.ibm.com/products/spss-statistics). Descriptive statistics was used to manage data associated with the clinical status due to COVID-19 in the two groups, as well as their seropositivity to HCoV. To determine whether seropositivity to HCoV-OC43, HCoV-NL63, HCoV-HKU1 and HCoV-229E was related to the severity or absence of clinical signs present in the two patient groups, the prevalence odds ratio (OR) was used as a risk association measure. OR was calculated through the binomial logistic regression model, with a 95% CI using the SPSS software. Continuous variables were transformed, where appropriate, to approximate normal distributions. The two groups (non-hospitalized versus hospitalized COVID-19 patients) were assessed for association with positive HCoV serology using Chi-square, Fisher’s exact, or Mann–Whitney U test, as appropriate. p-values are two-tailed and were considered significant if <0.05.
Results
Clinical characteristics
The clinical characteristics of the two patient groups according to COVID-19 severity are listed in Table 1 (group 1: asymptomatic with positive SARS-CoV-2 serology; group 2: hospitalized COVID-19 patients). Briefly, 55% of patients were women and 45% men, with a median age of 45 years (range: 20 - 85). Most of the patients (47%) were in the range between 41 and 60 years. Regarding HIV-1 status, 65% of patients were HIV-1 positive (100% in group 1 and 28% in group 2), 95% of whom were on antiretroviral therapy (ART). The median HIV-1 VL was 49 copies/mL (range 1 – 1648565). The median CD4 count was 144 cells/mL (range: 3 – 903). Neither HIV VL nor CD4 count differed between the HIV-infected patients among the two groups. However, immune cell parameters such as white cell count, lymphocyte and monocyte counts significantly differed between both groups indicating severe inflammatory disease in group 2 patients (Table 1).
Seroprevalence of common human coronaviruses
The overall anti-HCoV IgG seroprevalence in the entire patient cohort (n = 186) was 60.8% (n = 113; [95% CI: 53.7 – 67.8]), with 37.1% HCoV-NL63 (n = 69; [95% CI: 30.0 – 44.0]), 30.6% HCoV-229E (n = 57; [95% CI: 24 – 37.3]), 22.6% HCoV-HKU1 (n = 42, [95% CI: 16.6 – 28.6]), and 21.0% HCoV-OC43 (n = 39, [95% CI: 15.1 – 26.8]). 73 patients (39.2%) were found to be HCoV seronegative. The proportion of patients exposed to only one HCoV was 29.0% (n = 54), exposed to two HCoV: 15.6% (n = 29), exposed to three HCoV: 13.4% (n = 25) and exposed to all four HCoV: 2.7% (n = 5). In total, 59 coinfection events (31.7%) were observed. The majority of the HCoV seropositive patients (60.2%; 68/113) as well as the majority of coinfections (69.5%; 41/59) were found in group 1 (asymptomatic, non-hospitalized COVID-19 patients), Table 1 and Figure 1. Interestingly, group 1 patients had a significantly higher prevalence of HCoV-NL63 serology compared to group 2 patients (n=63 in group 1 versus n=6 in group 2; p<0.0001, Table 1) of whom 40% occurred as single infection in group 1 and only 17% in group 2. While HCoV-229E seroprevalence was comparable in both groups, 0% occurred as single infection in group 1 but 52% were found as single-infection in group 2 (Figure 1). Significantly more group 1 patients were infected with HCoV-HKU1 compared to group 2 patients (n=30 in group 1 versus n=12 in group 2, p=0.0027, Table 1); however, the coinfection prevalence of HCoV-HKU1 was comparable in both groups, as was the total and coinfection prevalence of HCoV-OC43 (Figure 1).
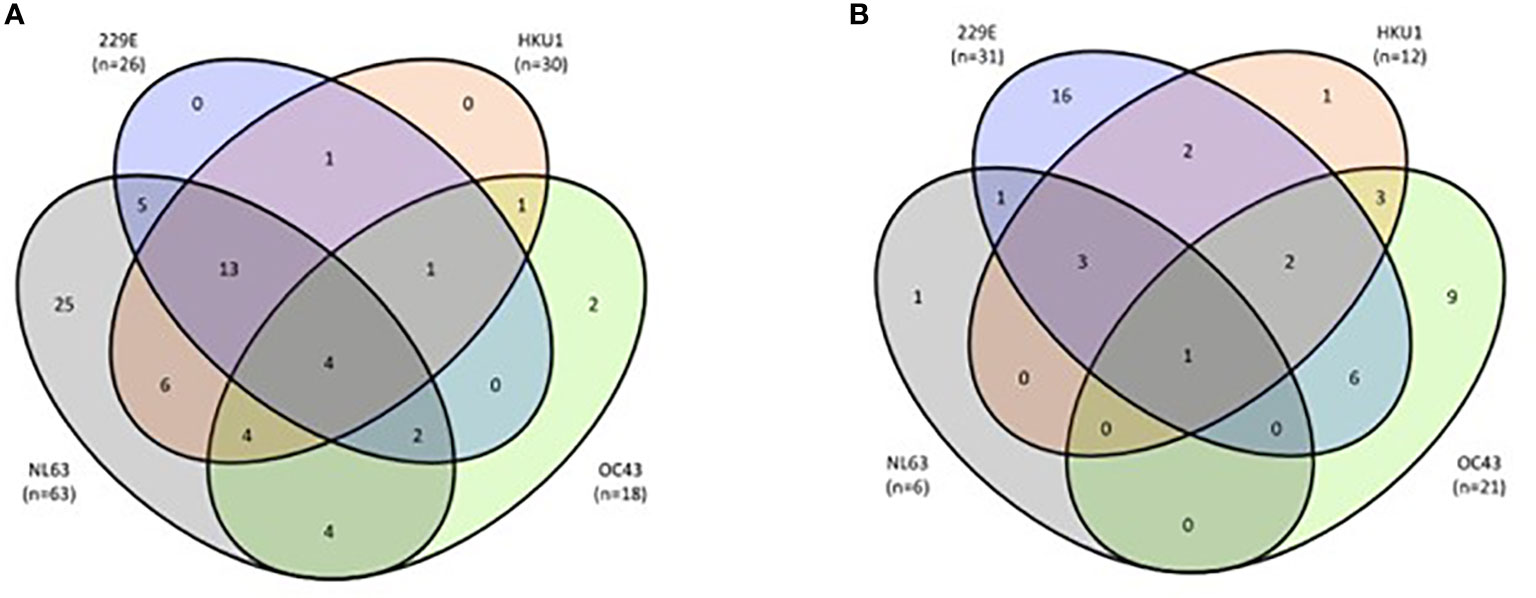
Figure 1 Coinfection frequency between the four HCoV. (A) Group 1 (n=94) asymptomatic patients (total infections: n=68; coinfections: n=41). (B) Group 2 (n=92): hospitalized patients (total infections: n=45 confections: n=18). Grey color indicates HCoV-NL63, purple color indicates HCoV-229E, orange color HCoV-HKU1 and green color HCoV-OC43 seropositivity, respectively.
