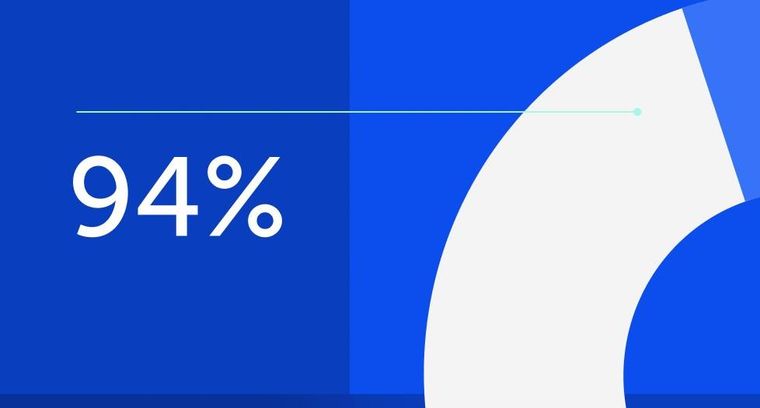
94% of researchers rate our articles as excellent or good
Learn more about the work of our research integrity team to safeguard the quality of each article we publish.
Find out more
ORIGINAL RESEARCH article
Front. Vet. Sci., 21 March 2025
Sec. Animal Nutrition and Metabolism
Volume 12 - 2025 | https://doi.org/10.3389/fvets.2025.1556330
This article is part of the Research TopicDietary Supplements for Optimizing Rumen Health and Nutrient Digestibility in LivestockView all 10 articles
Introduction: Mushrooms are rich in protein, polysaccharides, and other nutrients as a natural homology of medicine and food species, among which Agaricus bisporus is one of the most widely cultivated and consumed mushrooms worldwide. The mushroom stipe is a by-product of the mushroom industry that can be utilized as a feed resource based on its rich nutritional value. This study examined the effects of Agaricus bisporus stipe (ABS) as a feed additive on growth performance, blood indexes, rumen fermentation parameters, nutrient digestion and utilization, and rumen microbiota of heifers.
Methods: Twenty Holstein heifers (268 ± 9.43 d of age) were blocked in 10 groups of 2 heifers according to their live weights and ages, and within each group, the 2 heifers were randomly allocated to 1 of 2 treatments: control diet (CON), control diet plus ABS (ABS, 150 g·DM/animal/d).
Results: Heifers supplemented with ABS had higher average daily gain and a tendency to decrease feed conversion ratio, but rumen fermentation parameters were similar between the two treatments. Heifers in ABS had increased IgG, IgA, IgM, SOD, T-AOC, and GSH-Px concentrations while decreasing MDA concentration than heifers in CON. Although energy and nitrogen utilization were similar between treatments, the apparent total-tract digestibilities of NDF and ADF were higher for ABS than for CON heifers. Feeding ABS increased the relative abundance of one phylum (Verrucomicrobiota), two genera (Akkermansia and Ligilactobacillus), and two species (Akkermansia muciniphila and Ligilactobacillus murinus) in the rumen of heifers.
Discussion: In conclusion, heifers receiving ABS have greater growth performance, improved serum immune and antioxidant indexes, increased the relative abundance of some rumen bacteria, and higher nutrient digestion than CON heifers.
Mushrooms are an important source of nutrients worldwide, rich in protein and dietary fiber but low in fat (1). They are an excellent source of several micronutrients (including vitamin B3, vitamin B5, copper, selenium, and phosphorus), and it is important to note that mushrooms are the only vegetarian source of vitamin B12 and vitamin D (2). In addition, mushrooms are a potential source of bioactive molecules that promote health, including glycoproteins, triterpenes, chitin, α and β-glucan, mannan, xylans, and galactans (3). Therefore, mushrooms are natural homologies of medicine and food species, with anti-oxidative, immunity-boosting, anti-viral, anti-carcinogenic, and anti-tumor effects (4). According to the Food and Agriculture Organization (5) of the United Nations, global mushroom production increased fivefold from 2000 to 2023, reaching 44 million tons based on the nutritional value of mushrooms. The global market sales of mushrooms reached $399.67 billion in 2023, with China being the largest trading market, followed by Europe and North America, and it is predicted that the global mushroom market size will gradually expand.1
Agaricus bisporus is a member of the Agaricaceae family (6), also known as the white button mushroom or metacercaria (7), and is one of the most widely consumed and cultivated mushrooms worldwide (8). Agaricus bisporus is rich in nutrients, including essential amino acids, dietary fiber, B-vitamins, and vitamin D2 (9). Agaricus bisporus is also used as an effective alternative to animal protein due to their crude protein (CP) content of 19–38% on a dry matter (DM) basis (10). The main active components of the Agaricus bisporus are polysaccharides, which mainly include β-glucan polymers, xylans, galactans, mannans, and chitin (11, 12). Extensive studies have shown that the polysaccharide has antioxidant, antibacterial, immunomodulatory, treatment of diabetes and cardiovascular disease, prevention and treatment of various cancers, regulation of intestinal microbiota diversity and abundance, and other biological activities (11, 13). In addition, Agaricus bisporus is a good source of selenium (2.82 ± 1.48 mg/kg DM), which is widely recognized to reduce the risk of many health problems in humans and animals (14). In comparison to Pleurotus eryngii (DC.) Quel, Grifola frondosa (Dicks.) Gray, Pleurotus ostreatus, and Lentinus edodes, Agaricus bisporus exhibits greater antioxidant activity, attributed to its natural antioxidants, including phenols, serotonin, and tocopherols (6).
