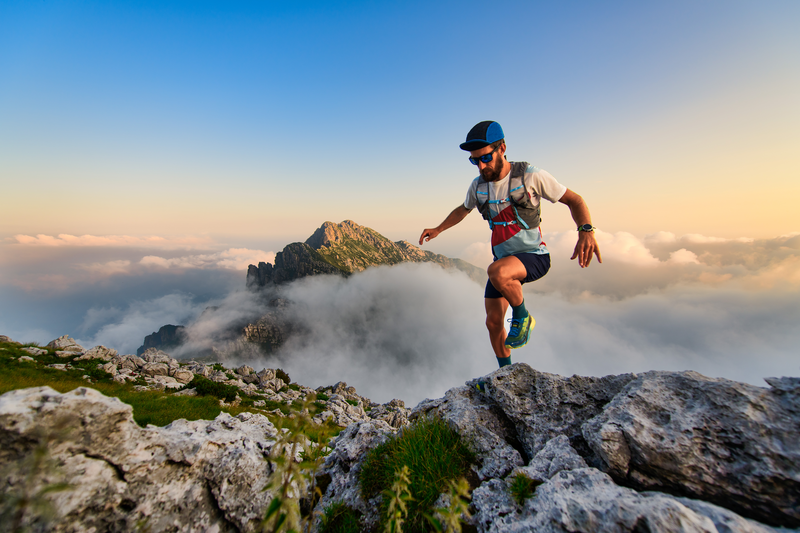
94% of researchers rate our articles as excellent or good
Learn more about the work of our research integrity team to safeguard the quality of each article we publish.
Find out more
BRIEF RESEARCH REPORT article
Front. Vet. Sci.
Sec. Oncology in Veterinary Medicine
Volume 12 - 2025 | doi: 10.3389/fvets.2025.1546872
This article is part of the Research Topic Cancer in Domestic, Exotic and Wild Animals: New Horizons in Tumorigenesis, Diagnosis, Prognosis and Therapeutics through Comparative Oncology View all 8 articles
The final, formatted version of the article will be published soon.
You have multiple emails registered with Frontiers:
Please enter your email address:
If you already have an account, please login
You don't have a Frontiers account ? You can register here
Canine osteosarcomas (COS) are the most common bone tumors in dogs, characterized by high metastatic rates, poor prognosis, and poor responsiveness to routine therapies, which highlights the need for new treatment targets. In this context, the metabolism of neoplastic cells represents an increasingly studied element, as cancer cells depend on particular metabolic pathways that are also elements of vulnerability. Among these, tumor cells (TCs) show higher iron requirements to sustain proliferation (so-called iron addiction), which are achieved by increasing iron uptake and/or by activating ferritinophagy, a process mediated by the Nuclear receptor Co-Activator 4 (NCOA4) leading to iron mobilization from ferritin (Ft) deposits. Previous studies have shown that COS cells overexpress Transferrin Receptor 1 (TfR1) to increase iron uptake. In this study we evaluated the immunohistochemical expression of ferritinophagy-related proteins, namely Ferritin Heavy chain (FTH1) and NCOA4, and proliferating cell nuclear antigen (PCNA) in canine normal bone and canine osteoblastic osteosarcoma (COOS) samples. Normal samples revealed negative/weak immunoreactivity for FTH1, NCOA4 and PCNA in < 10% of osteocytes. In COOS samples the majority of neoplastic cells showed immunoreactivity to FTH1, NCOA4 and PCNA. Our data suggest that the activation of ferritinophagy by COOS cells responds to the need for feed their "iron addiction". These data, though preliminary, further suggest that targeting iron metabolism represents a new potential strategy worthy of further study to be transferred into clinical practice.
Keywords: Bone cancer, Canine osteosarcoma, Immunohistochemistry, iron metabolism, therapy
Received: 17 Dec 2024; Accepted: 06 Mar 2025.
Copyright: © 2025 Power, Leandri, Federico, De Vico and Leonardi. This is an open-access article distributed under the terms of the Creative Commons Attribution License (CC BY). The use, distribution or reproduction in other forums is permitted, provided the original author(s) or licensor are credited and that the original publication in this journal is cited, in accordance with accepted academic practice. No use, distribution or reproduction is permitted which does not comply with these terms.
* Correspondence:
Karen Power, Department of Biology, Polytechnic and Basic Sciences School, University of Naples Federico II, Naples, Italy
Disclaimer: All claims expressed in this article are solely those of the authors and do not necessarily represent those of their affiliated organizations, or those of the publisher, the editors and the reviewers. Any product that may be evaluated in this article or claim that may be made by its manufacturer is not guaranteed or endorsed by the publisher.
Research integrity at Frontiers
Learn more about the work of our research integrity team to safeguard the quality of each article we publish.