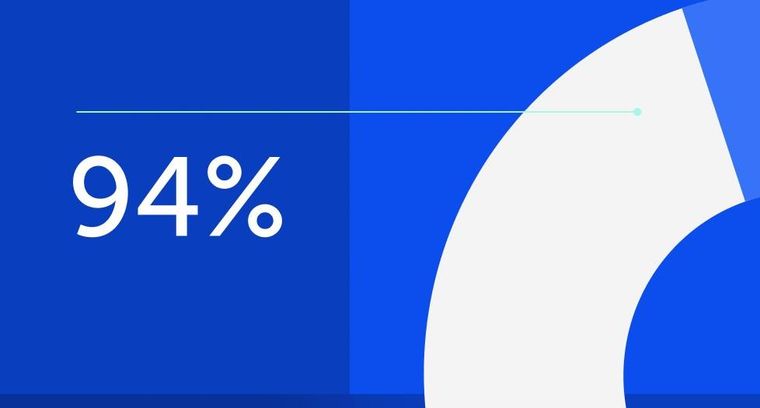
94% of researchers rate our articles as excellent or good
Learn more about the work of our research integrity team to safeguard the quality of each article we publish.
Find out more
ORIGINAL RESEARCH article
Front. Vet. Sci., 25 March 2025
Sec. Comparative and Clinical Medicine
Volume 12 - 2025 | https://doi.org/10.3389/fvets.2025.1570564
This article is part of the Research TopicBiomarkers of Health and Disease in Veterinary Science - Volume IIView all articles
The present study evaluated serum cortisol levels as a stress indicator in camels and analyzed the influence of some physiological and pathological factors on cortisol levels and their relationship with immunological parameters. A total number of 169 camels (Camelus dromedarius) were grouped in a healthy group (n = 106 camels), a slaughterhouse group (n = 20 camels), a Surra-affected group (n = 27 camels), and a metritis-affected group (n = 16 camels). Female camels exhibited higher cortisol levels compared to males, with non-pregnant and lactating she-camels showing elevated levels relative to their pregnant and non-lactating counterparts. No significant differences in cortisol levels were observed among camel breeds (Majaheem, Magateer, Sawahli, and Omani). Elevated cortisol levels were observed in stressed camels (pre-slaughter) and diseased camels, including those with Surra and bacterial metritis, confirming the reliability of cortisol as a stress marker in this species. Increased cortisol levels were associated with leukocytosis, neutrophilia, and a higher neutrophil-to-lymphocyte ratio. Phenotypically, elevated cortisol level was associated with an expanded CD4 T-cell population, reduced γδ T cells percentage, decreased CD172a expression on neutrophils and monocytes, reduced CD14 and CD163 expression on monocytes, and enhanced CD45 and MHC I expression on lymphocytes. Functionally, higher cortisol levels were linked to increased reactive oxygen species (ROS) production in blood phagocytes. These findings highlight the modulatory effects of cortisol on the camel immune system and emphasize the importance of considering gender and reproductive status when evaluating stress in camels.
Early detection of stress is one of the most effective strategies to counteract stress-induced disorders in humans and animals. Various markers have been identified as potent indicators of stress including endocrine changes leading to elevated levels of hormones, changes in blood levels of heat shock proteins, acute phase proteins, lipid peroxidation markers, and microRNAs (1, 2). High circulating level of cortisol has been identified as one of the most reliable indicators of stress in several species (1, 3).
The most common factors that are responsible for stress in livestock include road transportation, type of the production and housing system (extensive or intensive, social mixing or regrouping of animals), and environmental stress such as heat stress (3–9). In addition, several physiological factors such as breed, gender, age, pregnancy, lactation, and parturition were found associated with variations in serum cortisol levels in different species (10–14).
In camels, Saeb et al. reported a serum cortisol level between 26 and 40 nmol/L (9.4 and 14.5 ng/mL) in healthy animals (15). Other studies, however, reported lower concentration (3.8 nmol/L) of plasma cortisol in healthy camels (16). Elevated cortisol levels have been found indicative for different stressful situations in camels including road transportation and long-term dehydration (13, 15–17). Animal management practices at slaughterhouses, such as type and duration of animals loading and transportation are among the stressful situations resulting in increased serum cortisol levels in camels.
Stress response in animals is regulated by the hypothalamic–pituitary–adrenal (HPA) axis that regulates the secretion of cortisol (18). Stress-induced activation of HPA axis results in the release of corticotrophin-releasing factor by hypothalamus which stimulates the adenohypophysis to secrete adrenocorticotropic hormone (ACTH) in blood circulation which in turn stimulates the secretion of cortisol by the adrenal gland. As a result, the immune system gets sensitized and responds immediately to the peripheral stress through several changes in humoral and cellular immune parameters (19). Studies in cattle reported a marked stress-associated leukocytosis and neutrophilia with a decrease in lymphocyte numbers and shifts in their composition (20). Especially, the decrease in T cell numbers with their reduced blastogenic and cytokine production response have been linked to higher susceptibility to infectious diseases in stressed cattle (4, 20, 21).
Studies on stress indicators in camels and their immune response to stress are scarce. The present study was, therefore, undertaken to investigate the impact of selected physiological and pathological factors on serum cortisol levels in camels. In addition, the study aimed at the analysis of the association between high cortisol levels in camels and changes in some phenotypic and functional immune parameters.