SARS-CoV-2 and HCoV seropositivity
All patients were previously determined to be SARS-CoV-2 positive by in-house ELISA against the S protein [group 1 (22)] or by diagnostic RT-qPCR [group 2 (23)], respectively. In order to directly compare reactivity against the N protein of all HCoV and SARS-CoV-2, all patient samples were re-tested for seropositivity against SARS-CoV-2 N protein by in-house ELISA. 94.1% (n= 175) were confirmed to be SARS-CoV-2 N protein seropositive (Figure 2A).
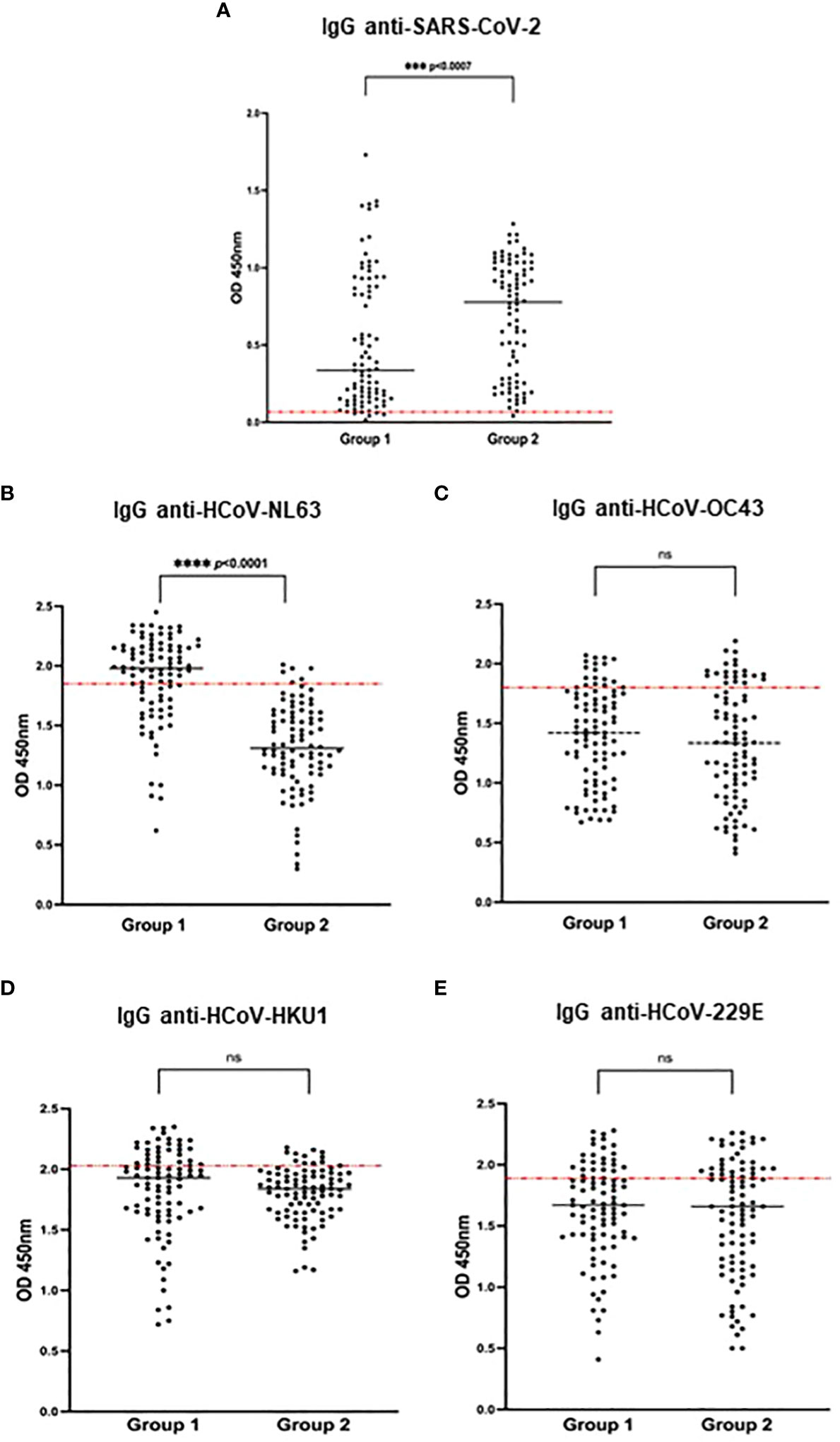
Figure 2 Seroprevalence of SARS-CoV-2 and HCoV in both patient groups (group 1: asymptomatic with positive SARS-CoV-2 serology, group 2: hospitalized COVID-19 patients). SARS-CoV-2 and HCoV N-specific IgG antibodies were detected in the study participants' serum using an in-house ELISA. Results are represented by the OD units. The cut-off was determined by the mean OD + 2SD of 12 pre-pandemic samples and is indicated by the dotted red line in the individual figures. (A) IgG anti-SARS-CoV-2 (cut-off 0.06). (B) IgG anti-HCoV-NL63 (cut-off 1.85). (C) IgG anti-HCoV OC43 (cut-off 1.80) (D) IgG anti-HCoV-HKU1 (cut-off 2.03), (E) IgG anti-HCoV-229E (cut-off 1.89) Significances are indicated by p-values which were considered significant if <0.05. (ns, non-significant; ***p < 0.001, ****p < 0.0001).
The optical density as a measure for relative quantification of N-specific IgG antibodies against HCoV and SARS-CoV-2 is represented in Figure 2. Hospitalized COVID-19 patients (group 2) expectedly showed a significantly higher SARS-CoV-2 antibody titer compared to asymptomatic previously infected SARS-CoV-2 patients (group 1) (Figure 2A); at the same time, group 2 patients presented with a significantly lower titer for HCoV-NL63 (Figure 2B; Table 1), while all other tested HCoV showed no differences in the two patient groups (Figures 2C–E). The median OD for HCoV-NL63 in asymptomatic patients (group 1) was 1.90 (95% CI: 0.62 – 2.45) and 1.32 (95% CI: 0.30 – 2.01) in hospitalized patients (group 2) (p <0.0001). Since the cut-off for determining seropositivity was 1.85, a total of n=63 (67.0%) of group 1 patients was considered positive for HCoV-NL63, while only n=6 (6.6%) of group 2 patients were seropositive (p <0.0001), Figure 2B and Table 1. When stratified by age group, there was a trend to higher HCoV-NL63 seroprevalence in the 20-40 age group compared to the other age groups, but this did not reach statistical significance (Supplementary Table S1). Likewise, no significant differences in HCoV-NL63 titers were found between the different age groups when assessing the entire patient cohort or the individual groups (Supplementary Figure S1, Supplementary Table S1). As mentioned before, most cross-reactive responses in patients have been described to target SARS-CoV-2 N (13); we therefore cannot exclude that there might also be some cross-reactivity in our serological assay. However, we assume this to be minimal as the clear difference seen in SARS-CoV-2 serology between the two patient groups (Figure 2A) was not reflected in the individual HCoV assays (Figures 2B–E).
