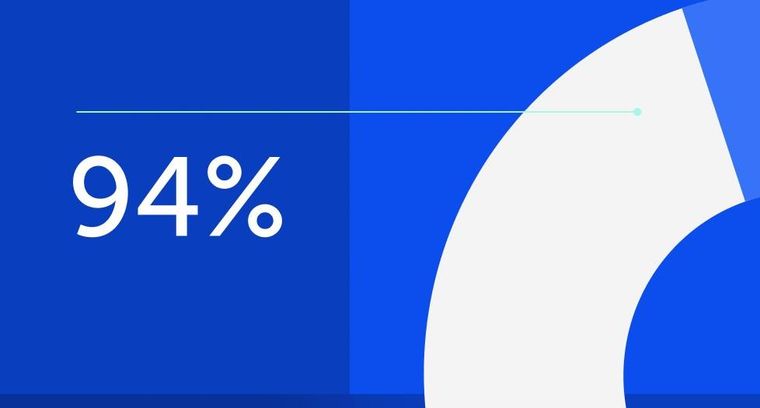
94% of researchers rate our articles as excellent or good
Learn more about the work of our research integrity team to safeguard the quality of each article we publish.
Find out more
ORIGINAL RESEARCH article
Front. Virol., 06 September 2021
Sec. Emerging and Reemerging Viruses
Volume 1 - 2021 | https://doi.org/10.3389/fviro.2021.727163
Subtype H9N2 avian influenza viruses (AIV), now the predominant avian influenza virus subtype in poultry in China, cause sporadic human infections manifesting as mild influenza-like illness. We isolated an H9N2 AIV from a critical case of respiratory illness in a 9-year-old with no underlying conditions. As this virus was associated with critical illness, we conducted a risk assessment to determine its mammalian pathogenicity. Clinical and laboratory data were collected from hospital records. A/Suzhou/GIRD01/2019 (H9N2) (GIRD01) was isolated from a throat swab and used in risk-assessment studies in comparison to prototypical and contemporary H9N2 AIVs and contemporary seasonal subtype H1N1 and H3N2 influenza viruses. The patient presented with fever, vomiting but rapidly declined to progressive wheezing followed by dyspnea. The patient was admitted to the intensive care unit and placed on mechanical ventilation. A diagnosis of pneumonia and type I respiratory failure was made. Viral RNA was detected in the bronchiolavelolar lavage and anal swab specimens, suggesting lower lung and extrapulmonary involvement. Respiratory syncytial virus was also detected by immunofluorescence in bronchiolavelolar lavage. Following an aggressive regimen of antiviral and antibacterial therapy, the patient recovered and was discharged from hospital after 13 days. GIRD01 was closely related to poultry-origin H9N2 AIVs in the area and contained several known markers of mammalian pathogenicity. GIRD01 also showed a strong affinity for mammalian-type over avian-type sialic acids. GIRD01 replicated more efficiently compared to older H9N2 viruses and contemporary seasonal viruses in vitro and produced asymptomatic infections in mice. In summary, GIRD01 was well-adapted to replication in in vitro and in vivo mammalian models but was not more pathogenic compared to similar contemporary strains of H9N2 AIVs. Therefore, these viruses may pose a risk of causing severe respiratory disease in humans.
Subtype H9N2 avian influenza A viruses (AIVs) are now the predominant AIVs in poultry in China (1). Whilst the positivity rate for AIV detection during poultry surveillance in China decreased from the period of 2014–2016 to 2016–2019, the proportion of viruses that were of the H9N2 subtype increased, such that 72.75% of viruses isolated were of the H9N2 subtype (1). H9N2 AIVs were particularly dominant in chickens, at 89.95% of isolates compared to ducks, geese, pigeon and environmental samples, at 35.71, 7.32, 62.51, and 51.52%, respectively (1). Human cases of H9N2 AIV are mild and relatively uncommon, with only 59 laboratory confirmed cases reported since 1998, the majority of which (69.6%) were in children below the age of eight (2, 3). These cases have been mild with only one death linked to an underlying condition reported (4). Cases of H9N2 AIV infections in humans appear to be increasing, with the number of cases in the last 5 years exceeding half of the known cases since the first case was reported (3). The vast majority of these cases in recent years have been in China. Here we report a critical case of influenza-like illness (ILI) in a 9-year-old patient with no prior history of respiratory illness or underlying conditions. An H9N2 AIV, A/Suzhou/GIRD01/2019 (H9N2) (GIRD01) that was isolated from a throat swab obtained from this patient was genetically similar to poultry viruses isolated in the region. As H9N2 AIVs are not known to cause severe disease in otherwise healthy individuals, the zoonotic potential of this virus was of concern. As such, here we present this case and a virological risk assessment of GIRD01 based on the Influenza Risk Assessment Tool (IRAT) (Centers for Disease Control and Prevention, USA) (5).