The study was conducted between July and November 2024. Some samples were, however, collected during the years 2020 to 2023. A total number of 169 camels (Camelus dromedarius) were involved in the present study. The animals included a healthy group (H), a slaughterhouse group (SL), a Surra group (S), and a metritis group (ME). The healthy group included 106 clinically healthy camels including 88 female and 18 male camels aged between 0.5 and 13 years (mean age 6.6 years). The female camel group contained 30 non-pregnant she-camels and 58 pregnant she-camels from the 3rd to 7th month. The camels were selected from different private camel farms in the eastern and middle region of Saudi Arabia as healthy animals without any signs of disease based on clinical examination by a trained veterinarian. The slaughterhouse group included 20 clinically healthy male camels (2 years old) selected from the animals that were admitted for normal slaughtering at Al-Omran Slaughterhouse (Eastern Province, Saudi Arabia). The Surra-affected camel group included 27 animals (23 females and 4 males) aged between 2 and 15 years (mean age 5.8 years). The clinical metritis group included 16 she-camels aged between 6 and 16 years (mean age 10.3 years). The serum samples related to the Surra-affected and the metritis-affected groups were from two previously published studies (22, 23), and were retrospectively analyzed for serum cortisol levels. Detailed information for animal age, breed, gender, lactation and pregnancy status are described in (Supplementary Table 1). All farms included in the study used similar nutritional and production practices. For all farms, camels were kept under a traditional management system, where they grazed during the daytime. At night, the camels were housed in a fen barn and fed on hay and barley in addition to bread and dates with water provided ad libitum.
Blood samples were collected from the jugular vein in EDTA-containing tubes (for cellular analysis) or serum collection tubes (for cortisol measurement) and transported within 1–2 h to the laboratory. All tubes were from Guangzhou Improve Medical Instruments Co., Ltd. (Guangzhou, China). Serum collection was performed by centrifugation of blood at 1,000 × g for 15 min at room temperature. Blood sampling from the healthy and slaughterhouse groups was performed between July and October 2024 and separated serum samples were stored at −80°C until analysis. For the metritis- and Surra-affected animals, blood samples were collected between January and June from 2020 to 2023 and separated serum samples were stored at −80°C until analysis. For all animals, the blood sampling time was during the morning time between 7:00 and 9:00 am.
Quantitative determination of cortisol in serum was performed using a commercial enzyme immunoassay (DRG® Cortisol ELISA Kit), according to the guidelines of the manufacturer (The, DRG, Springfield, NJ 07081 USA). For this, 20 μl serum sample was added together with 100 μl of the kit conjugate (horseradish peroxidase-conjugated cortisol) followed by incubation for 1 h at room temperature. During this time, sample Cortisol will compete with the conjugated cortisol for binding to the plate-bound antibody. After incubation, the plate was washed three times using ELISA washing buffer followed by adding 100 μl of 3,3′,5,5′-Tetramethylbenzidine (TMB) ready to use substrate and chromogen solution. After 20 min of incubation at room temperature in the dark, the reaction was stopped by the addition of 100 μl of the stop solution (0.16 M H2SO4). Finally, the optical density values, which are inversely proportional to the concentration of Cortisol in the sample, were measured using ELISA reader (iMark Bio-Rad laboratories). For each plate, a standard curve was prepared from different standard Cortisol concentrations. The assay has a sensitivity of 1.0 ng/ml (6.0 nmo/L) and a detection range between 1.3 and 800 ng/ml. Sample Cortisol concentrations were calculated using a standard semi-logarithmic curve fit.
Blood leukocytes were separated by hypotonic lysis of erythrocytes followed by centrifugation as previously described. Briefly, EDTA blood (1 ml) was incubated for 20 s with 5 ml distilled water in a 15 ml sterile falcon tube to induce red blood cell lysis. Subsequently, tonicity was restored by the addition of 5 ml 2× PBS, and the tube was centrifuged at 3000 RPM for 10 min at 10°C. For complete removal of red blood cells, the lysis step was repeated twice followed by centrifugation at 2,200 and 1,500 RPM for 10 min each round. Finally, the pellet was resuspended in cold PBS and adjusted to 1 × 106 cell/ml. Cell vitality was measured by the addition of propidium iodide and was always above 93%.
Separated leukocytes were labeled with monoclonal antibodies (mAbs) to the leukocyte antigens CD45, CD44, CD11a, major histocompatibility (MHC) class-I, MHC class II, BAQ44A (B cell), WC1 (γδ T cell), CD4 (helper T cell), CD172a, CD14, and CD163 (24, 25). In the first staining step, cells (1 × 106 cell/well) were incubated in a 96-well plate with the primary mAbs for 15 min at 4°C. After washing with PBS/BSA buffer, the second staining step was done by incubating the cells with fluorochrome-labeled antibodies to mouse IgM, IgG1, and IgG2a (Invitrogen). Finally, the cells were washed and analyzed by flow cytometry (Becton Dickinson Accuri C6 flow cytometer; Becton Dickinson Biosciences, San Jose, California, USA).
The level of reactive oxygen species (ROS) in camel neutrophils and monocytes was analyzed using the ROS-dye dehydrorohdamin-123 (DHR-123) and flow cytometry. Leukocytes (1 × 106 in 100 μl RPMI medium) were loaded with DHR-123 for 30 min at 37°C and 5% CO2. Stained cells were analyzed by flow cytometry (Accuri C6 flow cytometer; BD Biosciences). Baseline ROS level was measured based on the increase in the green fluorescence (mean FL-1) of neutrophils and monocytes.
