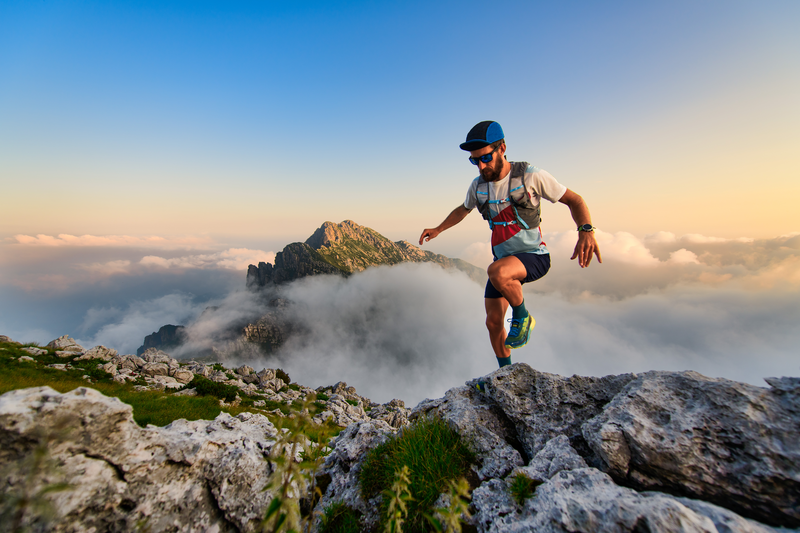
95% of researchers rate our articles as excellent or good
Learn more about the work of our research integrity team to safeguard the quality of each article we publish.
Find out more
ORIGINAL RESEARCH article
Front. Vet. Sci. , 26 February 2025
Sec. Veterinary Neurology and Neurosurgery
Volume 12 - 2025 | https://doi.org/10.3389/fvets.2025.1549205
Introduction: Volumetric studies in relation to CM/SM have not been reported in Pomeranians. In this study, we aim to (1) report the intermodality agreement between CT- and MRI-based volumetric measurements of the skull and cervical spinal canal, and (2) assess for differences and associations between the volumetric measurements and CM/SM status.
Methods: Pomeranians were included that underwent CT and MRI studies during the period of February 2022–June 2024. Frontal sinus volume (FSV), caudal cranial fossa volume (CCFV), rostral and middle cranial fossa volume (RMCFV), caudal cranial fossa parenchymal volume (CCFPV), rostral and middle cranial fossa parenchymal volume (RMCFPV), cerebellar parenchymal volume (CPV), brain stem parenchymal volume (BSPV), ventricular system volume (VSV), and spinal canal volume between C1-C7 (CSCV) were measured. CCFV to RMCFV volume index (VI) and CCFPV to CCFV (CCFPV%) were calculated. Agreement between MRI- and CT-based quantitative measurements was assessed with intraclass correlation coefficients. Inferential statistical tests including logistic regression modeling were performed to assess for associations between variables and CM/SM status.
Results: For all volumetric assessments that were performed on both CT and MRI, agreement was good or excellent. There were significant differences between SM normal and abnormal dogs for body weight as well as all volumetric parameters except for CCFPV% and RMCFV. Multiple logistic regression showed that a smaller CCFV and larger VSV were associated with SM.
Conclusion: Smaller CCFV and larger VSV are associated with the development of SM in Pomeranians and have increased odds of SM.
The pathogenesis of Chiari-like malformation (CM) and syringomyelia (SM) in dogs is incompletely understood. In the last two decades, numerous studies have evaluated clinical features, morphometric characteristics, and hereditary factors associated with these disorders (1–21). Most of these studies involved the ‘poster breed’ for CM/SM, the Cavalier King Charles Spaniel (CKCS), although other breeds including the Affenpinscher, Chihuahua, Griffon Bruxellois, and, more recently, the Pomeranian dog breed has also been included in large studies. Moreover, a great many other breeds as well as crossbreed dogs have been reported to be affected (22). Collectively, this research has contributed to improve our understanding of these disorders in dogs and humans (23).
For the Pomeranian, our research group has published studies concerning the phenotypic characteristics (15), longitudinal magnetic resonance imaging findings (24), craniocervical 2-dimensional (2D) morphometry (16, 17), and manual external skull measurements (25) of affected versus unaffected dogs. However, volumetric studies in relation to CM/SM have not been reported in this breed.
Morphometric studies in other breeds, most prominently the CKCS, have provided significant clues as to the predisposing anatomical characteristics of dogs with CM/SM. One of the most often cited findings is a decreased size or volume of the caudal cranial fossa and/or relatively increased cerebellar parenchymal volume (3–5, 26–30). Additionally, researchers have found differences between dogs with and without CM/SM for (semi-)quantitative assessments of frontal sinus volume, cranial vault volume indices, spinal canal width, and ventricular system volumes (5, 21, 27, 29, 31, 32). A recent study investigating the prevalence of CM/SM in a cohort or small-sized dog breeds revealed that SM also was found in a number of mesocephalic and dolichocephalic dogs (not necessarily associated with CM), consistent with an even more complex pathogenesis for SM (22).
Most morphometric studies in relation to canine CM/SM have been conducted by making use of MRI, some also or only including computed tomography (CT). Comparability between these modalities for volumetric measurements of the canine skull has not been assessed and most volumetric studies have been based on an original CT-based study in normal dogs (33). It will be valuable to know if measurements performed on MRI and CT images yield comparable results.
In this study, we aim to (1) report the intermodality agreement between CT- and MRI-based volumetric measurements of the skull and cervical spinal canal, and (2) assess for differences and associations between the volumetric measurements and CM/SM status.
