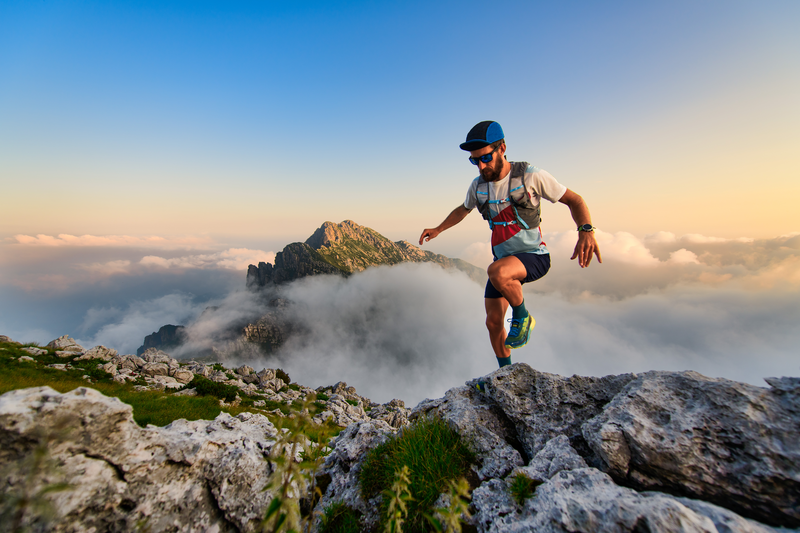
95% of researchers rate our articles as excellent or good
Learn more about the work of our research integrity team to safeguard the quality of each article we publish.
Find out more
ORIGINAL RESEARCH article
Front. Vet. Sci. , 19 March 2025
Sec. One Health
Volume 12 - 2025 | https://doi.org/10.3389/fvets.2025.1551710
This article is part of the Research Topic Emerging Zoonotic Diseases: Understanding and Mitigating Risks at Animal-Human Interfaces View all 7 articles
Introduction: Mycobacterium tuberculosis complex (MTBC) infections are characterized by the development of granulomatous lesions in different parts of the bodies of animals and humans. MTBC infections cause significant economic and public health consequences in Ethiopia. However, there is a shortage of epidemiological data on MTBC infections in the pastoral regions of the country. The objective of the present study was to investigate the epidemiology of MTBC infections in cattle and humans in the remote pastoral setting of southern Ethiopia.
Methods: A cross-sectional study design was used to recruit 2,396 cattle and 1,200 human presumptive tuberculosis (TB) cases for this study from the southern pastoral districts of Ethiopia. The single intradermal comparative cervical tuberculin test (SICCTT) was used to screen for bovine TB in the cattle, while mycobacterial culture and spoligotyping were used to identify mycobacterial species and strains in the pastoralists.
Results: The herd and animal prevalences of bovine TB were 14.9% [95% confidence interval (CI) = 10.2–19.5%] and 3.2% (95% CI: 2.5–4.0), respectively. The herd prevalence was associated with the districts (χ2 = 40.10, p < 0.001). Based on the multivariable binary logistic regression analysis, the male animals were 1.77 (95% CI: 1.02–3.05) times more likely to be TB positive than the female animals. Similarly, the cattle kept in the Dasenech and Benetsemi districts were 10.65 (95% CI: 2.47–45.87) and 22.94 (95% CI: 5.48–95.94) times more likely to be TB positive than the cattle kept in the Selamago district, respectively. Mycobacterial culture positivity was 13.4%, while spoligotyping identified Euro-American (EA), East African-Indian (EAI), Indo-Oceanic (IO), lineage 7, M. bovis, and M. africanum as the major lineages, with proportions of 67.3% (105/156), 22.4% (35/156), 6.4% (10/156), 1.9% (3/156), 1.3% (2/156), and 0.6% (1/156), respectively.
Conclusion: In general, the prevalence of bovine TB was relatively lower than that recorded in intensive dairy farms in central Ethiopia. Three species of MTBC, namely M. tuberculosis, M. africanum, and M. bovis, were isolated from the pastoralists of southern Ethiopia. The isolation of M. bovis from the pastoralists could suggest its zoonotic transmission from cattle to humans.
Mycobacterium tuberculosis complex (MTBC) causes mycobacterial diseases in animals and humans. Mycobacterial infections are characterized by the development of progressive granulomatous lesions in different tissues. The major disease-causing members of MTBC are M. tuberculosis, M. bovis, M. caprae, and M. africanum (1). Bovine tuberculosis (TB) is primarily caused by M. bovis and is a World Organization for Animal Health (WOAH)-listed disease. Bovine TB affects cattle health, reduces the productivity of infected cattle, restricts the trading of cattle, and affects genetic improvement (2). In addition, although M. tuberculosis is the major cause of human TB, M. bovis is transmitted to humans through the consumption of contaminated animal products or the inhalation of aerosol droplets, causing a significant public health problem (3). Historical data have indicated a direct correlation between M. bovis infection in cattle and human populations (4). However, there is a shortage of data on the epidemiology of mycobacterial infections in cattle and humans in developing countries (5).
According to the WOAH (6), bovine TB remains endemic and largely uncontrolled in Africa, Asia, Latin America, and most countries in the Middle East. Bovine TB is a legally notifiable disease in livestock in several African countries (7), although it is widespread in livestock at the continental level (8). In the East African region, existing evidence generally suggests a low prevalence of bovine TB in both wild and domestic animals (9), although high prevalence values have been recorded in intensive husbandry systems in Ethiopia, Uganda, and Tanzania (10). In addition, high prevalence was reported in pastoral livestock in Uganda (11).
In Ethiopia, individuals living close to the livestock–human interface and consuming mostly unpasteurized milk and dairy products are at risk of infection with M. bovis (8). In addition, infection with HIV/AIDS facilitates the transmission and progression of TB in humans. Subsequently, studies have shown a significantly increased proportion of M. bovis infections in individuals co-infected with HIV and TB (12–14). This observation highlights the role of M. bovis in public health in countries endemic to bovine TB (15), although Ethiopian authors have reported a low prevalence of M. bovis in human extrapulmonary TB cases (16).
Epidemiological data have identified poor or absent control strategies, uncontrolled movements of animals, lack of herd screening for bovine TB, increased contact between different herds, and contact with wild species as risk factors for the transmission of bovine TB (17, 18). Pastoralists and their animals are considered to be at high risk of contracting TB because of their lifestyles. Animals congregate on grazing lands and watering points, which creates a conducive environment for the transmission of TB among animals. In addition, pastoralists live in close contact with their animals and also consume raw milk, which facilitates the transmission of TB from animals to pastoralists (19). A few studies have been conducted on TB in the pastoral zones of Ethiopia (20–22). M. bovis, M. tuberculosis, and NTM were isolated as causative agents of TB in animals in the pastoral zones of Ethiopia (23, 24). Additional studies are required on mycobacterial infections in animals and pastoralists in a comprehensive way so that adequate data are generated and used for planning appropriate control strategies. Therefore, the objective of the present study was to investigate the epidemiology of MTBC infections in cattle and pastoralists in the remote pastoral setting of southern Ethiopia.