Means and standard error of the mean (SEM) were calculated using the column statistic function of the Prism software (GraphPad). Data normality was calculated using the Shapiro–Wilk test. The comparison between the four camel breeds was performed using the Kruskal-Wallis one-way analysis-of-variance (ANOVA) in combination with Dunn’s post hoc test. The comparison between values based on pregnancy and lactation was done using unpaired student’s t test. The impact of gender on serum cortisol level was statistically analyzed using Mann–Whitney U test (not normally distributed values). In addition, the Common Language Effect Size (CLES) was calculated based on Cohen’s d using an online calculation tool1 and was presented alongside the p-values. Although both male and female camels from different ages and breeds were represented in the population of the studied camels, which was available for the study, the lack of power analysis to determine sample size at the beginning of the study is a limitation of the present study.
Normal values for cortisol levels in serum of healthy camels ranged between 3.6 and 32.6 ng/ml with a mean ± SEM of 10.3 ± 0.6 ng/ml (Table 1). The comparison between samples collected from four camel breeds revealed no significant differences (p > 0.05) between cortisol levels in healthy Majaheem, Magateer, Sawahli, and Omani camels (Figure 1A). Female camels (10.8 ± 0.6 ng/ml) showed significantly (p = 0.01; CLES = 0.64) higher cortisol levels in their serum than males (7.9 ± 0.9 ng/ml) (Figure 1B). Within the female camels, the comparison between pregnant and non-pregnant she-camels (Figure 1C) revealed significantly higher cortisol levels in serum of non-pregnant (12.1 ± 1.0) than pregnant (10.2 ± 0.8) she-camels (p = 0.01; CLES = 0.58). In addition, lactating she-camels (12.5 ± 1.0 ng/ml) had significantly (p = 0.01; CLES = 0.65) higher cortisol levels than non-lactating (9.2 ± 0.7 ng/ml) she-camels (Figure 1D). Furthermore, correlation analysis revealed no significant (p > 0.05) association (r = 0.184) between animal age and cortisol level (data not shown).
Figure 1. Analysis of serum cortisol levels. Serum samples were collected from healthy camels and analyzed for cortisol using competitive ELISA. Cortisol concentration in camel serum was calculated based on the standard curve generated using standard cortisol concentrations. Cortisol levels were presented as scattered dot plot graph based on camel breed (A), camel gender (B), pregnancy (C), and lactation (D) status. The comparison between the four camel breeds was performed using the one-way analysis of variance (ANOVA). The comparison between values based on pregnancy and lactation was done using student’s t test. The impact of gender was statistically analyzed using Mann–Whitney U test.
To avoid interaction with a possible impact of camel gender on the results and as all camels in the slaughterhouse group were male camels, we compared this group with male camels from the healthy group. Serum samples collected from camels at slaughterhouse directly before slaughtering showed increased cortisol levels (116.6 ± 15.8 ng/ml) which were 10 times higher (p = 0.0001; CLES = 0.92) than cortisol levels in serum samples collected from male camels on their farms (6.8 ± 0.4 ng/ml) (Figure 2).
Figure 2. Impact of slaughterhouse stress on serum cortisol levels in camels. Serum samples were collected from camels at slaughterhouse (n = 20) and camels on their farms (n = 11) and analyzed for cortisol using competitive ELISA. Cortisol levels were calculated and presented as scattered dot plot graph. p values were calculated using student’s t test.
To evaluate the impact of infectious diseases on blood cortisol levels in camels, serum samples previously collected from camels affected by Surra and camels with bacterial metritis were retrospectively analyzed for cortisol levels. The results indicate significantly elevated cortisol levels in blood of diseased animals. Cortisol concentration in serum of Surra-affected camels (19.4 ± 3.1 ng/ml) was about two times higher than healthy (10.3 ± 0.6 ng/ml) camels (p = 0.02; CLES = 0.76) (Figure 3A). Similarly, she-camels with metritis (104.6 ± 17.7 ng/ml) had 10 times higher cortisol in their serum compared to healthy she-camels (10.8 ± 0.6 ng/ml) (p = 0.0001; CLES = 0.99) (Figure 3B).
Figure 3. Impact of disease on serum cortisol levels in camels. Serum samples were collected from (A) surra-affected camels (n = 27), (B) camels with metritis (n = 16), and healthy camels (n = 106) and analyzed for cortisol using competitive ELISA. Cortisol levels were calculated and presented as scattered dot plot graph. p values were calculated using student’s t test.
The correlation results between selected immune parameters and serum cortisol levels in camels are shown in Table 2. The total number of leukocytes (r = 0.56) in camel blood, the number of neutrophils (r = 0.52) and the neutrophil to lymphocyte ratio (r = 0.32) were positively correlated with serum cortisol levels (p < 0.05). Within blood lymphocytes, a negative correlation was found between the percentage of gd T cells (r = −0.36) and cortisol level, while the number of CD4+ T cells (0.39) was positively correlated with cortisol level. Phenotypic analysis of neutrophils revealed negative correlation (r = −0.51) between CD172a expression and cortisol level. For lymphocytes, the expression density of CD45 (r = −0.30) and MHC class I (r = −0.39) molecules was positively correlated with serum cortisol level. The abundance of the monocyte markers CD14 (r = −0.40), CD163 (r = −0.47), and CD172a (r = −0.51) was negatively correlated with serum cortisol level. On the other hand, a positive correlation was identified between reactive oxygen species level in neutrophils (r = 0.67) and monocytes (r = 0.69) and serum cortisol level (Table 2).
