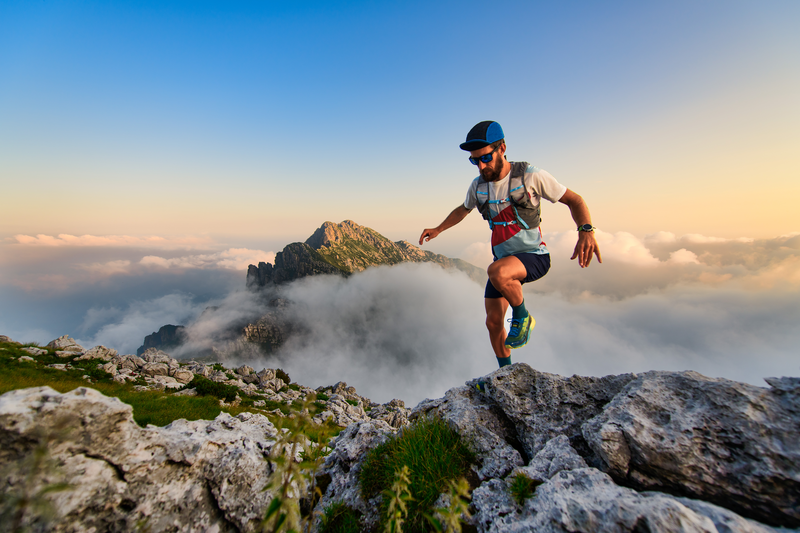
94% of researchers rate our articles as excellent or good
Learn more about the work of our research integrity team to safeguard the quality of each article we publish.
Find out more
ORIGINAL RESEARCH article
Front. Vet. Sci.
Sec. Animal Nutrition and Metabolism
Volume 12 - 2025 | doi: 10.3389/fvets.2025.1573555
The final, formatted version of the article will be published soon.
You have multiple emails registered with Frontiers:
Please enter your email address:
If you already have an account, please login
You don't have a Frontiers account ? You can register here
Background: Holstein bull calves were given a one-time intraperitoneal injection of Diquat to explore the effects of Diquat on growth , body frame, blood oxidation indices, and fecal scores and pathogenic bacteria in weaned calves.Methods: Twelve 70-d Holstein bull calves of similar body weight (BW) and body condition were randomly assigned to 1 of 4 treatments. Treatments were: Control: calves were injected with 0 mg/kg BW Diquat in 0.9 % sterilized saline; treatment: 6, 8 and 10 mg/kg BW; Diquat, respectively. The experimental period was 24 days. Measurements of BW, average daily gain (ADG), faecal scores, frame gains, fecal pathogen count, and blood samples for monitoring oxidative stress were collected at 0, 6, 12, 18 and 24 d. Data were analysed as a randomized complete block design with d as a repeated measurement. In addition, exponential polynomial contrasts were used to test the linear, quadratic, and cubic treatment responses.Results: Growth performance (BW), and ADG demonstrated a cubic response (P < 0.02) by decreasing then increasing with increasing Diquat dosage. Faecal scores and faecal ratios demonstrated a quadratic response (P < 0.02) by increasing at a decreasing rate with increasing Diquat injection dosage. Frame gains for body slope, body length, hip heights, and abdominal girth demonstrated a linear (P < 0.03) decrease with increasing Diquat injection dosage. Serum aspartate aminotransferase, glutathione, total antioxidant capacity, catalase, malondialdehyde, cortisol, and noradrenaline concentrations demonstrated a linear increase (P < 0.01) with increasing Diquat injection dosage, while alanine transaminase, superoxide oxide dismutase, and glutathione peroxidase demonstrated a quadratic response (P < 0.02) by increasing at a decreasing rate. Faecal Escherichia coli concentrations demonstrated a cubic response (P < 0.01), while Staphylococcus aureus and Salmonella-shigella demonstrated linear increases (P < 0.01) with increasing Diquat dosage.Conclusions: Diquat injection caused oxidative stress, resulting in reduced growth performance, with increasing indices of serum oxidative stress, faecal scores, and faecal pathogens, a response that can last for up to 24 days. 8 mg/kg BW being the optimal dosage as a target dosage for elucidating oxidative stress for evaluating future technologies to reduce, eliminate, or prevent oxidative stress.
Keywords: Diquat, Oxidative Stress, Weaned calves, Serum antioxidant, Fecal score;
Received: 09 Feb 2025; Accepted: 24 Feb 2025.
Copyright: © 2025 Liu, Chen, CaiRang, Cheng, Luo, Zhang and Casper. This is an open-access article distributed under the terms of the Creative Commons Attribution License (CC BY). The use, distribution or reproduction in other forums is permitted, provided the original author(s) or licensor are credited and that the original publication in this journal is cited, in accordance with accepted academic practice. No use, distribution or reproduction is permitted which does not comply with these terms.
* Correspondence:
Ting Liu, College of Animal Science and Technology, Gansu Agricultural University, Lanzhou, Gansu Province, China
Disclaimer: All claims expressed in this article are solely those of the authors and do not necessarily represent those of their affiliated organizations, or those of the publisher, the editors and the reviewers. Any product that may be evaluated in this article or claim that may be made by its manufacturer is not guaranteed or endorsed by the publisher.
Research integrity at Frontiers
Learn more about the work of our research integrity team to safeguard the quality of each article we publish.