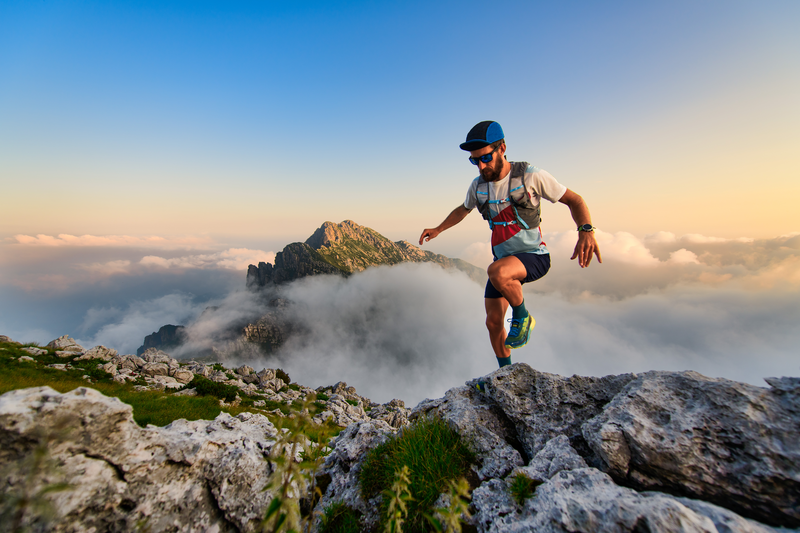
94% of researchers rate our articles as excellent or good
Learn more about the work of our research integrity team to safeguard the quality of each article we publish.
Find out more
ORIGINAL RESEARCH article
Front. Vet. Sci.
Sec. Parasitology
Volume 12 - 2025 | doi: 10.3389/fvets.2025.1568298
This article is part of the Research Topic Epidemiology, Diagnosis, and Treatment of Toxoplasma gondii Infection in a One Health Context View all articles
The final, formatted version of the article will be published soon.
You have multiple emails registered with Frontiers:
Please enter your email address:
If you already have an account, please login
You don't have a Frontiers account ? You can register here
Despite Pakistan's rich amphibian fauna, frog and toad species remain largely unexplored regarding blood-borne parasites. This study aims to investigate potential infections by Toxoplasma gondii and Hepatozoon spp. in four amphibian species (N n = 223) collected from various regions in Punjab and Khyber Pakhtunkhwa provinces. Molecular analyses revealed that 17 out of 223 amphibians (7.6%) were infected with Hepatozoon spp., with the highest infection rates found in Bufo olivaceous (20.0%), followed by Bufo stomaticus (9.3%) and Hoplobatrachus tigerinus (5.05%). DNA sequencing and BLAST analysis confirmed the presence of Hepatozoon ixoxo and Lankesterella sp. Phylogenetic analysis of both pathogens demonstrated genetic diversity among the Pakistani isolates, clustering with isolates from birds, amphibians, and reptiles worldwide. To the best of our knowledge, this is first ever report globally where we are documenting that Notably, 4.5% of the screened Pakistani anurans, including frogs (H. tigerinus, 8.1%) and toads (B. stomaticus, 1.9%), were found to be infected with Toxoplasma gondii-like strains for the first time. Parasite prevalence varied between sampling sites and amphibian species. This study represents the first report from Pakistan documenting the prevalence and genetic characterization of Hepatozoon sp., Lankesterella sp., and T. gondii-like strains among amphibians. We recommend conducting similar large-scale studies across various geo-climatic regions of Pakistan to further explore the epidemiology, genetic diversity, host-parasite interactions, and effective control of these pathogens among local frog and toad species. Identifying genetically related T. gondii strains in unexpected host animals, such as amphibians, has been crucial for contributing to the elucidation of the parasite's evolutionary history.
Keywords: Formatted: Left, Line spacing: single, Tab stops: Not at 0.5" + 1" + 2.54 Bufo olivaceous, Bufo stomaticus, Hoplobatrachus tigerinus, prevalence and phylogeny, Amphibians, Pakistan
Received: 29 Jan 2025; Accepted: 03 Mar 2025.
Copyright: © 2025 Iqbal, Salim, Khan, Niazi, Aqdas, ALDAWOUD, Usman, Muqaddas, Ullah, Belkahia, Khan and Ben Said. This is an open-access article distributed under the terms of the Creative Commons Attribution License (CC BY). The use, distribution or reproduction in other forums is permitted, provided the original author(s) or licensor are credited and that the original publication in this journal is cited, in accordance with accepted academic practice. No use, distribution or reproduction is permitted which does not comply with these terms.
* Correspondence:
Furhan Iqbal, Bahauddin Zakariya University, Multan, Pakistan
Disclaimer: All claims expressed in this article are solely those of the authors and do not necessarily represent those of their affiliated organizations, or those of the publisher, the editors and the reviewers. Any product that may be evaluated in this article or claim that may be made by its manufacturer is not guaranteed or endorsed by the publisher.
Research integrity at Frontiers
Learn more about the work of our research integrity team to safeguard the quality of each article we publish.