This study was conducted in the South Omo Zone of southern Ethiopia, located near the border with Kenya (25), as shown in Figure 1. The administrative center of the Zone is located in Jinka town. The zone was named after the Omo River, which flows through the zone into Lake Turkana in Kenya. South Omo Zone is the most sparsely populated part of Ethiopia, inhabited by 16 ethnic groups (25). The livestock found in the South Omo Zone include cattle, sheep, goats, donkeys, camels. Accordingly, the livestock population of the study area is estimated as follows: cattle 1,134,120; sheep 489,449; goats 1,230,399; donkeys 90,630; camels 495; poultry 424,538; and bee colonies 132,500 (South Omo Zone Agricultural and Rural Development Offices, 2014).
Figure 1. Study districts in South Omo Zone. South Omo Zone is located in the Southern Nations, Nationalities, and People’s Region, Ethiopia. Reproduced with permission from Enyew (27).
A cross-sectional study was carried out from August 2020 to December 2022 on the indigenous zebu cattle found in the remote pastoral setting of southern Ethiopia. Five districts were included in the study, and settlements (villages) in each sub-district were selected based on the inclusion criteria (accessibility, security, and willingness of pastoralists to participate in the research) and after obtaining consent from the responsible body. Cattle herds were selected using cluster sampling, where each cattle herd unit was considered a cluster. The sample size was calculated using the cluster sampling formula, as described by Bennett et al. (26). The initial sample size was determined based on a 95% confidence level, a 0.05 margin of error, and a 0.8% prevalence, as described by Tschopp et al. (9), resulting in a sample size of 246 per district. The design effect was calculated using the formula from Bennett et al. (26), with a rho(p) value of 10%, and 15 animals were selected from each cluster. Consequently, the total sample size was calculated to be 600 animals per district from 40 clusters, which resulted in a total sample size of 3,000 animals across five districts. However, as not all owners availed their animals during the second reading, complete results could not be obtained for all the 600 animals per district.
Regarding the sampling, a complete list of sub-districts and villages within the sub-districts was obtained from each district agricultural office. Since the cattle of all villagers were kept together during the day, at least during grazing and watering, each village was considered one large herd for the assessment of tuberculin reactivity status. Herd selection was made proportionally to represent each cluster within the large herd. Thus, cattle owned by one or more owners, where the animals shared common grazing sites and watering points, were kept at night at a common site (Figure 2), and moved together during migration, were considered a herd. Animals younger than 6 months of age were excluded. The demographic data of each study animal were recorded. All animals included in the study were dewormed. In total, 2,396 cattle that fulfilled the inclusion criteria were tested using the SICCTT.
Figure 2. Single intradermal comparative cervical tuberculin test. (A) Gathering of the tested cattle in one place. (B) Shaving of two sites on the middle-left side of the neck. (C) Measuring the skin thickness with a caliper. (D) Injection of aliquots of 0.1 mL of avian purified protein derivative (PPD) and 0.1 mL of bovine PPD into the dermis of the cattle. (E) Measuring the skin thickness at each injection site after 72 h.
On the other hand, sample size calculation was not performed to estimate the sample size for human participants, as the objective of the human study was to evaluate the transmission of MTBC between cattle and humans. Since cattle are central to the various social events of the South Omo Zone pastoralists, such as those of the Hammer pastoralists, the contact between cattle and humans is very frequent (Figure 3). This contact is expected to facilitate the transmission of mycobacterial infections between humans and cattle. Due to security and logistic problems, it was not possible to test the owners of the cattle with bovine TB. Instead, all human presumptive TB cases were recruited and used for bacterial isolation and identification to estimate the burden of M. bovis as a causative agent of human TB in the South Omo Zone. Accordingly, 1,200 presumptive cases were recruited and used for sample collection.
Figure 3. Hammer bull jumping ceremony. (A) Gathering of the male cattle at the ceremony location. (B) Lining up of the cattle in a row by the men of the tribes (C) Leaping up onto and running over the deep row of lined cattle.
Demographic data for each study cattle (sex, age, body condition score, BCS) were collected when the study animals were tested for bovine TB. The ages of the cattle were determined using dental eruption and wear according to the established procedure (28). The body condition of each of the study cattle was scored using previously established guidelines (29).
The single intradermal comparative cervical tuberculin test (SICCTT) was performed using both bovine purified protein derivative (PPD) and avian PPD obtained from Prionics, Lelystad, The Netherlands (Figure 2). Two sites on the middle-left side of the neck, separated by 12 cm, were shaved, and skin thicknesses were measured with a caliper. Aliquots of 0.1 mL of 2,500 IU per ml of the avian PPD and 0.1 mL of 2000 IU per ml of the bovine PPD were injected into the dermis of the study animals. Subsequently, the skin thickness at each injection site was measured again after 72 h. The interpretation of the result was based on the recommendation of the WOAH (30).
Health centers and a hospital providing healthcare services in the study zone were used for the recruitment of presumptive TB cases and the collection of samples for mycobacterial culture. Sputum samples were collected on a regular basis when patients submitted samples for the routine diagnosis of TB. In addition, fine needle aspirates (FNAs) were collected from the extrapulmonary TB cases by a pathologist. In total, 1,200 samples (1,166 sputum and 34 FNA samples) were collected for laboratory analysis. Both the sputum and FNA samples were stained with the Ziehl–Neelsen stain for the detection of acid-fast bacilli. The remaining samples were transported to the TB laboratory at Jinka hospital, in a cold chain for culturing. The samples were stored at 20°C in a freezer until thawed and processed for culturing.
The FNA samples were directly inoculated onto the LJ medium, while the sputum samples were processed (decontaminated and neutralized) for culturing according to the standard operating procedure described earlier (31). In brief, an equal volume of 4% NaOH was mixed with the sputum samples, and the mixture was centrifuged at 3000 rpm for 15 min at room temperature. After decanting the supernatant, the sediment was neutralized with 2 N HCl using phenol red as an indicator. Neutralization was achieved when the color of the solution changed from purple to yellow. Thereafter, 100 μL of the suspension was inoculated onto two sterile LJ medium slopes, which were enriched with either pyruvate or glycerol. The inoculated media were then incubated at 37°C in a slanted position for 1 week and in an upright position for 4–5 weeks. The growth of mycobacteria was monitored every week for up to 8 weeks. Slants with no evidence of growth after 8 weeks were considered negative (32). Specimens with colony growth were examined for acid-fast bacilli (AFB) after staining with the Ziehl–Neelsen stain.
The colonies positive for acid-fast bacilli (AFB) were harvested and re-suspended in 200 μL sterile distilled water. Afterward, the suspension was inactivated by heating at 80°C for 45 min in a water bath to release the DNA. Spoligotyping was performed following the procedure described by Kamerbeek et al. (33). The laboratory results of spoligotyping were interpreted in binary format, and lineages were assigned using an updated version of SITVITWEB (34). Major lineages were assigned using the “TB insight” database. Isolates that exhibited similar patterns to those in the SITVIT database were assigned shared international types (SITs), i.e., SIT numbers. Isolates not assigned to shared international types (i.e., SIT numbers) were referred to as “Orphans.”