For this study, Pomeranians were included that underwent both CT and MRI studies during the period of February 2022–June 2024. Signalment was recorded (including sex and neuter status, age, and body weight). Exclusion criteria were (1) MRI or CT scans with artifacts or inadequate image quality, preventing accurate assessment or measurement, (2) age < 12 months (considered skeletally immature), and (3) a prior history or diagnosis of central nervous system (CNS) disease other than CM/SM, ventriculomegaly, supracollicular fluid accumulation, findings related to craniocervical junction abnormalities (CJAs) [e.g., atlanto-occipital overlapping (AOO)], dorsal constriction at C1/C2 [atlantoaxial band (AAB)], atlantoaxial instability (AAI, also referred to as atlantoaxial subluxation), or non-structural disorders such as epilepsy or paroxysmal dyskinesia.
MRI and CT studies were performed under general anesthesia (individualized anesthetic protocols) with a high-field MRI scanner (1.5 T Canon Vantage Elan, The Netherlands) and 16-slice CT scanner (Siemens SOMATOM.go, The Netherlands). Dogs were positioned in sternal recumbency on the horizontal surface of the table with the head in a flexible coil (MRI) or a head rest (CT), both resulting in elevation of the head of about 2–3 cm to the table. MRI sequences obtained included sagittal T2W (echo time (TE) 110 ms, repetition time (TR) 2.6 s, 2.5 mm slice thickness, 256 × 320 matrix), sagittal T1W (TE 10 ms, TR 0.5 s, 2.5 mm slices, 256 × 320 matrix), transverse T2W of the brain (TE 115 ms, TR 4.1 s, 3.0 mm slices, 160 × 192 matrix), transverse T2W of the cervical spinal cord (TE 115 ms, TR 4.1 s, 3.0 mm slices, 160 × 192 matrix) and transverse T1W of the cervical spinal cord (TE 10 ms, TR 0.4 s, 3.0 mm slices, 160 × 192 matrix). Transverse slices at the level of the cervical spinal cord were adjusted to center the syrinx, if visible. In dogs without a visible syrinx on sagittal images, transverse images were acquired at the level of C2-C3 vertebrae. CT scans were performed with the following parameters: 130 kVp tube voltage, 220 mAs tube current, 256 × 256 image matrix, 0.6 and 0.8 mm slice thickness, 0.4 and 0.6 mm slice increment, 1.0 s rotation time, a pitch of 0.6, and Hr60f (Siemens) kernel.
One observer (KS) reviewed the MRI studies and performed classifications for CM/SM using criteria as previously reported, classifying as CM/SM normal or abnormal (15–17).
One observer (KS) performed quantitative volumetry measurements using dedicated imaging software (Dragonfly 2024 [Computer software]. Comet Technologies Canada Inc., Montreal, Canada; software available at: https://www.theobjects.com/dragonfly). Computer-assisted manual segmentation using the active contouring feature was performed on both MRI and CT images. Window length and width were adjusted as necessary for optimal measurements. Boundaries of volumes of interest were traced on sequential images in three planes of view (transverse plane, and reconstructed dorsal and sagittal planes) (Figure 1).
Figure 1. Example of segmentation of volume of interest (rostral and middle cranial fossa volume) on CT images. (A) Sagittal plane, (B) transverse plane, (C) dorsal plane.
Tracing was assisted by the software active contouring feature, visually assessed, and manually adjusted where necessary. Values (in mm3) were recorded for the following volumes of interest:
(a) Frontal sinus volume (FSV).
Frontal sinus boundaries were defined as gas-filled space and/or diploë between the superficial and deep laminae of the frontal bone. CT only (Figure 2).
(b) Caudal cranial fossa volume (CCFV)*.
Figure 2. Examples of frontal sinus variations (dorsal plane, CT images). (A) Frontal diploë, (B) no frontal sinuses, (C) frontal sinuses (air-filled).
Caudal cranial fossa boundaries were defined the plane between the tentorium cerebelli osseum and the dorsum sellae rostrally, the skull boundaries laterally, dorsally and ventrally, and the plane between the ventral aspect of the supraoccipital bone and the caudal aspect of the basioccipital bone.
(c) Rostral and middle cranial fossa volume (RMCFV)*.
Rostral and middle cranial fossa boundaries were defined the plane between the tentorium cerebelli osseum and the dorsum sellae caudally and the skull boundaries laterally, dorsally, ventrally, and rostrally.
(d) Caudal cranial fossa parenchymal volume (CCFPV)**.
(e) Rostral and middle cranial fossa parenchymal volume (RMCFPV) **.
(f) Cerebellar parenchymal volume (CPV)**.
(g) Brain stem parenchymal volume (BSPV)**.
(h) Ventricular system volume (VSV).
The ventricular system was defined as to include all ventricles (lateral, third, and fourth).
(i) Spinal canal volume between C1-C7 (CSCV).
The spinal canal boundaries were defined as the plane between the ventral aspect of the supraoccipital bone and the caudal aspect of the basioccipital bone rostrally, the vertebral (spinal) canal itself, and the plane between the caudal aspect of the dorsal lamina of C7 and the floor of the vertebral canal at the level of the C7 caudal endplate. CT only.
* A CCFV to RMCFV volume index (VI) was calculated as VI = CCFV/RMCFV*100.
** Parenchymal volumes were defined as brain tissue present in the respective anatomical compartments or the respective parts of the brain as mentioned.
CCFPV% was calculated as CCFPV/CCFV*100.
As illustration, Figure 3 includes an example of the volumes segmented for CCFV, RMCFV, and CSCV.
Figure 3. Example of segmented volumes. Frontal sinus volume (green), caudal cranial fossa volume (orange), rostral and middle cranial fossa volume (blue), and spinal canal volume between C1-C7 (yellow) in 3-dimensional image with overlay of the skeleton (transparent). (A) Lateral view. (B) Dorsal view.
