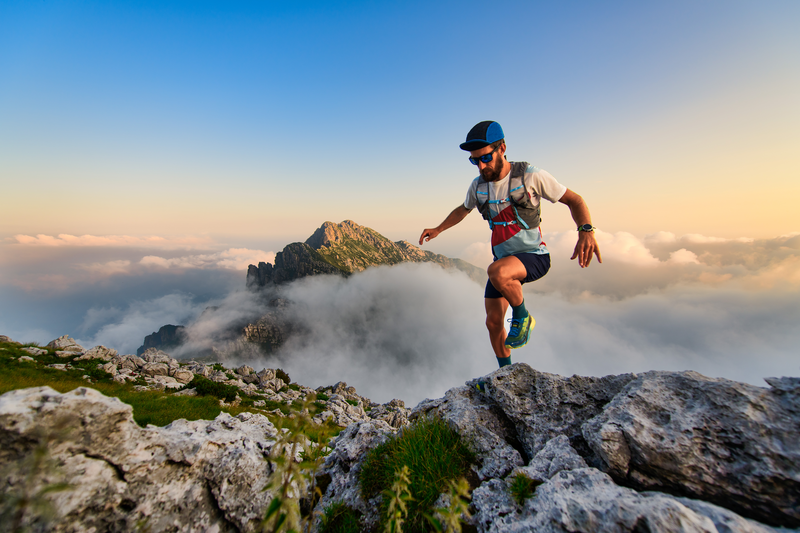
95% of researchers rate our articles as excellent or good
Learn more about the work of our research integrity team to safeguard the quality of each article we publish.
Find out more
ORIGINAL RESEARCH article
Front. Vet. Sci. , 18 March 2025
Sec. Animal Nutrition and Metabolism
Volume 12 - 2025 | https://doi.org/10.3389/fvets.2025.1554502
Introduction: Lactobacillus species have attracted more and more attention as a potential antibiotic substitute for human health and animal production due to their remarkable antibacterial effects. However, the underlying mechanism is unclear. This experiment’s goal was to investigate the impacts of lactic acid bacteria (LAB) on the growth performance, carcass characteristics, immune function of broiler chickens and their mechanism.
Methods: One hundred and eighty 1-day-old AA broilers were used and randomly allocated into 3 treatment groups with 6 replicates of 10 chickens per replicate. The 3 treatment groups were control group (CK), L. acidophilus added group (LAB-E, 1.0 × 108 CFU/kg) for the first 7 days; L. acidophilus added group (LAB-A, 1.0 × 108 CFU/kg) for the whole experimental period. Broilers had free access to water and feed.
Results: The results showed that addition of L. acidophilus for the whole experimental period significantly decreased ADFI, FCR and the abdominal fat percentage of broilers (p < 0.05), tended to increase the levels of IgG in broiler serum (p = 0.093). The LAB-A group had higher HDL-C content and IL-2, IL-4 content, and lower level of LPS in broiler serum compared to the controls (p < 0.05).
Discussion: In conclusion, L. acidophilus improved feed efficiency and immune function of broilers by controlling nutrient metabolism and inflammation responses of broilers. L. acidophilus can be used as a potential substitute for antibiotics in broiler production.
The negative effects of feeding antibiotics on animals, humans and the environment have aroused widespread concern, such as problems with drug residue, antibiotic resistance, and an imbalance in the gut microbiota (1, 2). Finding safe, effective, and environmentally friendly feed additives to replace antibiotics in feed has become a key issue in the development of animal husbandry.
The alternatives include among others prebiotics, enzymes and organic acids, plant essential oils etc. Among them, probiotics are beneficial due to their low cost of production and broad range of use in many host animal types (3–5). The probiotics were finally defined as live micro-organisms that, when administered in adequate amounts, confer a health benefit on the host (6, 7) in 2013 by an expert panel convened by the International Scientific Association for Probiotics and Prebiotics.
Numerous studies have demonstrated that probiotics can regulate the function of the intestinal barriers in poultry (8–11), and positively influence diversity and structure of microbiota, but also nutrient digestibility (12), antioxidant capacity (13) and immune function (14). Probiotic bacteria called L. plantarum, which have a wide range of activities, are employed extensively in human and veterinary health as well as the food sector (15). Due to its positive effects on immunology, intestinal barrier function, and cell apoptosis inhibition, L. plantarum has the potential to be a widely utilized dietary probiotic (16).
Dowarah et al. (17) isolated an effective probiotic LAB named Lacp from piglet feces and found that supplementation of this probiotic improved FCR compared to control (p < 0.001). According to several studies, LAB addition in the diet enhanced broiler performance during the starter phase (18, 19). Olnood et al. (20) found four strains of LAB that altered the gut microflora of birds. Kalavathy et al. (21) discovered that broilers fed a mixture of Lactobacillus strains from 1 to 42 days of age demonstrated improved body weight growth (BWG) and feed conversion ratio (FCR), as well as a hypolipidemic impact. Additionally, it has been observed that chickens fed a Lactobacillus culture constantly show higher BWG (22). According to the data presented in the paper, LP-8 administration considerably enhanced the broiler growth-related matrix and enhanced immune markers in an industrial chicken farming environment (23, 24). Previous studies on the application of L. acidophilus in broiler chickens mainly focused on growth performance, with limited research on the comprehensive effects on production performance, immunity and metabolism. Based on the probiotic properties of L. acidophilus and its potential interaction with the gut microbiota, we hypothesized that the addition of L. acidophilus to broiler chicken diets would enhance growth performance, boost immunity. We anticipate that L. acidophilus can be used as a potential substitute for antibiotics in broiler production. Our study comprehensively analyzes these aspects, aiming to look into how LAB affect the growth performance, carcass characteristics and immune function of broiler chickens and the underlying mechanism.