Table 2. The correlation results between selected immune parameters and serum cortisol levels in camels.
The employment of various indicators in the early detection of stress is one of the most effective strategies to counteract stress-induced disorders in humans and animals. Recent reports support the role of early stress detection in preventing systemic complications (26). The present study aimed at the evaluation of serum cortisol level as an indicator of stress in camels. For this, cortisol levels were retrospectively measured in serum collected from camels at slaughterhouse, from trypanosoma-affected camels, and from camels with bacterial metritis and compared it with normal cortisol levels in healthy camels. In addition, the impact of selected physiological factors on serum cortisol levels in healthy camels was investigated.
The comparison between blood cortisol levels in Majaheem, Magateer, Sawahli, and Omani camels indicates no impact of camel breed on blood cortisol level. As the animals involved in the present study were healthy non-stressed animals, the conclusion that breed does not influence cortisol levels in camels requires validation under stress-inducing circumstances, necessitating further research to evaluate breed-specific cortisol responses after exposing camels from different breeds to stressful stimuli (road transportation, heat stress, housing stress). Few studies investigated the impact of age, gender, and reproductive status of animal on serum cortisol levels in camel. Although the results of the present study are in agreement with the study of Sazmand et al., who reported no association between camel age and serum cortisol level, they, however, contrast the same study regarding the observed higher cortisol concentration in female than male camels (13). Similarly, the results of the present study are in contrast to a recent study by Mohamed et al., which reported the association between pregnancy and increased serum cortisol levels in camels (27). Pregnancy-associated change in serum cortisol levels was recently reported for the donkey species with increased cortisol levels during late pregnancy (28). Given that all the pregnant she-camels in the present study were in their early to mid-pregnancy, further studies should follow up the changes in serum cortisol levels during the whole pregnancy period in camels. In agreement with our study, Mohamed et al. reported no impact of camel lactation status on serum cortisol concentration. Further studies with higher numbers of camels are required to see whether the observed differences between the present study and previous studies are related to different analytic methodologies. Additionally, the impact of several factors including environmental conditions, animal nutrition, and management practices on cortisol levels in camels were investigated in several studies (29–31). In the present study, although the homogeneity in production systems and nutritional practices in the camel farms located in this geographical area (Eastern of Saudi Arabia) reduces the probability that these factors would affect our results, we cannot exclude a possible impact of these factors on the measured cortisol levels in camel serum. Furthermore, several studies reported seasonal variation in cortisol levels and the circadian regulation and diurnal secretion of cortisol leading to changes in serum cortisol levels (32–35). In the present study, although blood samples were collected during the morning time (between 7:00 and 9:00 am), we cannot exclude the possible impact of this minimal variation in sample collection time or a possible seasonal effect on the obtained cortisol levels, presenting an additional limitation of the study.
A wide range of values were reported in the scientific literature for cortisol concentration in serum of healthy camels. Although serum cortisol concentrations measured in healthy camels in the present study are in line with values reported for camels by several authors (15, 17, 36, 37), lower as well as higher levels were also reported in the literature (27, 31, 38). Radioimmunoassay and enzyme immunoassays are the main analytic methods used by most studies to measure cortisol levels in camel serum or plasma. Whether different analytic methods that were employed for cortisol measurement may have contributed to the variation in the reported ranges, is still to be investigated.
The response of camels to stressful situations has been investigated in some studies. In a recent study, radioimmunoassay was employed to measure cortisol levels in serum samples collected from 50 male dromedary camels at slaughterhouse. The study reported high (80.2–107.2 ng/ml) to very high (133.7–198.0 ng/ml) levels of serum cortisol in 26 camels (about 50% of the animals), while the other 24 camels showed low serum cortisol levels (13.0–67.9 ng/ml) (36). Similarly, Sayah et al. (39) measured high to very high serum cortisol levels in male Sahraoui camels at slaughterhouse. The study reported higher cortisol levels in animals held in lairage for short period (874 ± 631 ng/ml) after arrival and before slaughter (1 to 8 h) than in animals held for longer time (48–96 h) (127 ± 39 ng/ml). Although the present study confirms findings of previous studies on the impact of pre-slaughter handling on stress in camels (17, 36, 39), it could not specifically identify the exact factors responsible for the increased cortisol levels at slaughterhouse. Based on other studies in camels, common factors with major responsibility for elevated blood cortisol in pre-slaughtered camels mainly include the duration of pre-slaughter operations, the time until loading of the animals, unloading, water and food deprivation, and the transportation to the slaughterhouse (15, 17). The reliability of blood cortisol level as an effective indicator of stress in camels was proven in numerous studies (15, 36). In the present study, the elevated concentrations of serum cortisol in camels at slaughterhouse and in diseased camels (Surra and metritis) is in support of this. In addition, the observed differences in cortisol levels between males and females, pregnant and non-pregnant camels, and lactating and non-lactating camels confirm previous findings reporting that physiological parameters, such as gender and reproductive status of the animal, are within the factors that largely affect the animal response to stress (10–12).