Descriptive statistics are reported using mean (standard deviation) for approximately normally distributed continuous variables and median (interquartile range; IQR) for continuous variables with skewed distributions. Continuous data were tested for normality using a Kolmogorov–Smirnov test. Agreement between MRI- and CT-based quantitative measurements was assessed with intraclass correlation coefficients (ICC, model = two-way random effects, type = agreement, unit = single rating). ICC values including 95% confidence intervals are reported and were interpreted as: < 0.5 = poor; 0.5–0.75 = moderate; 0.75–0.90 = good; > 0.90 = excellent. For further analyses, CT determined values for the volumes of interest were used. Chi-squared tests were used to assess for differences in sex distributions, two-sample t-tests assuming unequal variances (normally distributed data) and Mann Whitney U tests (skewed distributed data) were performed to analyze for differences between CM and SM normal versus abnormal groups of volumetric parameters, age, and body weight. p-values of <0.05 were regarded as significant.
Univariable (binary) logistic regression analysis was performed on the datasets with volumetric parameters, age, and body weight as independent (predictor) variables and CM and SM status (normal versus abnormal) as dependent variables. Variables with (Wald) p-values of <0.20 on univariable modeling were carried forward to a multiple logistic regression model using manual stepwise regression. Spearman coefficients were calculated between independent variable to assess for collinearity and only one parameters of pairs with coefficients of >0.80 were included in multiple logistic regression modeling. Variables with a (Wald) p-value of <0.05 were regarded as significant. Odds ratios and 95% confidence intervals are given for variables included in the final model. Statistical analyses were performed using Microsoft Excel® v2404 and R v4.3.1.
A total of 137 dogs were included in the study. Median weight (3.2 kg), age (3.0 years) and sex distribution (approximately equal) are presented in Table 1. The prevalences of CM and SM in the study population were 65 and 44%, respectively (Table 2).
Table 2. Contingency table including numbers and percentages (of total) of included dogs’ CM and SM classifications.
For all volumetric assessments that were performed on both CT and MRI, ICCs were good (CCFV, RMCFV, RMCFPV, CPV, and BSPV) or excellent (VSV) (Table 3). Of note, 95% confidence interval lower limits were poor (RMCFV, and CPV) or moderate (CCFV, CCFPV, RMCFPV, and BSPV) for all ICCs. Figure 4 illustrates the CCFV of a dog based on CT and MRI.
Figure 4. Caudal cranial fossa volume of a Pomeranian dog segmented on CT and MRI. (A–C) 3-dimensional CT-based images in lateral view, rostrocaudal view, and dorsal view, respectively. (D–F) 3-dimensional MRI-based images in lateral view, rostrocaudal view, and dorsal view, respectively. The CT-based volume was 7,805 mm3 and the MRI-based volume was 9,101 mm3.
Table 4 includes mean and median values for CT-based volumetric parameters as well as results of testing for significant differences between CM/SM normal versus abnormal dogs. These data are graphically depicted in Figure 5.
Figure 5. Bar graph for parameters between SM normal and abnormal dogs. The mean or median (error bars indicating standard deviation or interquartile range respectively) are depicted for each parameter (see Table 4). BSPV, brain stem parenchymal volume; CCFPV, caudal cranial fossa parenchymal volume; CCFV, caudal cranial fossa volume; CPV, cerebellar parenchymal volume; CSCV, spinal canal volume between C1-C7; RMCFPV, rostral and middle cranial fossa parenchymal volume; RMCFV, rostral and middle cranial fossa volume; SM, syringomyelia; VI, volume index; VSV, ventricular system volume. All presented values except for age (years), body weight (kg), and VI (%) are in mm3. *, p < 0.05. Parameters are numerically adjusted to fit the y-axis (10-x).
There were no significant differences between CM normal and abnormal dogs for sex distribution, age, body weight, or any volumetric parameter.
There were significant differences between SM normal and abnormal dogs for body weight, as well as all volumetric parameters except for CCFPV% and RMCFV. There was no difference in sex distribution or age between SM normal versus abnormal groups.
Univariable logistic regression with CM status as dependent variable yielded CCFV (p = 0.10), RMCFV (p = 0.15), and RMCFPV (p = 0.11) as parameters with a p-value of <0.20. Multiple logistic regression did not result in any significantly associated parameters.
Upon univariable logistic regression with SM status as dependent variable, all tested independent variables with the exception of sex distribution, age, and CCFPV% had a p-value of <0.20 [BW (p = 3.4*10−3), FSV (p = 3.6*10−3), CCFV (p = 1.0*10−4), RMCFV (p = 0.10), VI (p = 3.2*10−3), CPV (p = 2.7*10−5), BSPV (p = 3.7*10−4), CCFPV (p = 1.0*10−7), RMCFPV (p = 1.2*10−4), VSV (p = 1.0*10−4), and CSCV (p = 5.1*10−4)].
Multiple logistic regression modeling with SM as dependent variable yielded a best fit model including CCFV (odds ratio for every cm3 increase in CCFV = 0.41, 95% confidence interval 0.25–0.68, p = 5.1*10−4) and VSV (odds ratio for every cm3 increase in VSV = 1.40, 95% confidence interval 1.17–1.67, p = 2.2*10−4); a smaller CCFV and larger VSV were associated with SM. For illustration, Figure 6 includes MRI and CT images of dogs classified as SM normal and abnormal with CCFV and VSV segmentation highlighted.
Figure 6. Magnetic resonance images and computed tomography images of a dog without SM (upper row) and with syringomyelia (SM) (bottom row). (A–E) MRI, sagittal T2W. (B,F) MRI, transverse T2W at the level of the interthalamic adhesion. Segmented caudal cranial fossa volumes (CCFV) and ventricular system volumes (VSV) are shown on corresponding CT images (C,D,G,H). The dog without SM had a CCFV of 8,675 mm3 and a VSV of 1703 mm3. The dog with SM had a CCFV of 6,817 mm3 and a VSV of 7,781 mm3.