Clinical data were retrieved from hospital records. This is a retrospective study in which only the data from hospital record was used without the direct involvement of human patient or swab sample. Viral RNA was extracted from patient swabs using the High Pure Viral RNA Kit (Roche, USA) and used in real time RT-PCR reactions using the Bioperfectus Influenza A/B Real-Time PCR Reagent Kit (bioPerfectus Technologies, Jiangsu, China) according to the manufacturer's instructions. Bronchoalveolar lavage fluid samples were tested for the presence of common respiratory viruses (influenza A and B, respiratory syncytial virus (RSV), adenovirus and parainfluenza 1, 2, and 3) using the D3 Ultra DFA Respiratory Virus Screening & ID Kit (Diagnostic Hybrids, Athens, OH, USA) according to the manufacturer's instructions.
GIRD01 was isolated from 10-day-old specific-pathogen free embryonated chicken eggs that were inoculated with throat swab material collected from the patient who was admitted to the Suzhou Children's Hospital. The sample was collected by the Suzhou Centre for Disease Control and Prevention. These studies were performed on virus cultured from a throat swab, not on the swab material itself. A/chicken/Zhejiang/198/2019 (H9N2) (ZJ/198) was isolated from a chicken in Zhejiang province of China. A/duck/Hong Kong/Y280/1999 (H9N2) (Y280), A/guinea fowl/Hong Kong/WF10/1999 (H9N2) (WF10), A/China/GLW/2018 (H1N1) (GLW), A/Guangzhou/MSLAB01/2017 (MSLAB01), and A/Puerto Rico/8/1934 (H1N1) (PR8) were obtained from the State Key Laboratory of Respiratory Diseases, Guangzhou, China. The genomes of GIRD01 and ZJ/198 were sequenced (accession numbers MT875134-MT875141 and MT875142-MT875149, respectively). Virus stocks were prepared by propagation in 10-day-old specific-pathogen free embryonated chicken eggs. Viral titres were determined by plaque assay using Madin-Darby Canine Kidney (MDCK) cells (ATCC, USA). Plaque sizes were measured using Image J (National Institutes of Health, USA). Growth kinetics of the viruses were determined by inoculating MDCK cells (ATCC, Manassas, VA) with a multiplicity of infection of 0.001 of virus in triplicate wells. At specified time points an aliquot of culture medium was collected and tissue culture infectious dose 50% (TCID50) titers were determined in MDCK cells using the Reed and Muench method (6). MDCK cells were maintained in minimal essential medium (MEM) supplemented with 10% (vol/vol) foetal bovine serum (Thermo Scientific, Waltham, MA).
Viral genomes were downloaded from the Influenza Research Database (http://www.fludb.org). Virus gene segments were aligned using MUSCLE. Phylogenetic trees were constructed using MEGA-X using the neighbour-joining algorithm with Kimura 2 and bootstrap values of 1,000. Final trees were constructed using iTOL (https://itol.embl.de/).
Viral RNA was extracted from harvested allantoic fluid using the RNeasy Plus Mini Kit (QIAGEN, Germany) according to the manufacturer's instructions. RT-PCR was performed using specific primers and the HiScript II One Step RT-PCR kit (Vazyme, Nanjing, China) according to the manufacturer's instructions.
Solid-phase receptor binding assays were performed as described previously (7) using the following biotinylated glycans; Neu5Acα2-3Galβ1-4Glcβ (α2,3′SL), Neu5Aca2-6Galb1-4Glcb (α2,6′SL), Neu5Acα2-3Galβ1-4GlcNAcβ-PAA-biotin (α2,3′SLN) and 6‘-SiaLacNAc-PAA-biotin (α2,6′SLN) (GlycoTech, Gaithersburg, MD).
Neuraminidase activity was measured using a fluorimetric assay using MUNANA (2'-(4-methylumbelliferyl)-α-D-N-acetylneuraminic acid) (Sigma) as a substrate (8, 9). Input viruses were normalised by hemagglutination titre and a 2-fold virus dilution series was incubated with MUNANA at a final concentration of 100 μM.
Specific pathogen free BALB/c female mice of 6–8 weeks of age were purchased from the Guangdong Medical Laboratory Animal Centre (Guangzhou, China). Mice were 19.81 ± 0.15 g at the beginning of the experiment. Mice were acclimatised post transportation for 1 week. Food and water were provided ad libitum. N = 8 mice were used in each experimental group, 56 mice in total. The experimental unit of this study was a single mouse. Mice were lightly anaesthetised using isofluorane anaesthesia prior to intranasal inoculation of virus in a total volume of 30 μL PBS. Mice inoculated with GIRD01, ZJ/198, Y280, or WF10 received 106 plaque forming units (PFU). Mice inoculated with PR8 received 104 or 102 PFU. The control group was inoculated with 30 μL PBS only and the weight loss observed in mice inoculated with virus was compared to this group. All inocula and intranasal inoculations were prepared and performed by a single investigator on the same day. The main outcome measured was changes in body weight and mice were also monitored twice daily for symptoms, namely ruffled fur, reduced mobility and arching of the back. Animals that lost >30% of their initial body weight during the study were humanely euthanised according to institutional guidelines.
