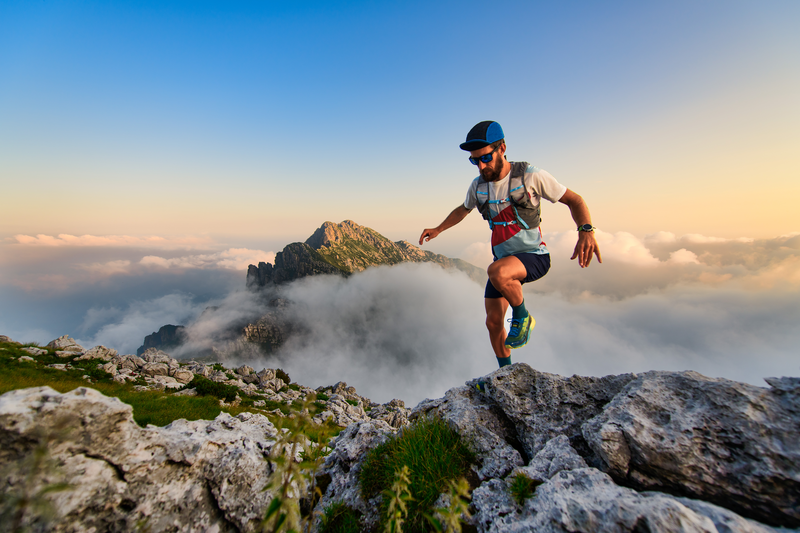
94% of researchers rate our articles as excellent or good
Learn more about the work of our research integrity team to safeguard the quality of each article we publish.
Find out more
BRIEF RESEARCH REPORT article
Front. Virol. , 13 July 2022
Sec. Antivirals and Vaccines
Volume 2 - 2022 | https://doi.org/10.3389/fviro.2022.956113
This article is part of the Research Topic Emerging and Re-emerging Viral Diseases View all 37 articles
The ongoing pandemic of coronavirus disease 2019 (COVID-19) caused by severe acute respiratory syndrome coronavirus 2 (SARS-CoV-2) urgently needs effective antivirals. After over 2 years since the beginning of the pandemic, only a few FDA approved therapeutic options are available to treat the population. Combination therapies have become a standard for the treatment of other infectious diseases such as HIV and hepatitis C due to their improved efficacy compared to monotherapy, reduced toxicity, the ability to prevent the development of resistant viral strains and their potential to treat co-infection. The interest in identifying molecules displaying bioactivity against SARS-CoV-2 has led to extensive search for promising molecules from the natural pharmacopoeia and polyphenols have been shown to display antiviral activity against a number of viruses including SARS-CoV-2. Here we evaluated the in vitro efficacy of two polyphenols, Epigallocatechin gallate (EGCG) and Isoquercetin, in combination with Remdesivir, the first-approved drug for the treatment of severe COVID-19. We confirmed the inhibitory effects of EGCG and isoquercetin against SARS-CoV-2 and demonstrated their strong antiviral synergistic effects with Remdesivir in vitro. These combinational therapies represent an interesting avenue for the treatment of COVID-19 and grant further studies.
The Severe Acute Respiratory Syndrome coronavirus 2 (SARS-CoV-2) outbreak is causing an unprecedented global pandemic that has led to an unparalleled worldwide public health emergency. As of June 2022, the scale of the coronavirus disease 2019 (COVID-19) pandemic, with over 544 million cases and 6.3 million deaths globally over a 2-year period (1) exceeds the 1968 influenza A/H3N2 pandemic, which reported ~1 million deaths worldwide. Patients with COVID-19 can develop pneumonia (2), severe symptoms of acute respiratory distress (ARDS), and multiple organ failure (3). This RNA virus is related to SARS-CoV-1, which emerged in 2002-03 in China, but has distinct features provoking specific morbid symptoms. Infected persons display strong respiratory syndrome including fever, cough, shortness of breath and dyspnea. Severe symptoms will lead to pneumonia and are associated with a major inflammatory crisis in the lungs which leads to improper alveolar pulmonary function and poor gas exchange and blood oxygenation. The disease can also lead to kidney failure and death. Increasing evidence shows that immune patterns are closely associated with the disease progression of patients infected with SARS-CoV-2, where the altered responses are due to both replication in different organs as well as the exacerbation of the inflammatory reactions. This outcome is even more important in high-risk individuals and elderly people (4).
While there are currently multiple effective vaccines, there is still a considerable amount of the global population which has not yet received any dose of COVID-19 vaccine due to vaccine hesitancy and public mistrust, no equitable access to vaccines in many countries, or not yet eligible for vaccination. In addition, immunocompromised individuals can have reduced responses to vaccines, due to their weak immune system. Therefore, the combination of these events is promoting the emergence of viral variants that are increasingly transmissible and are evolving to escape human immunity, as it is the case with omicron variant and its different sub-lineages (5). There is, thus, an immediate unmet need for antivirals that can be rapidly used to treat COVID-19 patients in the unvaccinated, the immunocompromised, and those infected with virus variants that escape human immunity.
COVID-19 antiviral therapy plays a pivotal role in limiting the burden of diseases, preventing infection and minimizing household or community transmission, reducing long-term sequelae of the disease, as well as reducing hospitalization, thus, having an important impact on public health. Very few therapeutic options are available for the treatment of COVID-19 patients, including monoclonal antibodies, which are in general very expensive, and recently, two antivirals, Paxlovid and Molnupiravir, have been authorized in the US. However, these antivirals have significant caveats, including low supply and use only among patients at high risk of severe illness and death.