In our study, good or excellent agreement between CT and MRI volumetric measurements was found. Such agreement has not specifically been reported before. Of importance is that the lower limits of the 95% confidence intervals were poor to moderate. This means that it is possible that agreement between these two modalities is indeed poor to moderate, rather than good or excellent. In any case, the agreement was not perfect, implying that CT-based and MRI-based measurements and analysis thereof might result in different outcomes. This impacts the interpretation and comparison of different studies using either modality for volumetric assessments.
Many previous studies using MRI referred back to a CT-based publication for validation of their MRI-based measurements (34). Those studies looking at, for instance, CCFV or associated linear measurements in CKCS in relation to SM have mostly employed MRI-based measurements (3, 4, 7, 8, 26, 29, 32, 35). MRI lacks spatial resolution in comparison to CT and bony landmarks are less clearly defined, hampering precise measurements. Moreover, slice thickness and thus voxel dimensions differ between studies, which subjects comparison between absolute and relative values between studies to yet more variability than other factors such as interobserver variability (16).
Unsurprisingly, linear measurements have been found to be associated with volumetric measurements (34). Linear measurements require less time than volumetry and are easier to perform and possibly use for clinical cases. Many of the cited publications on CM/SM in dogs included 2D, linear measurements. Although linear and volume measurements are associated, differences between linear measurements associated with volume and measured volumes will arise. Simply put, measuring associated, indirect indicators of volumes, is not identically valuable compared to actual measurements of the volume (36). Differences between published study results both in comparison to each other and to our study might be explained by these differences in methods (i.e., linear versus volume measurements and CT versus MRI measurements).
For our study, we have made use of specific software that enabled volumetric measurements as detailed in the materials and methods section. There are numerous other software options available, as listed in the references cited above, that could be considered and there are no studies that evaluate the differences between these options specifically. Considerations for the selection of particular software may include for instance: user-friendliness, licensing costs versus open-access, hardware requirements, and suitability to study designs.
We identified no statistically significant differences between dogs with or without CM for any of the studied volumetric variables. While some morphometric differences have been identified between CM affected and non-affected Pomeranians (e.g., foramen magnum height and distance between the dorsal arch of the atlas and the foramen magnum), many other variables studied so far were not significantly different (17, 25). This may be partly explained by questionable reliability of CM grading, with suboptimal interobserver agreement having been identified for both the CKCS and Pomeranian dog breeds (16, 33). Otherwise, the lack of any such differences may represent dissimilarities between Pomeranians and other breeds like the CKCS in relation to the pathogenesis of CM/SM and to what actually constitutes CM in dogs in general; we might not be talking about the exact same thing in all studies as the exact definitions and grading systems are imperfect and partly open to interpretation. Moreover, the effect of positioning for imaging studies is often not accounted for and existing classification schemes reduce CM to a categorical variable with inherent limitations.
Of particular interest might be the lack of associations between CCFV and CM. This is in agreement with the lack of such an association for caudal cranial fossa area (a 2D measurement) as studied previously in Pomeranians (17). This might seem in disagreement with some publication centering on other breeds, like the CKCS (7, 8, 26, 29). However, it must be remembered that these studies looked for differences in CCFV between dogs in relation to SM status, not CM status. As almost all CKCS have CM, these studies were not able to assess for differences between CM affected and unaffected dogs. We too found differences in CCFV as well as VI between dogs with and without SM. This will be discussed below.
For dogs with SM versus without SM, we identified significant differences between Pomeranians with and without SM for numerous volumetric parameters as well as body weight.
Body weight was lower for the dogs with SM than dogs without SM. Interestingly, body weight was not accounted for as a variable in many studies looking at CM/SM in CKCS; a note of great importance as emphasized by Schmidt et al. (35). An effect of body weight on morphometric comparisons among and between dogs with or without SM exists (35). We expected no different for Pomeranians and already recognized this in a previous study (25). Body weight can partly substitute for ‘size’ but body condition has great implications for body weight as well (e.g., dogs of similar size may differ in body weight due to difference in muscle and fat mass). Like Schmidt et al. (35), we did not include body condition scores and can therefore not correct body weights to more accurately reflect size. Nevertheless, assuming – as is our clinical impression – that most of the included dogs had an average body condition score, the difference in body weight implies that dogs with SM were overall smaller than those without. Like body weight, many of the variables we studied are a related to overall size of the dog [allometric relationships between variables exist (see discussion in Santifort et al. (25))]. It can therefore be concluded that, as far as SM in Pomeranians is considered, size matters.
FSV was smaller in dogs with SM than dogs without SM. There is one previous study that looked at frontal sinus morphology in relation to SM in small breed (<15 kg) dogs (31). This study did not measure FSV, but classified dogs into groups based on frontal sinus morphology as ‘absent (no air and no diploë)’, ‘miniscule (some diploë may be seen)’, ‘small (but normal)’, and ‘normal (air-filled)’ (31). The imaging modality used in that study was MRI. The absent and miniscule categorized dogs had higher odds to be affected by SM than dogs in the other categories. In our study, we actually measured FSV on CT images (with better delineation of the bony boundaries) but the conclusion is the same: a smaller frontal sinus is linked to SM. This by no means implies a causal relationship. The association could be coincidental as a smaller FSV may simply be associated to the same factors or be part of the same overall pathogenesis leading to SM. Similarly, Scrivani et al. postulated that SM ‘in many small breed dogs…develops as a result of global malformation of the entire cranial cavity or supratentorial portion of the cavity and is not limited to the infratentorial portion of the cranial cavity’ (31). Just based on FSV, regarding Pomeranians, our results support this theory. However, the argument of allometry needs to be considered (see above under ‘body weight’).
