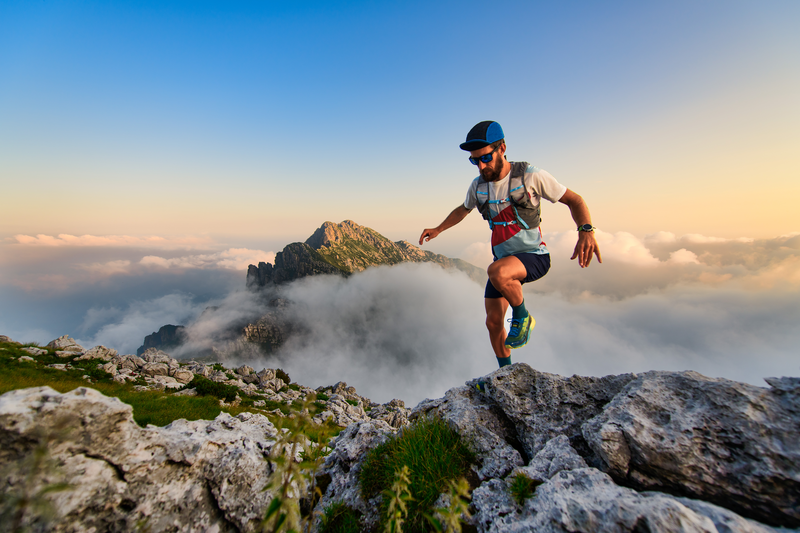
94% of researchers rate our articles as excellent or good
Learn more about the work of our research integrity team to safeguard the quality of each article we publish.
Find out more
ORIGINAL RESEARCH article
Front. Vet. Sci.
Sec. Parasitology
Volume 12 - 2025 | doi: 10.3389/fvets.2025.1566720
The final, formatted version of the article will be published soon.
You have multiple emails registered with Frontiers:
Please enter your email address:
If you already have an account, please login
You don't have a Frontiers account ? You can register here
Bioactive plants provide therapeutic and prophylactic effects to ruminants. We determined the effect of grazing on natural meadow grassland enriched with experimentally sown chicory (Cichorium intybus) on parasitological status, pasture larval infectivity, antioxidant parameters, and the histology of abomasal tissue in lambs experimentally infected with the parasitic gastrointestinal nematode (GIN) Haemonchus contortus. We also qualitatively identified the main polyphenols in the meadow grassland and phenolic metabolites in the faeces of the lambs. Sixteen lambs were orally infected with approximately 5000 infective larvae (L3) of H. contortus. The lambs were divided into two groups: lambs grazing on a plot consisting exclusively of meadow pasture which serves as control group and lambs grazing on a plot where approximately 25% of a meadow grassland was reclaimed with chicory. The experimental period was 144 days. The number of eggs per gramme (EPG) of faeces was quantified on D21, D34, D48, D62, D76, D89, D103, D118, D131, and D144 post-infection. Pasture contamination with H. contortus L3 was examined. EPG in both groups of lambs was highest at D34. Egg shedding was significantly lower in both groups from D48 onwards, with a reduction of >95% from D103 onwards. Pasture contamination with L3 was highest at D41 but was then significantly lower in both groups. The total antioxidant capacity, the activity of glutathione peroxidase and the concentration of malondialdehyde in the serum changed significantly during the experiment (P < 0.003, < 0.001, and < 0.016, respectively). At least 54 species of meadow plants were identified on both pasture plots; plant bioactive compounds identified were mainly phenolic acids, flavonoids, and glucosides. Phenolic metabolites (e.g. coumaric acid, chicory acid, salvigenin, and esters of gallic acid) were identified in the faeces of the lambs. In some lambs, the morphological observation identified small histopathological changes in the abomasal tissues typical of hemonchosis. Both the natural meadow pasture and the pasture enriched with experimentally sown chicory slowed the dynamics of GIN infection and pasture contamination with L3 by mobilising the antioxidant defensive system and gradually increasing the resistance of the infected lambs, probably due to the beneficial effects of plant bioactive substances.
Keywords: Antioxidant status, Chicory, Histology, Lambs, parasitological status, phytochemicals
Received: 25 Jan 2025; Accepted: 25 Feb 2025.
Copyright: © 2025 Komaromyova, Petric, Demcakova, Lesko, Čobanova, Babjak, Konigova, Kuzmina, Slusarczyk, Fortuna, Łukomska, Sidoruk, Cieslak, Varadyova and Varady. This is an open-access article distributed under the terms of the Creative Commons Attribution License (CC BY). The use, distribution or reproduction in other forums is permitted, provided the original author(s) or licensor are credited and that the original publication in this journal is cited, in accordance with accepted academic practice. No use, distribution or reproduction is permitted which does not comply with these terms.
* Correspondence:
Zora Varadyova, Institute of Animal Physiology, Centre of Biosciences, Slovak Academy of Sciences, Kosice, Slovakia
Marian Varady, Institute of Parasitology (SAS), Košice, Slovakia
Disclaimer: All claims expressed in this article are solely those of the authors and do not necessarily represent those of their affiliated organizations, or those of the publisher, the editors and the reviewers. Any product that may be evaluated in this article or claim that may be made by its manufacturer is not guaranteed or endorsed by the publisher.
Research integrity at Frontiers
Learn more about the work of our research integrity team to safeguard the quality of each article we publish.