The Agaricus bisporus stipe (ABS), which measures about 3 cm at the root, is frequently removed during the Agaricus bisporus production process to ensure the cleanliness and quality of Agaricus bisporus products. Agaricus bisporus has the characteristics of a low planting environmental footprint, but by-products account for 20% of the total weight of Agaricus bisporus (15), which affects the ecological sustainable development of the mushroom industry. Notably, ABS has chitin comparable to mushroom caps and a high protein content, making it a valuable by-product. Therefore, ABS can be applied to animal feed, and some literature has reviewed the reuse of nutrients and bioactive ingredients in mushrooms (15–17). The replacement of 10 and 20% conventional feedstuffs with Agaricus bisporus did not adversely affect the growth performance of lambs (18), while the addition of 20 g/kg ABS in the basal diet improved the feed conversion ratio of broilers (19). Furthermore, adding Agaricus bisporus to the diet increased the meat color index of mutton (20), and increased the added value of quail eggs such as reducing yolk 1,1-diphenyl-2-picrylhydrazyl and malondialdehyde (MDA) content (21). Intestinal health is essential for both dietary nutrient absorption and animal immunity. In some previous studies, mushrooms have been demonstrated to improve intestinal morphology in broilers by increasing the ratio of villus height to crypt depth in the jejunal and villus height in the ileum (19), as well as by reducing the number of Escherichiacoli and Enterobacteriaceae in the rectum to reduce the average diarrhea severity in broilers (22). It is unclear whether ABS affects the rumen microbial community, in particular, the application effect of ABS in cattle diet. The objective of the study was to evaluate the effects of ABS supplementation on the growth performance and rumen bacterial populations of dairy heifers in a commercial herd.
The experiment was performed from June 8, 2024 to August 28, 2024 at the Yinan Dairy Farm in Shanghai, China. Experimental animal handling procedures were approved by the Animal Care and Use Committee of the Shanghai Academy of Agricultural Sciences.
A total of 20 Holstein heifers (mean ± SD; 268 ± 9.43 d of age, 335.8 ± 31.71 kg of BW) were used in a randomized complete block design and were blocked in 10 groups of 2 cows according to initial age and BW. Heifers from each block were randomly assigned into 1 of 2 treatments. The treatments were as follows: basal diet without ABS (control, CON) and diet with fresh ABS (ABS). Heifers were fed diets as total mixed ration (TMR) twice daily at 04:00 and 11:00 h, and the TMR offered was adjusted daily to allow a 5–10% refusal rate. The dosage of ABS was 150 g/animal per day based on DM basis (19), and the nutrient composition of ABS is shown in Table 1. The ABS was fed to each heifer before feeding TMR to ensure that each heifer received a set amount of ABS. The ingredient and nutrient composition of the basal diet are shown in Table 1. The TMR was formulated to contain 50.77% neutral detergent fiber (NDF), 33.55% acid detergent fiber (ADF), 17.26% CP, and minerals and vitamins to meet NRC (2001) (23) requirements to maintain a growth rate of ~0.9 kg/d for heifers. Experimental diets contained (on a DM basis) 25.8% corn silage, 21% soybean meal, 15% ground corn, 9.8% oatgrass hay, 8.6% soybean hulls, 6.1% alfalfa hay, 6.1% wheat straw, 6.1% dried distillers grains with solubles, and 1.5% mineral mix. Control and experimental heifers were fed individually in a tiestall barn, with an average feed bunk spacing of 0.80 m per heifer. During the experiment, each heifer was allowed to drink freely from an individual water bowl throughout the day. The experimental period lasted 50 d, with the first 10 d used for diet adaptation and the last 40 d for data and sample collection.
The amount of feed delivered and refused for each heifer was weighed and recorded daily. The TMR and ABS were sampled daily, mixed on a weekly basis, subsampled, and then dried at 55°C under forced air for 48 h to evaluate DM concentrations. The dietary samples were then ground through a mill fitted with a 1-mm screen and stored for subsequent nutritional analysis. Feed samples were tested for DM (method 930.15, AOAC International, 2000), CP (method 990.03; AOAC International, 2000), ash (method 942.05, AOAC International, 2000), ether extract (EE; method 920.29 AOAC International, 2000), and minerals (method 985.01, AOAC International, 2000). Moreover, according to the method of Van Soest et al. (24), samples were treated with heat-stable α-amylase and sodium sulfite, and NDF and ADF were analyzed sequentially, which was applied to the Ankom200 fiber analyzer (Ankom Technology, Macedon, NY, United States). The gross energy (GE) content in feed samples was also analyzed by a bomb calorimeter (Calorimeter Parr6400, Parr Instrument Company, Moline, IL, USA). Nutrient compositions of the TMR and ABS are shown in Table 1, respectively. Daily refusals were converted to a DM basis and then subtracted from the daily dietary DM provided to determine the daily DMI for each heifer.
From d 46 to 49, 4 heifers in each group were randomly selected for measurement of apparent total-tract nutrient digestibility, energy utilization, and nitrogen utilization. Feed consumption was obtained on a daily basis by weighing feed delivered to and refused by heifers. Diets and refusals were sampled daily, dried for 48 h at 55°C, ground through a mill fitted with a 1-mm screen, compounded over 4 days, and then sealed and stored for subsequent nutrient (DM, OM, CP, EE, NDF, ADF, and GE) analysis. Total fecal and urine output were collected and weighed from each heifer for 4 consecutive days. Representative (2%, on a fresh matter basis) samples of thoroughly mixed feces and urine were collected daily. Fecal samples were stored at −20°C until nutritional analysis was performed. The urine sample was diluted with 4 volumes of 0.072 mol/L sulfuric acid and stored at −20°C for further analyses. Fecal samples were dried for 72 h at 55°C and then ground using a mill to pass a 1-mm sieve. Fecal samples were composited in proportion to the DM for each heifer based on the daily fecal outputs. Ground fecal samples were analyzed for DM, OM, CP, EE, NDF, ADF, and GE using the same methods as diet samples. Measurement of urine CP and GE was performed as described in Jia et al. (25). Apparent total-tract nutrient digestibility was calculated according to the following equation: nutrient digestibility = 100% − [(fecal output, kg) × (fecal nutrient content, %)/(DMI, kg) × (feed nutrient content, %)]% (26).
At the initiation and termination of the experiment, each heifer was weighed before morning feeding on 2 consecutive days using an electronic scale (Shanghai Yaohua Weighing System Co., Ltd., Shanghai, China). On the last day of the experiment, blood samples were collected from the tail vein of each heifer into serum separator tubes (BD Vacutainer, Franklin Lakes, NJ, United States) before morning feeding. After collection, the samples were immediately stored in the shade and centrifuged at 3500 r/s for 20 min. The supernatant was pipetted into two 2-mL tubes and stored at −20°C for detection. Blood serums were evaluated for immunoglobulin A (IgA), immunoglobulin G (IgG), immunoglobulin M (IgM), superoxide dismutase (SOD), glutathione peroxidase (GSH-Px), MDA, total antioxidant capacity (TAC) by Beijing Jinhaikeyu Biotechnology Development Co., Ltd. (Beijing, China).