CCFV as well as VI were smaller in dogs with SM than dogs without SM, while there was no statistically significant difference for RMCFV. It follows that while the CCFV is both absolutely and relatively smaller in Pomeranians with SM, the RMCFV is not. CCFV was also one of two (the other being VSV) variables included in the multivariable logistic regression model, identifying it as not only significantly different between SM affected and unaffected dogs, but also as robustly associated with SM status in comparison to other studied variables. In our previous study using 2D measurements, caudal cranial fossa area measured on MRI and CT was significantly smaller in dogs with SM as well (17). These findings are in line with some previous studies in other dog breeds (7, 26, 29). However, there are studies that did not identify statistically significant differences (3, 5, 27, 28, 32). Importantly, some studies that actually (directly) measured CCFV did not identify differences between affected and non-affected dogs (3, 32). In a human meta-analysis, posterior cranial fossa volume was associated with SM (36). However, size considerations and impact thereof (see above under ‘body weight’) are less of a confounder in these human studies (35). Hence, future canine studies considering this parameter will provide more information for the continued debate on relevance of CCFV in the pathogenesis of SM.
VSV was larger in dogs with SM than dogs without SM. VSV was also one of two (the other being CCFV) variables included in the multivariable logistic regression model, identifying it as not only significantly different between SM affected and unaffected dogs, but also as robustly associated with SM status in comparison to other studied variables. The association of SM and VSV has been reported in the CKCS and, indirectly, in Chihuahuas as well (5, 6). No association was found in a study that assessed lateral ventricles parameters in 2D and categorically (i.e., grouped as 0–14% = absent ventriculomegaly; 15–25% = moderate ventriculomegaly; >25% = severe ventriculomegaly) (21). As VSV is a continuous variable and volumetry is more accurately assessed by 3D than 2D measurements, current evidence seems to support a role of VSV increase in dogs with SM. Whether this variable is causative or secondary to, for instance, altered cerebrospinal fluid (CSF) flow dynamics (i.e., merely being associated with SM in a non-causative, coincidental manner) remains to be shown. Longitudinal studies evaluating both VSV as well as SM development might help to gain more insights to begin to answer this question.
While CCFPV was significantly smaller in dogs with SM, CCFPV% was not. This indicates that relative overcrowding of the caudal cranial fossa does not seem to be implicated in the pathogenesis of SM in Pomeranians as has been suggested for the CKCS (4, 5, 29, 37, 38). These studies differed in many aspects to ours and among themselves, such as (1) mainly CKCS dogs included, (2) some of the studies used 2D measurements, (3) weight was not always considered (see discussion under ‘body weight’) for absolute measurements, and (4) ventricular size was not accounted for. The differences in study results could be due to each or a combination of these factors. Likewise, though RMCFPV, CPV, and BSPV were significantly smaller in dogs with SM, these are influenced by overall size of the dog (see discussion under ‘body weight’) and also ventricular dimensions. The Monro-Kellie Doctrine dictates that increase in one intracranial compartment (CSF, blood, parenchyma) has to be compensated by decrease in another; in dogs with increased ventricular volume, brain parenchyma volume (mostly white matter) is reduced (39). Our finding of association between VSV and SM is indicative of influence on the parenchymal volume factors as well. This is supported by the fact that these parenchymal volumes were not valuable for the logistic regression model.
CSCV was smaller for dogs with SM than dogs without SM in our study. Spinal canal width (a 2D measurement) at C2-3 was increased for dogs with SM in another study (27). Differences between study methods could explain this contrast in findings (e.g., dog breeds, positioning for scans, 2D versus 3D). As for the other parameters, CSCV was not of value in the logistic regression and the argument of allometry is of importance to consider (i.e., smaller dogs have smaller CSCV, see discussion above under ‘body weight’). While absolute volume of the spinal canal might be interesting, it is important also to consider the effect of dynamics and its postulated role in the development of SM (40). Cervical spinal cord movements and the effect thereof on volumes measured may have an important effect on SM development. Cervical stabilization has even been proposed as an (adjunctive) surgical method to treat SM in people (40). Pomeranians are often affected by numerous other CJAs that might influence cervical and CSF dynamics. The role of CSCV and dynamics in the development of SM is therefore complex. Future studies assessing associations between CJAs and SM in Pomeranians could help to gain more understanding of their influence.
Consistent with the literature, no sex predispositions were identified for CM/SM in dogs in this study. Age was not identified as significantly different between the SM affected and non-affected dogs, like in two previously published studies (17, 25) but unlike our other study with the largest study population (15). In another previous study on a small cohort of Pomeranians, we found that SM was progressive over time (24). Other studies have also identified SM to be associated with age or have characterized SM to be progressive over time in CKCS (28, 41–46). While we did not identify an association with age in this study, SM was not evaluated longitudinally over time. Differences in the studied number of dogs between this and previous studies could also have impacted the lack of a significant difference in this study (15, 17).
There are limitations to this study, including the lack of intra- and interobserver reliability assessments and 3D sequences for MRI studies that would facilitate more accurate volumetry compared to 2D sequences, and, as for other studies, the possibility of misclassification of dogs with CM (16, 33). Nevertheless, our study provides valuable insights into the pathogenesis of CM/SM in Pomeranians.
In conclusion, smaller CCFV and larger VSV are associated with increased odds of SM in Pomeranians. Such parameters, along with others that we have studied (14, 16, 17, 25) may be taken into account in the overall assessment of CM/SM and the selection of dogs for breeding programs of this breed. The correlation between body weight and SM and its implication on overall size might be considered in breeding practices but further research accounting for body condition scores would be valuable to verify such findings.
The raw data supporting the conclusions of this article will be made available by the authors, without undue reservation.
The animal study was approved by Animal Welfare Body Utrecht (Utrecht University, The Netherlands). The study was conducted in accordance with the local legislation and institutional requirements.