The immune response to stress has been characterized in several species including cattle (40), sheep (19) rats (41), zebrafish (42), and human (43). Such studies are still lacking in camels. In the present study, higher cortisol levels were found associated with significant changes in some phenotypic and functional parameters of the camel immune system. Due to the lack of previous studies in camels, the changes observed in the present study were compared with findings in other species. The observed association between elevated cortisol levels in camels and increased numbers of total leukocytes and neutrophils as well as the higher neutrophil to lymphocyte ratio is in agreement with a typical stress leukogram pattern characterized by leukocytosis and neutrophilia (42, 43), while the positive correlation between cortisol level and the number of CD4+ T cells seems in contrast to the well-known stress-associated lymphopenia with reduced numbers of T helper cells. Studies in several species showed that glucocorticosteroid-induced leukocytosis is a result of demargination of neutrophils (shifting of marginal neutrophils to the circulation) due to modulation of cell adhesion molecules (44). Although the observed association between high cortisol levels and lower expression of CD172a on neutrophils, a signal-regulatory protein alpha (SIRPα) with essential role in adhesion and trans-endothelial migration of neutrophils (45), supports this finding, further studies are required to explore the exact mechanisms behind the changes in camel leukogram associated with elevated cortisol levels.
The impact of stress on blood monocytes was investigated in many species (46). The observed negative correlation between the abundance of the LPS receptor CD14, the scavenger receptor CD163, and the signal regulatory protein (SIRP-alpha) CD172a on monocytes and serum cortisol level supports a stress-induced alteration in monocyte phenotype. Together with toll-like receptor (TLR)-4, CD14 plays an essential role in the recognition of the cell wall of gram-negative bacteria by monocytes and macrophages. The best characterized function of CD163 is related to the binding of Hemoglobin:Haptoglobin complexes playing a role in tissue homeostasis (47). On the other hand, CD172a is known for its regulatory role in immune function by binding to its ligand CD47 (48). Given the different roles of these surface molecules during the immune response, the observed correlations indicate the complexity of the relationship between cortisol on monocyte biology. While reduced CD14 expression in animals with higher cortisol levels suggests reduced innate immune sensing of pathogens by monocytes, downregulation of CD163 and CD172a indicates negative impact of cortisol on regulatory mechanisms responsible for resolution of inflammation. Therefore, further studies are required to explore how those phenotypic alterations are reflected on monocyte function in stressed camels.
Innate immune cells such as neutrophils and monocytes produce reactive oxidative species and free radicals upon activation. Enhanced ROS production has been found associated with an inflammatory response with tissue injury and the release of danger signals (DAMPs) such as s100 proteins (49). In the present study, the association between higher reactive oxygen species levels in neutrophils and monocytes and serum cortisol levels reflects an oxidative stress response in immune cells from stressed camels.
As reported in the scientific literature, the stability of cortisol concentrations in saliva samples after 6 years of storage at −80°C and in serum samples after 3 to 40 years of storage at −20 (50, 51), it is unlikely that obtained serum cortisol results were affected by the long time storage (from 2020 for the metritis group and 2022 for the Surra group).
Increased cortisol levels in pre-slaughtering camels, Surra-diseased camels, and camels with bacterial metritis confirm the reliability of serum cortisol as stress indicator in this species. The variation in cortisol levels according to gender and reproductive status of the animal indicates the importance of considering these factors when evaluating the stress situation in camels. The observed fluctuations in the numbers of immune cell subsets and the changes in their phenotype may affect their functions, thus affecting the animal’s resistance to infectious diseases. Future research should, therefore, address the relationship between stress and the functional capacity of the immune system in camels.
The original contributions presented in the study are included in the article/Supplementary material, further inquiries can be directed to the corresponding author.
The animal studies were approved by Institutional Review Board. This study was performed in line with the principles of the Declaration of Helsinki. Approval was granted by the Ethics Committee of King Faisal University, Saudi Arabia (KFU-REC-2024-JUN-ETHICS1843). The studies were conducted in accordance with the local legislation and institutional requirements. Written informed consent was obtained from the owners for the participation of their animals in this study.
JH: Conceptualization, Data curation, Formal analysis, Funding acquisition, Investigation, Methodology, Project administration, Resources, Software, Supervision, Validation, Visualization, Writing – original draft, Writing – review & editing. HA: Conceptualization, Funding acquisition, Methodology, Project administration, Resources, Writing – review & editing.
The author(s) declare that financial support was received for the research and/or publication of this article. This research was funded by Princess Nourah bint Abdulrahman University Researchers Supporting Project number (PNURSP2025R460), Princess Nourah bint Abdulrahman University, Riyadh, Saudi Arabia, and the Deanship of Scientific Research, Vice Presidency for Graduate Studies and Scientific Research, King Faisal University, Saudi Arabia (KFU250126).
The authors declare that the research was conducted in the absence of any commercial or financial relationships that could be construed as a potential conflict of interest.
The author(s) declare that no Gen AI was used in the creation of this manuscript.
All claims expressed in this article are solely those of the authors and do not necessarily represent those of their affiliated organizations, or those of the publisher, the editors and the reviewers. Any product that may be evaluated in this article, or claim that may be made by its manufacturer, is not guaranteed or endorsed by the publisher.
The Supplementary material for this article can be found online at: https://www.frontiersin.org/articles/10.3389/fvets.2025.1570564/full#supplementary-material
ROS, Reactive oxygen species; CD, Cluster of differentiation; TMB, Tetramethylbenzidine; HPA, Hypothalamic-pituitary-adrenal; ACTH, Adrenocorticotropic hormone.