The study obtained ethical clearance from the Aklilu Lemma Institute of Pathobiology (ALIPB), Addis Ababa University (Ethiopia), the Institutional Review Board (ALIPB/IRB/22-B/2012/13), and the National Research Ethics Committee (Ref no. 3.10/785/07). Permission to conduct the study was also obtained from the South Omo Zone Health Department. Written informed consent was obtained from each of the study participants, and for those under 18 years of age, consent was also obtained from their parents or guardians.
The data were coded using a Microsoft Excel sheet and transferred and analyzed using SPSS version 28 (IBM SPSS Statistics). Pearson’s chi-squared test was used to evaluate the statistical significance of the associations between different categorical variables and the prevalence of bovine TB. Univariable and multivariable binary logistic regression analyses were used to estimate the strength of the associations between the risk factors and the prevalence of bovine TB. A p < 5% was considered statistically significant. When estimating the effect of the different risk factors in terms of odds ratio (OR) with the corresponding 95% confidence interval, statistical significance was assumed when the confidence interval did not include one.
The herd prevalence of bovine TB was 14.9% (95% CI: 10.2–19.5%) in the South Omo Zone. The herd prevalence significantly differed among the five districts of South Omo Zone (χ2 = 40.9; p < 0.001), while there was no significant difference in the herd prevalence across different herd sizes (p > 0.05) (Table 1).
Table 1. Herd prevalence of bovine TB and associated risk factors in South Omo zone of southern Ethiopia.
The animal prevalence of bovine TB was 3.2% (95% CI: 2.5–4.0) in the South Omo Zone. The animal prevalence differed by age (p < = 0.05), sex (p < 0.01), body condition score (p < 0.05), and districts (p < 0.001) (Table 2).
Table 2. Animal prevalence of bovine TB and associated risk factors in South Omo zone of southern Ethiopia.
The results of the univariable binary logistic regression analysis of the association between the risk factors and the animal prevalence are presented in Table 3. The univariable binary logistic regression analysis showed that the cattle aged between 5 and 9 years and those aged above 9 years were 4.25 (95% CI: 0.55–33.11) and 1.87 (95% CI: 1.18–2.96) times more likely to test positive for bovine TB than the cattle younger than 2 years, respectively.
Table 3. Association of animal prevalence of bovine TB with risk factors based on univariable binary logistic regression analysis.
The results of the multivariable binary logistic regression analysis are presented in Table 4. According to the multivariable binary logistic regression analysis, the male animals were 1.77 (95% CI: 1.02–3.05) times more likely to be TB positive compared to the female animals. Similarly, the cattle kept in Dasenech and Benetsemi were 10.65 (95% CI: 2.47–45.87) and 22.94 (95% CI: 5.48–95.94) times more likely to be TB positive than the cattle kept in Selamago, respectively.
Table 4. Multivariate logistic regression analysis of tuberculin reactors with various host-related risk factors at 4 mm cut-off point.
A total of 1,200 human presumptive TB cases (1,166 pulmonary TB and 34 extrapulmonary TB cases) were identified by healthcare providers and then included in this study. Smear positivity was observed in 21.3% (255/1200) of the presumptive cases. Acid-fast bacilli were confirmed in all of the 34 extrapulmonary TB cases and 221 pulmonary TB cases. The overall prevalence of culture positivity was 13.4% (161/1200). Culture positivity was 76.5% (26/34) in the FNA samples, while it was 11.6% in the pulmonary TB cases (135/1166). The isolation rate of M. bovis was 1.2% (two out of 161 isolates). The two M. bovis isolates were from the pulmonary TB cases.
Spoligotyping was performed on 156 of the 161 culture-positive DNA samples (Table 5), identifying 66 different patterns (24 clustered and 42 single strains). The majority of the isolates (76.3%) had shared international types (SITs), while the remaining 23.7% were orphan strains. The most common major lineages identified were Euro-American (EA), East African-Indian (EAI), and Indo-Oceanic (IO), with proportions of 67.3% (105/156), 22.4% (35/156), and 6.4% (10/156), respectively. Lineage 7, M. bovis, and M. africanum were isolated, with proportions of 1.9% (3/156), 1.3% (2/156), and 0.6% (1/156), respectively.
Table 5. Spoligotype patterns of M. bacterium tuberculosis complex species isolated from human in the South Omo zone of southern Ethiopia.
The present study was conducted in the remote South Omo Zone pastoral districts of southern Ethiopia on mycobacterial infections in cattle and pastoralists. Herd and animal prevalences of bovine TB were estimated, and lineages of MTBC members were identified from pastoralists. Two M. bovis were isolated from the sputum samples of the human TB cases.
Bovine TB is endemic in Ethiopia (35), and over the last two decades, a significant number of studies have been conducted, primarily in the central zones of the country, while only a few studies have been conducted in the pastoral zones (36). In this context, the present study is considered an addition to the limited studies conducted on humans and animals in the pastoral zones. The animal prevalence recorded in this study was low in general; however, it was higher than the animal prevalence values recorded in other pastoral zones of Ethiopia (9, 24, 37). Furthermore, it was lower than the values reported by other researchers in different pastoral areas of Ethiopia (21, 38–40). The difference in the prevalence of bovine TB across different pastoral zones could be attributed to factors such as herd size, the availability of adequate grazing pasture, the extent of co-grazing among different herds, and the magnitude of interaction with wildlife. These factors also affect the herd prevalence of bovine TB. The observed herd prevalence was similar to the herd prevalence reported earlier by other researchers in different pastoral zones of Ethiopia (24, 41). However, it was lower than the herd prevalence values reported in other studies (21, 38, 42–45). In contrast, some other studies reported herd prevalence values lower than those recorded in this study (46, 47).
The animal prevalence was associated with age, sex, body condition, and the districts in which the cattle were kept. Similar observations were previously reported in Ethiopia (21, 38, 39, 48, 49), Ecuador (50), and India (51). Consistent with the findings of this study, other studies also reported an association between animal prevalence and the body condition of cattle (44, 49, 52). The animal prevalence of bovine TB increased with the age of the cattle, as indicated by the multinomial binomial logistic regression analysis. This observation was also previously reported by other researchers (15, 53–58). The probable reason for this is that older animals have had repeated opportunities for exposure to mycobacterial infections throughout their lifetimes. In addition, age-related immune suppression makes older animals more susceptible to mycobacterial infections (59). The results of this study showed that the animals with moderate body condition were at a higher risk of having BTB than the animals with poor body condition. It is known that the SICCTT is influenced by the immune status of animals. Animals with poor body condition are prone to false-negative results because of their weak immune responses to the tuberculin injection. The weak immune response to the tuberculin injection could be due to malnutrition, the advanced stage of TB causing a shift in the immune response from Th1 to Th2, or parasite infestation, which can also lead to a shift in the immune response from Th1 to Th2. In addition, the results of this study showed that the male animals were at a higher risk of being reactors to the SICCTT compared to the female animals. This difference in reactivity between male and female animals could be due to their physiological differences, which could affect their immune responses to the tuberculin injection. Female animals could be either pregnant or lactating, both of which can compromise their responses to the tuberculin injection.