HCoV-NL63 seroprevalence and HIV-1 status
In order to assess whether HIV infection in group 2 patients might have had a confounding effect on the observed significantly lower HCoV-NL63 seroprevalence compared to group 1 patients (Table 1), we excluded all HIV negative patients from the comparison (Figure 3A). Similar to our previous observations (Figure 2B), we found a significantly lower HCoV-NL63 seroprevalence in HIV-infected group 2 patients compared to all HIV-infected group 1 patients (Figure 3A), p <0.0001. Moreover, when all group 2 patients were stratified according to their HIV status, no statistically significant differences in HCoV-NL63 seroprevalence was detected (Figure 3B). While the majority of samples derived from group 2 patients displayed HCoV-NL63 antibody levels below cut-off, there was a tendency to higher titers in HIV positive individuals (Figure 3B) which was also reflected in the entire group 1 (Figure 3A). These results were also observed when the ART-negative patients from group 2 (n=6) were excluded from the analysis (Supplementary Figure S2).
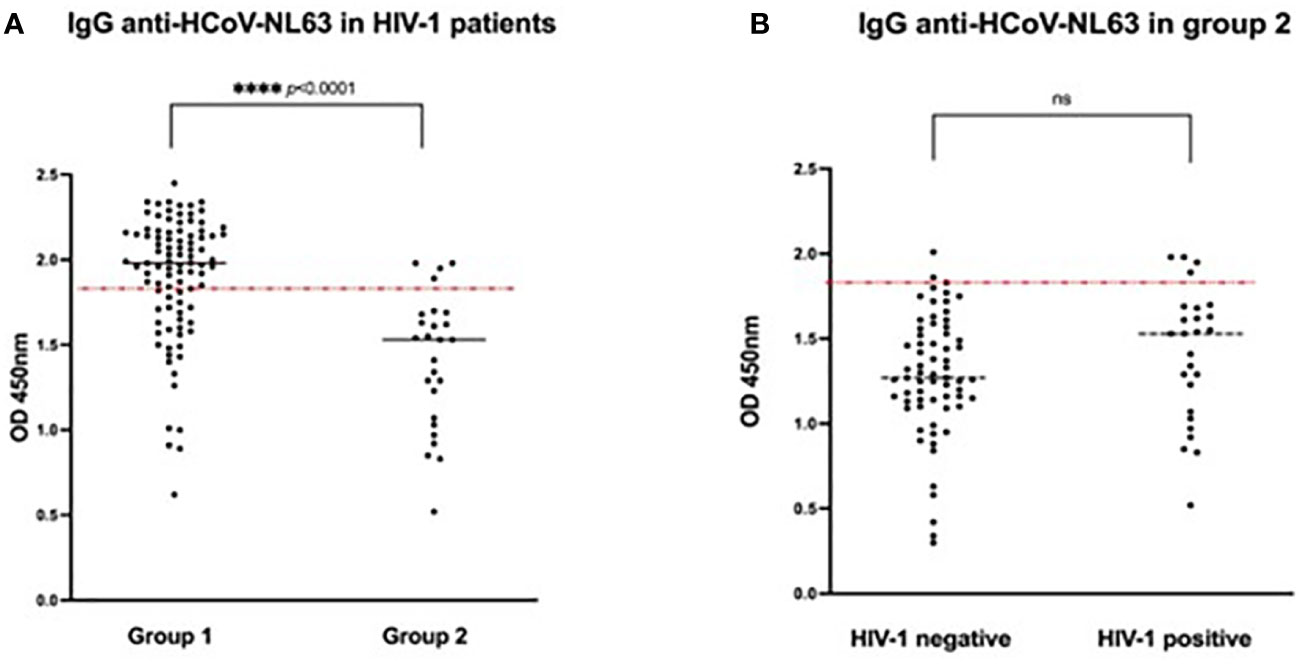
Figure 3 Seroprevalence of HCoV-NL63 in (A) HIV-1 positive patients (n=120) from both patient groups (group 1: asymptomatic with positive SARS-CoV-2 serology, n = 94; group 2 hospitalized COVID-19 patients, n=26) and (B) Group 2 patients (hospitalized COVID-19 patients, n=92) stratified according to their HIV-1 status (HIV-1 negative, n=66, HIV-1 positive, n=26). Results are represented by the Optical Density units. The cut-off was determined by the mean OD + 25 of 12 pre-pandemic samples and is indicated by the dotted line (cut-off 1.85). (ns, non- significant; ****p < 0.0001). Significances are indicated by p-values which were considered significant if <0.05.
Given the relatively small sample size we cannot generally exclude that HIV infection affects HCoV-NL63 antibody levels; however, in the context of this study we found that HIV infection does not impact on the association of pre-exposure to HCoV-NL63 and COVID-19 severity.
Association between HCoV exposure and COVID-19 severity
Assessment of parameters that differed between group 1 (asymptomatic COVID-19 patients) and group 2 (hospitalized COVID-19 patients) indicated that male sex, age range between 40 - 61 years, age range between 61 - 85 years, HCoV status, HCoV coinfection frequency and white cell counts were statistically different between both groups (Table 1); these parameters were therefore considered for logistic regression analysis. In patients exposed to SARS-CoV-2, previous HCoV-NL63 exposure was associated with reduced severity [Table 2, Model A, p <0.001, adjusted OR = 0.0230 (95% CI: 0.0046 – 0.1146)]. On the other hand, previous HCoV-229E exposure was associated with increased severity [Table 2, Model A, p = 0.0052, adjusted OR = 14.4211 (95% CI: 2.2218 – 93.6044)]. Additionally, age range between 40 - 61 years [Table 2, Model A, p <0.001, adjusted OR = 8.7738 (95% CI: 2.9782 – 25.8480)], and age range between 61 - 85 years [Table 2, Model A, p = 0.0015, adjusted OR = 13.6463 (95% CI: 2.7197 – 68.4704)], were as well associated with increased COVID-19 severity.