On the last day of the experiment, 6 heifers from each group were randomly selected to collect rumen fluid to obtain a representative sample. At 2 h after morning feeding, rumen fluid from heifers was collected using an oral stomach tube. The first 200 mL of fluid was discarded to avoid contamination of the sample by saliva, and 100 mL of rumen fluid was collected. Rumen fluid samples were measured for pH value (basic pH meter PB-20, Startorius AG, Germany) immediately after collection. Subsequently, 2 2-mL rumen fluid samples were collected and frozen in liquid nitrogen, and stored at −80°C until DNA extraction. The remaining rumen fluid was filtered through 4 layers of cheesecloth, transferred to 2 10-mL tubes with either 200 μL of 50% sulfuric acid or 2 mL of 25% meta-phosphoric acid, and stored at −20°C for subsequent analysis of ammonia nitrogen (NH3-N) and volatile fatty acids (VFA), respectively. The colorimetric assay described by Chaney and Marbach (27) was used to determine rumen NH3-N using a microplate spectrophotometer (Spectra max 190, Molecular Devices, United States). Sample preparation and measurement of VFA were performed according to the protocol described in Qumar et al. (28), using a gas chromatography apparatus (Agilent 6,890 N; Agilent Technologies Canada Inc., Mississauga, ON, Canada) equipped with a capillary column of 30-m length, 0.53-mm diameter, and 0.53-μm film thickness (Trace TR Wax, Thermo Fisher Scientific, Waltham, MA, United States).
Genomic DNA was extracted from rumen fluid samples using a commercial kit (TIANamp Stool DNA Kit DP328, Tiangen Biotech, Beijing, China) in accordance with the manufacturer’s protocols. The concentration and purity of the extracted DNA were determined by 1.0% agarose gel electrophoresis and a NanoDrop 2000 spectrophotometer (Thermo Fisher Scientific, Waltham, MA, United States). A pair of primers, 338F (5′-ACTCCTACGGGAGGCA GCAG-3′) and 806R (5′-GGACTACHVGGGTWTCTAAT-3′) was used to amplify 16S rRNA genes. PCR amplification was conducted under the following conditions: 95°C for 2 min, followed by 27 cycles at 95°C for 30 s, 55°C for 30 s, and 72°C for 60 s and a final extension at 72°C for 5 min. PCR reactions were carried out in triplicate within a 20 μL mixture comprising 10 μL of 2X Pro Taq, 0.8 μL of each primer (5 μM), 10 ng of template DNA, and double-distilled H2O. The products OF the PCR amplification were extracted from 2% agarose gels and purified using a commercial kit (AxyPrep DNA Gel Extraction Kit, Axygen Biosciences, Union City, CA, United States) according to the manufacturer’s instructions. Purified amplicons were pooled in equimolar amounts, and sequencing of the amplicons was performed by Shanghai Biozeron Biotechnology Co., Ltd. (Shanghai, China).
The amplicon sequences were filtered using FASTP (v0.19.6) (41) and subsequently merged with FLASH (v1.2.11) (29). The reads were processed on the QIIME2 platform (v2022.2) (30), where high-quality sequences were denoised with the DADA2 plugin. This process completed sequence quality control and construction of amplicon sequence variants (ASV) (31). Using a pretrained QIIME2-compatible SILVA 138 database (32) for bacteria, ASV was classified by a pre-fitted classifier based on scikit-learn (33) with a similarity threshold of 99%. Rarefaction analysis, utilizing ASV information based on Mothur v.1.30.1 (34), further revealed the α-diversity indexs, including Chao richness, abundance-based coverage estimators (ACE) index, Shannon index, and Simpson index. Beta diversity analysis was conducted using the Vegan v2.5–3 package in R studio (ver. 2023.06.2 + 561, PBC, Boston, MA, United States), where the similarity between microbial communities in different samples was assessed through principal coordinate analysis (PCoA) based on Bray-Curtis distance matrices. Spearman correlation was used to evaluate the correlations between the top 20 bacterial genera and physiological parameters in dairy heifers.
Data were analyzed using the SAS 9.4 (SAS Institute Inc., Cary, NC). Before analysis, data were tested for normality using the UNIVARIATE procedure, and the PROC Univariate was used to remove outliers from the statistical analysis when the absolute studentized residual values were > 3.0. The DMI, BW, apparent total-tract nutrient digestibility, energy utilization, nitrogen utilization, blood indexes, and rumen fermentation parameters were analyzed using the one-way ANOVA procedure according to the following model:
where Yi is the dependent variable, μ is the overall mean, Ti is the effect of treatment (i = 1, 2), and ei is the residual error. Compared diversity and relative abundance of bacteria between the CON and ABS using the Wilcoxon rank-sum test in SPSS 19.0. Statistical significance was declared at p ≤ 0.05, and tendencies at 0.05 < p ≤ 0.10.
Growth performance and ruminal fermentation parameters of heifers are presented in Table 2. Initial BW was similar between treatments (p > 0.05). Addition of ABS did not affect final weight but increased average daily gain (ADG) by 0.16 kg/d (p ≤ 0.05). The DMI did not differ (p > 0.05) between heifers fed control and ABS diets. However, heifers on ABS treatment had a tendency to decrease the feed conversion ratio by 9.7% (0.05 < p ≤ 0.10). Additionally, ABS had no effect on pH value, as well as concentrations of total VFA, and molar proportions of acetate, propionate, butyrate, isobutyrate, valerate, and isovalerate (p > 0.05).
Table 2. Effects of feeding ABS for dairy heifers on the growth performance and ruminal fermentation parameters.
The influences of ABS supplementation on serum immunity and antioxidant status of heifers are shown in Table 3. Supplementation with ABS increased (p ≤ 0.05) the concentrations of IgG, IgA, and IgM compared to CON (11.12 vs. 10.07 mg/mL, 0.833 vs. 0.683 mg/mL, 2.921 vs. 2.480 mg/mL). Similarly, SOD, TAC, and GSH-Px increased (p ≤ 0.05) by 8.8, 1.55, and 80.6 U/mL for the ABS when compared with CON, respectively (111.8 vs. 103.0 U/mL, 11.21 vs. 9.66 U/mL, 1,015 vs. 934.4 U/mL). However, ABS supplementation decreased (p ≤ 0.05; 3.888 vs. 4.672 nmol/mL) MDA concentration compared with CON.