Remdesivir (GS-5734) is an inhibitor of the viral RNA-dependent RNA polymerase with in vitro inhibitory activity against SARS-CoV-2 and other coronaviruses such as SARS-CoV-1 and the Middle East respiratory syndrome (MERS-CoV) (6, 7). Remdesivir has been granted emergency or conditional authorization in the U.S., Europe, Australia, Singapore, Japan and Canada for people with severe symptoms (8) as a promising treatment option to reduce hospitalization by an average of 4 days (9). The current available Remdesivir requires intravenous administration, however, an orally bioavailable nucleoside prodrug (GS-621763) has been designed for optimal delivery of the parent nucleoside (GS-441524) into the systemic circulation and is being evaluated in in vitro and in vivo models (10).
In the context of the actual pandemic, it is urgent to revisit the arsenal of already developed synthetic drugs and natural molecules from the traditional pharmacopoeia with potential action against the virus. The latter category appears promising to fight viruses and especially SARS-CoV-2. In particular, polyphenols have been shown to display antiviral activity against a number of viruses such as influenza (11–14), Zika (15), Ebola (16), Dengue (17, 18), hepatitis (19), HIV (20, 21) and many others (22–24). As a matter of fact, flavonoids like epigallocatechin gallate (EGCG), present in green tea, display strong antiviral activity against many human viruses including HIV type I, Human T-cell lymphotrophic virus type I, Hepatitis B and C viruses, Herpes types 1 and 2, Epstein-Barr virus, Human Papilloma virus, Influenza virus, rotavirus, and several enteroviruses (25–33). The flavanone hesperidin, present in citruses, has also been shown to inhibit the replication of influenza virus type A (H1N1) (34, 35), and rotaviruses (29), yet, not as efficiently as EGCG. Oligognol™, a catechin oligomer (proanthocyanidin) extract found in the litchi exocarp, blocks the fixation of influenza A viruses to its receptor on Madin-Darby Canine Kidney cells and suppresses the nuclear transport of viral ribonucleoprotein (RNP) (36). Finally, quercetin glycosides (i.e. quercetin-3-β-O-d-glucoside or Isoquercetin, quercetin-4-o-β-glucoside, rutin, quercetin-sophoroside) present in apples, onions, and many Brassicas have been shown to prevent Ebola infection in Vero cells and in murine models (16). Quercetin, also prevents the replication of Zika virus (37), Dengue (38) and influenza A/H1N1 viruses (39).
The renewed interest in identifying molecules displaying bioactivity against SARS-CoV-2 has led to extensive search for promising molecules from the natural pharmacopoeia. Using computational molecular docking and in silico binding affinity, candidate molecules targeting SARS-CoV-2 proteins (PLpro, 3CLpro, RNA-dependent RNA polymerase (RdRp), helicase, Spike) have been tested for their potential use for inhibiting replication of this virus (40–44). Using this strategy, many molecules belonging to the flavonoid family, like EGCG, quercetin, hesperidin, chrysin and baicalain, all flavonoids, have shown potential (45), thus warranting further in vitro testing.
Based on this preliminary information, we investigated the in vitro antiviral activity of selected polyphenols against SARS-CoV-2 and we provide evidence for their synergistic effect when used concomitantly with Remdesivir, the first-approved drug for the treatment of severe COVID-19 (8).
To assess the ability of polyphenols to inhibit SARS-CoV-2 replication in vitro, we used Vero E6 cells (ATCC® CRL-1586™) maintained at 37°C, 5% of CO2 in Minimum Essential Medium (Gibco, Paisley, UK) supplemented with HEPES (Wisent, Québec, CA) and 10% of fetal bovine serum (Cytiva, Utah, USA).
The wild-type virus SARS-CoV-2/Quebec City/21697/2020 used for the experiments was recovered from a patient in April 2020 and passaged twice in Vero E6 cells to produce a stock of the virus. All manipulations using SARS-CoV-2 were carried out at the Containment Level 3 (CL3) laboratory at the CHU de Québec-Université Laval Research Center.
EGCG and Isoquercetin (Quercetin-3-O-glucopyranoside) were obtained from Sigma-Aldrich (St Louis. MO, USA) and 10 mM stock solution were prepared in DMSO. Remdesivir was purchased from MedChem Express (Princeton, NJ, USA) and 100 mM stock solution was prepared in DMSO.
Vero E6 cells (30000 cells per well) were cultured in 96-well plates. One day later, cells were infected with 200 Median Tissue Culture Infectious Dose (TCID50) of SARS-CoV-2 per well. After 1 hour of incubation at 37°C in a 5% of CO2 atmosphere, antivirals in two-fold serial dilutions were added with final concentrations ranging from 15.6 μM to 125 μM for EGCG, 7.8 μM to 62.5 μM for Isoquercetin and 1.25 μM to 20 μM for Remdesivir. Three days later, cell viability was evaluated using 3-(4,5-dimethylthiazol-2-yl)-5-(3-carboxymethoxyphenyl)-2-(4-sul-fophenyl)-2H-tetrazolium (MTS) (CellTiter 96® AQueous One Solution Cell Proliferation Assay, Promega, Nepean, ON, Canada) as previously described (46, 47). Ten microliters per well of MTS was added and absorbance was measured 3 hours later at 490 nm using a Synergy HTX Multi-Mode 96-well plate reader (Agilent). The percentage of cell survival was calculated and the EC50 values were determined (48).
We also combined Remdesivir with the two polyphenols (Remdesivir/EGCG and Remdesivir/Isoquercetin) to evaluate antiviral activity. The EC50 of combined polyphenols and antiviral were evaluated using the same principle as for single component. Serial dilutions of the two combinations, with concentrations surrounding the EC50 of each molecule tested separately were cross-mixed, resulting in 20 different pairwise comparisons. Cell culture infection and viability were processed as for monotherapy. The EC50 of one molecule (considered as the reference molecule) combined with a constant concentration of a second molecule was calculated. Experiments were done twice in quadruplicate. Dose-response curves were performed with Graph-Pad Prism 8.