Animal studies were performed in accordance with the relevant guidelines and approval from the Animal Care & Welfare Committee of The First Affiliated Hospital of Guangzhou Medical University. The human aspects of this study were retrospective and conducted in accordance with the relevant guidelines and the approval of the ethics committee of Suzhou University (approval number 2018CS093). The need for informed consent was waived by the ethics committee of Suzhou University.
On March 16, 2019, the patient presented to the hospital with fever, vomiting and progressive wheezing which was followed by dyspnoea. The patient was admitted to the hospital later that day (Figure 1). Closed drainage was performed to remove 350 mL of fluid from the right pleural cavity. The dyspnoea was not controlled and the patient was then admitted to the intensive care unit (ICU), with a temperature of 39.4°C, and a pulse rate of 167 beats per minute. The respiratory rate was 47 breaths per minute with three depressions observed in the inspiratory phase and an oxygen saturation (SPO2%) of 82%, despite oxygen delivery via nasal catheter at 3 L/min. The patient was consequently diagnosed with pneumonia and type I respiratory failure.
Figure 1. A/Suzhou/GIRD01/2019 (H9N2) was isolated from a critical case of respiratory disease in 9-year-old. (A) Timeline of disease course showing clinical features, treatments, and chest radiography. (B–D) imaging of the lung on day seven showing main lesions in the right upper lung, including an interlobular fissure (C,D) (yellow arrows).
In the ICU the patient was placed on mechanical ventilation and given methylprednisolone. On day two post admission, plastic bronchitis was confirmed by bronchoscopy and bronchoalveolar lavage was performed to remove the embolus. No gastrointestinal symptoms were reported. A respiratory swab collected upon admittance tested positive for influenza A virus (IAV) RNA but negative for IAV subtypes H1 and H3 and influenza B virus. As per national reporting requirements for pneumonia of unknown aetiology, the Suzhou Centre for Disease Control and Prevention subsequently determined the subtype of the virus as H9N2. Throat and anal swabs were collected from the patient twice per day from day three (Figure 1). IAV RNA was detected in, (i) bronchoalveolar lavage taken on day two, (ii) throat swabs on days three, five to eight and day 10, and, (iii) in anal swabs on days five and six by RT-PCR (Table 1). Direct immunofluorescence on bronchoalveolar lavage taken on day two tested positive for RSV but negative for IAV. An aggressive regimen of antiviral and antibacterial therapy was administered over the next 10 days that included meropenem, azithromycin, linezolid, and peramivir. Body temperature returned to normal on March 19 and the patient was discharged on March 28 after testing negative for IAV RNA twice in respiratory specimens and showing improvement of lung inflammatory lesions.
Table 1. Detection of influenza A virus hemagglutinin and neuraminidase genes by real-time PCR in throat and anal swabs.
Hemagglutination inhibition (HAI) titres in serum samples collected from the patient at acute and convalescent phases of disease tested positive for H9N2 antibodies, with titres of 80 and 1,280, respectively. A serum sample collected from the patient's father had a HAI titre < 10 at these same timepoints, indicating that transmission of this virus to close family members most likely did not occur (Table 2).
GIRD01 was isolated from a throat swab collected on day three. Sequencing and phylogenetic analyses showed that GIRD01was a triple reassortant virus with gene segments that clustered closely with other viruses isolated in poultry in the Yangtze river delta. A comparison of the virus sequence obtained from the original swab material and the egg-passaged virus revealed only one amino acid change in the egg-passaged virus. This mutation was D4N in neuraminidase. The hemagglutinin (HA), neuraminidase (NA) and non-structural (NS) gene segments were of Beijing/1994 (BJ/94) lineage origin (originated from A/chicken/Beijing/1/1994 (H9N2)- or A/duck/Hong Kong/Y280/1997 (H9N2)-like strains) (10). The polymerase basic 2 (PB2) and matrix (M) gene segments were of G1 lineage origin (originated from an A/quail/Hong Kong/G1/1997 (H9N2)-like strain) (11, 12). The polymerase basic 1 (PB1) and polymerase acidic (PA) gene segments were of F/98 lineage origin (originated from A/chicken/Shanghai/F/1998 (H9N2)-like strain) (Table 3) (10). Sequence analysis revealed that GIRD01 was a low pathogenic virus, with an HA cleavage motif of PSRSSRGL, and also contained multiple amino acids known to facilitate binding to α2,6-linked sialic acids, namely 226L in the receptor binding domain (H3 numbering) and 155T, 190T, 197K, and 212I (13–18). These amino acids were also present in A/chicken/Zhejiang/198/2019 (ZJ/198), a contemporary poultry virus isolated in the same region. In PB2 588V, associated with increased pathogenicity in the mouse model, was in GIRD01 and ZJ/198. At PB2 598, I and V were in GIRD01 and ZJ/198, respectively, whilst at PB2 292, V and I were in GIRD01 and ZJ/198, respectively. V598I and I292V have been linked to increased virulence in the mouse model (Table 4). As these markers were also found in other subtype H9N2 AIVs isolated in poultry, GIRD01 did not contain any known markers of mammalian pathogenicity that would make this virus unique amongst other similar H9N2 AIVs.