1. Garcia-Torres, S, Cabeza de Vaca, M, Tejerina, D, Romero-Fernandez, MP, Ortiz, A, Franco, D, et al. Assessment of stress by serum biomarkers in calves and their relationship to ultimate pH as an Indicator of meat quality. Animals (Basel). (2021) 11:2291. doi: 10.3390/ani11082291
2. Grelet, C, Vanden Dries, V, Leblois, J, Wavreille, J, Mirabito, L, Soyeurt, H, et al. Identification of chronic stress biomarkers in dairy cows. Animal. (2022) 16:100502. doi: 10.1016/j.animal.2022.100502
3. Ataallahi, M, Cheon, SN, Park, GW, Nugrahaeningtyas, E, Jeon, JH, and Park, KH. Assessment of stress levels in lactating cattle: analyzing cortisol residues in commercial Milk products in relation to the temperature-humidity index. Animals (Basel). (2023) 13:2407. doi: 10.3390/ani13152407
4. Earley, B, Buckham Sporer, K, and Gupta, S. Invited review: relationship between cattle transport, immunity and respiratory disease. Animal. (2017) 11:486–92. doi: 10.1017/S1751731116001622
5. Tufekci, H, and Sejian, V. Stress factors and their effects on productivity in sheep. Animals (Basel). (2023) 13:2769. doi: 10.3390/ani13172769
6. Proudfoot, K, and Habing, G. Social stress as a cause of diseases in farm animals: current knowledge and future directions. Vet J. (2015) 206:15–21. doi: 10.1016/j.tvjl.2015.05.024
7. Disanto, C, Celano, G, Varvara, M, Fusiello, N, Fransvea, A, Bozzo, G, et al. Stress factors during cattle slaughter. Ital J Food Saf. (2014) 3:1682. doi: 10.4081/ijfs.2014.1682
8. Bova, TL, Chiavaccini, L, Cline, GF, Hart, CG, Matheny, K, Muth, AM, et al. Environmental stressors influencing hormones and systems physiology in cattle. Reprod Biol Endocrinol. (2014) 12:58. doi: 10.1186/1477-7827-12-58
9. Kim, WS, Peng, DQ, Jo, YH, Nejad, JG, and Lee, HG. Responses of beef calves to long-term heat stress exposure by evaluating growth performance, physiological, blood and behavioral parameters. J Therm Biol. (2021) 100:103033. doi: 10.1016/j.jtherbio.2021.103033
10. Olivan, M, Gonzalez, J, Bassols, A, Diaz, F, Carreras, R, Mainau, E, et al. Effect of sex and RYR1 gene mutation on the muscle proteomic profile and main physiological biomarkers in pigs at slaughter. Meat Sci. (2018) 141:81–90. doi: 10.1016/j.meatsci.2018.03.018
11. Pena, F, Aviles, C, Domenech, V, Gonzalez, A, Martinez, A, and Molina, A. Effects of stress by unfamiliar sounds on carcass and meat traits in bulls from three continental beef cattle breeds at different ageing times. Meat Sci. (2014) 98:718–25. doi: 10.1016/j.meatsci.2014.07.021
12. Plusquellec, P, and Bouissou, M. Behavioural characteristics of two dairy breeds of cows selected (Herens) or not (Brune des Alpes) for fighting and dominance ability. Appl Anim Behav Sci. (2001) 72:1–21. doi: 10.1016/S0168-1591(00)00198-2
13. Sazmand, A, Tajik, J, Rasooli, A, and Moghaddam, SHH. Evaluation of serum concentration of cortisol, and the effects of age, sex and season in dromedary camels (Camelus dromedarius). Vet Res Biol Prod. (2017) 30:3213. doi: 10.22092/vj.2017.113213
14. Garcia-Belenguer, S, Palacio, J, Gascon, M, Acena, C, Revilla, R, and Mormede, P. Differences in the biological stress responses of two cattle breeds to walking up to mountain pastures in the Pyrenees. Vet Res. (1996) 27:515–26.