To avoid overfitting the model, variables that were not significant in model A were removed and a stripped-down logistic regression was run confirming that previous HCoV-NL63 exposure was associated with reduced COVID-19 severity (protection factor) [Table 2, Model B, p <0.001, adjusted OR = 0.0176 (95% CI: 0.0039 – 0.0786)], while previous HCoV-229E exposure [Table 2, Model B, p = 0.0051, adjusted OR = 7.3239 (95% CI: 1.8195–29.4800)], male sex [Table 2, Model B, p = 0.0467, adjusted OR = 2.5242 (95% CI: 1.0136 – 6.2862)], age range between 40 - 61 years [Table 2, Model B, p <0.001, adjusted OR = 7.2237 (95% CI: 2.7511 – 18.9680)] and age range between 61 - 85 years [Table 2, Model B, p = 0.0012, adjusted OR = 12.5092 (95% CI: 2.7022 – 57.9072)] were associated with increased COVID-19 severity (risk factors). The protective association of previous exposure with HCoV-NL63 also remained significant when only adjusted for age [Table 2, Model C, p <0.001, adjusted OR = 0.034 (95% CI: 0.012 – 0.095)]. Interestingly, when this logistic regression was performed for death outcome in group 2 patients (n=26, 28.3%), no significant associations were identified (Supplementary Table S2). Figure 4 shows a summary of the association between previous HCoV exposure and other relevant demographic parameters with COVID-19 severity (Adjusted OR and 95% CI).
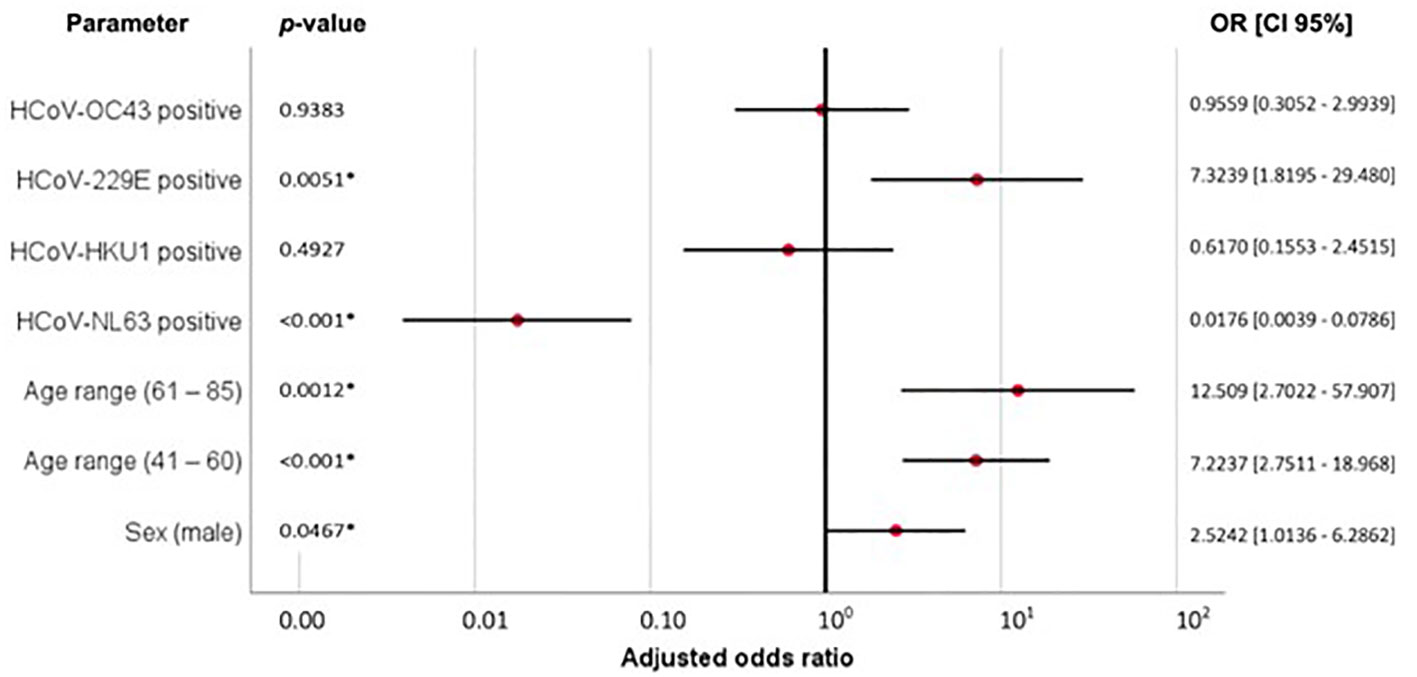
Figure 4 Adjusted ORs and 95% confident intervals of Model B (Table 2) describing the association between previous HCoV exposure, age range (61-85), age range (41-60) and male sex, with COVID-19 severity, p < 0.05 is considered significant and indicated with *.
Discussion
Various hypotheses have been proposed to explain the lower COVID-19 related morbidity and mortality in selected populations, such as underlying host immunity due to prior exposure to cross-reactive viruses (13). Indeed, several studies have been conducted to test the possible cross-protection against COVID-19 severity due to pre-exposure to other common coronaviruses in the global North (10, 28–30) as well as elsewhere in Africa such as Senegal (31); Gabon (31, 32); Ghana, Madagascar and Nigeria (33) and Tanzania and Zambia (13). Most studies identified significant correlations between previous HCoV exposure and protection against COVID-19 severity; however, there are also some examples of no reported associations (34, 35), as well as contradictory studies (36–39). To our knowledge, this is the first report on HCoV seroprevalence in South Africa and its possible association with cross-protection against COVID-19 severity.
In South Africa, clinical studies on HCoV have primarily focused on acute respiratory infections in children (17, 19–21) as HCoV infections are more frequent in the younger age groups. HCoV prevalences in pediatric patients ranged between 0.6% and 8.3%, with HCoV-NL63 being the most commonly detected HCoV in hospitalized patients [2.1% (17)] and in non-hospitalized birth cohorts [3.0% (21)]. Studies on adults are relatively rare; available data indicate a prevalence of 3.7% acute HCoV-229E and < 2% of other HCoV infections (18). While serology data from South Africa were lacking, several studies from other African countries indicate a high seroprevalence of HCoV, such as 48.3% for three HCoV (229E, OC43, NL63) in Ghana (40), 87.5% overall HCoV seroprevalence among influenza patients in Uganda (41) and 92% and 50% seropositivity against HCoV-NL63 and HCoV-229E, respectively, among patients from Tanzania and Zambia (13). In our study from South Africa, the overall HCoV seroprevalence on hospitalized COVID-19 patients and non-hospitalized HIV-infected patients was 60.8%, with HCoV-NL63 being the predominant one (37.1%), followed by HCoV-229E (30.6%), HCoV-HKU1 (22.6%) and HCoV-OC43 (21.0%). Together, these studies demonstrate high seroprevalences of HCoV in Sub-Saharan Africa which support the hypothesis that pre-exposure to common HCoV may be cross-protective and associated with low COVID-19 severity in selected populations on the continent.