We studied the pathogenicity of GIRD01 in comparison to the closely related poultry virus ZJ/198 and two older prototypical H9N2 viruses, A/duck/Hong Kong/Y280/1999 (H9N2) (Y280) and A/guinea fowl/Hong Kong/WF10/1999 (H9N2) (WF10). All eight genes of GIRD01 and ZJ/198 fell into the same genetic clade (Table 3). Overall, GIRD01 shared over 95% sequence identity in all eight gene segments with ZJ/198 and >85% with Y280 and WF10 (Table 5).
Table 5. Percentage sequence similarity of the gene segments of A/Suzhou/GIRD01/2019 (H9N2) with A/Chicken/Zhejiang/198/2019 (H9N2), A/Guinea fowl/Hong Kong/WF10/1999 (H9N2) and A/Duck/Hong Kong/Y280/1997 (H9N2).
GIRD01 and ZJ/198 were well-adapted to replication in mammalian cells, showing rapid kinetics and higher peak titres in comparison to the older H9N2 AIVs Y280 and WF10. GIRD01 also replicated to significantly higher titres compared to the contemporary seasonal influenza viruses A/China/GLW/2018 (H1N1) (GLW) and A/Guangzhou/MSLAB01/2017 (H3N2) (MSLAB01) (Figure 2A). Plaque morphologies also showed that GIRD01 and ZJ/198 were well-adapted to replication in mammalian cells, producing significantly larger diameter plaques compared to Y280, WF10, GLW, and MSLAB01 (Figures 2B–H).
Figure 2. A/Suzhou/GIRD01/2019 (H9N2) replicated to high titres in mammalian cells and showed greater peak viral titres compared to older prototypical H9N2 avian influenza viruses and contemporary seasonal viruses. (A) Peak viral titres of A/Suzhou/GIRD01/2019 (H9N2) (GIRD01) and A/Chicken/Zhejiang/198/2019 (H9N2) (ZJ/198) were greater compared to the older H9N2 viruses A/Guinea fowl/Hong Kong/WF10/1999 (H9N2) (WF10) and A/Duck/Hong Kong/Y280/1997 (H9N2) (Y280) and the contemporary seasonal viruses A/China/GLW/2018 (H1N1) (GLW) and A/Guangzhou/MSLAB01/2017 (MSLAB01) in MDCK cells. Black, grey and purple asterisks indicate statistically significant differences between GIRD01 and Y280, WF10 and GLW, respectively (B–H) Plaque diameters produced by GIRD01 was significantly greater compared to plaques produced by WF10, Y280, GLW and MSLAB01, but not ZJ/198. Data points in (A) are the mean ± the standard error of the mean. Data points in (B) are individual plaque diameters. ***p < 0.001, **p < 0.01, *p < 0.05.
We next studied the receptor binding preferences of GIRD01 for “avian-type” and “mammalian-type” (α2,3 and α2,6-linked, respectively) sialic acids (Figure 3A). At the higher concentrations of sialylglycopolymers, GIRD01 bound most strongly to 6'SL and 3'SLN sialic acids. However, at sialylglycopolymer concentrations ≤9.38μg/mL, GIRD01 showed relatively strong binding to 6'SLN, the longer α2,6-linked moiety, and almost undetectable binding to the other sialylglycopolymers, with an association constant (Ka) of 0.1178μM−1 for 6'SLN compared to 0.0065, 0.0090, and 0.0113μM−1 for 3'SL, 6'SL, and 3'SLN sialylglycopolymers, respectively (Figure 3B). These data indicate that GIRD01 could bind to both avian- and mammalian-type sialic acids but showed the strongest affinity for the longer, 6'SLN sialylglycopolymer.
Figure 3. A/Suzhou/GIRD01/2019 (H9N2) bound with the highest affinity to a mammalian-type α2,6-linked sialylglycopolymers and showed similar binding preferences to A/chicken/Zhejiang/198/2019 (H9N2). A/Suzhou/GIRD01/2019 (H9N2) (GIRD01) bound to α2,3- and α2,6-linked sialylglycopolymer (A) but showed the highest affinity to the longer α2,6SLN (association constants (Ka) of 0.0065, 0.0090, 0.0113, and 0.1178 μM−1 for 3'SL, 6'SL, 3'SLN, and 6'SLN sialylglycopolymers, respectively) (B). Binding of GIRD01 was similar to that of A/chicken/Zhejiang/198/2019 (H9N2) (ZJ/198) for all sialylglycopolymers studies here (C–F). A/duck/Hong Kong/Y280/1999 (H9N2) (Y280) showed similar binding affinity as GIRD01 for α2,3-linked sialylglycopolymers (C,E) but showed greater affinity for α2,6-linked sialylglycopolymers compared to GIRD01 and ZJ/198 (D,F). A/guinea fowl/Hong Kong/WF10/1999 (H9N2) (WF10) showed the highest affinity for all sialic acids moieties studies here (C–F). Graphs show the mean ± the standard error of the mean calculated from three independent experiments. *p < 0.05, **p < 0.01, ***p < 0.001.