The effects of ABS on the nutrient apparent digestibility of dairy heifers are presented in Table 4. Intakes of DM, OM, NDF, ADF, and EE were not different between the CON and ABS groups (p > 0.05). Supplementation of ABS significantly increased the apparent total-tract digestibilities of NDF (64.81% vs. 69.46%) and ADF (64.11% vs. 68.67%) compared with the control group (p ≤ 0.05), but did not affect the apparent total-tract digestibilities of DM, OM, and EE (p > 0.05). Energy intake, output, and utilization did not differ between the two groups (p > 0.05), nor did nitrogen intake, output, and utilization (p > 0.05) (Table 5).
Table 4. Effects of feeding ABS for dairy heifers on the apparent total-tract digestibility of nutrients.
The effects of ABS on the α and β diversity of the rumen bacterial community are presented in Figure 1 and Supplementary Table S1. Supplementation of ABS significantly increased the community richness including Sobs and ACE indices of the ASV level compared with the control group (p ≤ 0.05), but did not affect the Chao, Shannon, and Simpson indices (p > 0.05) (Figure 1A and Supplementary Table S1). The Venn diagram revealed that two groups shared 792 common ASVs, while 12 and 23 unique ASVs were illustrated in CON and ABS groups, respectively (Figure 1B). PCoA based on the Bray-Curtis distance showed clear separation in rumen microbial structure between the CON and ABS groups (Figure 1C). The effects of ABS on the relative abundances of ruminal bacteria phyla and genera are presented in Figure 2 and Supplementary Table S2. In the rumen, the dominant phyla for both groups were Bacillota (Firmicutes, 49.08%) and Bacteroidota (Bacteroidetes, 44.98%), with the ratio of Bacillota/Bacteroidota were 1.03 and 1.25 in CON and ABS groups, respectively. At the phylum level, heifers fed ABS had a higher relative abundance of Verrucomicrobiota (p ≤ 0.05). At the genus level, supplementation of ABS increased (p ≤ 0.05) the relative abundance of the ruminal bacteria genera Akkermansia (member of the Bacillota phylum) and Ligilactobacillus (member of the Bacillota phylum) in the ABS group compared with the CON group.
Figure 1. Effects of ABS on the rumen bacterial community richness and diversity in dairy heifers. (A) Alpha-diversity indexes of the rumen bacteria. (B) Venn diagram of the rumen bacterial community. (C) Principal coordinate analysis (PCoA) plot of the rumen bacterial community based on Bray–Curtis dissimilarity. *p < 0.05. CON, basal diet; ABS, basal diet including 150 g·DM/heifer daily of fresh ABS.
Figure 2. The differential bacteria at the phylum and genus levels in the rumen digesta between the CON and ABS groups. CON, basal diet; ABS, basal diet including 150 g·DM/heifer daily of fresh ABS.
The correlations between the top 20 bacterial genera and physiological parameters were analyzed using Spearman correlation analysis (Figure 3). Ligilactobacillus was significantly positively correlated with IgA, IgG, IgM, TAC, GSH-PX, and SOD, but significantly negatively correlated with MDA (p ≤ 0.05). A highly significant positive correlation existed between Akkermansia and GSH-PX, and a highly significant negative correlation existed between Butyrivibrio and DMI (p ≤ 0.05). The molar proportion of butyrate was positively correlated with unclassified_c__Bacteroidia and unclassified_f__Lachnospiraceae, while negatively correlated with Prevotella and unclassified_o__Bacteroidales (p ≤ 0.05). Norank_f__Oscillospiraceae exhibited positive correlations with the molar proportion of isobutyrate and isovalerate whereas it was negatively correlated with DMI (p ≤ 0.05).
Figure 3. Correlation analysis of rumen microbiota and physiological parameters in dairy heifers. Red indicates a positive correlation, blue indicates a negative correlation. *p < 0.05, **p < 0.01, ***p < 0.001. IgA, immunoglobulin A; IgG, immunoglobulin G; IgM, immunoglobulin M; TAC, total antioxidant capacity; GSH-Px, glutathione peroxidase; SOD, superoxide dismutase; MDA, malondialdehyde; TVFA, total volatile fatty acids; DMI, dry matter intake; ADG, average daily gain; FCR, feed conversion ratio.
In the present study, we compared the ADG, feed conversion ratio, as well as feed digestibility and utilization, blood immunity and antioxidant capacity, rumen fermentation patterns and microbial abundance. The main results of this study suggest that there is a potential to improve the growth performance of heifers by the addition of ABS to the diet.
Our results show that the heifers in the two groups had similar DMI despite the supplementation of ABS. However, the heifers fed the control diet faced challenges, as evidenced by a significantly lower ADG compared with heifers fed the diet supplementation with ABS. This variation may be attributable to the polysaccharides in the ABS cell wall, which have a growth-promoting effect on animals (35). Not surprisingly, ABS has a tendency to improve the feed conversion ratio of heifers. Similar to our results, Dong et al. (11) indicated that whereas the body weight of broilers did not respond to the addition of ABS (20 g/kg·DM) in diet, dietary ABS reduced feed intake and thus increased the feed conversion ratio of broilers. Numerous studies have shown that ABS contains rich nutrients such as protein, healthy substances such as antioxidants, and important bioactive substances such as phenols, which comprehensively support the beneficial effects on the growth performance of heifers [see, e.g., review by Yang et al. (9)]. In the previous study, however, there was no effect on the growth performance of the lambs and only a reduction in the feed cost when ABS was added to the diet (18). The inconsistency with the results of this study may be attributed to the proportion of ABS added to the lamb diet reached 10 and 20% respectively, and the ME of ABS was 20.6% lower than that of dietary alfalfa, which may affect the initial energy and nitrogen balance of the diet.