The low prevalence of bovine TB could also be due to the low sensitivity of the SICCTT. Furthermore, exposure to environmental mycobacteria, infection with Mycobacterium avium subspecies paratuberculosis (MAP), infestation with intestinal parasites, and malnutrition could further lower the sensitivity of the SICCTT, potentially resulting in a high rate of false-negative animals. The high rate of false-negative cases could also contribute to the low prevalence. Therefore, the SICCTT should be supplemented by other tests, such as the interferon gamma test and serological tests, to increase the detection rate. Particularly, parallel testing of study animals with the SICCTT and a rapid serological test allows for the detection of animals both in the early and advanced stages of bovine TB, thereby increasing detection sensitivity.
Differences were observed in the prevalence of bovine TB among the five study districts. The prevalence was relatively higher in the Dasenech and Benetsemi districts. This difference could be due to factors such as cattle population density, the degree of interaction between cattle from different herds and wild animals, the prior introduction of infected cattle to the district, and the nutritional and health status of cattle, as well as environmental and climatic factors (53).
The proportion of culture positivity in the sputum samples was very low. Other researchers have reported relatively higher proportions of culture positivity in Ethiopia (60, 61), although one study reported a similarly low proportion of sputum culture positivity (62). The low proportion of sputum culture positivity was mainly associated with issues in sample handling. Due to the harsh environment and remote location, the samples could not be maintained at the optimal temperature during collection. The cold chain was used, but it was not easy to replace the ice packs. In addition, the sputum samples were stored for approximately 1 month at the field sites before being transported to the laboratory for culturing. These sample handling conditions could kill the bacilli, and since culture positivity is directly related to the availability of bacilli in the sputum, such poor sample handling procedures could lead to low sputum culture positivity.
Based on spoligotyping, six lineages were isolated from the pastoralists—Euro-American, EAI, Indo-Oceanic, lineage 7, M. bovis, and M. africanum. The dominant lineage, the Euro-American lineage, constituted 67.3% of the total isolates. Similar observations have been reported in earlier studies (63–66). This highlights the rapid spread of the Euro-American lineage from central Ethiopia to the peripheral areas of the country. The second, third, fourth, fifth, and sixth lineages, isolated in decreasing order of frequency, included EAI, Indo-Oceanic lineage, lineage 7, M. bovis, and M. africanum, respectively. Other studies have also isolated these lineages with varying frequencies across different regions of Ethiopia (63–65, 67). M. africanum has been reported as a significant cause of human TB in West African countries (68–70).
Two M. bovis isolates were obtained from the pastoralists. Although the proportion of the M. bovis isolates was low, it highlights the significance of zoonotic TB in the South Omo Zone. Animals play a significant role in the livelihoods of pastoralists. In addition, animals are central to various social events for pastoralists. For example, in the South Omo Zone, a young Hammer male is expected to participate in bull jumping before marriage (Figure 3). The bull jumping ceremony is performed by every young Hammer boy before marriage. During this event, people from different villages gather to celebrate the ceremony. Cattle are also gathered together in the same place as the people and are used for jumping, as demonstrated in Figure 3. This gathering of cattle and humans during such and other social events is expected to facilitate the transmission of MTBC species between humans and cattle. In addition to physical contact between cattle and pastoralists, pastoralists consume raw milk and undercooked meat, which promote the transmission of M. bovis from animals to humans.
In agreement with the present study, other studies have also reported the isolation of M. bovis from humans in Ethiopia (16, 24, 46). These studies have reported a low percentage of M. bovis isolates among humans in Ethiopia. Therefore, the results of this study and previous studies suggest the minimal role of M. bovis in causing human TB in Ethiopia. This minimal role could be due to the fact that over 95% of the cattle in Ethiopia are of the Zebu breed, which is kept extensively on pasture. The prevalence of bovine TB is low in the Zebu breed and in cattle grazing on pasture. Therefore, since the prevalence of bovine TB is low in the Zebu breed, the rate of its transmission to humans is also low (46).
Based on spoligotyping, the clustering rate was 57.7%%, which is similar to the report of a previous study conducted in Gambella, southwest Ethiopia (71). However, the clustering rate reported in this study was lower than the rate reported by the national survey (72) and studies from Addis Ababa (73), northwest Ethiopia (74), eastern Ethiopia (75), and central Ethiopia (67). On the other hand, the clustering rate reported in this study was slightly higher than that reported from western Ethiopia (76). The difference in the clustering rate among different studies could be due to variations in population density and the interaction of people through socio-economic activities (77). In general, the clustering rate recorded in this study can be considered low, which could be due to the low density of the population in the South Omo Zone. The study districts are sparsely populated, and the interaction among the population is limited.
Nonetheless, it is important to highlight the poor discriminatory power of spoligotyping in genotyping MTBC. This limitation of spoligotyping has been discussed in previous publications (78, 79). In addition, several authors have evaluated the discriminatory power of spoligotyping, and based on the results of their studies, concluded that spoligotyping demonstrates low discriminatory power in genotyping MTBC species compared to the other genotyping tools (78, 80, 81). For this reason, Comas et al. (78) recommended combining spoligotyping with mycobacterial interspersed repetitive unit (MIRU)-based variable number tandem repeats (VNTRs) typing for the initial exploratory screening of strains.
One of the objectives of the study was to investigate the zoonotic transmission of tuberculosis between cattle and pastoralists. However, the isolation and identification of mycobacteria could not be done directly from the pastoralists and their cattle. The cattle and human studies were performed separately in the same geographic districts. The cattle study involved testing animals in the field, while the human study was conducted at a hospital and health centers on presumptive human TB cases who visited these healthcare institutions seeking treatment. Therefore, the study could not show direct human–animal transmission. On the other hand, since these human cases were residents of the area where the study cattle were raised, it was assumed that the isolation of M. bovis from these human cases might suggest the transmission of M. bovis from cattle to humans.
The present study revealed a low prevalence of bovine TB in the remote pastoral setting of southern Ethiopia. In addition, the isolation rate of M. bovis from human TB cases in the same setting was low. The low prevalence of bovine TB could be due to the low sensitivity of the SICCTT, exposure of the study animals to environmental mycobacteria, infection with MAP, infestation with intestinal parasites, and malnutrition which could affect the sensitivity of SICCTT, leading to a high rate of false-negative animals. Therefore, to improve the detection of bovine TB, it is recommended to use the SICCTT in parallel with a rapid serological test. The role of M. bovis in human TB cases should be investigated, particularly among pastoralists who have direct contact with infected animals and those who consume raw milk from infected animals.
The raw data supporting the conclusions of this article will be made available by the authors, without undue reservation.