Overall we found no significant differences in receptor binding preferences or affinity between GIRD01 and ZJ/198. Both viruses could bind to the avian and mammalian-type sialic acids used in this study (Figures 3C–F). The binding of GIRD01, ZJ/198, and Y280 to α2,3-linked sialic acids were similar (Figures 3C,E). Interestingly, considering the replication kinetics of these viruses in mammalian cells, the binding affinity of the GIRD01 and ZJ/198 to α2,6-linked sialic acids was less than the older H9 viruses used in this study, Y280 and WF10 (Figures 3D,F).
As GIRD01 was able to infect humans, we next assessed whether this virus was susceptible to the neuraminidase inhibitors oseltamivir and zanamivir. GIRD01 was susceptible to both oseltamivir and zanamivir, with EC50 values calculated as 5.52 ± 1.69 and 5.45 ± 0.44 μM, respectively (Figure 4). The susceptibility of GIRD01 to oseltamivir and zanamivir were similar to ZJ/198, Y280, and WF10 (Figure 4).
Figure 4. A/Suzhou/GIRD01/2019 (H9N2) was susceptible to the neuraminidase inhibitors oseltamivir and zanamivir. A/Suzhou/GIRD01/2019 (H9N2) (GIRD01) was susceptible to oseltamivir (A) and zanamivir (B) (mean EC50 of 5.52 ± 1.69 and 5.45 ± 0.44 μM, respectively). The susceptibility of GIRD01 to oseltamivir and zanamivir were similar to those of A/chicken/Zhejiang/198/2019 (H9N2) (ZJ/198), A/guinea fowl/Hong Kong/WF10/1999 (H9N2) (WF10) and A/duck/Hong Kong/Y280/1999 (H9N2) (Y280). Data points represent the mean ± the standard error of the mean of three replicates.
In the mouse model, GIRD01, Y280, and WF10 did not cause weight loss or mortality. ZJ/198 caused weight loss but not mortality and all mice inoculated with 104 PFU of PR8, a relatively pathogenic IAV used as control, were euthanised, having reached humane endpoints (Figures 5A,B). Whilst the weight loss curves determined for mice inoculated with 102 PFU of PR8 and ZJ/198 were similar, those mice inoculated with ZJ/198 did not display as severe symptoms as those inoculated with PR8 and recovered. HAI assays performed on serum collected from these mice at 28 days post inoculation revealed the presence of specific antibodies against the viruses used to inoculate these mice, thus confirming that all mice were infected (Figure 5C). Overall, GIRD01 was of low pathogenicity in the mouse model.
Figure 5. A/Suzhou/GIRD01/2019 (H9N2) was of low pathogenicity in the mouse model. Six- to eight-week-old BALB/c mice (n = 8 mice per virus) were intranasally inoculated with 106 plaque forming units (PFU) of A/Suzhou/GIRD01/2019 (H9N2) (GIRD01), A/chicken/Zhejiang/198/2019 (H9N2) (ZJ/198), A/duck/Hong Kong/Y280/1999 (H9N2) (Y280), or A/guinea fowl/Hong Kong/WF10/1999 (H9N2) (WF10). Three additional groups were inoculated with 104 or 102 PFU of A/Puerto Rico/8/1934 (H1N1) (PR8) or PBS as a control. Mice inoculated with GIRD01, Y280 or WF10 did not lose weight, whilst mice inoculated with ZJ/198 or PR8 at 102 PFU lost 24.81±1.33% and 27.30±2.20% of their body weight by day eight post inoculation, respectively (A). All mice inoculated with PR8 at 104 PFU reached humane endpoints by day six post inoculation (A,B). All mice inoculated with GIRD01, ZJ/198, Y280, and WF10 survived and 25% of mice inoculated with 102 PFU of PR8 survived (B). Mice inoculated with PBS survived and did not lose weight (A,B). Hemagglutination inhibition assays performed using mouse serum obtained 28 days post inoculation revealed the presence of specific antibodies against viruses used to inoculate respective animals (C). Data points in (A) represent the mean ± the standard error of the mean. Data points in (C) are individual mice. Means ± the standard error of the means are also shown.