Research in human nutrition and medicine has shown that Agaricus bisporus has antioxidant and immune-boosting properties (8), and is considered a natural prebiotic (36). In the current study, heifers fed ABS had on average 10.4, 22.01, and 17.7% greater IgG, IgA, and IgM than that offered the control diet, respectively. Dietary Agaricus bisporus stimulates salivary secretory immunoglobulin A secretion in humans, which is known to be the first line of adaptive humoral immune defense of mucosal surfaces. A large number of studies have shown that Agaricus bisporus polysaccharides have the biological activity of immunomodulatory (37–39), which contributed to an increase in the level of immunity in heifers. In the present study, the addition of ABS resulted in lower blood serum levels of MDA, indicating weakened peroxidation. A decrease in MDA levels was accompanied by an increase in SOD, GSH-Px, and TAC, which was important for the activation of oxygen free radicals. On the other hand, several studies have shown that Agaricus bisporus is a food source of antioxidants (6), such as Agaricus bisporus polysaccharides, which have biological activities in antioxidant (40). In agreement with our findings, Yang et al. (9) demonstrated that ABS as a dietary additive increased SOD activity and GSH-Px activity in serum, and also increased SOD activity but decreased MDA content in the yolk of laying hens. These help to explain the results observed in this trial, where ABS improved the serum immunity and antioxidant indicators in heifers.
In the nylon bag and gas production techniques test, Agaricus bisporus had slow rumen degradability and fermentability and did not affect total VFA concentration (10). In another previous study, Agaricus bisporus had no effect on rumen microbial protein concentrations in sheep (42). Consistent with these findings, there was no change in rumen fermentation parameters of heifers fed the ABS diets in this study. The promotion of feed digestion by adding mushrooms has previously been reviewed (8, 9). In the review by Mohan et al. (17), mushroom products such as mycelia, stems, dried powder, and extracts have been widely used in aquaculture, which can improve the digestive enzyme activity of the gastrointestinal tract of cultivable aquatic animals. More recently, zebrafish fed a synbiotic combination (Agaricus bisporus and probiotics) showed higher digestive enzyme activity (43). Moradzadeh-Somarin et al. (42) reported that replacing alfalfa with Agaricus bisporus to 210 g/kg·DM in sheep diets increased OM digestibility and metabolizable energy, but decreased NDF and CP digestibility. Nevertheless, we observed that adding ABS to the diet increased NDF digestibility by 4.65% and ADF digestibility by 4.56% in heifers. This variation may be attributed to differences in the inherent physicochemical composition of Agaricus bisporus and alfalfa, as well as the different species of experimental animals (44).
Regarding rumen microbiota, although ABS has been recognized as an additive or a component of animal feed (10, 41), its effect on rumen microbiota community structure is still unclear. Bacillota and Bacteroidota are two key bacteria in the rumen, and the Bacillota/Bacteroidota ratio is usually positively correlated with feed efficiency and milk yield of dairy cows (45). The Bacillota/Bacteroidota ratio of the ABS group was higher than that of the CON group (1.25 vs. 1.03), which supported the higher growth performance of the ABS group in this study. At the phylum level, ABS only increased the relative abundance of Verrucomicrobiota in heifers in this experiment. Verrucomicrobota is considered a major degrader of glycosaminoglycans due to its presence of glycoside hydrolases and sulfatases (46). Therefore, Verrucomicrobota contains species that are highly specialized in degrading complex polysaccharides (47). A disproportionately high number of carbohydrate-active enzymes per Mb of the genome suggests that Verrucomicrobiota has a significant metabolic capacity to support rumen function, even though it is not very abundant in the rumen, according to research by Gharechahi et al. (48). A recent study reported that the Verrucomicrobiota genome has the second-highest metabolic capacity for carbohydrate polysaccharides, trailing only the Bacteroidota genome among rumen bacteria (48). Further analysis of the taxonomic origin of enzymes using secretion signals revealed that 36% of the enzymes secreted by Verrucomicrobiota act on the degradation of rumen lignocellulose (48). Liu et al. (49) demonstrated that the abundance of Verrucomicrobiota in the rumen of cattle in the high-cellulose forage group was higher than that in the low-cellulose forage group. The majority of the Verrucomicrobiota in the rumen of Hu sheep belong to the genus Akkermansia (50), which is enriched in the gastrointestinal tract of animals fed crude fiber diet. In the present study, Akkermansia was enriched in the rumen of heifers fed the ABS diet. Similarly, Agaricus bisporus polysaccharides increased the relative abundance of Akkermansia in the gastrointestinal tract of mice (49). These findings support the results that ABS improved the digestibility of NDF and ADF in heifers in this trial. In addition, Fujio-Vejar et al. (51) found that Verrucomicrobia has anti-inflammatory properties. Remarkably, Akkermansia muciniphila was the first cultured representative of Verrucomicrobia at the species level, and there was a positive correlation between host immunity and Akkermansia muciniphila abundance (52). The rumen of heifers supplemented with ABS had a higher abundance of Akkermansia muciniphila in this experiment (Supplementary Table S2). These may explain why dietary ABS improved the serum immune and antioxidant indexes of heifers. Ligilactobacillus is made up of former members of Lactobacillus and is a type of lactic acid bacterium that benefits gastrointestinal health (53). Moreover, Li et al. (54) found that Ligilactobacillus was positively correlated with intestinal anti-inflammatory. Our results also showed that Ligilactobacillus was positively correlated with serum immune and antioxidant parameters. The genus Ligilactobacillus and species Ligilactobacillus murinus were abundant in the rumen of heifers in the ABS group (Supplementary Table S2), suggesting again that ABS was beneficial to the growth and immunity of heifers.
The findings observed in this study suggested that ABS supplementation improves growth performance by increasing ADG and promoting the feed conversion ratio of dairy heifers. Additionally, our results suggest ABS has the ability to both enhance the immunity and antioxidant capacity of heifers. The ABS was effective in nutrient digestion because NDF and ADF digestibilities were greater in ABS heifers. Feeding ABS enhanced some ruminal bacteria populations, including Verrucomicrobia, Akkermansia, Akkermansia muciniphila, Ligilactobacillus, and Ligilactobacillus murinus, which may partially explain the higher nutrient digestibility, blood indicators, and growth performance in ABS heifers.