KS: Conceptualization, Data curation, Formal Analysis, Funding acquisition, Investigation, Methodology, Resources, Visualization, Writing – original draft, Writing – review & editing. IC: Conceptualization, Methodology, Supervision, Writing – review & editing. PM: Conceptualization, Data curation, Funding acquisition, Investigation, Methodology, Project administration, Resources, Supervision, Validation, Writing – review & editing.
The author(s) declare that financial support was received for the research, authorship, and/or publication of this article. The authors declare that this study received funding from IVC Evidensia. The funder was not involved in the study design, collection, analysis, interpretation of data, the writing of this article, or the decision to submit it for publication.
The authors would like to thank IVC Evidensia’s research fund and publication fund for providing financial support for this study and its publication.
IC was employed by Vet Oracle Teleradiology.
The remaining authors declare that the research was conducted in the absence of any commercial or financial relationships that could be construed as a potential conflict of interest.
The author(s) declared that they were an editorial board member of Frontiers, at the time of submission. This had no impact on the peer review process and the final decision.
The authors declare that no Generative AI was used in the creation of this manuscript.
All claims expressed in this article are solely those of the authors and do not necessarily represent those of their affiliated organizations, or those of the publisher, the editors and the reviewers. Any product that may be evaluated in this article, or claim that may be made by its manufacturer, is not guaranteed or endorsed by the publisher.
1. Ancot, F, Lemay, P, Knowler, SP, Kennedy, K, Griffiths, S, Cherubini, GB, et al. A genome-wide association study identifies candidate loci associated to syringomyelia secondary to Chiari-like malformation in cavalier king Charles spaniels. BMC Genet. (2018) 19:16. doi: 10.1186/s12863-018-0605-z
2. Andrino, S, Lorenzo, V, Dunner, S, Contreras, E, Cañón, J, and Sevane, N. Syringohydromyelia in dogs: the genomic component underlying a complex neurological disease. Animals. (2022) 12:2622. doi: 10.3390/ani12192622
3. Cerda-Gonzalez, S, Olby, NJ, McCullough, S, Pease, AP, Broadstone, R, and Osborne, JA. Morphology of the caudal fossa in cavalier king Charles spaniels. Vet Radiol Ultrasound. (2009) 50:37–46. doi: 10.1111/j.1740-8261.2008.01487.x
4. Cross, HR, Cappello, R, and Rusbridge, C. Comparison of cerebral cranium volumes between cavalier king Charles spaniels with Chiari-like malformation, small breed dogs and Labradors. J Small Anim Pract. (2009) 50:399–405. doi: 10.1111/j.1748-5827.2009.00799.x
5. Driver, CJ, Rusbridge, C, Cross, HR, McGonnell, I, and Volk, HA. Relationship of brain parenchyma within the caudal cranial fossa and ventricle size to syringomyelia in cavalier king Charles spaniels. J Small Anim Pract. (2010) 51:382–6. doi: 10.1111/j.1748-5827.2010.00952.x
6. Kiviranta, AM, Rusbridge, C, Lappalainen, AK, Junnila, JJT, and Jokinen, TS. Persistent fontanelles in Chihuahuas. Part II: association with craniocervical junction abnormalities, syringomyelia, and ventricular volume. J Vet Intern Med. (2021) 35:1848–56. doi: 10.1111/jvim.16123
7. Knowler, SP, Kiviranta, AM, McFadyen, AK, Jokinen, TS, La Ragione, RM, and Rusbridge, C. Craniometric analysis of the hindbrain and Craniocervical junction of Chihuahua, Affenpinscher and cavalier king Charles spaniel dogs with and without Syringomyelia secondary to Chiari-like malformation. PLoS One. (2017) 12:e0169898. doi: 10.1371/journal.pone.0169898
8. Knowler, SP, Cross, C, Griffiths, S, McFadyen, AK, Jovanovik, J, Tauro, A, et al. Use of morphometric mapping to characterise symptomatic Chiari-like malformation, secondary Syringomyelia and associated Brachycephaly in the cavalier king Charles spaniel. PLoS One. (2017) 12:e0170315. doi: 10.1371/journal.pone.0170315
9. Knowler, SP, McFadyen, AK, Freeman, C, Kent, M, Platt, SR, Kibar, Z, et al. Quantitative analysis of Chiari-like malformation and syringomyelia in the griffon Bruxellois dog. PLoS One. (2014) 9:e88120. doi: 10.1371/journal.pone.0088120
10. Limpens, C, Smits, VTM, Fieten, H, and Mandigers, PJJ. The effect of MRI-based screening and selection on the prevalence of syringomyelia in the Dutch and Danish cavalier king Charles spaniels. Front Vet Sci. (2024) 11:1326621. doi: 10.3389/fvets.2024.1326621
11. Pedersen, TR, Bach, MBT, Stougaard, CL, Gredal, H, Rusbridge, C, Finnerup, NB, et al. Clinical predictors of syringomyelia in cavalier king Charles spaniels with chiari-like malformation based on owners' observations. Acta Vet Scand. (2024) 66:5. doi: 10.1186/s13028-024-00725-1
12. Rusbridge, C, Knowler, SP, Pieterse, L, and McFadyen, AK. Chiari-like malformation in the griffon Bruxellois. J Small Anim Pract. (2009) 50:386–93. doi: 10.1111/j.1748-5827.2009.00744.x