The studies involving humans were approved by Aklilu Lemma Institute of Pathobiology (ALIPB), Addis Ababa University (Ethiopia), Institutional Review Board (ALIPB/IRB/22-B/2012/13) and the National Research Ethics Committee (Ref No: 3.10/785/07). The studies were conducted in accordance with the local legislation and institutional requirements. The participants provided their written informed consent to participate in this study. The animal studies were approved by Aklilu Lemma Institute of Pathobiology (ALIPB), Addis Ababa University (Ethiopia), Institutional Review Board (ALIPB/IRB/22-B/2012/13) and the National Research Ethics Committee (Ref No: 3.10/785/07). The studies were conducted in accordance with the local legislation and institutional requirements. Written informed consent was obtained from the owners for the participation of their animals in this study. Written informed consent was obtained from the individual(s) for the publication of any identifiable images or data included in this article.
TM: Investigation, Methodology, Writing – original draft. FD: Data curation, Investigation, Methodology, Writing – review & editing. BW: Investigation, Methodology, Writing – review & editing. AZ: Investigation, Writing – review & editing. GM: Investigation, Supervision, Writing – review & editing. HK: Data curation, Formal analysis, Software, Writing – review & editing. BB: Data curation, Formal analysis, Software, Writing – review & editing. GA: Conceptualization, Funding acquisition, Investigation, Project administration, Resources, Supervision, Validation, Visualization, Writing – review & editing.
The author(s) declare that financial support was received for the research and/or publication of this article. The research was funded by the National Institutes of Health (NIH), USA with grant number U01HG007472-01, the Addis Ababa University, Funding for publication of the manuscript was obtained by the United Arab Emirates University, Ethiopia. The funders had no role in the study design, data collection, analysis and interpretation, and writing of the manuscript.
The authors would like to thank the study participants, the owners of the animals, the South Omo Zone communities, and the staff members of the South Omo Zone Health Offices, Jinka Regional Laboratory, Jinka General Hospital, health centers, and health posts for their invaluable support during field sample collection.
The authors declare that the research was conducted in the absence of any commercial or financial relationships that could be construed as a potential conflict of interest.
The author(s) declare that no Gen AI was used in the creation of this manuscript.
All claims expressed in this article are solely those of the authors and do not necessarily represent those of their affiliated organizations, or those of the publisher, the editors and the reviewers. Any product that may be evaluated in this article, or claim that may be made by its manufacturer, is not guaranteed or endorsed by the publisher.
1. Smith, RMM, Drobniewski, F, Gibson, A, Montague, JDE, Logan, MN, Hunt, D, et al. Mycobacterium bovis infection, United Kingdom. Emerg Infect Dis. (2004) 10:539–41. doi: 10.3201/eid1003.020819
2. Boland, F, Kelly, GE, Good, M, and More, SJ. Bovine tuberculosis and milk production in infected dairy herds in Ireland. Prev Vet Med. (2010) 93:153–61. doi: 10.1016/j.prevetmed.2009.09.021
3. O’Reilly, LM, and Daborn, CJ. The epidemiology of Mycobacterium bovis infections in animals and man: a review. Tuber Lung Dis. (1995) 76:1–46. doi: 10.1016/0962-8479(95)90591-x
4. Cosivi, O, Meslin, FX, Daborn, CJ, and Grange, JM. Epidemiology of Mycobacterium bovis infection in animals and humans, with particular reference to Africa. Rev Sci Tech. (1995) 14:733–46. doi: 10.20506/rst.14.3.875
5. Shitaye, JE, Tsegaye, W, and Pavlik, I. Bovine tuberculosis infection in animal and human populations in Ethiopia: a review. Vet Med. (2007) 52:317–32. doi: 10.17221/1872-VETMED
6. Jebara, KB, Cáceres, P, Berlingieri, F, and Weber-Vintzel, L. Ten years’ work on the world organisation for animal health (OIE) worldwide animal disease notification system. Prev Vet Med. (2012) 107:149–59. doi: 10.1016/j.prevetmed.2012.08.008
7. De Garine-Wichatitsky, M, Caron, A, Kock, R, Tschopp, R, Munyeme, M, Hofmeyr, M, et al. A review of bovine tuberculosis at the wildlife-livestock-human interface in sub-Saharan Africa. Epidemiol Infect. (2013) 141:1342–56. doi: 10.1017/S0950268813000708
8. Ayele, W, Neill, S, Zinsstag, J, Weiss, M, and Pavlik, I. Bovine tuberculosis: an old disease but a new threat to Africa. Int J Tuberc Lung Dis Off. (2004) 8:924–37.
9. Tschopp, R, Aseffa, A, Schelling, E, Berg, S, Hailu, E, Gadisa, E, et al. Bovine tuberculosis at the wildlife-livestock-human interface in Hamer Woreda, south Omo, Southern Ethiopia. PLoS One. (2010) 5:e12205. doi: 10.1371/journal.pone.0012205
10. Tsegaye, W, Aseffa, A, Mache, A, Mengistu, Y, Berg, S, and Ameni, G. Conventional and molecular epidemiology of Bovine tuberculosis in dairy farms in Addis Ababa City, the Capital of Ethiopia. Int J Appl Res Vet Med. (2010) 8:143
11. Oloya, J, Opuda-Asibo, J, Kazwala, R, Demelash, AB, Skjerve, E, Lund, A, et al. Mycobacteria causing human cervical lymphadenitis in pastoral communities in the Karamoja region of Uganda. Epidemiol Inf. (2008) 136:636–43. doi: 10.1017/S0950268807009004
12. Hlavsa, MC, Moonan, PK, Cowan, LS, Navin, TR, Kammerer, JS, Morlock, GP, et al. Human tuberculosis due to Mycobacterium bovis in the United States, 1995-2005. Clin Infect Dis. (2008) 47:168–75. doi: 10.1086/589240
13. LoBue, PA, and Moser, KS. Treatment of Mycobacterium bovis infected tuberculosis patients: San Diego County, California, United States, 1994-2003. Int J Tuberc Lung Dis. (2005) 9:333–8.
14. Rodwell, TC, Moore, M, Moser, KS, Brodine, SK, and Strathdee, SA. Tuberculosis from Mycobacterium bovis in binational communities. United States Emerg Infect Dis. (2008) 14:909–16. doi: 10.3201/eid1406.071485
15. Ameni, G, Aseffa, A, Engers, H, Young, D, Gordon, S, Hewinson, G, et al. High prevalence and increased severity of pathology of Bovine tuberculosis in Holsteins compared to zebu breeds under field cattle husbandry in Central Ethiopia. Clin Vaccine Immunol. (2007) 14:1356–61. doi: 10.1128/CVI.00205-07
16. Firdessa, R, Berg, S, Hailu, E, Schelling, E, Gumi, B, Erenso, G, et al. Mycobacterial lineages causing pulmonary and extra pulmonary tuberculosis, Ethiopia. Emerg Infect Dis. (2013) 19:460–3. doi: 10.3201/eid1903.120256
17. Cleaveland, S, Shaw, DJ, Mfinanga, SG, Shirima, G, Kazwala, RR, Eblate, E, et al. Mycobacterium bovis in rural Tanzania: risk factors for infection in human and cattle populations. Tuberculosis. (2007) 87:30–43. doi: 10.1016/j.tube.2006.03.001
18. Humblet, M-F, Boschiroli, ML, and Saegerman, C. Classification of worldwide bovine tuberculosis risk factors in cattle: a stratified approach. Vet Res. (2009) 40:50. doi: 10.1051/vetres/2009033
19. Mfinanga, SGM, Morkve, O, Kazwala, RR, Cleaveland, S, Sharp, MJ, Kunda, J, et al. Mycobacterial adenitis: role of Mycobacterium bovis, non-tuberculous mycobacteria, HIV infection, and risk factors in Arusha, Tanzania. East Afr Med J. (2004) 81:171–8. doi: 10.4314/eamj.v81i4.9150
20. Kassa, GM. (2014). Molecular epidemiology and transmission patterns of Mycobacterium tuberculosis complex in Afar pastoral communities and their livestock in Ethiopia. Available online at: https://www.duo.uio.no/handle/10852/41436 (accessed September 3, 2024).