H9N2 AIV human infections are generally associated with uncomplicated, and self-limiting ILI. Here, we report a case of H9N2 infection that required intensive care and aggressive clinical management. The detection of viral RNA in respiratory swabs confirmed the presence of H9N2 AIV in the upper respiratory tract while viral RNA in rectal swabs was suggestive of spread to extrapulmonary sites. Detection of IAV RNA in faecal samples has been reported in cases of seasonal influenza although the clinical significance of this is unknown and, as observed in our study, gastrointestinal symptoms were not always present (19, 20). In human cases of the highly-pathogenic subtype H5N1 AIV, viral RNA was often detected in critically ill or terminal patients (21). The human gut tissue expresses both α2,3- and α2,6-linked sialic acids and could therefore support replication of AIVs, particularly viruses like GIRD01 that exhibit binding to both moieties (22).
RSV was also detected by immunofluorescence in a single BALF sample obtained from this patient, however, as we were unable to obtain sequence data or isolate the virus, we could not confirm the presence of RSV. As RSV causes severe disease in those <2 years of age and infections in older children rarely result in complications, the contribution of RSV to the disease severity observed is unclear (23). Further, it is not known if RSV was contracted prior to or following hospitalisation. However, it cannot be ruled out that RSV may have contributed to the severity of disease observed in this patient. Evidence for co-infection with seasonal influenza and RSV leading to severe disease is conflicting and most studies have focused on children younger than 2 years of age (24, 25). Further, we are uncertain how applicable clinical findings from cases of RSV/seasonal influenza virus co-infections are to AIVs. Notably, another severe H9N2 case was reported recently in a 14-month-old female in Oman (26). Along with IAV, this patient was also tested positive for human rhinovirus and parechovirus in her nasopharyngeal swab. This and our report suggest that co-infection with other respiratory viruses may exacerbate H9N2 infections.
Although GIRD01 contained known markers of mammalian replication and pathogenicity, it is unclear whether these markers were present in the virus prior to human infection or if they were acquired during replication in the human host. However, these markers were shared with ZJ/198, suggesting that these mammalian pathogenicity markers could be present in circulating poultry H9N2 AIVs. Despite the presence of these markers, GIRD01 did not cause significant morbidity in mice and, surprisingly, was less pathogenic than ZJ/198. This is similar to a previous study that reported different pathogenicity profiles for two viruses that were highly similar genetically (27). One caveat to our study is that, unlike the highly-pathogenic H5N1 AIVs, whose mammalian pathogenicity can be somewhat recapitulated using mice and ferret models, H9N2 AIVs have not typically caused severe infections in humans. Therefore, it is unknown to what degree standard influenza animal models will accurately reflect the mammalian pathogenicity profiles of H9N2 AIVs (28). Within this context, we can only surmise that GIRD01, unlike ZJ/198, can successfully infect mice without causing significant morbidity. However, as GIRD01 and ZJ/198 replicated more efficiently compared to human IAVs in mammalian cell lines and displayed binding to mammalian-type sialic acids, they are a cause for concern.
In summary, our virology data showed that GIRD01 was less pathogenic compared to ZJ/198 but both viruses showed mammalian receptor binding preferences and enhanced replication kinetics in MDCK cells compared to older H9N2 viruses. While GIRD01 was not more virulent than ZJ/198, it displayed a binding preference for mammalian-type sialic acids and robust replication in mammalian cells, indicating that currently circulating H9N2 AIVs pose a risk of zoonoses to humans.
The original contributions presented in the study are included in the article/supplementary material, further inquiries can be directed to the corresponding author/s.
The studies involving human participants were reviewed and approved by Ethics committee of Suzhou University. Written informed consent from the participants' legal guardian/next of kin was not required to participate in this study in accordance with the national legislation and the institutional requirements. The animal study was reviewed and approved by Animal Care & Welfare Committee of The First Affiliated Hospital of Guangzhou Medical University.
MZ, S-SW, ZY, and CH: conceptualisation. MZ, MD, S-SW, and WS: methodology. FZ and MZ: software, formal analysis, writing—original draught preparation, and visualisation. MZ: validation, writing—review and editing, and project administration. LC, JH, YX, ZD, ZH, TL, and ZL: investigation. YW, XZ, ZB, JY, and ZW: resources. FZ, ZC, and WG: data curation. MZ, S-SW, and CH: supervision. MZ and S-SW: funding acquisition. All authors read and approved the final manuscript.
Funding for this study was provided by Open Project funding mechanism of the State Key Laboratory of Respiratory Diseases, The Department of Education of the Guangdong Provincial Government, The National Institutes of Health of the USA and the National Natural Science Foundation of China (Grant No. 81761128014), The Jiangsu Natural Science Fund (Grant No. SBK2020043364), and The Priority Academic Program Development of Jiangsu Higher Education Institutions.
The authors declare that the research was conducted in the absence of any commercial or financial relationships that could be construed as a potential conflict of interest.