15. Saeb, M, Baghshani, H, Nazifi, S, and Saeb, S. Physiological response of dromedary camels to road transportation in relation to circulating levels of cortisol, thyroid hormones and some serum biochemical parameters. Trop Anim Health Prod. (2010) 42:55–63. doi: 10.1007/s11250-009-9385-9
16. Ali, MA, Abu Damir, H, Adem, MA, Ali, OM, Amir, N, Shah, AAM, et al. Effects of long-term dehydration on stress markers, blood parameters, and tissue morphology in the dromedary camel (Camelus dromedarius). Front Vet Sci. (2023) 10:1236425. doi: 10.3389/fvets.2023.1236425
17. Lemrhamed, A, Moussahil, A, Tabite, R, Farh, M, Iddar, A, and Khasmi, ME. Correlation between circulating cortisol and indicators of stress and oxidant stress during the preslaughter operations in camels. J Aridland Agric. (2023) 9:16–21. doi: 10.25081/jaa.2023.v9.8046
18. Knezevic, E, Nenic, K, Milanovic, V, and Knezevic, NN. The role of cortisol in chronic stress, neurodegenerative diseases, and psychological disorders. Cells. (2023) 12:2726. doi: 10.3390/cells12232726
19. Caroprese, M, Albenzio, M, Marzano, A, Schena, L, Annicchiarico, G, and Sevi, A. Relationship between cortisol response to stress and behavior, immune profile, and production performance of dairy ewes. J Dairy Sci. (2010) 93:2395–403. doi: 10.3168/jds.2009-2604
20. Blecha, F, Boyles, SL, and Riley, JG. Shipping suppresses lymphocyte blastogenic responses in Angus and Brahman X Angus feeder calves. J Anim Sci. (1984) 59:576–83. doi: 10.2527/jas1984.593576x. PubMed6490547
21. Murata, H, and Hirose, H. Suppression of bovine lymphocyte and macrophage functions by sera from road-transported calves. Br Vet J. (1991) 147:455–62. doi: 10.1016/0007-1935(91)90088-5
22. Hussen, J, Shawaf, T, Al-Mubarak, AIA, Humam, NAA, Almathen, F, and Schuberth, HJ. Leukocyte populations in peripheral blood of dromedary camels with clinical endometritis. Anim Reprod Sci. (2020) 222:106602. doi: 10.1016/j.anireprosci.2020.106602
23. Hussen, J, Althagafi, H, Alalai, MA, Alrabiah, NA, Al Abdulsalam, NK, Falemban, B, et al. Surra-affected dromedary camels show reduced numbers of blood B-cells and in vitro evidence of Trypanosoma-induced B cell death. Trop Anim Health Prod. (2024) 56:223. doi: 10.1007/s11250-024-04078-9
24. Hussen, J. Changes in cell vitality, phenotype, and function of dromedary camel leukocytes after whole blood exposure to heat stress in vitro. Front Vet Sci. (2021) 8:647609. doi: 10.3389/fvets.2021.647609
25. Hussen, J, Alkuwayti, MA, Falemban, B, Alhojaily, SM, Adwani, SA, Hassan, EAE, et al. Impact of selected bacterial and viral toll-like receptor agonists on the phenotype and function of camel blood neutrophils. Vet Sci. (2023) 10:154. doi: 10.3390/vetsci10020154
26. Spiljak, B, Vilibic, M, Glavina, A, Crnkovic, M, Seserko, A, and Lugovic-Mihic, L. A review of psychological stress among students and its assessment using salivary biomarkers. Behav Sci (Basel). (2022) 12:400. doi: 10.3390/bs12100400
27. Mohamed, RH, Khalphallah, A, Nakada, K, Elmeligy, E, Hassan, D, Ebissy, EA, et al. Clinical and correlated responses among steroid hormones and oxidant/antioxidant biomarkers in pregnant, non-pregnant and lactating CIDR-pre-synchronized dromedaries (Camelus dromedarius). Vet Sci. (2021) 8:247. doi: 10.3390/vetsci8110247
28. Alberghina, D, Statelli, A, Monteverde, V, Vazzana, I, Cascone, G, and Panzera, M. Serum cortisol and its correlation with leucocyte profile and circulating lipids in donkeys (Equus asinus). Animals (Basel). (2022) 12:841. doi: 10.3390/ani12070841
29. Fatnassi, M, Padalino, B, Monaco, D, Khorchani, T, Lacalandra, GM, and Hammadi, M. Effects of two different management systems on hormonal, behavioral, and semen quality in male dromedary camels. Trop Anim Health Prod. (2021) 53:275. doi: 10.1007/s11250-021-02702-6
30. Brahmi, M, Atigui, M, Hammadi, I, Portanguen, J, Hammadi, M, and Marnet, PG. Oxytocin and cortisol release during suckling, hand-milking and machine milking in camels. J Dairy Res. (2021) 88:278–85. doi: 10.1017/S0022029921000522
31. Nasreldin, N, El-Shoukary, RD, Abdel-Raheem, GSE, Gharib, HS, Zigo, F, Farkasova, Z, et al. Effect of mineral-vitamin premix supplementation on behavioral, performance, hormonal, oxidative stress, and serum biochemical profiles on rutting male Camelus dromedarius in Egypt. Front Vet Sci. (2023) 10:1221830. doi: 10.3389/fvets.2023.1221830
32. Huang, XX, Jiang, XM, Zheng, QX, and Chen, XQ. The association between circadian rhythm of cortisol and shift work regularity among midwives-a multicenter study in Southeast China. Front Public Health. (2022) 10:965872. doi: 10.3389/fpubh.2022.965872
33. O'Byrne, NA, Yuen, F, Butt, WZ, and Liu, PY. Sleep and circadian regulation of cortisol: a short review. Curr Opin Endocr Metab Res. (2021) 18:178–86. doi: 10.1016/j.coemr.2021.03.011
34. Mohd Azmi, NAS, Juliana, N, Azmani, S, Mohd Effendy, N, Abu, IF, Mohd Fahmi Teng, NI, et al. Cortisol on circadian rhythm and its effect on cardiovascular system. Int J Environ Res Public Health. (2021) 18:676. doi: 10.3390/ijerph18020676
35. Chergui, N, Mormede, P, Foury, A, Khammar, F, and Amirat, Z. Seasonal effects on plasma cortisol concentrations in the Bedouin buck: circadian studies and response to ACTH. Animal. (2017) 11:445–51. doi: 10.1017/S1751731116001671
36. Tabite, R, Lemrhamed, A, Abbadi, NE, Belhouari, A, Faye, B, and Khasmi, ME. Relationship between circulating levels of cortisol at slaughter and changes of some parameters of the camel meat during ageing. Emirates J Food Agric. (2019) 31:874–83. doi: 10.9755/ejfa.2019.v31.i11.2031
37. Agarwal, SP, Rai, AK, and Khanna, ND. Hormonal studies in postpartum female camels and their neonates. Theriogenology. (1992) 38:735–47. doi: 10.1016/0093-691X(92)90036-Q
38. Elias, E, and Weil, S. Serum cortisol levels in camels (Camelus dromedarius) during the reproductive cycle. Comp Biochem Physiol A Comp Physiol. (1989) 94:787–90. doi: 10.1016/0300-9629(89)90634-8
39. Sayah, TM, Smili, H, Hafid, K, Miloudi, A, Maamra, Y, Sentandreu, MA, et al. Effect of lairage time on stress in dromedary camels "Camelus dromedarius" from the El-Oued region (Algeria) and its impact on meat quality. Food Sci Technol Int. (2025). doi: 10.1177/10820132251315215
40. Gouvêa, VN, Cooke, RF, and Marques, RS. Impacts of stress-induced inflammation on feed intake of beef cattle. Front Anim Sci. (2022) 3:2748. doi: 10.3389/fanim.2022.962748
41. Stefanski, V, and Engler, H. Effects of acute and chronic social stress on blood cellular immunity in rats. Physiol Behav. (1998) 64:733–41. doi: 10.1016/S0031-9384(98)00127-9
42. Grzelak, AK, Davis, DJ, Caraker, SM, Crim, MJ, Spitsbergen, JM, and Wiedmeyer, CE. Stress leukogram induced by acute and chronic stress in zebrafish (Danio rerio). Comp Med. (2017) 67:263–9.