21. Gumi, B, Schelling, E, Firdessa, R, Aseffa, A, Tschopp, R, Yamuah, L, et al. Prevalence of bovine tuberculosis in pastoral cattle herds in the Oromia region, southern Ethiopia. Trop Anim Health Prod. (2011) 43:1081–7. doi: 10.1007/s11250-010-9777-x
22. Demelash, B, Inangolet, F, Oloya, J, Asseged, B, Badaso, M, Yilkal, A, et al. Prevalence of bovine tuberculosis in Ethiopian slaughter cattle based on post-mortem examination. Trop Anim Health Prod. (2009) 41:755–65. doi: 10.1007/s11250-008-9248-9
23. Biffa, D, Skjerve, E, Oloya, J, Bogale, A, Abebe, F, Dahle, U, et al. Molecular characterization of Mycobacterium bovis isolates from Ethiopian cattle. BMC Vet Res. (2010) 6:28. doi: 10.1186/1746-6148-6-28
24. Gumi, B, Schelling, E, Berg, S, Firdessa, R, Erenso, G, Mekonnen, W, et al. Zoonotic transmission of tuberculosis between pastoralists and their livestock in south-East Ethiopia. Eco Health. (2012) 9:139–49. doi: 10.1007/s10393-012-0754-x
25. SNNPRS. South Omo zone finance and economic development coordination Main department. Population projection at Kebele level, Jinka. Hawassa: SNNPRS (2007).
26. Bennett, S, Woods, T, Liyanage, WM, and Smith, DL. A simplified general method for cluster-sample surveys of health in developing countries. World Health Stat Q. (1991) 44:98–106.
27. Enyew, B. (2012). Climate Change Impact and Adaptation in South Omo Zone, Ethiopia. North Carolina Agricultural and Technical State University, MSc thesis. doi: 10.13140/2.1.2355.0246
28. De-Lahunta, A, and Habel, RE. (2024). Teeth. Applied veterinary anatomy by Alexander Delahunta | open library. Available online at: https://openlibrary.org/books/OL7862801M/Applied_Veterinary_Anatomy (accessed January 21, 2024).
29. Nicholson, MJ, and Butterworth, MH. (1986). A guide to condition scoring of zebu cattle. Available online at: https://www.semanticscholar.org/paper/A-guide-to-condition-scoring-of-zebu-cattle-Nicholson-Butterworth/b3f13681e798f3ab4d8ae01b0ea1c92ba968f01f (accessed January 21, 2024).
30. WOAH Bovine tuberculosis OIE manual of diagnostic tests and vaccines for terrestrial animals (mammals, birds and bees). Office international des epizootics (OIE).WOAH Paris. 683–617. (2008)
32. Brudey, K, Driscoll, JR, Rigouts, L, Prodinger, WM, Gori, A, Al-Hajoj, SA, et al. Mycobacterium tuberculosis complex genetic diversity: mining the fourth international spoligotyping database (SpolDB4) for classification, population genetics and epidemiology. BMC Microbiol. (2006) 6:23. doi: 10.1186/1471-2180-6-23
33. Kamerbeek, J, Schouls, L, Kolk, A, van Agterveld, M, van Soolingen, D, Kuijper, S, et al. Simultaneous detection and strain differentiation of Mycobacterium tuberculosis for diagnosis and epidemiology. J Clin Microbiol. (1997) 35:907–14. doi: 10.1128/jcm.35.4.907-914.1997
34. Couvin, D, David, A, Zozio, T, and Rastogi, N. Macro-geographical specificities of the prevailing tuberculosis epidemic as seen through SITVIT2, an updated version of the Mycobacterium tuberculosis genotyping database. Infect Genet Evol. (2019) 72:31–43. doi: 10.1016/j.meegid.2018.12.030
35. Hailemariam. A brief analysis of activities of meat inspection and quarantine division. Addis Ababa: Department of Veterinary Service, MoA (1975).
36. PFE-IIRR DF. Pastoralism and land: Land tenure, administration and use in pastoral areas of Ethiopia. Nairobi: PFE-IIRR DF (2010).
37. Kanchora, G, Jiso, R, Tsegaye, M, and Bisrat, A. Epidemiological study on Bovine tuberculosis in Boran breeds cattle at Yabello District, southern, Ethiopia. BIO. (2020) 8:105. doi: 10.11648/j.bio.20200806.12
38. Mamo, G, Abebe, F, Worku, Y, Hussein, N, Legesse, M, Getachew, T, et al. Bovine tuberculosis and its associated risk factors in pastoral and agro-pastoral cattle herds of Afar region, Northeast Ethiopia. J Vet Med Anim Health. (2013) 5:171–9. doi: 10.5897/JVMAH2013.0204
39. Kemal, J, Sibhat, B, Abraham, A, Terefe, Y, Tulu, KT, Welay, K, et al. Bovine tuberculosis in eastern Ethiopia: prevalence, risk factors and its public health importance. BMC Infect Dis. (2019) 19:39. doi: 10.1186/s12879-018-3628-1
40. Hussein, HA, Ahmed, JM, Musse, AH, and Gizaw, Y. Prevalence and risk factors of bovine tuberculosis in cattle in selected districts of Fafan pastoral settings, eastern Ethiopia. Heliyon. (2024) 10:e24998. doi: 10.1016/j.heliyon.2024.e24998
41. Romha, G, Egziabher, GG, and Ameni, G. Assessment of Bovine tuberculosis and its risk factors in cattle and humans, at and around Dilla town, southern Ethiopia. Anim Vet Sci. (2014) 2:94–100. doi: 10.11648/j.avs.20140204.12
42. Tigre, A, and Abatu, D. (2011). Preliminary study on public health implication of Bovine tuberculosis in Jimma town, south West Ethiopia. Available online at: https://www.researchgate.net/publication/269391896_Tigre_W_Alemayehu_G_Abetu_T_and_Deressa_B_2011_Preliminary_study_on_public_health_implication_of_Bovine_tuberculosis_in_Jimma_town_South_west_Ethiopia_Global_Vet_6_4 (accessed September 6, 2024).