To evaluate the synergistic effect of antiviral compounds, we used the SynergyFinder software version 2.0 (https://synergyfinder.fimm.fi/synergy/20210225212818919177/). We obtained a dose-response percent matrix inhibition of single and combined compounds (Remdesivir/EGCG and Remdesivir/Isoquercetin) and a 3D interaction surface response between these compounds, and calculated the synergy score for the combination using ZIP (Zero Interaction Potency) reference model as described previously (49). Red areas in the surface response and a synergy score larger than 10 correspond to a synergistic interaction between compounds (https://synergyfinder.fimm.fi/synergy/synfin_docs/#datanal).
Polyphenols and Remdesivir cytotoxic effects were evaluated using the same protocol as for antiviral combinations except for the addition of the virus. Cell viability was calculated and normalized with untreated control, which was defined as 100%.
We first determined the 50% effective concentration (EC50) of each compound used in monotherapy. As shown in Table 1, EGCG and Isoquercetin were effective to inhibit SARS-CoV-2 replication by 50% at 85.65 ± 6.45 μM and 23.99 ± 1.07 μM, respectively. Isoquercetin has already shown its effectiveness against Influenza and Ebola virus (16, 50) and has been proposed for SARS-CoV-2 treatment (51, 52). Although the EC50 of EGCG was relatively high using this assay, it was previously described as effective to block infection of ancestral SARS-CoV-2 and UK-B.1.1.7, SA-B.1.351 and CA-B.1.429 variants, in vitro (53). Remdesivir, the first drug approved for severe COVID-19 (8), has been tested in parallel with polyphenols. The EC50 of Remdesivir was 9.18 ± 4.88 μM. Another study using Vero cells found a similar EC50 value at 8.24 μM (54). These findings confirm the capacity of EGCG, Isoquercetin, and Remdesivir to inhibit SARS-CoV-2 replication.
Table 1 Antiviral activity of polyphenols (EGCG or Isoquercetin) and Remdesivir alone or in combination against SARS-CoV-2. EC50 of one compound alone or combined was determined.
We investigated the antiviral effects of the combination of Remdesivir with EGCG or Isoquercetin by mixing different concentrations of each compound, and calculated the EC50 of the reference compound combined with a constant concentration of a second one. The combination of remdesivir and EGCG or Isoquercetin exhibited highly potent synergism as compared with their individual EC50. Considering Remdesivir as reference compound, the addition of 15.65 μM of EGCG or Isoquercetin, reduced the Remdesivir EC50 values by at least half (8.18 ± 4.88 μM to 4.42 ± 2.13 and 2.29 ± 0.02, respectively). The EC50 was reduced 5 times with 31.5 μM of EGCG (Table 1). When EGCG and Isoquercetin concentrations increased, the Remdesivir EC50 could not be determined because cell viability was higher than 50% for all concentrations tested. These results indicate that low concentrations of polyphenols, about 5 times of EGCG EC50 and 1.5 times of Isoquercetin EC50 can potentiate the activity of Remdesivir.
We then used EGCG or Isoquercetin as reference compounds (15.6 to 125 μM and 7.8 to 62.5 μM respectively), and calculated their EC50 at a constant concentration of Remdesivir. The addition of much lower concentrations of EGCG-Remdesivir drastically reduced the EC50 of EGCG (with 1.25 μM of Remdesivir, 3.1-fold reduction; with 2.5 μM of Remdesivir, 3.4-fold reduction) (Table 1). When 5 μM of Remdesivir were added, a concentration below the EC50, we could not determine the EGCG EC50 because cell viabilities were higher than 50% for all concentrations tested, indicating a potential effect of EGCG to improve the antiviral effect of Remdesivir. The EC50 of Isoquercetin was also improved with different concentrations of Remdesivir (Table 1), but not to a greater extent as with EGCG.
Dose-response curves of compounds tested alone or in combination against SARS-CoV-2 were also evaluated. Serial dilutions of Remdesivir were tested with different constant concentrations of EGCG or Isoquercetin and vice versa. As shown in Figure 1A, EGCG with concentration higher than 15.62 μM potentiated the effect of Remdesivir. This finding was observed for Isoquercetin combined with Remdesivir, but only at certain concentrations (1.25 to5 μM of Remdesivir) (Figure 1B). Based on serial dilutions of EGCG or Isoquercetin, in combination with different constant concentrations of Remdesivir showed a higher potency for EGCG/Remdesivir than that for Isoquercetin/Remdesivir (Figures 1C, D).
Figure 1 Dose-response curves of antivirals compounds tested alone or in combination against SARS-CoV-2. Serial dilutions of Remdesivir (1.25-20 μM) combined with constant concentrations of EGCG (A) or Isoquercetin (B). (C) Serial dilutions of EGCG (15.62-125 μM) combined with constant concentrations of Remdesivir. (D) Serial dilutions of Isoquercetin (7.81-62.5 μM) combined with constant concentrations of Remdesivir. X axis: Compound concentration in Log10 (μM). Y axis: Percentage of cell survival. Three different experiments were done in quadruplicates each time for both drug combinations.
Overall, these observations suggested a reciprocal positive effect of EGCG and Isoquercetin with Remdesivir.
To assess the synergistic interaction between Remdesivir and EGCG or Isoquercetin, we used the SynergyFinder web application (https://synergyfinder.fimm.fi) which generated dose-response matrix, 3D interaction surface response between these compounds and allowed to calculate the synergy score.
For Remdesivir/EGCG combination, the dose-response matrix showed that most of the boxes are red, corresponding to a clear synergistic inhibitory effect of Remdesivir/EGCG against SARS-CoV-2 (Figure 2A).
Figure 2 Synergistic antiviral effects of polyphenols and Remdesivir against SARS-CoV-2. (A) Combination of Remdesivir- EGCG. At the top, dose-response matrix with the percentage of inhibition induced by single and combination drugs on SARS-CoV-2 infected cells (red box=high percentage of virus inhibition); In the middle, interaction surface response between these compounds with the synergy score for the combination using ZIP reference model (red areas correspond to a synergy score larger than 10 = synergistic interaction between two drugs); At the bottom, cytotoxicity evaluation on uninfected cells (red box=high percentage of cell viability) of (A) Remdesivir-EGCG and (B) Remdesivir-Isoquercetin.