All animal management and experimental procedures for this study were approved by the Animal Ethic Committee of the Chinese Academy of Agricultural Sciences and performed according to the guidelines for animal experiments set by the National Institute of Animal Health.
One hundred and eighty 1-old Arbor Acres broiler chicks, were randomly assigned into three treatment groups with 6 replicates of 10 chickens per replicate. These 3 treatments were control, LAB-E, LAB-A. The feeding trial lasted for 42 days. Diet (Table 1) and water were provided ad libitum. Bird management followed the Arbor Acre broiler management guidelines.
The L. acidophilus was screened from the mixture of fermented silage and soil by the Institute of Feed Research, Chinese Academy of Agricultural Sciences. The strain was identified by the Institute of Microbiology, Chinese Academy of Sciences and deposited in the China General Microbiological Culture Collection Center (CGMCC, address: No. 3, Yard 1, Beichen West Road, Chaoyang District, Beijing City), and the preservation registration number is CGMCC NO. 14437.
The ingredients and composition of the experimental diets are shown in Table 1.
Chicken body weight (BW) and feed intake (FI) were weekly recorded to recorded to calculate weight gain (WG), and feed conversion ratio (FCR). After chickens’ sacrifice, the FI and BW were recorded, and the average WG and FCR calculated.
On day 42 following 8 h of fasting, all chickens were weighed, and feed intake was measured on a per cage basis. Average daily feed intake (ADFI), average daily gain (ADG), and the feed intake/weight gain ratio (F/G ratio) were calculated. 6 birds per treatment were randomly chosen to analyze the carcass yield. The birds were bled, scalded, and defeathered in a rotary picker. After bleeding, the broilers were defeathered, eviscerated, and discarded the head and feet to determine carcass weight. The abdominal adipose tissue from the surrounding proventriculus and the gizzard down to the cloaca, breast muscle, and legs from each bird were collected and weighed to calculate the carcass characteristics including the dressing percentage, semi-eviscerated percentage, eviscerated percentage, breast muscle percentage, thigh muscle percentage, abdominal fat percentage by the method of Chen et al. (25).
At 42 days of age, blood samples were extracted from the brachial vein of hens. Following centrifugation (3,000 × g, 150 min), serum was kept for subsequent analysis at −20°C.
At d 42, 1 healthy chicken per replicate was randomly chosen and humanely killed after fasting 8 h. Thymus, bursa, and spleens were gathered and weighed. The ratio of organ weight to body weight was used to determine relative organ weights.
ELISA kits (Shanghai Enzyme-linked Biotechnology Co., Ltd) were used to measure the amounts of immunoglobulin A (IgA), immunoglobulin G (IgG), immunoglobulin M (IgM), Interferon-gamma (IFN-γ); tumor necrosis factor-alpha (TNF-α); interleukin 2 (IL-2), interleukin 4 (IL-4) and lipopolysaccharide (LPS).
The serum biochemical indices (Urea; UA, uric acid; NH3; TC, total cholesterol; TG, triglyceride; HDL-C, high-density lipoprotein cholesterol; LDL-C, low-density lipoprotein cholesterol; VLDL-C: Very low-density lipoprotein cholesterol) were determined using the automatic biochemical analyzer (Model 7,600 Series Automatic Analyzer, Hitachi, Japan).
The data was analyzed by a one-factor ANOVA procedure of the SPSS 19.0 software package for Windows (SPSS Inc., Chicago, IL, United States). Duncan’s multiple range test was used to distinguish significant differences between treatment means. The mean and standard error of the mean (SEM) are used to show the results. All statements of significance are based on a probability of p < 0.05.
As shown in Table 2, the ADG among the 3 treatment groups were similar. LAB-A significantly decreased the ADFI and FCR of broilers during d1-21 compared with other groups (p < 0.001). During the whole period, LAB-A significantly decreased ADFI and FCR (p < 0.05).
As shown in Table 3, the LAB addition group had less abdominal fat percentage of broilers (p < 0.05). The other carcass traits, such as dressing, semi-eviscerated, eviscerated, breast muscle and thigh muscle percentage, were not affected by Lactobacillus addition.
As shown in Table 4, LAB addition did not significantly affect immune organ indexes (spleen, thymus, bursa of Fabricius) (p > 0.05).
As shown in Table 5, LAB-A tended to increase the levels of IgG in broiler blood (p = 0.093), but it was not significant.
As shown in Table 6, LAB addition did not significantly affect the content of UREA and UA, but significantly reduced the content of NH3 in serum of broilers (p < 0.01).
As shown in Table 7, LAB-A significantly increased the content of HDL-C in serum of broilers compared with CK or LAB-E group (p < 0.05), but other differences were observed across groups (p > 0.05).