13. Rusbridge, C, Stringer, F, and Knowler, SP. Clinical application of diagnostic imaging of Chiari-like malformation and Syringomyelia. Front Vet Sci. (2018) 5:280. doi: 10.3389/fvets.2018.00280
14. Rusbridge, C, McFadyen, AK, and Knower, SP. Behavioral and clinical signs of Chiari-like malformation-associated pain and syringomyelia in cavalier king Charles spaniels. J Vet Intern Med. (2019) 33:2138–50. doi: 10.1111/jvim.15552
15. Santifort, KM, Carrera, I, Bossens, K, and Mandigers, PJJ. Phenotypic characterization of Pomeranians with or without Chiari-like malformation and syringomyelia. Front Vet Sci. (2023) 10:1320942. doi: 10.3389/fvets.2023.1320942
16. Santifort, K, Bellekom, S, Carrera, I, and Mandigers, P. Craniocervical morphometry in Pomeranians-part I: intra-observer, Interobserver, and Intermodality (CT vs. MRI) Agreement. Animals. (2024) 14:1854. doi: 10.3390/ani14131854
17. Santifort, K, Bellekom, S, Carrera, I, and Mandigers, P. Craniocervical morphometry in Pomeranians-part II: associations with Chiari-like malformation and Syringomyelia. Animals. (2024) 14:1859. doi: 10.3390/ani14131859
18. Sanchis-Mora, S, Pelligand, L, Thomas, CL, Volk, HA, Abeyesinghe, SM, Brodbelt, DC, et al. Dogs attending primary-care practice in England with clinical signs suggestive of Chiari-like malformation/syringomyelia. Vet Rec. (2016) 179:436. doi: 10.1136/vr.103651
19. Sparks, CR, Cerda-Gonzalez, S, Griffith, EH, Lascelles, BDX, and Olby, NJ. Questionnaire-based analysis of owner-reported scratching and pain signs in cavalier king Charles spaniels screened for Chiari-like malformation and Syringomyelia. J Vet Intern Med. (2018) 32:331–9. doi: 10.1111/jvim.14856
20. Spiteri, M, Knowler, SP, Rusbridge, C, and Wells, K. Using machine learning to understand neuromorphological change and image-based biomarker identification in cavalier king Charles spaniels with Chiari-like malformation-associated pain and syringomyelia. J Vet Intern Med. (2019) 33:2665–74. doi: 10.1111/jvim.15621
21. Tirrito, F, Cozzi, F, Bonaldi, M, Corazzo, S, Contiero, B, and Lombardo, R. Ventriculomegaly in cavalier king Charles spaniels with Chiari-like malformation: relationship with clinical and imaging findings. J Vet Med Sci. (2022) 84:1185–93. doi: 10.1292/jvms.22-0134
22. Hoholm, F, Bruining-Staal, K, and Mandigers, PJJ. Incidence of Chiari-like malformation/Syringomyelia in a cohort of small dog breeds scanned using MRI over a period of 8 years in the Netherlands. Pets. (2024) 1:267–76. doi: 10.3390/pets1030019
23. Toader, C, Ples, H, Covache-Busuioc, R-A, Costin, HP, Bratu, B-G, Dumitrascu, D-I, et al. Decoding Chiari malformation and Syringomyelia: from epidemiology and genetics to advanced diagnosis and management strategies. Brain Sci. (2023) 13:1658. doi: 10.3390/brainsci13121658
24. Santifort, KM, Carrera, I, and Mandigers, PJJ. Longitudinal assessment of syringomyelia in Pomeranians. Front Vet Sci. (2024) 11:1364464. doi: 10.3389/fvets.2024.1364464
25. Santifort, KM, Bellekom, S, Carrera, I, and Mandigers, PJJ. CT-based and manual external skull measurements for Chiari-like malformation and syringomyelia in Pomeranians. PLoS One. (2024) 19:e0313203. doi: 10.1371/journal.pone.0313203
26. Carrera, I, Dennis, R, Mellor, DJ, Penderis, J, and Sullivan, M. Use of magnetic resonance imaging for morphometric analysis of the caudal cranial fossa in cavalier king Charles spaniels. Am J Vet Res. (2009) 70:340–5. doi: 10.2460/ajvr.70.3.340
27. Carruthers, H, Rusbridge, C, Dubé, MP, Holmes, M, and Jeffery, N. Association between cervical and intracranial dimensions and syringomyelia in the cavalier king Charles spaniel. J Small Anim Pract. (2009) 50:394–8. doi: 10.1111/j.1748-5827.2009.00768.x
28. Couturier, J, Rault, D, and Cauzinille, L. Chiari-like malformation and syringomyelia in normal cavalier king Charles spaniels: a multiple diagnostic imaging approach. J Small Anim Pract. (2008) 49:438–43. doi: 10.1111/j.1748-5827.2008.00578.x
29. Driver, CJ, Rusbridge, C, McGonnell, IM, and Volk, HA. Morphometric assessment of cranial volumes in age-matched cavalier king Charles spaniels with and without syringomyelia. Vet Rec. (2010) 167:978–9. doi: 10.1136/vr.c4109
30. Shaw, TA, McGonnell, IM, Driver, CJ, Rusbridge, C, and Volk, HA. Caudal cranial fossa partitioning in cavalier king Charles spaniels. Vet Rec. (2013) 172:341. doi: 10.1136/vr.101082
31. Scrivani, PV, Thompson, MS, Winegardner, KR, Dewey, CW, and Scarlett, JM. Association between frontal-sinus size and syringohydromyelia in small-breed dogs. Am J Vet Res. (2007) 68:610–3. doi: 10.2460/ajvr.68.6.610
32. Schmidt, MJ, Biel, M, Klumpp, S, Schneider, M, and Kramer, M. Evaluation of the volumes of cranial cavities in cavalier king Charles spaniels with Chiari-like malformation and other brachycephalic dogs as measured via computed tomography. Am J Vet Res. (2009) 70:508–12. doi: 10.2460/ajvr.70.4.508
33. Weber, SM, Hostnik, ET, Drost, WT, Hamlin, AN, Ledesma, MA, Timperman, L, et al. Comparison of high-field MRI and multidetector CT for grading Chiari-like malformation and syringomyelia in cavalier king Charles spaniels. Vet Radiol Ultrasound. (2020) 61:444–52. doi: 10.1111/vru.12862