This profile was confirmed by analyzing the 3D interaction surface responses between the polyphenols and the antiviral and indicated a large red area and a synergy score of 30.615 (Figure 2A). The dose-response matrix for the combination of Isoquercetin and Remdesivir showed a modest synergistic effect, observed by pallor red boxes and a synergy score of 11.072 (Figure 2B).
In this study, we aimed to investigate the antiviral effects of the combination of Remdesivir with EGCG or Isoquercetin. We confirmed the inhibitory effects of EGCG and isoquercetin against ancestral SARS-CoV-2 and demonstrated their strong antiviral synergistic effects with Remdesivir in vitro. Remdesivir combined with EGCG showed a synergy score of 30.615. This score is high, compared to previous studies testing the Remdesivir combination using Vero cells (55, 56). EGCG interacts with viral membrane proteins and/or cellular proteins and blocks the early stages of infection such as attachment, post-adsorption entry, and genome replication by inhibiting reverse transcriptase, both in vitro and in vivo (31). Interestingly, EGCG appears to boost the antiviral action of Remdesivir, which means that lower concentration of the latter is needed to provide a similar antiviral activity. This is possibly due to the different mechanism of action of EGCG and Remdesivir. Preliminary data from our group (not published) and others (53, 57) have shown that EGCG inhibit SARS-CoV-2 in vitro through their interaction with the virions rather than the cells because pretreatment of virions but not cells with catechin derivatives significantly suppressed the viral infection (53, 57).
Colpitts and Schang (58) have shown that EGCG acts directly on the surface of several virus surface proteins without affecting the fluidity or integrity of the virion envelope by competing with heparan sulfate or sialic acid moieties in cellular glycans preventing virion binding to cells. Additionally, EGCG was also shown to have an inhibitory activity against the SARS-CoV-2 3CL-protease and reduce viral replication in cell culture (59). A multicenter, double-blind, randomized, placebo-controlled clinical trial has been proposed in health care workers directly exposed to clinical care, daily contact, or traffic of individuals with suspected COVID-19 during the epidemic outbreak. The aim of this latter study is to determine the efficacy of Previfenon® (EGCG) to prevent COVID-19, enhance systemic immunity, and decrease the frequency and intensity of selected symptoms when used as pre-exposure chemoprophylaxis to SARS-CoV-2 (https://clinicaltrials.gov/ct2/show/NCT04446065?term=EGCG+%28Previfenon%29&cond=COVID-19&draw=2&rank=1).
The active triphosphate form of Remdesivir acts as a nucleoside analog and inhibits the RdRp of SARS-CoV-2 and other coronaviruses. Remdesivir is incorporated by the RdRp into the growing RNA product and allows the addition of three more nucleotides before RNA synthesis stalls (60). Thus, since targets and mode of actions of each antiviral differ, and are potentiated by one another, we surmise that Remdesivir/EGCG combination could be used as an alternative therapy for immunocompromised patients or for severe cases of COVID-19. EGCG has been shown to inhibit SARS-CoV 3CLproteases with a IC50 in similar ranges as observed in the present study (45). This concentration of the molecule in circulation is attainable by dietary consumption of a green tea extract supplements (61). Moreover, EGCG has an antioxidant and anti-inflammatory effects (62–64). Of note, one case of resistance to Remdesivir was recently reported in immunocompromised patients just about a year after this drug was approved by the U.S. FDA, justifying the improvement of COVID-19 treatment (65). Therefore, using polyphenols along with Remdesivir could be an option to prevent mild to severe disease or to improve outcomes of patients with severe COVID-19 as well as to limit antiviral resistance.
Remdesivir combined with Isoquercetin showed a synergy score of 11.072. Using an in vitro fluorescence resonance energy transfer (FRET) SARS-CoV 3CLpro assay, Ryu et al. (66) have found that quercetin had an IC50 of 24 µM, which is in the activity range observed with isoquercetin in our study. Isoquercetin is the glycosylated form of quercetin found in plants. Its absorption is either intact through GLUT2 transporters or after deglycosylation from enterocyte produced lactose phloridzin hydrolase releasing the aglycone in circulation. Absorbed quercetin is then rapidly metabolized by hepatic phase II enzymes, leading to the glucuronidated form of the molecule. The molecule is thus bioavailable and can act systemically. Of note, an open-label randomized phase-2 study of Isoquercetin and standard of care versus standard of care only for the treatment of COVID-19 will be undertaken in 2022 (https://clinicaltrials.gov/ct2/show/record/NCT04536090).
Although the antiviral combinations demonstrated efficacy against SARS-CoV-2, they do not appear to be cytotoxic. Antiviral cytotoxicity was evaluated alone or in combination. Indeed, no cytotoxic effect was observed with all antiviral concentrations tested (Figures 2A, B). This observation confirms the low cytotoxic concentration 50 (CC50) of Quercetin observed previously (67).
This study confirmed the inhibitory effects of two polyphenols, EGCG and Isoquercetin, against SARS-CoV-2 and demonstrated their antiviral synergistic effects with Remdesivir in vitro. In order to improve antiviral therapy, to reduce the development of resistant strains or intolerance by using a high dose of Remdesivir alone, this study demonstrated that combining polyphenols and Remdesivir, which have different mechanisms of action, could be considered as an efficient therapeutic alternative. Also, EGCG and Isoquercetin could be used for prophylaxis in high-risk populations, or for the treatment of COVID-19 patients as an adjunct to Remdesivir due to their strong synergistic effect against SARS-CoV-2, especially for EGCG which has shown the highest synergy score. Moreover, if an orally bioavailable formulation of Remdesivir shows promising results, this combination could be extended to outpatients. Our results warrant further studies to explore the efficacy of each combination therapy using animal models as well as clinical investigations for the prophylaxis and treatment of SARS-CoV-2 infections.