As shown in Table 8, the LAB addition increased the levels of IL-4 and IL-2, and reduced the level of LPS in broiler serum significantly (p < 0.05).
Table 8. Effect of lactobacillus acidophilus on the content of inflammatory factors in serum of broilers.
Numerous studies have shown that LAB, a predominant group in the intestinal tract, are instrumental in maintaining the balance of the gastrointestinal microbiota. They are essential for safeguarding the integrity of the intestinal barrier, enhancing mucosal immunity both in vivo and in vitro, and preventing pathogenic infections (26–29). Thus, lactobacillus is considered to be a type of probiotic alternative to antibiotics to control and prevent animal’s disease (27, 28)and widely used in the field of livestock and poultry production.
Growth performance and carcass characteristics are the most direct indicators of broiler production, which are critical to improving economic performance.
Lactobacillus is a typical probiotic, which can colonize the digestive tract of animals, release a range of digestive enzymes, and enhance the environment of the digestive tract through metabolic processes. Moreover, it has the ability to enhance intestinal development by augmenting the villi height - to - crypt depth ratio in the ileum, thus accelerating nutrient absorption, improving feed conversion efficiency, and promoting animal growth (26, 29–31).
Numerous studies have selected and applied LAB to enhance the growth performance of animals. Salehizadeh et al. (32) used the selected mixture of lactobacillus and commercial probiotics in their experiments, which had a significant effect on the growth performance and carcass characteristics of broilers.
According to Shokryazdan et al. (33), adding an L. aalivarius blend to the ration considerably raised the ADG and feed conversion ratio of broilers. Some studies discovered that, final weight, total weight increase, and average daily growth of broilers fed L. plantarum were all significantly higher than those of the control group, according to Humam et al. (34). This might be attributable to the fact that lactobacillus produce bacteriocins and organic acids, which inhibit the growth and reproduction of pathogenic bacteria in the gut (35). LAB can colonize the digestive tract of animals and produce various digestive enzymes, including amylase and protease, which help promote the absorption of nutrients and improve the feed conversion rate (26, 36). In the present study, we found that LBA-A significantly decreased ADFI, FCR of broilers during the 1–21D and whole period. These results are similar to those obtained in previous studies (37, 38). It may be beneficial for growth performance improvement. Concerning carcass traits, our results are consistent with the result of Junaid et al. (14) that supplementing Lactobacillus did not affect carcass traits in broiler chickens. There is little evidence that probiotics significantly affect carcass percentage and related parameters, such as breast, thigh and drumstick, and wings (39).
Thymus and bursa are the central immune organs of poultry, which affect the cellular and humoral immune function of the body. The spleen, the body’s biggest lymphoid organ, is home to a vast population of macrophages and lymphocytes (14), which is both the peripheral immune organ and the site of lymphocytes to settle and respond to antigen stimulation. For example, the growing relative weight of immune organs is an indication that both the cellular and humoral immune functions have been strengthened (26). Vineetha et al. (40) found that weight of immune organs was higher in the L. plantarum LGFCP4- supplemented group. Awad et al. (22) found that lactobacilli can promote the growth and development of immune organs in chickens. LAB dietary supplementation in this study did not significantly affect the weight of broiler immunological organs. The inconsistency may be attributed to the added dosage of lactic acid bacteria or the small statistical unit-size.
The immunoglobulins play a crucial role in the immune system, thus serum immunoglobulin level is the most commonly used index to measure immune function. IgA is involved in the immune process of mucosal infection, IgG is a reactive immune response antibody with antibacterial and antiviral effects, IgM is involved in the initial immune response and has the same anti-infective effect as IgA (41–44). In the study by Riaz Rajoka et al. (45), LAB strengthened the host’s immune system by boosting the production of IgA and the number of immunological and epithelial cells. In our experiment we found that LAB addition tended to increase the levels of IgG in broiler blood, indicating that lactobacillus enhance immune function of broilers. IgG is mostly produced and released by plasma cells found in lymph nodes and the spleen. Vineetha et al. (40) proved that the weight of immune organs was higher in L. plantarum LGFCP4-supplemented group. In this study, dietary supplementation with LAB for the whole experimental period tend to increase the immunoglobulin levels of broilers, which may show that the dosage of lactic acid bacteria needs to be further adjusted.
In poultry, amino acid metabolism is primarily regulated by the purine nucleotide cycle, with uric acid being the major end product. External microorganisms can further break down uric acid into urea through the enzymatic activities of uric acid oxidase and allantoinase. Subsequently, microbial urease facilitates the conversion of urea into ammonia (46). The concentration of urea, a metabolite of protein, in the bloodstream serves as an indicator of the bird’s immunological status. In this study, compared with the control group, both experimental groups, LAB - A and LAB - B, showed a decrease in the levels of uric acid and urea nitrogen in broilers. Moreover, there was a significant reduction in NH3 levels in broilers compared to the control group, indicating an effective regulation of nitrogen metabolism in chickens.