43. Firdessa, R, Tschopp, R, Wubete, A, Sombo, M, Hailu, E, Erenso, G, et al. High prevalence of bovine tuberculosis in dairy cattle in Central Ethiopia: implications for the dairy industry and public health. PLoS One. (2012) 7:e52851. doi: 10.1371/journal.pone.0052851
44. Fikre, Z, Gebremedhin, R, Gebretsadik, B, Gezahegne, M, Tesfaye, S, and Gobena, A. Prevalence of bovine tuberculosis and assessment of cattle owners awareness on its public health implication in and around Mekelle, northern Ethiopia. J Vet Med Anim Health. (2014) 6:159–67. doi: 10.5897/JVMAH2014.0288
45. Ameni, G, Wudie, A, Ameni, G, and Wudie, A. Preliminary study on bovine tuberculosis in Nazareth municipality abattoir of Central Ethiopia. Bull Anim Health Prod Afr. (2003) 51:125–32.
46. Nuru, A, Mamo, G, Teshome, L, Zewdie, A, Medhin, G, Pieper, R, et al. Bovine tuberculosis and its risk factors among dairy cattle herds in and around Bahir Dar City, Northwest Ethiopia. Ethiop Vet J. (2015) 19:27. doi: 10.4314/evj.v19i2.3
47. Tamiru, F, HaileMariam, M, and Terfa, W. (2013). Preliminary study on prevalence of bovine tuberculosis in cattle owned by tuberculosis positive and negative farmers and assessment of zoonotic awareness in ambo and toke Kutaye districts, Ethiopia. Available online at: https://www.semanticscholar.org/paper/Preliminary-study-on-prevalence-of-bovine-in-cattle-Tamiru-HaileMariam/ba41e9fe99d09cf7bd570b437023b8a0269fd957 (accessed September 6, 2024).
48. Ameni, G, and Erkihun, A. Bovine tuberculosis on small-scale dairy farms in Adama town, Central Ethiopia, and farmer awareness of the disease. Rev Sci Tech. (2007) 26:711–9. doi: 10.20506/rst.26.3.1778
49. Elias, K, Hussein, D, Asseged, B, Wondwossen, T, and Gebeyehu, M. Status of bovine tuberculosis in Addis Ababa dairy farms. Rev Sci Tech. (2008) 27:915–23. doi: 10.20506/rst.27.3.1850
50. Proaño-Perez, F, Benitez-Ortiz, W, Celi-Erazo, M, Ron-Garrido, L, Benitez-Capistros, R, Portaels, F, et al. Comparative intradermal tuberculin test in dairy cattle in the north of Ecuador and risk factors associated with bovine tuberculosis. Am J Trop Med Hyg. (2009) 81:1103–9. doi: 10.4269/ajtmh.2009.09-0182
51. Thakur, A, Sharma, M, Katoch, V, Dhar, P, and Katoch, R. A study on the prevalence of Bovine tuberculosis in farmed dairy cattle in Himachal Pradesh. Vet World. (2010) 3:408. doi: 10.5455/vetworld.2010.408-413
52. Admasu, P, Berihun, W, and Alemu, A. Prevalence of Bovine tuberculosis in dairy cattle of Yeki District, Southern Ethiopia. Afr J Basic Appl Sci. (2014) 6:135–40. doi: 10.5829/idosi.ajbas.2014.6.5.86219
53. Kazwala, RR, Daborn, CJ, Sharp, JM, Kambarage, DM, Jiwa, SF, and Mbembati, NA. Isolation of Mycobacterium bovis from human cases of cervical adenitis in Tanzania: a cause for concern? Int J Tuberc Lung Dis. (2001) 5:87–91.
54. Oloya, J, Opuda-Asibo, J, Djønne, B, Muma, JB, Matope, G, Kazwala, R, et al. Responses to tuberculin among zebu cattle in the transhumance regions of Karamoja and Nakasongola district of Uganda. Trop Anim Health Prod. (2006) 38:275–83. doi: 10.1007/s11250-006-4322-7
55. Inangolet, FO, Demelash, B, Oloya, J, Opuda-Asibo, J, and Skjerve, E. A cross-sectional study of bovine tuberculosis in the transhumant and agro-pastoral cattle herds in the border areas of Katakwi and Moroto districts, Uganda. Trop Anim Health Prod. (2008) 40:501–8. doi: 10.1007/s11250-007-9126-x
56. Regassa, A, Tassew, A, Amenu, K, Megersa, B, Abunna, F, Mekibib, B, et al. A cross-sectional study on bovine tuberculosis in Hawassa town and its surroundings, southern Ethiopia. Trop Anim Health Prod. (2010) 42:915–20. doi: 10.1007/s11250-009-9507-4
57. Cadmus, SIB, Agada, CA, Onoja, II, and Salisu, I. Risk factors associated with bovine tuberculosis in some selected herds in Nigeria. Trop Anim Health Prod. (2010) 42:547–9. doi: 10.1007/s11250-009-9463-z
58. Biffa, D, Inangolet, F, Bogale, A, Oloya, J, Djønne, B, and Skjerve, E. Risk factors associated with prevalence of tuberculosis-like lesions and associated mycobacteria in cattle slaughtered at public and export abattoirs in Ethiopia. Trop Anim Health Prod. (2011) 43:529–38. doi: 10.1007/s11250-010-9729-5
59. Perez, AM, Ward, MP, Charmandarián, A, and Ritacco, V. Simulation model of within-herd transmission of bovine tuberculosis in argentine dairy herds. Prev Vet Med. (2002) 54:361–72. doi: 10.1016/s0167-5877(02)00043-0
60. Tilahun, M, Ameni, G, Desta, K, Zewude, A, Yamuah, L, Abebe, M, et al. Molecular epidemiology and drug sensitivity pattern of Mycobacterium tuberculosis strains isolated from pulmonary tuberculosis patients in and around ambo town, Central Ethiopia. PLoS One. (2018) 13:e0193083. doi: 10.1371/journal.pone.0193083
61. Bruchfeld, J, Aderaye, G, Palme, IB, Bjorvatn, B, Ghebremichael, S, Hoffner, S, et al. Molecular epidemiology and drug resistance of Mycobacterium tuberculosis isolates from Ethiopian pulmonary tuberculosis patients with and without human immunodeficiency virus infection. J Clin Microbiol. (2002) 40:1636–43. doi: 10.1128/JCM.40.5.1636-1643.2002
62. Desta, K, Asrat, D, Lemma, E, Gebeyehu, M, and Feleke, B. Prevalence of smear negative pulmonary tuberculosis among patients visiting St. Peter’s tuberculosis specialized hospital, Addis Ababa, Ethiopia. Ethiop Med J. (2009) 47:17–24.