The raw data supporting the conclusions of this article will be made available by the authors, without undue reservation.
Conceptualization, HR and MB; methodology, HR, AB, LC, KP, and MB; software, HR and LC; validation, YD and MB; formal analysis, HR and MB; supervision, YD and MB; writing—original draft preparation, HR; writing—review and editing, YD and MB; funding acquisition, YD and MB. All authors have read and agreed to the published version of the manuscript.
This research was funded by Natural Sciences and Engineering Research Council of Canada (NSERC), Alliance Grants, grant number ALLRP 552135-2020 to YD and MB and by the Canadian Institutes of Health Research (CIHR), grant number 170629 to MB. This research was supported in part by M.B. Sentinel North Research Chair at Université Laval, funded by the Canada First Research Excellence Fund
The authors declare that the research was conducted in the absence of any commercial or financial relationships that could be construed as a potential conflict of interest.
All claims expressed in this article are solely those of the authors and do not necessarily represent those of their affiliated organizations, or those of the publisher, the editors and the reviewers. Any product that may be evaluated in this article, or claim that may be made by its manufacturer, is not guaranteed or endorsed by the publisher.
The authors would like to acknowledge the in-kind support of Diana Food Canada and Millenial Tea.
1. World Health Organization. WHO Coronavirus Disease (COVID- 19) Dashboard. Available at: https://covid19.who.int.
2. Zhu N, Zhang D, Wang W, Li X, Yang B, Song J, et al. A Novel Coronavirus From Patients With Pneumonia in China, 2019. N Engl J Med (2020) 382(8):727–33. doi: 10.1056/NEJMoa2001017
3. Chen N, Zhou M, Dong X, Qu J, Gong F, Han Y, et al. Epidemiological and Clinical Characteristics of 99 Cases of 2019 Novel Coronavirus Pneumonia in Wuhan, China: A Descriptive Study. Lancet (2020) 395(10223):507–13. doi: 10.1016/S0140-6736(20)30211-7
4. Levy E, Delvin E, Marcil V, Spahis S. Can Phytotherapy With Polyphenols Serve as a Powerful Approach for the Prevention and Therapy Tool of Novel Coronavirus Disease 2019 (COVID-19)? Am J Physiol Endocrinol Metab (2020) 319(4):E689–708. doi: 10.1152/ajpendo.00298.2020
5. Dejnirattisai W, Shaw RH, Supasa P, Liu C, Stuart AS, Pollard AJ, et al. Reduced Neutralisation of SARS-CoV-2 Omicron B.1.1.529 Variant by Post-Immunisation Serum. Lancet (2021) 399(10321):234–236. doi: 10.1016/S0140-6736(21)02844-0
6. Agostini ML, Andres EL, Sims AC, Graham RL, Sheahan TP, Lu X, et al. Coronavirus Susceptibility to the Antiviral Remdesivir (GS-5734) Is Mediated by the Viral Polymerase and the Proofreading Exoribonuclease. mBio (2018) 9(2). doi: 10.1128/mBio.00221-18
7. Sheahan TP, Sims AC, Leist SR, Schafer A, Won J, Brown AJ, et al. Comparative Therapeutic Efficacy of Remdesivir and Combination Lopinavir, Ritonavir, and Interferon Beta Against MERS-CoV. Nat Commun (2020) 11(1):222. doi: 10.1038/s41467-019-13940-6
8. Lamb YN. Remdesivir: First Approval. Drugs (2020) 80(13):1355–63. doi: 10.1007/s40265-020-01378-w
9. Beigel JH, Tomashek KM, Dodd LE, Mehta AK, Zingman BS, Kalil AC, et al. Remdesivir for the Treatment of Covid-19 - Final Report. N Engl J Med (2020) 383(19):1813–26. doi: 10.1056/NEJMoa2007764
10. Schafer A, Martinez DR, Won JJ, Meganck RM, Moreira FR, Brown AJ, et al. Therapeutic Treatment With an Oral Prodrug of the Remdesivir Parental Nucleoside is Protective Against SARS-CoV-2 Pathogenesis in Mice. Sci Transl Med (2022) 14(643):eabm3410. doi: 10.1126/scitranslmed.abm3410
11. Abdal Dayem A, Choi HY, Kim YB, Cho SG. Antiviral Effect of Methylated Flavonol Isorhamnetin Against Influenza. PLoS One (2015) 10(3):e0121610. doi: 10.1371/journal.pone.0121610
12. Kim Y, Narayanan S, Chang KO. Inhibition of Influenza Virus Replication by Plant-Derived Isoquercetin. Antiviral Res (2010) 88(2):227–35. doi: 10.1016/j.antiviral.2010.08.016
13. Ikuta K, Hashimoto K, Kaneko H, Mori S, Ohashi K, Suzutani T. Anti-Viral and Anti-Bacterial Activities of an Extract of Blackcurrants (Ribes Nigrum L.). Microbiol Immunol (2012) 56(12):805–9. doi: 10.1111/j.1348-0421.2012.00510.x
14. Sekizawa H, Ikuta K, Ohnishi-Kameyama M, Nishiyama K, Suzutani T. Identification of the Components in a Vaccinium Oldhamii Extract Showing Inhibitory Activity Against Influenza Virus Adsorption. Foods (2019) 8(5):172. doi: 10.3390/foods8050172