Our results showed that the addition of LAB could reduce the content of HDL-C and TC in blood of broilers. LAB were able to assimilate cholesterol significantly in-vitro, Cell wall binding and incorporation of cholesterol within their phospholipid layer was reported as a possible mechanism for cholesterol assimilation (47), and bile salt hydrolytic activity (BSH) of probiotics also stand as the most significant mechanisms for cholesterol removal (48). Broiler triglyceride and cholesterol levels were significantly reduced by both native and commercial LAB, according to Salehizadeh et al. (32). Moreover, Shokryazdan et al. (33) found a similar effects of LAB probiotic strains on serum lipids of chickens. Jeon, Lee, and Chang (49) also confirmed that probiotic LAB (L. plamtarum E.M) showed a significant hypocholesterolemic effect and metabolism improvement in rats. While the exact mechanisms are unknown, there are a number of researchers that are focused on either increasing the breakdown and excretion of cholesterol or decreasing its synthesis (50). And another mechanisms were also proposed, some lactic acid bacteria are able to assimilate cholesterol into their cells resulting in cholesterol reduction of surrounding environment (51).
Cytokines are crucial for the inflammatory response and immune system (52). Assessing the production of cytokines is essential for assessing the cell-mediated immune response (53). Proinflammatory (IL-1β, IL-2, IL-6, IFN-γ, and TNF-α) and anti-inflammatory (IL-4, IL-10, and TGF-β) cytokines are the two main categories of cytokines. Proinflammatory cytokines are crucial for the innate and adaptive immune responses to form and function. Pathogens are removed from the host by these immune cells, while their overexpression is linked to pathological immune system disorders and may have detrimental impacts on host growth and health (54). Disease susceptibility, immunological tolerance, the magnitude of the inflammatory response, and antibody production are all modulated by anti - inflammatory cytokines (55).
LAB has been shown to control the expression of proinflammatory cytokines (such as IL-1β, IL-6, IL-10, TNF-α, and IFN-γ) and lessen inflammation in animals, including broiler chicks (56, 57). LAB generates pro-inflammatory cytokines and chemokines, release antimicrobial peptides, and activate NF-κB and MAPK signal pathways via PRRs (58). NF-κB is thought to be a significant factor in the inflammatory response and has the ability to control the expression of several cytokines, including TNF-α, IL-6, and interferon-γ (IFN-γ) (27). In our study, LAB supplementation significantly increased the levels of IL-4 and IL-2, especially in LAB-A. During the process of immune activation and regulation, IL - 2 exerts extensive up - regulative effects on not only the proliferation and differentiation of effector T cells but also on B lymphocytes. Moreover, it enables antigen dose sparing while enhancing both antigen - specific and innate immune responses (59). IL-4 has been proven to be positively correlated to IgA, which is known to control inflammation mechanisms and activate humoral immunity (60). And IgA levels have been observed to be modulated by expression of these factors (61). Lactobacillus strains have been reported to elicit positive immunomodulatory effects by regulating expression of key immune mediators (62). Our research suggests that dietary L. acidophilus treatment enhances proinflammatory and anti-inflammatory cytokine release, which in turn boosts cell-mediated immunological competence. Consequently, one of the key mechanisms underlying the immunomodulatory effects of the LAB may be the regulation of the expression of both inflammatory and anti - inflammatory cytokines, along with other immune mediators.
Lipopolysaccharide (LPS) is essential for the cell wall of gram-negative bacteria and is involved in the inflammatory reactions of hosts that E. coli challenges (63). Severe pathogen-induced inflammatory reactions can result in tissue damage, which is associated by elevated serum LPS levels and impaired development capacity. In this study, LAB addition reduced the level of LPS in broiler serum, which may mean the strains could alleviate the inflammation response.
L. acidophilus supplemented with diets decreased ADFI and F/G, reduced abdominal fat, and NH3 and HDL-C, which suggests that Lactobacillus acidophilus can improve feed conversion rate by controlling nutrient metabolism of broiler. Moreover, L. acidophilus increased the level of IL4 and IL-2, reduced LPS level in serum of broilers, which means that L. acidophilus may improve health through reducing inflammation responses of broilers.
In summary, this study found that the addition of L. acidophilus improved feed efficiency, antioxidant and immune function by regulating their nutrient metabolism and inflammatory responses. L. acidophilus holds significant potential as a substitute for antibiotics in broiler production, providing strong evidence for its beneficial impacts on broiler chickens.
The raw data supporting the conclusions of this article will be made available by the authors, without undue reservation.
The animal study was approved by Chinese Academy of Agricultural Sciences (statement no. AEC-CAAS-20191106) in China. The study was conducted in accordance with the local legislation and institutional requirements.
JL: Conceptualization, Data curation, Writing – original draft. HG: Conceptualization, Formal analysis, Writing – review & editing. RJ: Data curation, Formal analysis, Writing – original draft. SL: Data curation, Software, Writing – review & editing. ZC: Investigation, Software, Visualization, Writing – review & editing. AZ: Investigation, Methodology, Visualization, Writing – review & editing. WC: Investigation, Software, Supervision, Writing – review & editing. GL: Methodology, Project administration, Writing – review & editing.
The author(s) declare that financial support was received for the research and/or publication of this article. National Key R&D Program of China, Key Technologies for the Highly Efficient Utilization of Waste Resources (2023YFD1301603).
The authors declare that the research was conducted in the absence of any commercial or financial relationships that could be construed as a potential conflict of interest.
The authors declare that no Gen AI was used in the creation of this manuscript.