63. Diriba, B, Berkessa, T, Mamo, G, Tedla, Y, and Ameni, G. Spoligotyping of multidrug-resistant Mycobacterium tuberculosis isolates in Ethiopia. Int J Tuberc Lung Dis. (2013) 17:246–50. doi: 10.5588/ijtld.12.0195
64. Garedew, L, Mihret, A, Mamo, G, Abebe, T, Firdessa, R, Bekele, Y, et al. Strain diversity of mycobacteria isolated from pulmonary tuberculosis patients at Debre Birhan hospital, Ethiopia. Int J Tuberc Lung Dis. (2013) 17:1076–81. doi: 10.5588/ijtld.12.0854
65. Debebe, T, Admassu, A, Mamo, G, and Ameni, G. Molecular characterization of Mycobacterium tuberculosis isolated from pulmonary tuberculosis patients in Felege Hiwot referral hospital, Northwest Ethiopia. J Microbiol Immunol Infect. (2014) 47:333–8. doi: 10.1016/j.jmii.2013.03.012
66. Reed, MB, Pichler, VK, McIntosh, F, Mattia, A, Fallow, A, Masala, S, et al. Major Mycobacterium tuberculosis lineages associate with patient country of origin. J Clin Microbiol. (2009) 47:1119–28. doi: 10.1128/JCM.02142-08
67. Bedewi, Z, Worku, A, Mekonnen, Y, Yimer, G, Medhin, G, Mamo, G, et al. Molecular typing of Mycobacterium tuberculosis complex isolated from pulmonary tuberculosis patients in Central Ethiopia. BMC Infect Dis. (2017) 17:184. doi: 10.1186/s12879-017-2267-2
68. Källenius, G, Koivula, T, Ghebremichael, S, Hoffner, SE, Norberg, R, Svensson, E, et al. Evolution and clonal traits of Mycobacterium tuberculosis complex in Guinea-Bissau. J Clin Microbiol. (1999) 37:3872–8. doi: 10.1128/jcm.37.12.3872-3878.1999
69. de Jong, BC, Hill, PC, Brookes, RH, Gagneux, S, Jeffries, DJ, Otu, JK, et al. Mycobacterium africanum elicits an attenuated T cell response to early secreted antigenic target, 6 kDa, in patients with tuberculosis and their household contacts. J Infect Dis. (2006) 193:1279–86. doi: 10.1086/502977
70. Viana-Niero, C, Gutierrez, C, Sola, C, Filliol, I, Boulahbal, F, Vincent, V, et al. Genetic diversity of Mycobacterium africanum clinical isolates based on IS6110-restriction fragment length polymorphism analysis, spoligotyping, and variable number of tandem DNA repeats. J Clin Microbiol. (2001) 39:57–65. doi: 10.1128/JCM.39.1.57-65.2001
71. Asebe, G, Adane, W, Aboma, Z, Tafess, K, and Ameni, G. Molecular characterization of Mycobacterium tuberculosis complex in Gambella region, Southwest Ethiopia. Afr J Microbiol Res. (2016) 10:1086–93. doi: 10.5897/AJMR2015.7415
72. Getahun, M, Ameni, G, Kebede, A, Yaregal, Z, Hailu, E, Medihn, G, et al. Molecular typing and drug sensitivity testing of Mycobacterium tuberculosis isolated by a community-based survey in Ethiopia. BMC Public Health. (2015) 15:751. doi: 10.1186/s12889-015-2105-7
73. Mihret, A, Bekele, Y, Loxton, AG, Jordan, AM, Yamuah, L, Aseffa, A, et al. Diversity of Mycobacterium tuberculosis isolates from new pulmonary tuberculosis cases in Addis Ababa, Ethiopia. Tuberculosis Res Treatment. (2012) 2012:1–7. doi: 10.1155/2012/892079
74. Tessema, B, Beer, J, Merker, M, Emmrich, F, Sack, U, Rodloff, AC, et al. Molecular epidemiology and transmission dynamics of Mycobacterium tuberculosis in Northwest Ethiopia: new phylogenetic lineages found in Northwest Ethiopia. BMC Infect Dis. (2013) 13:131. doi: 10.1186/1471-2334-13-131
75. Belay, M, Ameni, G, Bjune, G, Couvin, D, Rastogi, N, and Abebe, F. Strain diversity of Mycobacterium tuberculosis isolates from pulmonary tuberculosis patients in Afar pastoral region of Ethiopia. Biomed Res Int. (2014) 2014:238532. doi: 10.1155/2014/238532
76. Disassa, H, Tafess, K, Worku, A, and Ameni, G. A preliminary study on molecular characterization of Mycobacterium tuberculosis in Benishangul Gumuz region, Western Ethiopia. BMRJ. (2015) 10:1–10. doi: 10.9734/BMRJ/2015/20032
77. Pareek, M, Evans, J, Innes, J, Smith, G, Hingley-Wilson, S, Lougheed, KE, et al. Ethnicity and mycobacterial lineage as determinants of tuberculosis disease phenotype. Thorax. (2013) 68:221–9. doi: 10.1136/thoraxjnl-2012-201824
78. Comas, I, Homolka, S, Niemann, S, and Gagneux, S. Genotyping of genetically monomorphic bacteria: DNA sequencing in Mycobacterium tuberculosis highlights the limitations of current methodologies. PLoS One. (2009) 4:e7815. doi: 10.1371/journal.pone.0007815
79. Hussien, B, Zewude, A, Wondale, B, Hailu, A, and Ameni, G. Spoligotyping of clinical isolates of Mycobacterium tuberculosis complex species in the Oromia region of Ethiopia. Front Public Health. (2022) 10:808626. doi: 10.3389/fpubh.2022.808626
80. Filliol, I, Motiwala, AS, Cavatore, M, Qi, W, Hazbón, MH, Bobadilla del Valle, M, et al. Global phylogeny of Mycobacterium tuberculosis based on single nucleotide polymorphism (SNP) analysis: insights into tuberculosis evolution, phylogenetic accuracy of other DNA fingerprinting systems, and recommendations for a minimal standard SNP set. J Bacteriol. (2006) 188:759–72. doi: 10.1128/JB.188.2.759-772.2006
Keywords: Mycobacterium tuberculosis complex, mycobacterial lineage, pastoralists, prevalence, risk factor, southern Ethiopia
Citation: Mohammed T, Desta F, Wondale B, Zewude A, Mamo G, Khalifa HO, Bayissa B and Ameni G (2025) Epidemiology of Mycobacterium tuberculosis complex infections in cattle and humans in the remote pastoral settings of southern Ethiopia. Front. Vet. Sci. 12:1551710. doi: 10.3389/fvets.2025.1551710
Received: 26 December 2024; Accepted: 17 February 2025;
Published: 19 March 2025.
Edited by:
Christopher Hamilton-West, University of Chile, ChileReviewed by:
Azlan Che-Amat, Putra Malaysia University, MalaysiaCopyright © 2025 Mohammed, Desta, Wondale, Zewude, Mamo, Khalifa, Bayissa and Ameni. This is an open-access article distributed under the terms of the Creative Commons Attribution License (CC BY). The use, distribution or reproduction in other forums is permitted, provided the original author(s) and the copyright owner(s) are credited and that the original publication in this journal is cited, in accordance with accepted academic practice. No use, distribution or reproduction is permitted which does not comply with these terms.
*Correspondence: Gobena Ameni, Z29iZW5hLmFtZW5pQHVhZXUuYWMuYWU=
Disclaimer: All claims expressed in this article are solely those of the authors and do not necessarily represent those of their affiliated organizations, or those of the publisher, the editors and the reviewers. Any product that may be evaluated in this article or claim that may be made by its manufacturer is not guaranteed or endorsed by the publisher.
Research integrity at Frontiers
Learn more about the work of our research integrity team to safeguard the quality of each article we publish.