15. Mohd A, Zainal N, Tan KK, AbuBakar S. Resveratrol Affects Zika Virus Replication In Vitro. Sci Rep (2019) 9(1):14336. doi: 10.1038/s41598-019-50674-3
16. Qiu X, Kroeker A, He S, Kozak R, Audet J, Mbikay M, et al. Prophylactic Efficacy of Quercetin 3-Beta-O-D-Glucoside Against Ebola Virus Infection. Antimicrob Agents Chemother (2016) 60(9):5182–8. doi: 10.1128/AAC.00307-16
17. Han YS, Penthala NR, Oliveira M, Mesplede T, Xu H, Quan Y, et al. Identification of Resveratrol Analogs as Potent Anti-Dengue Agents Using a Cell-Based Assay. J Med Virol (2017) 89(3):397–407. doi: 10.1002/jmv.24660
18. Jasso-Miranda C, Herrera-Camacho I, Flores-Mendoza LK, Dominguez F, Vallejo-Ruiz V, Sanchez-Burgos GG, et al. Antiviral and Immunomodulatory Effects of Polyphenols on Macrophages Infected With Dengue Virus Serotypes 2 and 3 Enhanced or Not With Antibodies. Infect Drug Resist (2019) 4(2):898–907. doi: 10.2147/IDR.S210890
19. Khachatoorian R, Arumugaswami V, Raychaudhuri S, Yeh GK, Maloney EM, Wang J, et al. Divergent Antiviral Effects of Bioflavonoids on the Hepatitis C Virus Life Cycle. Virology (2012) 433(2):346–55. doi: 10.1016/j.virol.2012.08.029
20. Clouser CL, Chauhan J, Bess MA, van Oploo JL, Zhou D, Dimick-Gray S, et al. Anti-HIV-1 Activity of Resveratrol Derivatives and Synergistic Inhibition of HIV-1 by the Combination of Resveratrol and Decitabine. Bioorg Med Chem Lett (2012) 22(21):6642–6. doi: 10.1016/j.bmcl.2012.08.108
21. Heredia A, Davis C, Amin MN, Le NM, Wainberg MA, Oliveira M, et al. Targeting Host Nucleotide Biosynthesis With Resveratrol Inhibits Emtricitabine-Resistant HIV-1. AIDS (2014) 28(3):317–23. doi: 10.1097/QAD.0000000000000168
22. Carvalho OV, Botelho CV, Ferreira CG, Ferreira HC, Santos MR, Diaz MA, et al. In Vitro Inhibition of Canine Distemper Virus by Flavonoids and Phenolic Acids: Implications of Structural Differences for Antiviral Design. Res Vet Sci (2013) 95(2):717–24. doi: 10.1016/j.rvsc.2013.04.013
23. dos Santos AE, Kuster RM, Yamamoto KA, Salles TS, Campos R, de Meneses MD, et al. Quercetin and Quercetin 3-O-Glycosides From Bauhinia Longifolia (Bong.) Steud. Show Anti-Mayaro Virus Activity. Parasit Vectors (2014) 7:130. doi: 10.1186/1756-3305-7-130
24. Bisignano C, Mandalari G, Smeriglio A, Trombetta D, Pizzo MM, Pennisi R, et al. Almond Skin Extracts Abrogate HSV-1 Replication by Blocking Virus Binding to the Cell. Viruses (2017) 9(7):187. doi: 10.3390/v9070178
25. Li S, Hattori T, Kodama EN. Epigallocatechin Gallate Inhibits the HIV Reverse Transcription Step. Antivir Chem Chemother (2011) 21(6):239–43. doi: 10.3851/IMP1774
26. Vazquez-Calvo A, Jimenez de Oya N, Martin-Acebes MA, Garcia-Moruno E, Saiz JC. Antiviral Properties of the Natural Polyphenols Delphinidin and Epigallocatechin Gallate Against the Flaviviruses West Nile Virus, Zika Virus, and Dengue Virus. Front Microbiol (2017) 8:1314. doi: 10.3389/fmicb.2017.01314
27. Pradhan P, Nguyen ML. Herpes Simplex Virus Virucidal Activity of MST-312 and Epigallocatechin Gallate. Virus Res (2018) 249:93–8. doi: 10.1016/j.virusres.2018.03.015
28. Song JM. Anti-Infective Potential of Catechins and Their Derivatives Against Viral Hepatitis. Clin Exp Vaccine Res (2018) 7(1):37–42. doi: 10.7774/cevr.2018.7.1.37
29. Lipson SM, Ozen FS, Louis S, Karthikeyan L. Comparison of Alpha-Glucosyl Hesperidin of Citrus Fruits and Epigallocatechin Gallate of Green Tea on the Loss of Rotavirus Infectivity in Cell Culture. Front Microbiol (2015) 6:359. doi: 10.3389/fmicb.2015.00359
30. Xu J, Xu Z, Zheng W. A Review of the Antiviral Role of Green Tea Catechins. Molecules (2017) 22(8):1337. doi: 10.3390/molecules22081337
31. Kaihatsu K, Yamabe M, Ebara Y. Antiviral Mechanism of Action of Epigallocatechin-3-O-Gallate and Its Fatty Acid Esters. Molecules (2018) 23(10):2475. doi: 10.3390/molecules23102475
32. Lee S, Mailar K, Kim MI, Park M, Kim J, Min DH, et al. Plant-Derived Purification, Chemical Synthesis, and In Vitro/In Vivo Evaluation of a Resveratrol Dimer, Viniferin, as an HCV Replication Inhibitor. Viruses (2019) 11(10):890. doi: 10.3390/v11100890
33. Espinoza JL, Takami A, Trung LQ, Kato S, Nakao S. Resveratrol Prevents EBV Transformation and Inhibits the Outgrowth of EBV-Immortalized Human B Cells. PloS One (2012) 7(12):e51306. doi: 10.1371/journal.pone.0051306
34. Ding Z, Sun G, Zhu Z. Hesperidin Attenuates Influenza A Virus (H1N1) Induced Lung Injury in Rats Through its Anti-Inflammatory Effect. Antivir Ther (2018) 23(7):611–5. doi: 10.3851/IMP3235