All claims expressed in this article are solely those of the authors and do not necessarily represent those of their affiliated organizations, or those of the publisher, the editors and the reviewers. Any product that may be evaluated in this article, or claim that may be made by its manufacturer, is not guaranteed or endorsed by the publisher.
1. Cheng, G, Hao, H, Xie, S, Wang, X, Dai, M, Huang, L, et al. Antibiotic alternatives: the substitution of antibiotics in animal husbandry? Front Microbiol. (2014) 5:217. doi: 10.3389/fmicb.2014.00217
2. Stanton, TB. A call for antibiotic alternatives research. Trends Microbiol. (2013) 21:111–3. doi: 10.1016/j.tim.2012.11.002
3. Gaggìa, F, Mattarelli, P, and Biavati, B. Probiotics and prebiotics in animal feeding for safe food production. Int J Food Microbiol. (2010) 141:S15–28. doi: 10.1016/j.ijfoodmicro.2010.02.031
4. Mehdi, Y, Létourneau-Montminy, M-P, Gaucher, M-L, Chorfi, Y, Suresh, G, Rouissi, T, et al. Use of antibiotics in broiler production: global impacts and alternatives. Anim Nutr. (2018) 4:170–8. doi: 10.1016/j.aninu.2018.03.002
5. Sopková, D, Hertelyová, Z, Andrejčáková, Z, Vlčková, R, Gancarčíková, S, Petrilla, V, et al. The application of probiotics and flaxseed promotes metabolism of N-3 polyunsaturated fatty acids in pigs. J Appl Anim Res. (2017) 45:93–8. doi: 10.1080/09712119.2015.1124333
6. Dong, ZL, Wang, YW, Song, D, Hou, YJ, Wang, WW, Qi, WT, et al. The effects of dietary supplementation of pre-microencapsulated enterococcus fecalis and the extract of camellia oleifera seed on growth performance, intestinal morphology, and intestinal mucosal immune functions in broiler chickens. Anim Feed Sci Technol. (2016) 212:42–51. doi: 10.1016/j.anifeedsci.2015.11.014
7. Ramos, OY, Basualdo, M, Libonatti, C, and Vega, MF. Current status and application of lactic acid bacteria in animal production systems with a focus on Bacteria from honey bee colonies. J Appl Microbiol. (2020) 128:1248–60. doi: 10.1111/jam.14469
8. Aliakbarpour, HR, Chamani, M, Rahimi, G, Sadeghi, AA, and Qujeq, D. The bacillus subtilis and lactic acid bacteria probiotics influences intestinal mucin gene expression, histomorphology and growth performance in broilers. Asian Australas J Anim Sci. (2012) 25:1285–93. doi: 10.5713/ajas.2012.12110
9. Rajput, IR, Li, LY, Xin, X, Wu, BB, Juan, ZL, Cui, ZW, et al. Effect of Saccharomyces boulardii and Bacillus subtilis B10 on intestinal ultrastructure modulation and mucosal immunity development mechanism in broiler chickens. Poult Sci. (2013) 92:956–65. doi: 10.3382/ps.2012-02845
10. Tsirtsikos, P, Fegeros, K, Balaskas, C, Kominakis, A, and Mountzouris, KC. Dietary probiotic inclusion level modulates intestinal mucin composition and mucosal morphology in broilers. Poult Sci. (2012) 91:1860–8. doi: 10.3382/ps.2011-02005
11. Waititu, SM, Yitbarek, A, Matini, E, Echeverry, H, Kiarie, E, Rodriguez-Lecompte, JC, et al. Effect of supplementing direct-fed microbials on broiler performance, nutrient digestibilities, and immune Responses1. Poult Sci. (2014) 93:625–35. doi: 10.3382/ps.2013-03575
12. Rodjan, P, Soisuwan, K, Thongprajukaew, K, Theapparat, Y, Khongthong, S, Jeenkeawpieam, J, et al. Effect of organic acids or probiotics alone or in combination on growth performance, nutrient digestibility, enzyme activities, intestinal morphology and gut microflora in broiler chickens. J Anim Physiol Anim Nutr. (2018) 102:e931–40. doi: 10.1111/jpn.12858
13. Inatomi, T, and Otomaru, K. Effect of dietary probiotics on the semen traits and antioxidative activity of male broiler breeders. Sci Rep. (2018) 8:5874. doi: 10.1038/s41598-018-24345-8
14. Junaid, N, Biswas, A, Kumawat, M, and Mandal, AB. Production performance, immune response and carcass traits of broiler chickens fed diet incorporated with probiotics. Indian J Anim Res. (2018) 52:1597–602. doi: 10.18805/ijar.B-3420
15. Dinev, T, Beev, G, Tzanova, M, Denev, S, Dermendzhieva, D, and Stoyanova, A. Antimicrobial activity of Lactobacillus plantarum against pathogenic and food spoilage microorganisms: a review. Bulg J Vet Med. (2018) 21:253–68. doi: 10.15547/bjvm.1084
16. Wu, Y, Wang, B, Zeng, Z, Liu, R, Tang, L, Gong, L, et al. Effects of probiotics Lactobacillus plantarum 16 and Paenibacillus polymyxa 10 on intestinal barrier function, antioxidative capacity, apoptosis, immune response, and biochemical parameters in broilers. Poult Sci. (2019) 98:5028–39. doi: 10.3382/ps/pez226