All claims expressed in this article are solely those of the authors and do not necessarily represent those of their affiliated organizations, or those of the publisher, the editors and the reviewers. Any product that may be evaluated in this article, or claim that may be made by its manufacturer, is not guaranteed or endorsed by the publisher.
We gratefully acknowledge the contributions of the staff of Suzhou Children's Hospital, the Suzhou Centres for Disease Control and Prevention and Professor Xiu-Feng Wan of The University of Missouri.
1. Bi Y, Li J, Li S, Fu G, Jin T, Zhang C, et al. Dominant subtype switch in avian influenza viruses during 2016-2019 in China. Nat Commun. (2020) 11:5909. doi: 10.1038/s41467-020-19671-3
2. Guo Y, Li J, Cheng X. [Discovery of men infected by avian influenza A (H9N2) virus]. Zhonghua Shi Yan He Lin Chuang Bing Du Xue Za Zhi. (1999) 13:105–8.
3. Peacock THP, James J, Sealy JE, Iqbal M. A global perspective on H9N2 avian influenza virus. Viruses. (2019) 11:620. doi: 10.20944/preprints201906.0085.v1
4. World Health Organization. Influenza at the Human-Animal Interface: Summary and Assessment, 20 July to 3 October 2016. Geneva (2016)
5. Centers for Disease Control and Prevention. Influenza Risk Assessment Tool (Atlanta, GA: IRAT) (2020).
6. Reed LJ, Muench H. A simple method of estimating fifty percent endpoints. Am J Hygiene. (1938) 27:493–97. doi: 10.1093/oxfordjournals.aje.a118408
7. Matrosovich MN, Gambaryan AS. Solid-phase assays of receptor-binding specificity. Methods Mol Biol. (2012) 865:71–94. doi: 10.1007/978-1-61779-621-0_5
8. Gubareva LV, Webster RG, Hayden FG. Detection of influenza virus resistance to neuraminidase inhibitors by an enzyme inhibition assay. Antiviral Res. (2002) 53:47–61. doi: 10.1016/S0166-3542(01)00192-9
9. Potier M, Mameli L, Belisle M, Dallaire L, Melancon SB. Fluorometric assay of neuraminidase with a sodium (4-methylumbelliferyl-alpha-D-N-acetylneuraminate) substrate. Anal Biochem. (1979) 94:287–96. doi: 10.1016/0003-2697(79)90362-2
10. Sun Y, Pu J, Jiang Z, Guan T, Xia Y, Xu Q, et al. Genotypic evolution and antigenic drift of H9N2 influenza viruses in China from 1994 to 2008. Vet Microbiol. (2010) 146:215–5. doi: 10.1016/j.vetmic.2010.05.010
11. Butt KM, Smith GJ, Chen H, Zhang LJ, Leung YH, Xu KM, et al. Human infection with an avian H9N2 influenza A virus in Hong Kong in 2003. J Clin Microbiol. (2005) 43:5760–7. doi: 10.1128/JCM.43.11.5760-5767.2005
12. Guan Y, Shortridge KF, Krauss S, Webster RG. Molecular characterization of H9N2 influenza viruses: were they the donors of the “internal” genes of H5N1 viruses in Hong Kong? Proc Natl Acad Sci USA. (1999) 96:9363–7. doi: 10.1073/pnas.96.16.9363
13. Li X, Shi J, Guo J, Deng G, Zhang Q, Wang J, et al. Genetics, receptor binding property, and transmissibility in mammals of naturally isolated H9N2 Avian Influenza viruses. PLoS Pathog. (2014) 10:e1004508. doi: 10.1371/journal.ppat.1004508
14. Wan H, Perez DR. Amino acid 226 in the hemagglutinin of H9N2 influenza viruses determines cell tropism and replication in human airway epithelial cells. J Virol. (2007) 81:5181–91. doi: 10.1128/JVI.02827-06
15. Wan H, Sorrell EM, Song H, Hossain MJ, Ramirez-Nieto G, Monne I, et al. Replication and transmission of H9N2 influenza viruses in ferrets: evaluation of pandemic potential. PLoS ONE. (2008) 3:e2923. doi: 10.1371/journal.pone.0002923
16. Sealy JE, Yaqub T, Peacock TP, Chang P, Ermetal B, Clements A, et al. Association of increased receptor-binding avidity of influenza A(H9N2) viruses with escape from antibody-based immunity and enhanced zoonotic potential. Emerg Infect Dis. (2018) 25:63–72. doi: 10.3201/eid2501.180616
17. Yamada S, Suzuki Y, Suzuki T, Le MQ, Nidom CA, Sakai-Tagawa Y, et al. Haemagglutinin mutations responsible for the binding of H5N1 influenza A viruses to human-type receptors. Nature. (2006) 444:378–82. doi: 10.1038/nature05264
18. Yang W, Punyadarsaniya D, Lambertz RLO, Lee DCC, Liang CH, Hoper D, et al. Mutations during the adaptation of H9N2 avian influenza virus to the respiratory epithelium of pigs enhance sialic acid binding activity and virulence in mice. J Virol. (2017) 91:e02125-16. doi: 10.1128/JVI.02125-16