35. Saha RK, Takahashi T, Suzuki T. Glucosyl Hesperidin Prevents Influenza a Virus Replication In Vitro by Inhibition of Viral Sialidase. Biol Pharm Bull (2009) 32(7):1188–92. doi: 10.1248/bpb.32.1188
36. Gangehei L, Ali M, Zhang W, Chen Z, Wakame K, Haidari M. Oligonol a Low Molecular Weight Polyphenol of Lychee Fruit Extract Inhibits Proliferation of Influenza Virus by Blocking Reactive Oxygen Species-Dependent ERK Phosphorylation. Phytomedicine (2010) 17(13):1047–56. doi: 10.1016/j.phymed.2010.03.016
37. Wong G, He S, Siragam V, Bi Y, Mbikay M, Chretien M, et al. Antiviral Activity of Quercetin-3-Beta-O-D-Glucoside Against Zika Virus Infection. Virol Sin (2017) 32(6):545–7. doi: 10.1007/s12250-017-4057-9
38. Chiow KH, Phoon MC, Putti T, Tan BK, Chow VT. Evaluation of Antiviral Activities of Houttuynia Cordata Thunb. Extract, Quercetin, Quercetrin and Cinanserin on Murine Coronavirus and Dengue Virus Infection. Asian Pac J Trop Med (2016) 9(1):1–7. doi: 10.1016/j.apjtm.2015.12.002
39. Uchide N, Toyoda H. Antioxidant Therapy as a Potential Approach to Severe Influenza-Associated Complications. Molecules (2011) 16(3):2032–52. doi: 10.3390/molecules16032032
40. Pandey P, Rane JS, Chatterjee A, Kumar A, Khan R, Prakash A, et al. Targeting SARS-CoV-2 Spike Protein of COVID-19 With Naturally Occurring Phytochemicals: An in Silico Study for Drug Development. J Biomol Struct Dyn (2021) 39(16):6306–16. doi: 10.1080/07391102.2020.1796811
41. Das S, Sarmah S, Lyndem S, Singha Roy A. An Investigation Into the Identification of Potential Inhibitors of SARS-CoV-2 Main Protease Using Molecular Docking Study. J Biomol Struct Dyn (2021) 39(9):3347–57. doi: 10.1080/07391102.2020.1763201
42. Huynh T, Wang H, Luan B. In Silico Exploration of the Molecular Mechanism of Clinically Oriented Drugs for Possibly Inhibiting SARS-CoV-2's Main Protease. J Phys Chem Lett (2020) 11(11):4413–20. doi: 10.1021/acs.jpclett.0c00994
43. El-Missiry MA, Fekri A, Kesar LA, Othman AI. Polyphenols are Potential Nutritional Adjuvants for Targeting COVID-19. Phytother Res (2021) 35(6):2879–89. doi: 10.1002/ptr.6992
44. Surucic R, Tubic B, Stojiljkovic MP, Djuric DM, Travar M, Grabez M, et al. Computational Study of Pomegranate Peel Extract Polyphenols as Potential Inhibitors of SARS-CoV-2 Virus Internalization. Mol Cell Biochem (2021) 476(2):1179–93. doi: 10.1007/s11010-020-03981-7
45. Jo S, Kim S, Shin DH, Kim MS. Inhibition of SARS-CoV 3CL Protease by Flavonoids. J Enzyme Inhib Med Chem (2020) 35(1):145–51. doi: 10.1080/14756366.2019.1690480
46. Perreault M, Maltais R, Dutour R, Poirier D. Explorative Study on the Anticancer Activity, Selectivity and Metabolic Stability of Related Analogs of Aminosteroid RM-133. Steroids (2016) 115:105–13. doi: 10.1016/j.steroids.2016.08.015
47. Baz M, Goyette N, Griffin BD, Kobinger GP, Boivin G. In Vitro Susceptibility of Geographically and Temporally Distinct Zika Viruses to Favipiravir and Ribavirin. Antivir Ther (2017) 22(7):613–8. doi: 10.3851/IMP3180
48. Checkmahomed L, Padey B, Pizzorno A, Terrier O, Rosa-Calatrava M, Abed Y, et al. In Vitro Combinations of Baloxavir Acid and Other Inhibitors Against Seasonal Influenza A Viruses. Viruses (2020) 12(10):1139. doi: 10.3390/v12101139
49. Yadav B, Wennerberg K, Aittokallio T, Tang J. Searching for Drug Synergy in Complex Dose-Response Landscapes Using an Interaction Potency Model. Comput Struct Biotechnol J (2015) 13:504–13. doi: 10.1016/j.csbj.2015.09.001
50. Fan D, Zhou X, Zhao C, Chen H, Zhao Y, Gong X. Anti-Inflammatory, Antiviral and Quantitative Study of Quercetin-3-O-Beta-D-Glucuronide in Polygonum Perfoliatum L. Fitoterapia (2011) 82(6):805–10. doi: 10.1016/j.fitote.2011.04.007
51. Saeedi-Boroujeni A, Mahmoudian-Sani MR. Anti-Inflammatory Potential of Quercetin in COVID-19 Treatment. J Inflammation (Lond) (2021) 18(1):3. doi: 10.1186/s12950-021-00268-6
52. Kushwaha PP, Singh AK, Bansal T, Yadav A, Prajapati KS, Shuaib M, et al. Identification of Natural Inhibitors Against SARS-CoV-2 Drugable Targets Using Molecular Docking, Molecular Dynamics Simulation, and MM-PBSA Approach. Front Cell Infect Microbiol (2021) 11:730288. doi: 10.3389/fcimb.2021.730288
53. Liu J, Bodnar BH, Meng F, Khan AI, Wang X, Saribas S, et al. Epigallocatechin Gallate From Green Tea Effectively Blocks Infection of SARS-CoV-2 and New Variants by Inhibiting Spike Binding to ACE2 Receptor. Cell Biosci (2021) 11(1):168. doi: 10.1186/s13578-021-00680-8
54. Jeon S, Ko M, Lee J, Choi I, Byun SY, Park S, et al. Identification of Antiviral Drug Candidates Against SARS-CoV-2 From FDA-Approved Drugs. Antimicrob Agents Chemother (2020) 64(7):e00819-20. doi: 10.1128/AAC.00819-20