The raw data reads of 16S rRNA gene sequencing of microbiota are deposited in the National Center for Biotechnology Information (NCBI) repository, accession number PRJNA1224486.
The animal study was approved by the Shanghai Academy of Agricultural Sciences. The study was conducted in accordance with the local legislation and institutional requirements.
PJ: Data curation, Formal analysis, Writing – original draft, Writing – review & editing. CL: Funding acquisition, Writing – original draft. XY: Investigation, Writing – original draft. XJ: Methodology, Writing – original draft. HL: Methodology, Writing – original draft. NL: Funding acquisition, Writing – original draft. DX: Resources, Writing – review & editing.
The author(s) declare that financial support was received for the research and/or publication of this article. This work was funded by the Shanghai Agricultural Science and Technology Innovation Program (Grant No. K2024-02-08-00-12-F00017).
The authors thank colleagues at the Shanghai Academy of Agricultural Sciences. The authors are also grateful to the team of Jinshan Distict Animal Disease Control Center, Shanghai.
The authors declare that the research was conducted in the absence of any commercial or financial relationships that could be construed as a potential conflict of interest.
The authors declare that no Gen AI was used in the creation of this manuscript.
All claims expressed in this article are solely those of the authors and do not necessarily represent those of their affiliated organizations, or those of the publisher, the editors and the reviewers. Any product that may be evaluated in this article, or claim that may be made by its manufacturer, is not guaranteed or endorsed by the publisher.
The Supplementary material for this article can be found online at: https://www.frontiersin.org/articles/10.3389/fvets.2025.1556330/full#supplementary-material
1. Heleno, SA, Ferreira, RC, Antonio, AL, Queiroz, M-JRP, Barros, L, and Ferreira, ICFR. Nutritional value, bioactive compounds and antioxidant properties of three edible mushrooms from Poland. Food Biosci. (2015) 11:48–55. doi: 10.1016/j.fbio.2015.04.006
2. Blumfield, M, Abbott, K, Duve, E, Cassettari, T, Marshall, S, and Fayet-Moore, F. Examining the health effects and bioactive components in Agaricus bisporus mushrooms: a scoping review. J Nutr Biochem. (2020) 84:108453. doi: 10.1016/j.jnutbio.2020.108453
3. Zou, HK, Hoseinifar, SH, Miandare, HK, and Hajimoradloo, A. Agaricus bisporus powder improved cutaneous mucosal and serum immune parameters and up-regulated intestinal cytokines gene expression in common carp (Cyprinus carpio) fingerlings. Fish Shellfish Immunol. (2016) 58:380–6. doi: 10.1016/j.fsi.2016.09.050
4. Tian, J, Liu, H, Li, J, and Wang, Y. Recent trends in non-destructive techniques for quality assessment of edible mushrooms. J Food Compos Anal. (2024) 136:106805. doi: 10.1016/j.jfca.2024.106805
5. Food and Agriculture Organization. FAOSTAT statistics database 2023. Rome, Italy: Food and Agriculture Organization of the United Nations (2023).
6. Usman, M, Murtaza, G, and Ditta, A. Nutritional, medicinal, and cosmetic value of bioactive compounds in button mushroom (Agaricus bisporus): a review. Appl Sci. (2021) 11:5943. doi: 10.3390/app11135943
7. Lin, X, and Sun, DW. Research advances in browning of button mushroom (Agaricus bisporus): affecting factors and controlling methods. Trends Food Sci Technol. (2019) 90:63–75. doi: 10.1016/j.tifs.2019.05.007
8. Jiang, H, Wang, X, Ma, M, Yuan, S, Zhang, L, Jiao, L, et al. Epsilon-poly-l-lysine and methyl jasmonate synergistically inhibit Pseudomonas tolaasii-caused brown spot disease in postharvest button mushroom (Agaricus bisporus). Sci Hortic. (2024) 338:113710. doi: 10.1016/j.scienta.2024.113710
9. Yang, K, Zhao, M, and Argyropoulos, D. Machine learning based framework for the detection of mushroom browning using a portable hyperspectral imaging system. Postharvest Biol Technol. (2025) 219:113247. doi: 10.1016/j.postharvbio.2024.113247
10. Braaksma, A, and Schaap, DJ. Protein analysis of the common mushroom Agaricus bisporus. Postharvest Biol Technol. (1996) 7:119–27. doi: 10.1016/0925-5214(95)00034-8
11. Dong, K, Wang, J, Tang, F, Liu, Y, and Gao, L. A polysaccharide with a triple helix structure from Agaricus bisporus: characterization and anti-colon cancer activity. Int J Biol Macromol. (2024) 281:136521. doi: 10.1016/j.ijbiomac.2024.136521
12. Friedman, M. Mushroom polysaccharides: chemistry and antiobesity, antidiabetes, anticancer, and antibiotic properties in cells, rodents, and humans. Food Secur. (2016) 5:80. doi: 10.3390/foods5040080
13. Sima, P, Vannucci, L, and Vetvicka, V. Glucans and cancer: historical perspective. Cancer Transl Med. (2015) 1:209–14. doi: 10.4103/2395-3977.172860
14. Vetter, J, and Lelley, J. Selenium level of the cultivated mushroom Agaricus bisporus. Acta Aliment. (2004) 33:297–301. doi: 10.1556/aalim.33.2004.3.10
15. Wang, CY. A review on the potential reuse of functional polysaccharides extracted from the by-products of mushroom processing. Food Bioprocess Technol. (2020) 13:217–28. doi: 10.1007/s11947-020-02403-2
16. Ayimbila, F, and Keawsompong, S. Nutritional quality and biological application of mushroom protein as a novel protein alternative. Curr Nutr Rep. (2023) 12:290–307. doi: 10.1007/s13668-023-00468-x
17. Mohan, K, Karthick Rajan, D, Muralisankar, T, Ganesan, AR, Marimuthu, K, and Sathishkumar, P. The potential role of medicinal mushrooms as prebiotics in aquaculture: a review. Rev Fish Sci. (2022) 14:1300–32. doi: 10.1111/raq.12651
18. Yousefi, M, Malecky, M, Zaboli, K, and Najafabadi, HJ. In vitro and in sacco determining the nutritive value of button mushroom stipe and its application in growing lambs diet. Ital J Anim Sci. (2022) 21:279–90. doi: 10.1080/1828051X.2021.1987847
19. Altop, A, Coskun, I, Filik, AG, Cayan, H, Sahin, A, Gungor, E, et al. Dietary supplementation of Agaricus bisporus stalk meal on growth performance, carcass and organ traits, meat quality, cecum mesophilic aerobic bacteria counts and intestinal histology in broiler chickens. Cienc Rural. (2021) 52:e20201051. doi: 10.1590/0103-8478cr20201051
20. Cvrtila Fleck, Z, Kozacinski, L, Njari, B, Marencic, D, Mrsic, G, Spiranec, K, et al. Technological properties and chemical composition of the meat of sheep fed with Agaricus bisporus supplement. Vet Arh. (2015) 85:591–600. https://hrcak.srce.hr/149501
21. Sarmiento-Garcia, A, Olgun, O, Kılınç, G, and Sevim, B. Dietary supplementation of by-products on development, egg production, egg quality, and antioxidant capacity of yolk in laying quails. Anim Sci Paper Rep. (2024) 42:51–64. doi: 10.2478/aspr-2023-0022
22. Spoljaric, D, Srecec, S, Paro, MMK, Cop, MJ, Mrsic, G, Simpraga, B, et al. The effects of feed supplemented with Agaricus bisporus on health and performance of fattening broilers. Vet Arhiv. (2015) 85:309–22.