Interestingly, COVID-19 has been found to be less severe in children, not only in SSA, and it has been hypothesized that both antibody and T-cell mediated cross-protection from seasonal coronaviruses could explain the relatively low SARS-CoV-2 infection rate and/or COVID-19 morbidity and mortality in children which may last several years (42, 43). Moreover, it was recently reported that adults with exposure to young children showed less severe COVID-19 symptoms compared to adults without exposure (44). These findings strengthen the hypothesis that recent exposure to HCoV may also protect against COVID-19 severity in adults.
In support of the studies conducted in pediatric patient cohorts from the larger Cape Town area mentioned above (17, 21) we also found HCoV-NL63 as the most common of the four HCoV. There are several reports on frequent coinfections of HCoV-NL63 with other HCoV (17, 21, 45) as well as with other respiratory viruses, such as influenza A/H1N1 virus (46), Respiratory Syncytial Virus (RSV) (45, 47), Human Metapneumovirus (hMPV) (45) and Human Bocavirus (HBoV) (19). However, global HCoV seroepidemiology demonstrate wide ranges of prevalences of the individual HCoV: HCoV-NL63 [8.0% (40) - 70.3% (48)]; HCoV-229E [0.8% (49) – 35.1% (48)]; HCoV-HKU1 [6.5% (50) - 25.6% (49)] and HCoV-OC43 [5.8% (50) – 70.8% (49)], which may be explained by the different geographical regions and different methodologies to assess serology.
In addition to assessing HCoV seroprevalence in the larger Cape Town area, South Africa, our study also compared non-hospitalized SARS-CoV-2 seropositive patients (group 1) with patients hospitalized with severe COVID-19 (group 2). We found that the majority of group 1 patients had a previous infection with one or more HCoV. Specifically, antibody titers for HCoV-NL63 were significantly higher in group 1 (asymptomatic) patients (1.90 [95% CI: 0.62 – 2.45]) compared to group 2 (hospitalized) patients (1.32 [95% CI: 0.30 – 2.01]). Moreover, logistic regression analysis revealed a significantly protective effect by previous exposure to HCoV-NL63 [p< 0.001, adjusted OR = 0.0176 (95% CI: 0.0039 – 0.0786)]. These results suggest that previous exposure to HCoV-NL63 might be associated with protection against COVID-19 severity. Moreover, the majority of group 1 patients had multiple infections (43.6%) compared to group 2 patients (19.6%). It is therefore reasonable to speculate that group 2 patients presented with more severe COVID-19 due to their low level of cross-protective immunity elicited by previous HCoV, particularly HCoV-NL63, exposure. We also speculate that the herein studied patients presented a relatively recent HCoV exposure, since the protective immunity time of HCoV antibodies is short-lasting (between 0.9 and 3.8 years) (51, 52).
Other risk factors associated with more severe COVID-19 identified in our study was advanced age [p = 0.0012, adjusted OR = 12.5092 (95% CI: 2.7022 – 57.9072)] and male sex [p = 0.0467, adjusted OR = 2.5242 (95% CI: 1.0136 – 6.2862)], as previously reported (2), while HIV infection did not have a confounding effect on HCoV serology (and thereby potential protective associations) in our study, perhaps due to the majority of the HIV-infected patients being well controlled on ART. This is in contrast to a recent report showing HIV-infected individuals having lower cross-reactive responses towards SARS-CoV-2 (13).
Heterologous immunity can protect but also compromise an individual’s response to another related antigen via the Original Antigenic Sin (OAS) theory which entails antibody-mediated increased cellular virus entry and intensified inflammation against a secondary infection (53, 54). Interestingly, we found that previous HCoV-229E exposure was associated with increased COVID-19 severity when stratified for age, sex and individual HCoV infection [p = 0.0051, adjusted OR = 7.3239 (95% CI: 1.8195–29.4800)], despite both patient groups displaying comparable infection rates and titers. Indeed, some contradictory findings to the cross-protective hypothesis have been reported. For example, cross-reactive antibodies against HCoV-OC43 S protein were found to correlate with disease severity in elderly COVID-19 patients (39), and fatal COVID-19 outcomes were found to be associated with an antibody response targeting epitopes shared with HCoV (37). Moreover, pre-existing humoral immunity to HCoV were reported to negatively impact the protective SARS-CoV-2 antibody response (38), while SARS-CoV-2-specific humoral immunity in convalescent patients with mild COVID-19 was found to be supported by CD4+ T-cells and negatively correlated with HCoV-229E and HCoV-NL63 specific antibody titers (36). However, these reports should be interpreted in the context of the individual study design, methodology and geographical region. The vast majority of reports suggest protective cross-reactive impacts of previous exposure to HCoV.
There are some limitations to our study which may affect generalizability, such as the relatively low number of patients as well as the retrospective study design analyzing two non-synchronously recruited groups of patients from two different sites in the larger Cape Town area. While the first COVID-19 wave in South Africa was caused by the ancestral SARS-CoV-2 strain (group 2 patients), the second wave was dominated by the Beta variant (group 1 patients), but no difference in mortality was reported when both waves were compared (55). Differences in the variants mainly affected the S protein rather than the N protein (56) which has been reported to be targeted by most cross-reactive responses (13); therefore cross-reactivity due to previous exposure to HCoV may be comparable between the two patient groups. Moreover, due to lack of commercially available serology tests for the individual HCoV, we set up in-house ELISA assays to test convalescent patient plasma for IgG antibodies recognizing recombinant N. Although we did not have positive controls available for all tested individual HCoV, we assessed all patient samples at the same time and applied stringent cut-off values which allowed us to directly compare both clinical groups.
In conclusion, we assessed pandemic patient samples and found a significant association of presence and level of HCoV-NL63 antibodies with protection against adverse clinical COVID-19 outcome. While we only determined humoral immune responses, both pre-existing antibodies and pre-existing memory T cell responses against previous HCoV exposure may offer cross-protection against COVID-19 disease, if not SARS-CoV-2 infection.
Data availability statement
The original contributions presented in the study are included in the article/Supplementary Material. Further inquiries can be directed to the corresponding authors.
Ethics statement
The studies involving human participants were reviewed and approved by Human Research Ethical Committee (HREC), University of Cape Town. The patients/participants provided their written informed consent to participate in this study.
Author contributions
Conceptualization, LL-R, DJ and GS. Methodology, LL-R, HL, AC and GS. Formal analysis, LL-R, HL, DJ and GS. Writing—original draft preparation, LL-R and GS. Writing—review and editing, LL-R, DJ, HL, AC, CR, RW, WJ, LJ, CO and GS. Supervision, GS. All authors contributed to the article and approved the submitted version.