34. García-Real, I, Kass, PH, Sturges, BK, and Wisner, ER. Morphometric analysis of the cranial cavity and caudal cranial fossa in the dog: a computerized tomographic study. Vet Radiol Ultrasound. (2004) 45:38–45. doi: 10.1111/j.1740-8261.2004.04006.x
35. Schmidt, MJ, Amort, KH, Failing, K, Klingler, M, Kramer, M, and Ondreka, N. Comparison of the endocranial- and brain volumes in brachycephalic dogs, mesaticephalic dogs and cavalier king Charles spaniels in relation to their body weight. Acta Vet Scand. (2014) 56:30. doi: 10.1186/1751-0147-56-30
36. Wang, Z, Li, Z, Han, S, Hu, X, Pang, S, Li, Y, et al. Magnetic resonance imaging-related anatomic and functional parameters for the diagnosis and prognosis of Chiari malformation type I: a systematic review and Meta-analysis. Neurospine. (2024) 21:510–24. doi: 10.14245/ns.2347150.575
37. Fenn, J, Schmidt, MJ, Simpson, H, Driver, CJ, and Volk, HA. Venous sinus volume in the caudal cranial fossa in cavalier king Charles spaniels with syringomyelia. Vet J. (2013) 197:896–7. doi: 10.1016/j.tvjl.2013.05.007
38. Shaw, TA, McGonnell, IM, Driver, CJ, Rusbridge, C, and Volk, HA. Increase in cerebellar volume in cavalier king Charles spaniels with Chiari-like malformation and its role in the development of syringomyelia. PLoS One. (2012) 7:e33660. doi: 10.1371/journal.pone.0033660
39. Schmidt, MJ, Laubner, S, Kolecka, M, Failing, K, Moritz, A, Kramer, M, et al. Comparison of the relationship between cerebral white matter and grey matter in normal dogs and dogs with lateral ventricular enlargement. PLoS One. (2015) 10:e0124174. doi: 10.1371/journal.pone.0124174
40. Rai, SK, and Rai, PS. Volume change theory for syringomyelia: a new perspective. Asian J Neurosurg. (2015) 10:245–51. doi: 10.4103/1793-5482.162680
41. Cerda-Gonzalez, S, Olby, NJ, and Griffith, EH. Longitudinal study of the relationship among craniocervical morphology, clinical progression, and syringomyelia in a cohort of cavalier king Charles spaniels. J Vet Intern Med. (2016) 30:1090–8. doi: 10.1111/jvim.14362
42. Driver, CJ, De Risio, L, Hamilton, S, Rusbridge, C, Dennis, R, McGonnell, IM, et al. Changes over time in craniocerebral morphology and syringomyelia in cavalier king Charles spaniels with Chiari-like malformation. BMC Vet Res. (2012) 8:215. doi: 10.1186/1746-6148-8-215
43. Knowler, SP, McFadyen, AK, and Rusbridge, C. Effectiveness of breeding guidelines for reducing the prevalence of syringomyelia. Vet Rec. (2011) 169:681. doi: 10.1136/vr.100062
44. Loderstedt, S, Benigni, L, Chandler, K, Cardwell, JM, Rusbridge, C, Lamb, CR, et al. Distribution of syringomyelia along the entire spinal cord in clinically affected cavalier king Charles spaniels. Vet J. (2011) 190:359–63. doi: 10.1016/j.tvjl.2010.12.002
45. Parker, JE, Knowler, SP, Rusbridge, C, Noorman, E, and Jeffery, ND. Prevalence of asymptomatic syringomyelia in cavalier king Charles spaniels. Vet Rec. (2011) 168:667. doi: 10.1136/vr.d1726
46. Wijnrocx, K, van, L, Eggelmeijer, W, Noorman, E, Jacques, A, Buys, N, et al. Twelve years of chiari-like malformation and syringomyelia scanning in cavalier king Charles spaniels in the Netherlands: towards a more precise phenotype. PLoS One. (2017) 12:e0184893. doi: 10.1371/journal.pone.0184893
Keywords: CM/SM, volumetry, segmentation, caudal cranial fossa, ventricular system
Citation: Santifort KM, Carrera I and Mandigers PJJ (2025) CT- and MRI-based volumetry for Chiari-like malformation and syringomyelia in Pomeranians. Front. Vet. Sci. 12:1549205. doi: 10.3389/fvets.2025.1549205
Received: 20 December 2024; Accepted: 12 February 2025;
Published: 26 February 2025.
Edited by:
Marios Charalambous, University of Veterinary Medicine Hannover, GermanyReviewed by:
Sam Long, Veterinary Referral Hospital, AustraliaCopyright © 2025 Santifort, Carrera and Mandigers. This is an open-access article distributed under the terms of the Creative Commons Attribution License (CC BY). The use, distribution or reproduction in other forums is permitted, provided the original author(s) and the copyright owner(s) are credited and that the original publication in this journal is cited, in accordance with accepted academic practice. No use, distribution or reproduction is permitted which does not comply with these terms.
*Correspondence: Koen M. Santifort, a29lbi5zYW50aWZvcnRAZXZpZGVuc2lhLm5s; Paul J. J. Mandigers, cC5qLmoubWFuZGlnZXJzQHV1Lm5s
†ORCID: Paul J. J. Mandigers, orcid.org/0000-0003-2547-6673
Disclaimer: All claims expressed in this article are solely those of the authors and do not necessarily represent those of their affiliated organizations, or those of the publisher, the editors and the reviewers. Any product that may be evaluated in this article or claim that may be made by its manufacturer is not guaranteed or endorsed by the publisher.
Research integrity at Frontiers
Learn more about the work of our research integrity team to safeguard the quality of each article we publish.