43. Maes, M, Van Bockstaele, DR, Gastel, A, Song, C, Schotte, C, Neels, H, et al. The effects of psychological stress on leukocyte subset distribution in humans: evidence of immune activation. Neuropsychobiology. (1999) 39:1–9. doi: 10.1159/000026552
44. Ince, LM, Weber, J, and Scheiermann, C. Control of leukocyte trafficking by stress-associated hormones. Front Immunol. (2018) 9:3143. doi: 10.3389/fimmu.2018.03143
45. Konrad, FM, Wohlert, J, Gamper-Tsigaras, J, Ngamsri, KC, and Reutershan, J. How adhesion molecule patterns change while neutrophils traffic through the lung during inflammation. Mediat Inflamm. (2019) 2019:1208086–16. doi: 10.1155/2019/1208086
46. van de Wouw, M, Sichetti, M, Long-Smith, CM, Ritz, NL, Moloney, GM, Cusack, AM, et al. Acute stress increases monocyte levels and modulates receptor expression in healthy females. Brain Behav Immun. (2021) 94:463–8. doi: 10.1016/j.bbi.2021.03.005
47. Fabriek, BO, Dijkstra, CD, and van den Berg, TK. The macrophage scavenger receptor CD163. Immunobiology. (2005) 210:153–60. doi: 10.1016/j.imbio.2005.05.010
48. Scott, CL, Tfp, ZM, Beckham, KS, Douce, G, and Mowat, AM. Signal regulatory protein alpha (SIRPalpha) regulates the homeostasis of CD103(+) CD11b(+) DCs in the intestinal lamina propria. Eur J Immunol. (2014) 44:3658–68. doi: 10.1002/eji.201444859
49. Johnson, J, Jaggers, RM, Gopalkrishna, S, Dahdah, A, Murphy, AJ, Hanssen, NMJ, et al. Oxidative stress in neutrophils: implications for diabetic cardiovascular complications. Antioxid Redox Signal. (2022) 36:652–66. doi: 10.1089/ars.2021.0116
50. Imamovic, M, Backlund, N, Lundstedt, S, Brattsand, G, Aardal, E, and Dahlqvist, P. Salivary cortisol and cortisone are stable after long-term storage. Scand J Clin Lab Invest. (2024) 84:405–9. doi: 10.1080/00365513.2024.2403005
51. Stroud, LR, Solomon, C, Shenassa, E, Papandonatos, G, Niaura, R, Lipsitt, LP, et al. Long-term stability of maternal prenatal steroid hormones from the National Collaborative Perinatal Project: still valid after all these years. Psychoneuroendocrinology. (2007) 32:140–50. doi: 10.1016/j.psyneuen.2006.11.008
Keywords: camel, stress, cortisol, leukocytes, immunophenotype
Citation: Hussen J and Althagafi H (2025) Serum cortisol level as marker of stress in camels: relationship with immunological profile. Front. Vet. Sci. 12:1570564. doi: 10.3389/fvets.2025.1570564
Received: 03 February 2025; Accepted: 13 March 2025;
Published: 25 March 2025.
Edited by:
Alberto Muñoz, University of Murcia, SpainReviewed by:
Daniela Alberghina, University of Messina, ItalyCopyright © 2025 Hussen and Althagafi. This is an open-access article distributed under the terms of the Creative Commons Attribution License (CC BY). The use, distribution or reproduction in other forums is permitted, provided the original author(s) and the copyright owner(s) are credited and that the original publication in this journal is cited, in accordance with accepted academic practice. No use, distribution or reproduction is permitted which does not comply with these terms.
*Correspondence: Hind Althagafi, aGphbC10aGFnYWZpQHBudS5lZHUuc2E=
Disclaimer: All claims expressed in this article are solely those of the authors and do not necessarily represent those of their affiliated organizations, or those of the publisher, the editors and the reviewers. Any product that may be evaluated in this article or claim that may be made by its manufacturer is not guaranteed or endorsed by the publisher.
Research integrity at Frontiers
Learn more about the work of our research integrity team to safeguard the quality of each article we publish.