Funding
This work was supported by the International Center for Genetic Engineering and Biotechnology (ICGEB) and the United Nations Office for South South Cooperation (UNOSSC) – Youth4South initiative, who awarded LL-R with an EMPOWER Fellowship: Programme under the South-South and Triangular Cooperation for women scientists from developing countries. This research was also funded by the European and Developing Countries Clinical Trials Partnership EDCTP2 program supported by the European Union (EU)s Horizon 2020 program (Training and Mobility Action TMA2018SF-2446-KSHV/HIV morbidity), the National Research Foundation (NRF) South Africa (grant number 142089), the South African Medical Research Council (MRC) and the Poliomyelitis Research Foundation (PRF). RW is supported by the Francis Crick Institute which is funded by Cancer Research UK (FC0010218), Medical Research Council (FC0010218) and Wellcome (FC0010218). He also received funding from Wellcome (203135, 222754). For the purpose of open access the author has applied a CC-BY public copyright to any author-accepted manuscript arising from this submission.
Acknowledgments
The authors are grateful for the support of ICGEB, UNOSSC office and Youth4South Programme. The authors thank the study participants and the clinical staff and personnel at the clinical sites for their support and dedication.
Conflict of interest
The authors declare that the research was conducted in the absence of any commercial or financial relationships that could be construed as a potential conflict of interest.
Publisher’s note
All claims expressed in this article are solely those of the authors and do not necessarily represent those of their affiliated organizations, or those of the publisher, the editors and the reviewers. Any product that may be evaluated in this article, or claim that may be made by its manufacturer, is not guaranteed or endorsed by the publisher.
Supplementary material
The Supplementary Material for this article can be found online at: https://www.frontiersin.org/articles/10.3389/fviro.2023.1125448/full#supplementary-material
References
1. WHO. State of inequality: Hiv, tuberculosis and malaria (2021). Geneva: World Health Organization (Accessed January 13, 2023).
2. Jassat W, Cohen C, Tempia S, Masha M, Goldstein S, Kufa T, et al. Risk factors for covid-19-Related in-hospital mortality in a high hiv and tuberculosis prevalence setting in south Africa: A cohort study. Lancet HIV (2021) 8(9):e554–e67. doi: 10.1016/S2352-3018(21)00151-X
3. WHO. Global clinical platform for covid-19, clinical features and prognostic factors of covid-19 in people living with hiv hospitalized with suspected or confirmed sars-Cov-2 infection (2021) (Accessed March 03, 2022).
4. Alosaimi B, Naeem A, Hamed ME, Alkadi HS, Alanazi T, Al Rehily SS, et al. Influenza Co-infection associated with severity and mortality in covid-19 patients. Virol J (2021) 18(1):127. doi: 10.1186/s12985-021-01594-0
5. Blumenthal MJ, Lambarey H, Chetram A, Riou C, Wilkinson RJ, Schäfer G. Kaposi’s sarcoma-associated herpesvirus, but not Epstein-Barr virus, Co-infection associates with coronavirus disease 2019 severity and outcome in south African patients. Front Microbiol (2021) 12:795555. doi: 10.3389/fmicb.2021.795555
6. Xie Y, Cao S, Dong H, Lv H, Teng X, Zhang J, et al. Clinical characteristics and outcomes of critically ill patients with acute covid-19 with Epstein-Barr virus reactivation. BMC Infect Dis (2021) 21(1):955. doi: 10.1186/s12879-021-06638-y
7. Seyedjavadi SS, Bagheri P, Nasiri MJ, Razzaghi-Abyaneh M, Goudarzi M. Fungal infection in Co-infected patients with covid-19: An overview of case Reports/Case series and systematic review. Front Microbiol (2022) 13:888452. doi: 10.3389/fmicb.2022.888452
8. Lai CC, Wang CY, Hsueh PR. Co-Infections among patients with covid-19: The need for combination therapy with non-Anti-Sars-Cov-2 agents? J microbiol immunol infect = Wei mian yu gan ran za zhi (2020) 53(4):505–12. doi: 10.1016/j.jmii.2020.05.013
9. Gaye B, Khoury S, Cene CW, Kingue S, N’Guetta R, Lassale C, et al. Socio-demographic and epidemiological consideration of africa’s covid-19 response: What is the possible pandemic course? Nat Med (2020) 26(7):996–9. doi: 10.1038/s41591-020-0960-y
10. Sagar M, Reifler K, Rossi M, Miller NS, Sinha P, White LF, et al. Recent endemic coronavirus infection is associated with less-severe covid-19. J Clin Invest (2021) 131(1):1–5. doi: 10.1172/JCI143380
11. Grifoni A, Weiskopf D, Ramirez SI, Mateus J, Dan JM, Moderbacher CR, et al. Targets of T cell responses to sars-Cov-2 coronavirus in humans with covid-19 disease and unexposed individuals. Cell (2020) 181(7):1489–501.e15. doi: 10.1016/j.cell.2020.05.015
12. Mateus J, Grifoni A, Tarke A, Sidney J, Ramirez SI, Dan JM, et al. Selective and cross-reactive sars-Cov-2 T cell epitopes in unexposed humans. Science (2020) 370(6512):89–94. doi: 10.1126/science.abd3871
13. Tso FY, Lidenge SJ, Peña PB, Clegg AA, Ngowi JR, Mwaiselage J, et al. High prevalence of pre-existing serological cross-reactivity against severe acute respiratory syndrome coronavirus-2 (Sars-Cov-2) in Sub-Saharan Africa. Int J Infect diseases: IJID: Off Publ Int Soc Infect Dis (2021) 102:577–83. doi: 10.1016/j.ijid.2020.10.104
14. Chen B, Tian E-K, He B, Tian L, Han R, Wang S, et al. Overview of lethal human coronaviruses. Signal Transduction Targeted Ther (2020) 5(1):89. doi: 10.1038/s41392-020-0190-2
15. Kesheh MM, Hosseini P, Soltani S, Zandi M. An overview on the seven pathogenic human coronaviruses. Rev Med Virol (2022) 32(2):e2282. doi: 10.1002/rmv.2282