17. Dowarah, R, Verma, AK, Agarwal, N, Singh, P, and Singh, BR. Selection and characterization of probiotic lactic acid bacteria and its impact on growth, nutrient digestibility, health and antioxidant status in weaned piglets. PLoS One. (2018) 13:e0192978. doi: 10.1371/journal.pone.0192978
18. Bai, SP, Wu, AM, Ding, XM, Lei, Y, Bai, J, Zhang, KY, et al. Effects of probiotic-supplemented diets on growth performance and intestinal immune characteristics of broiler chickens. Poult Sci. (2013) 92:663–70. doi: 10.3382/ps.2012-02813
19. Zulkifli, I, Abdulllah, N, Azrin, NM, and Ho, YW. Growth performance and immune response of two commercial broiler strains fed diets containing Lactobacillus cultures and oxytetracycline under heat stress conditions. Br Poult Sci. (2000) 41:593–7. doi: 10.1080/713654979
20. Olnood, CG, Beski, SSM, Choct, M, and Iji, PA. Novel probiotics: their effects on growth performance, gut development, microbial community and activity of broiler chickens. Anim Nutr. (2015) 1:184–91. doi: 10.1016/j.aninu.2015.07.003
21. Kalavathy, R, Abdullah, N, Jalaludin, S, and Ho, YW. Effects of Lactobacillus cultures on growth performance, abdominal fat deposition, serum lipids and weight of organs of broiler chickens. Br Poult Sci. (2003) 44:139–44. doi: 10.1080/0007166031000085445
22. Awad, WA, Ghareeb, K, Abdel-Raheem, S, and Böhm, J. Effects of dietary inclusion of probiotic and synbiotic on growth performance, organ weights, and intestinal histomorphology of broiler chickens. Poult Sci. (2009) 88:49–56. doi: 10.3382/ps.2008-00244
23. Gao, P, Ma, C, Sun, Z, Wang, L, Huang, S, Su, X, et al. Feed-additive probiotics accelerate yet antibiotics delay intestinal microbiota maturation in broiler chicken. Microbiome. (2017) 5:91. doi: 10.1186/s40168-017-0315-1
24. Wang, L, Zhang, J, Guo, Z, Kwok, L, Ma, C, Zhang, W, et al. Effect of Oral consumption of probiotic Lactobacillus planatarum P-8 on fecal microbiota, SIgA, SCFAs, and TBAs of adults of different ages. Nutrition. (2014) 30:776–783.e1. doi: 10.1016/j.nut.2013.11.018
25. Chen, X, Zhang, G, Wang, W, Liu, G, Cai, H, Purba, A, et al. Compound non-starch polysaccharide enzymes improve growth performance, slaughter performance, immune function, and apparent utilization rate of nutrients in broiler chickens fed a low-metabolizable energy diet. Front Vet Sci. (2023) 10:1162811. doi: 10.3389/fvets.2023.1162811
26. Chen, F, Gao, SS, Zhu, LQ, Qin, SY, and Qiu, HL. Effects of dietary Lactobacillus rhamnosus CF supplementation on growth, meat quality, and microenvironment in specific pathogen-free chickens. Poult Sci. (2018) 97:118–23. doi: 10.3382/ps/pex261
27. Deng, Z, Hou, K, Zhao, J, and Wang, H. The probiotic properties of lactic acid Bacteria and their applications in animal husbandry. Curr Microbiol. (2022) 79:1–11. doi: 10.1007/s00284-021-02722-3
28. Reuben, RC, Roy, PC, Sarkar, SL, Alam, R-U, and Jahid, IK. Isolation, characterization, and assessment of lactic acid bacteria toward their selection as poultry probiotics. BMC Microbiol. (2019) 19:253. doi: 10.1186/s12866-019-1626-0
29. Zhang, L, Li, J, Yun, TT, Qi, WT, Liang, XX, Wang, YW, et al. Effects of pre-encapsulated and pro-encapsulated Enterococcus faecalis on growth performance, blood characteristics, and cecal microflora in broiler chickens. Poult Sci. (2015) 94:2821–30. doi: 10.3382/ps/pev262
30. Forte, C, Manuali, E, Abbate, Y, Papa, P, Vieceli, L, Tentellini, M, et al. Dietary Lactobacillus acidophilus positively influences growth performance, gut morphology, and gut microbiology in rurally reared chickens. Poult Sci. (2018) 97:930–6. doi: 10.3382/ps/pex396
31. Xin, J, Zeng, D, Wang, H, Sun, N, Zhao, Y, Dan, Y, et al. Probiotic Lactobacillus johnsonii BS15 promotes growth performance, intestinal immunity, and gut microbiota in piglets. Probiotics Antimicrob Proteins. (2020) 12:184–93. doi: 10.1007/s12602-018-9511-y
32. Salehizadeh, M, Modarressi, MH, Mousavi, SN, and Ebrahimi, MT. Effects of probiotic lactic acid Bacteria on growth performance, carcass characteristics, hematological indices, humoral immunity, and IGF-I gene expression in broiler chicken. Trop Anim Health Prod. (2019) 51:2279–86. doi: 10.1007/s11250-019-01935-w
