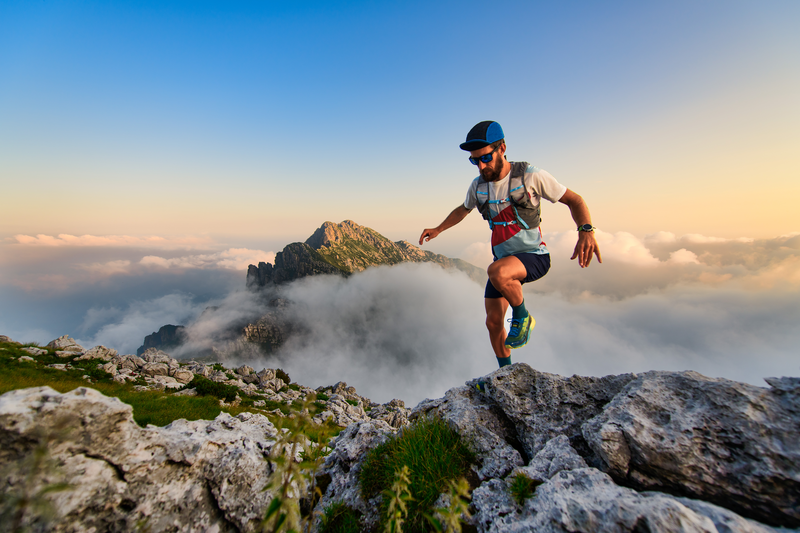
94% of researchers rate our articles as excellent or good
Learn more about the work of our research integrity team to safeguard the quality of each article we publish.
Find out more
ORIGINAL RESEARCH article
Front. Vet. Sci.
Sec. Veterinary Infectious Diseases
Volume 12 - 2025 | doi: 10.3389/fvets.2025.1570575
The final, formatted version of the article will be published soon.
You have multiple emails registered with Frontiers:
Please enter your email address:
If you already have an account, please login
You don't have a Frontiers account ? You can register here
African swine fever is a devastating viral disease of swine causing up to 100% mortality and significant impacts to the swine industry. The causative agent, African swine fever virus (ASFV), is a large, enveloped virus containing a linear, double-stranded DNA genome with 170-190 kb in length. Since its introduction into the Caucasus region in 2007, the genotype II ASFV has continued to spread to Europe, Asia, and Caribbean countries. Early detection is crucial to prevent and control ASF outbreaks for biosecurity purposes, and environmental samples can be used to evaluate the level of biosecurity. Therefore, we evaluated the effect of freeze-thaw cycles and storage at 4 °C and room temperature (RT) on ASFV DNA detection in environmental samples. ASFV DNA was stable in environmental samples with no organic contaminants after freeze-thaw and incubation at 4 °C and RT. However, incubation at RT negatively affects ASFV detection in swine feces and feed dust samples that were collected using premoistened gauze. There were significant reductions in ASFV detection in environmental samples in the presence of soil and organic mixture after freeze-thaw and incubation at 4 °C and RT. These results provide novel insights on the appropriate storage of environmental samples for ASFV detection and contribute to the control and prevention of ASF outbreaks and new introductions.
Keywords: African Swine Fever, ASFV, Environmental sample, freeze-thaw, Fomite, surface, storage
Received: 03 Feb 2025; Accepted: 17 Mar 2025.
Copyright: © 2025 Kwon, Gebhardt, Lyoo, Gaudreault, Trujillo and Richt. This is an open-access article distributed under the terms of the Creative Commons Attribution License (CC BY). The use, distribution or reproduction in other forums is permitted, provided the original author(s) or licensor are credited and that the original publication in this journal is cited, in accordance with accepted academic practice. No use, distribution or reproduction is permitted which does not comply with these terms.
* Correspondence:
Jordan Gebhardt, Kansas State University, Manhattan, United States
Juergen Richt, Kansas State University, Manhattan, United States
Disclaimer: All claims expressed in this article are solely those of the authors and do not necessarily represent those of their affiliated organizations, or those of the publisher, the editors and the reviewers. Any product that may be evaluated in this article or claim that may be made by its manufacturer is not guaranteed or endorsed by the publisher.
Research integrity at Frontiers
Learn more about the work of our research integrity team to safeguard the quality of each article we publish.