55. Ko M, Chang SY, Byun SY, Ianevski A, Choi I, Pham Hung d'Alexandry d'Orengiani AL, et al. Screening of FDA-Approved Drugs Using a MERS-CoV Clinical Isolate From South Korea Identifies Potential Therapeutic Options for COVID-19. Viruses (2021) 13(4):651. doi: 10.3390/v13040651
56. Chen T, Fei CY, Chen YP, Sargsyan K, Chang CP, Yuan HS, et al. Synergistic Inhibition of SARS-CoV-2 Replication Using Disulfiram/Ebselen and Remdesivir. ACS Pharmacol Transl Sci (2021) 4(2):898–907. doi: 10.1021/acsptsci.1c00022
57. Ohgitani E, Shin-Ya M, Ichitani M, Kobayashi M, Takihara T, Kawamoto M, et al. Significant Inactivation of SARS-CoV-2 In Vitro by a Green Tea Catechin, a Catechin-Derivative, and Black Tea Galloylated Theaflavins. Molecules (2021) 11;26(12):3572. doi: 10.3390/molecules26123572
58. Colpitts CC, Schang LM. A Small Molecule Inhibits Virion Attachment to Heparan Sulfate- or Sialic Acid-Containing Glycans. J Virol (2014) 88(14):7806–17. doi: 10.1128/JVI.00896-14
59. Jang M, Park YI, Cha YE, Park R, Namkoong S, Lee JI, et al. Tea Polyphenols EGCG and Theaflavin Inhibit the Activity of SARS-CoV-2 3cl-Protease In Vitro. Evid Based Complement Alternat Med (2020) 2020:5630838. doi: 10.1155/2020/5630838
60. Tchesnokov EP, Gordon CJ, Woolner E, Kocincova D, Perry JK, Feng JY, et al. Correction: Template-Dependent Inhibition of Coronavirus RNA-Dependent RNA Polymerase by Remdesivir Reveals a Second Mechanism of Action. J Biol Chem (2021) 297(2):101048. doi: 10.1016/j.jbc.2021.101048
61. Lee MJ, Maliakal P, Chen L, Meng X, Bondoc FY, Prabhu S, et al. Pharmacokinetics of Tea Catechins After Ingestion of Green Tea and (-)-Epigallocatechin-3-Gallate by Humans: Formation of Different Metabolites and Individual Variability. Cancer Epidemiol Biomarkers Prev (2002) 11(10 Pt 1):1025–32.
62. Khalatbary AR, Ahmadvand H. Anti-Inflammatory Effect of the Epigallocatechin Gallate Following Spinal Cord Trauma in Rat. Iran BioMed J (2011) 15(1-2):31–7.
63. Tozser J, Benko S. Natural Compounds as Regulators of NLRP3 Inflammasome-Mediated IL-1beta Production. Mediators Inflammation (2016) 2016:5460302. doi: 10.1155/2016/5460302
64. Zhong Y, Shahidi F. Lipophilized Epigallocatechin Gallate (EGCG) Derivatives as Novel Antioxidants. J Agric Food Chem (2011) 59(12):6526–33. doi: 10.1021/jf201050j
65. Gandhi S, Klein J, Robertson A, Pena-Hernandez MA, Lin MJ, Roychoudhury P, et al. De Novo Emergence of a Remdesivir Resistance Mutation During Treatment of Persistent SARS-CoV-2 Infection in an Immunocompromised Patient: A Case Report. medRxiv (2021). doi: 10.1101/2021.11.08.21266069
66. Ryu YB, Jeong HJ, Kim JH, Kim YM, Park JY, Kim D, et al. Biflavonoids From Torreya Nucifera Displaying SARS-CoV 3CL(Pro) Inhibition. Bioorg Med Chem (2010) 18(22):7940–7. doi: 10.1016/j.bmc.2010.09.035
Keywords: SARS-CoV-2, polyphenols, remdesivir, combinational therapy, synergistic effect, in vitro
Citation: Rabezanahary H, Badr A, Checkmahomed L, Pageau K, Desjardins Y and Baz M (2022) Epigallocatechin Gallate and Isoquercetin Synergize With Remdesivir to Reduce SARS-CoV-2 Replication In Vitro. Front. Virol. 2:956113. doi: 10.3389/fviro.2022.956113
Received: 29 May 2022; Accepted: 22 June 2022;
Published: 13 July 2022.
Edited by:
Xin Yin, Chinese Academy of Agricultural Sciences (CAAS), ChinaReviewed by:
Andrew Bubak, University of Colorado Anschutz Medical Campus, United StatesCopyright © 2022 Rabezanahary, Badr, Checkmahomed, Pageau, Desjardins and Baz. This is an open-access article distributed under the terms of the Creative Commons Attribution License (CC BY). The use, distribution or reproduction in other forums is permitted, provided the original author(s) and the copyright owner(s) are credited and that the original publication in this journal is cited, in accordance with accepted academic practice. No use, distribution or reproduction is permitted which does not comply with these terms.
*Correspondence: Mariana Baz, bWFyaWFuYS5iYXpAY3JjaHVkZXF1ZWJlYy51bGF2YWwuY2E=
Disclaimer: All claims expressed in this article are solely those of the authors and do not necessarily represent those of their affiliated organizations, or those of the publisher, the editors and the reviewers. Any product that may be evaluated in this article or claim that may be made by its manufacturer is not guaranteed or endorsed by the publisher.
Research integrity at Frontiers
Learn more about the work of our research integrity team to safeguard the quality of each article we publish.