33. Shokryazdan, P, Faseleh Jahromi, M, Liang, JB, Ramasamy, K, Sieo, CC, and Ho, YW. Effects of a Lactobacillus salivarius mixture on performance, intestinal health and serum lipids of broiler chickens. PLoS One. (2017) 12:e0175959. doi: 10.1371/journal.pone.0175959
34. Humam, AM, Loh, TC, Foo, HL, Samsudin, AA, Mustapha, NM, Zulkifli, I, et al. Effects of feeding different postbiotics produced by Lactobacillus plantarum on growth performance, carcass yield, intestinal morphology, gut microbiota composition, immune status, and growth gene expression in broilers under heat stress. Animals. (2019) 9:644. doi: 10.3390/ani9090644
35. Chai, C, Guo, Y, Mohamed, T, Bumbie, GZ, Wang, Y, Zeng, X, et al. Dietary Lactobacillus reuteri SL001 improves growth performance, health-related parameters, intestinal morphology and microbiota of broiler chickens. Animals. (2023) 13:1690. doi: 10.3390/ani13101690
36. Wang, J, Yao, L, Su, J, Fan, R, Zheng, J, and Han, Y. Effects of Lactobacillus plantarum and its fermentation products on growth performance, immune function, intestinal pH, and cecal microorganisms of lingnan yellow chicken. Poult Sci. (2023) 102:102610. doi: 10.1016/j.psj.2023.102610
37. De Cesare, A, Sirri, F, Manfreda, G, Moniaci, P, Giardini, A, Zampiga, M, et al. Effect of dietary supplementation with Lactobacillus acidophilus D2/CSL (CECT 4529) on caecum microbioma and productive performance in broiler chickens. PLoS One. (2017) 12:e0176309. doi: 10.1371/journal.pone.0176309
38. Monika, M, Tyagi, JS, Sonale, N, Biswas, A, Murali, D, Sky, AKT, et al. Evaluating the efficacy of Lactobacillus acidophilus derived postbiotics on growth metrics, health, and gut integrity in broiler chickens. Sci Rep. (2024) 14:24768. doi: 10.1038/s41598-024-74078-0
39. Nam, TVB, Anh, LH, Loc, HT, Trang, CTH, Thiet, N, Lan, LTT, et al. Effects of probiotic (Lactobacillus plantarum and Bacillus subtilis) supplementation on mortality, growth performance, and carcass characteristics of native Vietnamese broilers challenged with Salmonella typhimurium. Vet World. (2022) 15:2302–8. doi: 10.14202/vetworld.2022.2302-2308
40. Vineetha, PG, Tomar, S, Saxena, VK, Kapgate, M, Suvarna, A, and Adil, K. Effect of laboratory-isolated Lactobacillus plantarum LGFCP4 from gastrointestinal tract of guinea fowl on growth performance, carcass traits, intestinal histomorphometry and gastrointestinal microflora population in broiler chicken. J Anim Physiol Anim Nutr. (2017) 101:e362–70. doi: 10.1111/jpn.12613
41. Bunker, JJ, Erickson, SA, Flynn, TM, Henry, C, Koval, JC, Meisel, M, et al. Natural polyreactive IgA antibodies coat the intestinal microbiota. Science. (2017) 358, 358:eaan6619. doi: 10.1126/science.aan6619
42. Melo-Gonzalez, F, Kammoun, H, Evren, E, Dutton, EE, Papadopoulou, M, Bradford, BM, et al. Antigen-presenting ILC3 regulate T cell-dependent IgA responses to colonic mucosal Bacteria. J Exp Med. (2019) 216:728–42. doi: 10.1084/jem.20180871
43. Schroeder, HW, and Cavacini, L. Structure and function of immunoglobulins. J Allergy Clin Immunol. (2010) 125:S41–52. doi: 10.1016/j.jaci.2009.09.046
44. de Sousa-Pereira, P, and Woof, JM. IgA: structure, function, and developability. Antibodies. (2019) 8:57. doi: 10.3390/antib8040057
45. Riaz Rajoka, MS, Shi, J, Zhu, J, Shao, D, Huang, Q, Yang, H, et al. Capacity of lactic acid bacteria in immunity enhancement and Cancer prevention. Appl Microbiol Biotechnol. (2017) 101:35–45. doi: 10.1007/s00253-016-8005-7
46. Whang, KY, and Easter, RA. Blood urea nitrogen as an index of feed efficiency and lean growth potential in growing-finishing swine. Asian Australas J Anim Sci. (2000) 13:811–6. doi: 10.5713/ajas.2000.811
47. Ooi, L-G, and Liong, M-T. Cholesterol-lowering effects of probiotics and prebiotics: a review of in vivo and in vitro findings. Int J Mol Sci. (2010) 11:2499–522. doi: 10.3390/ijms11062499
48. Fossi, BT, Ekabe, DE, Toukam, LL, Pambou, HOT, Gagneux-Brunon, A, Nguefeu, CN, et al. Probiotic lactic acid bacteria isolated from traditional cameroonian palm wine and corn beer exhibiting cholesterol lowering activity. Heliyon. (2022) 8:e11708. doi: 10.1016/j.heliyon.2022.e11708
49. Jeon, YB, Lee, J-J, and Chang, HC. Characterization of juice fermented with Lactobacillus plantarum EM and its cholesterol-lowering effects on rats fed a high-fat and high-cholesterol diet. Food Sci Nutr. (2019) 7:3622–34. doi: 10.1002/fsn3.1217