16. Cicaloni V, Costanti F, Pasqui A, Bianchini M, Niccolai N, Bongini P. A bioinformatics approach to investigate structural and non-structural proteins in human coronaviruses. Front Genet (2022) 13:891418. doi: 10.3389/fgene.2022.891418
17. Venter M, Lassaunière R, Kresfelder TL, Westerberg Y, Visser A. Contribution of common and recently described respiratory viruses to annual hospitalizations in children in south Africa. J Med Virol (2011) 83(8):1458–68. doi: 10.1002/jmv.22120
18. Subramoney K, Hellferscee O, Pretorius M, Tempia S, McMorrow M, von Gottberg A, et al. Human bocavirus, coronavirus, and polyomavirus detected among patients hospitalised with severe acute respiratory illness in south Africa, 2012 to 2013. Health Sci Rep (2018) 1(8):e59. doi: 10.1002/hsr2.59
19. Smuts H, Workman L, Zar HJ. Role of human metapneumovirus, human coronavirus Nl63 and human bocavirus in infants and young children with acute wheezing. J Med Virol (2008) 80(5):906–12. doi: 10.1002/jmv.21135
20. Smuts H. Human coronavirus Nl63 infections in infants hospitalised with acute respiratory tract infections in south Africa. Influenza Other Respir Viruses (2008) 2(4):135–8. doi: 10.1111/j.1750-2659.2008.00049.x
21. Nicol MP, MacGinty R, Workman L, Stadler JAM, Myer L, Allen V, et al. A longitudinal study of the epidemiology of seasonal coronaviruses in an African birth cohort. J Pediatr Infect Dis Soc (2021) 10(5):607–14. doi: 10.1093/jpids/piaa168
22. Lambarey H, Blumenthal MJ, Chetram A, Joyimbana W, Jennings L, Tincho MB, et al. Sars-Cov-2 infection is associated with uncontrolled hiv viral load in non-hospitalized hiv-infected patients from gugulethu, south Africa. Viruses (2022) 14(6):1–18. doi: 10.3390/v14061222
23. Riou C, du Bruyn E, Stek C, Daroowala R, Goliath RT, Abrahams F, et al. Relationship of Sars-Cov-2–specific Cd4 response to covid-19 severity and impact of hiv-1 and tuberculosis coinfection. J Clin Invest (2021) 131(12):1–15. doi: 10.1172/JCI149125
24. Harris PA, Taylor R, Thielke R, Payne J, Gonzalez N, Conde JG. Research electronic data capture (Redcap)–a metadata-driven methodology and workflow process for providing translational research informatics support. J Biomed Inf (2009) 42(2):377–81. doi: 10.1016/j.jbi.2008.08.010
25. Haynes LM, Miao C, Harcourt JL, Montgomery JM, Le MQ, Dryga SA, et al. Recombinant protein-based assays for detection of antibodies to severe acute respiratory syndrome coronavirus spike and nucleocapsid proteins. Clin Vaccine Immunol (2007) 14(3):331–3. doi: 10.1128/CVI.00351-06
26. Dijkman R, Jebbink MF, Idrissi NBE, Pyrc K, Müller MA, Kuijpers TW, et al. Human coronavirus Nl63 and 229e seroconversion in children. J Clin Microbiol (2008) 46(7):2368–73. doi: 10.1128/JCM.00533-08
27. Makatsa MS, Tincho MB, Wendoh JM, Ismail SD, Nesamari R, Pera F, et al. Sars-Cov-2 antigens expressed in plants detect antibody responses in covid-19 patients. Front Plant Sci (2021) 12:589940. doi: 10.3389/fpls.2021.589940
28. Gouma S, Weirick ME, Bolton MJ, Arevalo CP, Goodwin EC, Anderson EM, et al. Health care worker seromonitoring reveals complex relationships between common coronavirus antibodies and covid-19 symptom duration. JCI Insight (2021) 6(16):1–9. doi: 10.1172/jci.insight.150449
29. Dugas M, Grote-Westrick T, Merle U, Fontenay M, Kremer AE, Hanses F, et al. Lack of antibodies against seasonal coronavirus Oc43 nucleocapsid protein identifies patients at risk of critical covid-19. J Clin Virol (2021) 139:104847. doi: 10.1016/j.jcv.2021.104847
30. Lavell AHA, Sikkens JJ, Edridge AWD, van der Straten K, Sechan F, Oomen M, et al. Recent infection with hcov-Oc43 may be associated with protection against sars-Cov-2 infection. iScience (2022) 25(10):105105. doi: 10.1016/j.isci.2022.105105
31. Pedersen J, Koumakpayi IH, Babuadze G, Baz M, Ndiaye O, Faye O, et al. Cross-reactive immunity against sars-Cov-2 n protein in central and West Africa precedes the covid-19 pandemic. Sci Rep (2022) 12(1):12962. doi: 10.1038/s41598-022-17241-9
32. Mveang Nzoghe A, Essone PN, Leboueny M, Maloupazoa Siawaya AC, Bongho EC, Mvoundza Ndjindji O, et al. Evidence and implications of pre-existing humoral cross-reactive immunity to sars-Cov-2. Immunity Inflammation Dis (2021) 9(1):128–33. doi: 10.1002/iid3.367
33. Emmerich P, Murawski C, Ehmen C, von Possel R, Pekarek N, Oestereich L, et al. Limited specificity of commercially available sars-Cov-2 igg elisas in serum samples of African origin. Trop Med Int Health (2021) 26(6):621–31. doi: 10.1111/tmi.13569
34. Imai K, Matsuoka M, Tabata S, Kitagawa Y, Nagura-Ikeda M, Kubota K, et al. Cross-reactive humoral immune responses against seasonal human coronaviruses in covid-19 patients with different disease severities. Int J Infect diseases: IJID: Off Publ Int Soc Infect Dis (2021) 111:68–75. doi: 10.1016/j.ijid.2021.08.026
35. Anderson EM, Goodwin EC, Verma A, Arevalo CP, Bolton MJ, Weirick ME, et al. Seasonal human coronavirus antibodies are boosted upon sars-Cov-2 infection but not associated with protection. Cell (2021) 184(7):1858–64.e10. doi: 10.1016/j.cell.2021.02.010
36. Odendahl M, Endler I, Haubold B, Rodionov RN, Bornstein SR, Tonn T. Sars-Cov-2-Specicific humoral immunity in convalescent patients with mild covid-19 is supported by Cd4+ T-cell help and negatively correlated with alphacoronavirus-specific antibody titer. Immunol Lett (2022) 251-252:38–46. doi: 10.1016/j.imlet.2022.09.007
37. McNaughton AL, Paton RS, Edmans M, Youngs J, Wellens J, Phalora P, et al. Fatal covid-19 outcomes are associated with an antibody response targeting epitopes shared with endemic coronaviruses. JCI Insight (2022) 7(13):1–17. doi: 10.1172/jci.insight.156372