19. Chan MC, Lee N, Chan PK, Leung TF, Sung JJ. Fecal detection of influenza A virus in patients with concurrent respiratory and gastrointestinal symptoms. J Clin Virol. (2009) 45:208–11. doi: 10.1016/j.jcv.2009.06.011
20. Wootton SH, Scheifele DW, Mak A, Petric M, Skowronski DM. Detection of human influenza virus in the stool of children. Pediatr Infect Dis J. (2006) 25:1194–5. doi: 10.1097/01.inf.0000245097.95543.11
21. de Jong MD, Simmons CP, Thanh TT, Hien VM, Smith GJ, Chau TN, et al. Fatal outcome of human influenza A (H5N1) is associated with high viral load and hypercytokinemia. Nat Med. (2006) 12:1203–7. doi: 10.1038/nm1477
22. Shu Y, Li CK, Li Z, Gao R, Liang Q, Zhang Y, et al. Avian influenza A(H5N1) viruses can directly infect and replicate in human gut tissues. J Infect Dis. (2010) 201:1173–7. doi: 10.1086/651457
23. Zhang T, Zhu Q, Zhang X, Ding Y, Steinhoff M, Black S, et al. Clinical characteristics and direct medical cost of respiratory syncytial virus infection in children hospitalized in Suzhou, China. Pediatr Infect Dis J. (2014) 33:337–41. doi: 10.1097/INF.0000000000000102
24. Harada Y, Kinoshita F, Yoshida LM, Minh le N, Suzuki M, Morimoto K, et al. Does respiratory virus coinfection increases the clinical severity of acute respiratory infection among children infected with respiratory syncytial virus? Pediatr Infect Dis J. (2013) 32:441–5. doi: 10.1097/INF.0b013e31828ba08c
25. Yoshida LM, Suzuki M, Nguyen HA, Le MN, Dinh Vu T, Yoshino H, et al. Respiratory syncytial virus: co-infection and paediatric lower respiratory tract infections. Eur Respir J. (2013) 42:461–9. doi: 10.1183/09031936.00101812
26. Almayahi ZK, Al Kindi H, Davies CT, Al-Rawahi B, Al-Jardani A, Al-Yaqoubi F, et al. First report of human infection with avian influenza A(H9N2) virus in Oman: the need for a One Health approach. Int J Infect Dis. (2020) 91:169–73. doi: 10.1016/j.ijid.2019.11.020
27. Liu Q, Liu Y, Yang J, Huang X, Han K, Zhao D, et al. Two genetically similar H9N2 influenza A viruses show different pathogenicity in mice. Front Microbiol. (2016) 7:1737. doi: 10.3389/fmicb.2016.01737
Keywords: influenza virus, H9N2, zoonosis, poultry, respiratory disease
Citation: Zhao F, Wang Y, Chen L, Zhang X, Ducatez M, He J, Wan Z, Ye J, Bai Z, Xia Y, Dong Z, Gu W, Huang Z, Liang T, Lin Z, Song W, Chen Z, Yang Z, Wong S-S, Hao C and Zanin M (2021) Critical Influenza-Like Illness in a Nine-Year-Old Associated With a Poultry-Origin H9N2 Avian Influenza Virus: Risk Assessment and Zoonotic Potential. Front. Virol. 1:727163. doi: 10.3389/fviro.2021.727163
Received: 18 June 2021; Accepted: 02 August 2021;
Published: 06 September 2021.
Edited by:
Danilo Bretas De Oliveira, Universidade Federal dos Vales do Jequitinhonha e Mucuri, BrazilReviewed by:
Pedro Augusto Alves, Oswaldo Cruz Foundation (Fiocruz), BrazilCopyright © 2021 Zhao, Wang, Chen, Zhang, Ducatez, He, Wan, Ye, Bai, Xia, Dong, Gu, Huang, Liang, Lin, Song, Chen, Yang, Wong, Hao and Zanin. This is an open-access article distributed under the terms of the Creative Commons Attribution License (CC BY). The use, distribution or reproduction in other forums is permitted, provided the original author(s) and the copyright owner(s) are credited and that the original publication in this journal is cited, in accordance with accepted academic practice. No use, distribution or reproduction is permitted which does not comply with these terms.
*Correspondence: Chuangli Hao, aGNsX21kQDEzNi5jb20=; Mark Zanin, bWFyay56YW5pbkBHSVJELmNu
†These authors have contributed equally to this work
Disclaimer: All claims expressed in this article are solely those of the authors and do not necessarily represent those of their affiliated organizations, or those of the publisher, the editors and the reviewers. Any product that may be evaluated in this article or claim that may be made by its manufacturer is not guaranteed or endorsed by the publisher.
Research integrity at Frontiers
Learn more about the work of our research integrity team to safeguard the quality of each article we publish.