50. Fukushima, M, and Nakano, M. The effect of a probiotic on faecal and liver lipid classes in rats. Br J Nutr. (1995) 73:701–10. doi: 10.1079/BJN19950074
51. Gilliland, SE, Nelson, CR, and Maxwell, C. Assimilation of cholesterol by Lactobacillus acidophilus. Appl Environ Microbiol. (1985) 49:377–81. doi: 10.1128/aem.49.2.377-381.1985
52. Postal, M, and Appenzeller, S. The importance of cytokines and autoantibodies in depression. Autoimmun Rev. (2015) 14:30–5. doi: 10.1016/j.autrev.2014.09.001
53. Siebert, JC, and Walker, EB. Monitoring cytokine profiles during immunotherapy. Immunotherapy. (2010) 2:799–816. doi: 10.2217/imt.10.76
54. Smith, AJP, and Humphries, SE. Cytokine and cytokine receptor gene polymorphisms and their functionality. Cytokine Growth Factor Rev. (2009) 20:43–59. doi: 10.1016/j.cytogfr.2008.11.006
55. O’Garra, A, and Vieira, P. TH1 cells control themselves by producing Interleukin-10. Nat Rev Immunol. (2007) 7:425–8. doi: 10.1038/nri2097
56. Chen, C-Y, Tsen, H-Y, Lin, C-L, Yu, B, and Chen, C-S. Oral administration of a combination of select lactic acid bacteria strains to reduce the Salmonella invasion and inflammation of broiler chicks. Poult Sci. (2012) 91:2139–47. doi: 10.3382/ps.2012-02237
57. Park, J-E, Oh, S-H, and Cha, Y-S. Lactobacillus brevis OPK-3 isolated from kimchi inhibits adipogenesis and exerts anti-inflammation in 3T3-L1 adipocyte. J Sci Food Agric. (2014) 94:2514–20. doi: 10.1002/jsfa.6588
58. Bergstrom, KSB, and Xia, L. Mucin-type O-Glycans and their roles in intestinal homeostasis. Glycobiology. (2013) 23:1026–37. doi: 10.1093/glycob/cwt045
59. Gao, X, Ren, X, Zhang, S, Song, H, Guo, X, Jia, H, et al. Interleukin-2 shows high adjuvanticity for an inactivated vaccine against duck tembusu virus disease. Poult Sci. (2020) 99:6454–61. doi: 10.1016/j.psj.2020.08.022
60. Šefcová, M, Larrea-Álvarez, M, Larrea-Álvarez, C, Karaffová, V, Revajová, V, Gancarčíková, S, et al. Lactobacillus fermentum administration modulates cytokine expression and lymphocyte subpopulation levels in broiler chickens challenged with Campylobacter coli. Foodborne Pathog Dis. (2020) 17:485–93. doi: 10.1089/fpd.2019.2739
61. Al-Banna, NA, Cyprian, F, and Albert, MJ. Cytokine responses in campylobacteriosis: linking pathogenesis to immunity. Cytokine Growth Factor Rev. (2018) 41:75–87. doi: 10.1016/j.cytogfr.2018.03.005
62. Hu, J-L, Yu, H, Kulkarni, RR, Sharif, S, Cui, SW, Xie, M-Y, et al. Modulation of cytokine gene expression by selected Lactobacillus isolates in the ileum, caecal tonsils and spleen of Salmonella-challenged broilers. Avian Pathol. (2015) 44:463–9. doi: 10.1080/03079457.2015.1086725
Keywords: Lactobacillus acidophilus , broiler chicken, growth performance, carcass characteristics, immunity
Citation: Liu J, Gu H, Jia R, Li S, Chen Z, Zheng A, Chang W and Liu G (2025) Effects of Lactobacillus acidophilus on production performance and immunity of broiler chickens and their mechanism. Front. Vet. Sci. 12:1554502. doi: 10.3389/fvets.2025.1554502
Received: 02 January 2025; Accepted: 03 March 2025;
Published: 18 March 2025.
Edited by:
Panagiotis E. Simitzis, Agricultural University of Athens, GreeceReviewed by:
Ilias Giannenas, Aristotle University of Thessaloniki, GreeceCopyright © 2025 Liu, Gu, Jia, Li, Chen, Zheng, Chang and Liu. This is an open-access article distributed under the terms of the Creative Commons Attribution License (CC BY). The use, distribution or reproduction in other forums is permitted, provided the original author(s) and the copyright owner(s) are credited and that the original publication in this journal is cited, in accordance with accepted academic practice. No use, distribution or reproduction is permitted which does not comply with these terms.
*Correspondence: Wenhuan Chang, Y2hhbmd3ZW5odWFuQGNhYXMuY24=; Guohua Liu, bGl1Z3VvaHVhQGNhYXMuY24=
†These authors have contributed equally to this work
Disclaimer: All claims expressed in this article are solely those of the authors and do not necessarily represent those of their affiliated organizations, or those of the publisher, the editors and the reviewers. Any product that may be evaluated in this article or claim that may be made by its manufacturer is not guaranteed or endorsed by the publisher.
Research integrity at Frontiers
Learn more about the work of our research integrity team to safeguard the quality of each article we publish.