38. Lin C-Y, Wolf J, Brice DC, Sun Y, Locke M, Cherry S, et al. Pre-existing humoral immunity to human common cold coronaviruses negatively impacts the protective sars-Cov-2 antibody response. Cell Host Microbe (2022) 30(1):83–96.e4. doi: 10.1016/j.chom.2021.12.005
39. Guo L, Wang Y, Kang L, Hu Y, Wang L, Zhong J, et al. Cross-reactive antibody against human coronavirus Oc43 spike protein correlates with disease severity in covid-19 patients: A retrospective study. Emerging Microbes Infect (2021) 10(1):664–76. doi: 10.1080/22221751.2021.1905488
40. Owusu M, Sylverken AA, El-Duah P, Acheampong G, Mutocheluh M, Adu-Sarkodie Y. Sero-epidemiology of human coronaviruses in three rural communities in Ghana. Pan Afr Med J (2021) 38:244. doi: 10.11604/pamj.2021.38.244.26110
41. Mulabbi EN, Tweyongyere R, Wabwire-Mangen F, Mworozi E, Koehlerb J, Kibuuka H, et al. Seroprevalence of human coronaviruses among patients visiting hospital-based sentinel sites in Uganda. BMC Infect Dis (2021) 21(1):585. doi: 10.1186/s12879-021-06258-6
42. Fraley E, LeMaster C, Banerjee D, Khanal S, Selvarangan R, Bradley T. Cross-reactive antibody immunity against sars-Cov-2 in children and adults. Cell Mol Immunol (2021) 18(7):1826–8. doi: 10.1038/s41423-021-00700-0
43. Waterlow NR, van Leeuwen E, Davies NG, Flasche S, Eggo RM. How immunity from and interaction with seasonal coronaviruses can shape sars-Cov-2 epidemiology. Proc Natl Acad Sci (2021) 118(49):e2108395118. doi: 10.1073/pnas.2108395118
44. Solomon MD, Escobar GJ, Lu Y, Schlessinger D, Steinman JB, Steinman L, et al. Risk of severe covid-19 infection among adults with prior exposure to children. Proc Natl Acad Sci (2022) 119(33):e2204141119. doi: 10.1073/pnas.2204141119
45. Canducci F, Debiaggi M, Sampaolo M, Marinozzi MC, Berrè S, Terulla C, et al. Two-year prospective study of single infections and Co-infections by respiratory syncytial virus and viruses identified recently in infants with acute respiratory disease. J Med Virol (2008) 80(4):716–23. doi: 10.1002/jmv.21108
46. Huang SH, Su MC, Tien N, Huang CJ, Lan YC, Lin CS, et al. Epidemiology of human coronavirus Nl63 infection among hospitalized patients with pneumonia in Taiwan. J microbiol immunol infect = Wei mian yu gan ran za zhi (2017) 50(6):763–70. doi: 10.1016/j.jmii.2015.10.008
47. van der Hoek L, Sure K, Ihorst G, Stang A, Pyrc K, Jebbink MF, et al. Croup is associated with the novel coronavirus Nl63. PloS Med (2005) 2(8):e240. doi: 10.1371/journal.pmed.0020240
48. Shao X, Guo X, Esper F, Weibel C, Kahn JS. Seroepidemiology of group I human coronaviruses in children. J Clin Virol (2007) 40(3):207–13. doi: 10.1016/j.jcv.2007.08.007
49. Gao X, Zhou H, Wu C, Xiao Y, Ren L, Paranhos-Baccalà G, et al. Antibody against nucleocapsid protein predicts susceptibility to human coronavirus infection. J Infecti (2015) 71(5):599–602. doi: 10.1016/j.jinf.2015.07.002
50. Khan T, Rahman M, Ali FA, Huang SSY, Ata M, Zhang Q, et al. Distinct antibody repertoires against endemic human coronaviruses in children and adults. JCI Insight (2021) 6(4):1–15. doi: 10.1172/jci.insight.144499
51. Rees E, Waterlow N, Centre for the Mathematical Modelling of Infectious Diseases COVID-19 Working Group, Lowe R, Kucharski A. Estimating the duration of seropositivity of human seasonal coronaviruses using seroprevalence studies [Version 3; peer review: 3 approved]. Wellcome Open Res (2021) 6(138):1–33. doi: 10.12688/wellcomeopenres.16701.3
52. Edridge AWD, Kaczorowska J, Hoste ACR, Bakker M, Klein M, Loens K, et al. Seasonal coronavirus protective immunity is short-lasting. Nat Med (2020) 26(11):1691–3. doi: 10.1038/s41591-020-1083-1
53. Vatti A, Monsalve DM, Pacheco Y, Chang C, Anaya J-M, Gershwin ME. Original antigenic sin: A comprehensive review. J Autoimmun (2017) 83:12–21. doi: 10.1016/j.jaut.2017.04.008
54. Steiner S, Sotzny F, Bauer S, Na IK, Schmueck-Henneresse M, Corman VM, et al. Hcov- and sars-Cov-2 cross-reactive T cells in cvid patients. Front Immunol (2020) 11:607918. doi: 10.3389/fimmu.2020.607918
55. Lalla U, Koegelenberg CFN, Allwood BW, Sigwadhi LN, Irusen EM, Zemlin AE, et al. Comparison of patients with severe covid-19 admitted to an intensive care unit in south Africa during the first and second wave of the covid-19 pandemic. Afr J Thorac Crit Care Med (2021) 27(4):156–62. doi: 10.7196/AJTCCM.2021.v27i4.185
Keywords: SARS-CoV-2, serology, HCoV-NL63, HCoV-229E, HCoV-OC43, HCoV-HKU1, COVID-19
Citation: Lesmes-Rodríguez LC, Lambarey H, Chetram A, Riou C, Wilkinson RJ, Joyimbana W, Jennings L, Orrell C, Jaramillo-Hernández DA and Schäfer G (2023) Previous exposure to common coronavirus HCoV-NL63 is associated with reduced COVID-19 severity in patients from Cape Town, South Africa. Front. Virol. 3:1125448. doi: 10.3389/fviro.2023.1125448
Received: 16 December 2022; Accepted: 26 January 2023;
Published: 08 February 2023.
Edited by:
Perumal Arumugam Desingu, Indian Institute of Science (IISc), IndiaReviewed by:
Ria Lassauniere, Statens Serum Institut (SSI), DenmarkKenji Maeda, Kagoshima University, Japan
Copyright © 2023 Lesmes-Rodríguez, Lambarey, Chetram, Riou, Wilkinson, Joyimbana, Jennings, Orrell, Jaramillo-Hernández and Schäfer. This is an open-access article distributed under the terms of the Creative Commons Attribution License (CC BY). The use, distribution or reproduction in other forums is permitted, provided the original author(s) and the copyright owner(s) are credited and that the original publication in this journal is cited, in accordance with accepted academic practice. No use, distribution or reproduction is permitted which does not comply with these terms.
*Correspondence: Lida C. Lesmes-Rodríguez, llesmes@unillanos.edu.co; Georgia Schäfer, georgia.schafer@icgeb.org