23. National Research Council (NRC). Nutrient requirements of dairy cattle. 7th rev. ed. Washington, DC: National Academies Press (2001).
24. Van Soest, PJ, Robertson, JB, and Lewis, BA. Methods for dietary fiber, neutral detergent fiber, and nonstarch polysaccharides in relation to animal nutrition. J Dairy Sci. (1991) 74:3583–97. doi: 10.3168/jds.S0022-0302(91)78551-2
25. Jia, P, Tu, Y, Liu, ZH, Li, FD, Yan, TH, Ma, SL, et al. Diets supplementation with Bacillus subtilis and Macleaya cordata extract improve production performance and the metabolism of energy and nitrogen, while reduce enteric methane emissions in dairy cows. Anim Feed Sci Technol. (2022) 294:115481. doi: 10.1016/j.anifeedsci.2022.115481
26. Beckman, JL, and Weiss, WP. Nutrient digestibility of diets with different fiber to starch ratios when fed to lactating dairy cows. J Dairy Sci. (2005) 88:1015–23. doi: 10.3168/jds.S0022-0302(05)72769-7
27. Chaney, AL, and Marbach, EP. Modified reagents for determination of urea and ammonia. Clin Chem. (1962) 8:130–2. doi: 10.1093/clinchem/8.2.130
28. Qumar, MR, Khiaosa-ard, P, Pourazad, SU, Wetzels, F, Klevenhusen, W, Kandler, JR, et al. Evidence of in vivo absorption of lactate and modulation of short chain fatty acid absorption from the reticulo rumen of non-lactating cattle fed high-concentrate diets. PLoS One. (2016) 11:e0164192. doi: 10.1371/journal.pone.0164192
29. Magoc, T, and Salzberg, SL. FLASH: fast length adjustment of short reads to improve genome assemblies. Bioinformatics. (2011) 27:2957–63. doi: 10.1093/bioinformatics/btr507
30. Bolyen, E, Rideout, JR, Dillon, MR, Bokulich, NA, Abnet, CC, Al-Ghalith, GA, et al. Reproducible, interactive, scalable and extensible microbiome data science using QIIME 2. Nat Biotechnol. (2019) 37:852–7. doi: 10.1038/s41587-019-0209-9
31. Callahan, BJ, McMurdie, PJ, Rosen, MJ, Han, AW, Johnson, AJ, and Holmes, SP. DADA2: high resolution sample inference from Illumina amplicon data. Nat Methods. (2016) 13:581–3. doi: 10.1038/nmeth.3869
32. Quast, C, Pruesse, E, Yilmaz, P, Gerken, J, Schweer, T, Yarza, P, et al. The SILVA ribosomal RNA gene database project: improved data processing and web-based tools. Nucleic Acids Res. (2013) 41:D590–6. doi: 10.1093/nar/gks1219
33. Pedregosa, F, Varoquaux, G, Gramfort, A, Michel, V, Thirion, B, Grisel, O, et al. Scikit-learn: machine learning in python. J Mach Learn Res. (2011) 12:2825–30. doi: 10.48550/arXiv.1201.0490
34. Schloss, PD, Westcott, SL, Ryabin, T, Hall, JR, Hartmann, M, Hollister, EB, et al. Introducing mothur: open-source, platform-independent, community-supported software for describing and comparing microbial communities. Appl Environ Microbiol. (2009) 75:7537–41. doi: 10.1128/AEM.01541-09
35. Fard, SH, Toghyani, M, and Tabeidian, SA. Effect of oyster mushroom wastes on performance, immune responses and intestinal morphology of broiler chickens. Int J Recycl Org Waste Agric. (2014) 3:141–6. doi: 10.1007/s40093-014-0076-9
36. Aida, FMNA, Shuhaimi, M, Yazid, M, and Maaruf, AG. Mushroom as a potential source of prebiotics: a review. Trends Food Sci Technol. (2009) 20:567–75. doi: 10.1016/j.tifs.2009.07.007
37. Khan, SH, Mukhtar, N, and Iqbal, J. Role of mushroom as dietary supplement on performance of poultry. J Diet Suppl. (2019) 16:611–24. doi: 10.1080/19390211.2018.1472707
38. El-Deeb, NM, Ibrahim, OM, Mohamed, MA, Farag, MMS, Farrag, AA, and ElAassar, MR. Alginate/κ-carrageenan oral microcapsules loaded with Agaricus bisporus polysaccharides MH751906 for natural killer cells mediated colon cancer immunotherapy. Int J Biol Macromol. (2022) 205:385–95. doi: 10.1016/j.ijbiomac.2022.02.058
39. Zhu, X, Xu, N, Liu, Y, Ai, X, and Yang, Y. The effects of Agaricus bisporus polysaccharides enriched diet on growth, nonspecific immunity and disease resistance in crayfish (Procambarus clarkii). Aquaculture. (2022) 24:101168. doi: 10.1016/j.aqrep.2022.101168
40. Harikrishnan, R, Devi, G, Van Doan, H, Balasundaram, C, Thamizharasan, S, Hoseinifar, SH, et al. Effect of diet enriched with Agaricus bisporus polysaccharides (ABPs) on antioxidant property, innate-adaptive immune response and pro-anti inflammatory genes expression in Ctenopharyngodon idella against Aeromonas hydrophila. Fish Shellfish Immunol. (2021) 114:238–52. doi: 10.1016/j.fsi.2021.04.025
41. Chen, S, Zhou, Y, Chen, Y, and Gu, J. FASTP: an ultra-fast all-inone FASTQ preprocessor. Bioinformatics. (2018) 34:i884–90. doi: 10.1093/bioinformatics/bty560
42. Moradzadeh-Somarin, Z, Seifdavati, J, Yalchi, T, Abdi-Benemar, H, Seyedsharifi, R, Elghandour, MMMY, et al. Valorization of dietary edible mushrooms waste: chemical and physical properties, nutrient digestibility, microbial protein synthesis and nitrogen balance in sheep. J Sci Food Agric. (2021) 101:5574–82. doi: 10.1002/jsfa.11208
43. Hosseini, SS, Sudaagar, M, Zakariaee, H, Paknejad, H, Baruah, K, and Norouzitalab, P. Evaluation of the synbiotic effects of Saccharomyces cerevisiae and mushroom extract on the growth performance, digestive enzyme activity, and immune status of zebrafish danio rerio. BMC Microbiol. (2024) 24:331. doi: 10.1186/s12866-024-03459-2
44. Van Soest, PJ. Nutritional ecology of the ruminant. 2nd ed. Ithaca, NY: Cornell University Press (1994).
45. Park, T, Ma, L, Ma, Y, Zhou, X, Bu, D, and Yu, Z. Dietary energy sources and levels shift the multi-kingdom microbiota and functions in the rumen of lactating dairy cows. J Anim Sci Biotechnol. (2020) 11:66–16. doi: 10.1186/s40104-020-00461-2
46. Orellana, LH, Ben Francis, T, Ferraro, M, Hehemann, JH, Fuchs, BM, and Amann, RI. Verrucomicrobiota are specialist consumers of sulfated methyl pentoses during diatom blooms. ISME J. (2022) 16:630–41. doi: 10.1038/s41396-021-01105-7
47. van Vliet, DM, Ayudthaya, SPN, Diop, S, Villanueva, L, Stams, AJM, and Sanchez-Andrea, I. Anaerobic degradation of sulfated polysaccharides by two novel Kiritimatiellales strains isolated from Black Sea sediment. Front Microbiol. (2019) 10:253. doi: 10.3389/fmicb.2019.00253
48. Gharechahi, J, Sarikhan, S, Han, JL, Ding, X, and Salekdeh, GH. Functional and phylogenetic analyses of camel rumen microbiota associated with different lignocellulosic substrates. Npj Biofilms Microbiomes. (2022) 8:46. doi: 10.1038/s41522-022-00309-9
49. Liu, W, Wang, L, Yuan, Q, Hao, W, Wang, Y, Wu, D, et al. Agaricus bisporus polysaccharides ameliorate ulcerative colitis in mice by modulating gut microbiota and its metabolism. Food Funct. (2024) 15:1191–207. doi: 10.1039/D3FO04430K
50. Chen, M, Xie, W, Zhou, S, Ma, N, Wang, Y, Huang, J, et al. A high-concentrate diet induces colonic inflammation and barrier damage in Hu sheep. J Dairy Sci. (2023) 106:9644–62. doi: 10.3168/jds.2023-23359
51. Fujio-Vejar, S, Vasquez, Y, Morales, P, Magne, F, Vera-Wolf, P, Ugalde, JA, et al. The gut microbiota of healthy Chilean subjects reveals a high abundance of the phylum Verrucomicrobia. Front Microb. (2017) 8:1221. doi: 10.3389/fmicb.2017.01221
52. Derrien, M, Van Baarlen, P, Hooiveld, G, Norin, E, Müller, M, and de Vos, WM. Modulation of mucosal immune response, tolerance, and proliferation in mice colonized by the mucin-degrader Akkermansia muciniphila. Front Microb. (2011) 2:166. doi: 10.3389/fmicb.2011.00166
53. Tohno, M, Tanizawa, Y, Sawada, H, Sakamoto, M, Ohkuma, M, and Kobayashi, H. A novel species of lactic acid bacteria, Ligilactobacillus pabuli sp. nov., isolated from alfalfa silage. Int J Syst Evol Microbiol. (2022) 72:005587. doi: 10.1099/ijsem.0.005587
Keywords: heifers, mushrooms, Agaricus bisporus, growth performance, serum immunity, serum antioxidant, rumen microbiota
Citation: Jia P, Lu C, Yang X, Jiang X, Lei H, Lu N and Xia D (2025) Agaricus bisporus stipe fed to dairy heifers: effects on growth performance, immunity and antioxidant capacity, and rumen microbiota. Front. Vet. Sci. 12:1556330. doi: 10.3389/fvets.2025.1556330
Received: 06 January 2025; Accepted: 28 February 2025;
Published: 21 March 2025.
Edited by:
Weiwei Wang, Guizhou University, ChinaReviewed by:
Bing Wang, China Agricultural University, ChinaCopyright © 2025 Jia, Lu, Yang, Jiang, Lei, Lu and Xia. This is an open-access article distributed under the terms of the Creative Commons Attribution License (CC BY). The use, distribution or reproduction in other forums is permitted, provided the original author(s) and the copyright owner(s) are credited and that the original publication in this journal is cited, in accordance with accepted academic practice. No use, distribution or reproduction is permitted which does not comply with these terms.
*Correspondence: Dong Xia, eGlhZG9uZ0BzYWFzLnNoLmNu
Disclaimer: All claims expressed in this article are solely those of the authors and do not necessarily represent those of their affiliated organizations, or those of the publisher, the editors and the reviewers. Any product that may be evaluated in this article or claim that may be made by its manufacturer is not guaranteed or endorsed by the publisher.
Research integrity at Frontiers
Learn more about the work of our research integrity team to safeguard the quality of each article we publish.