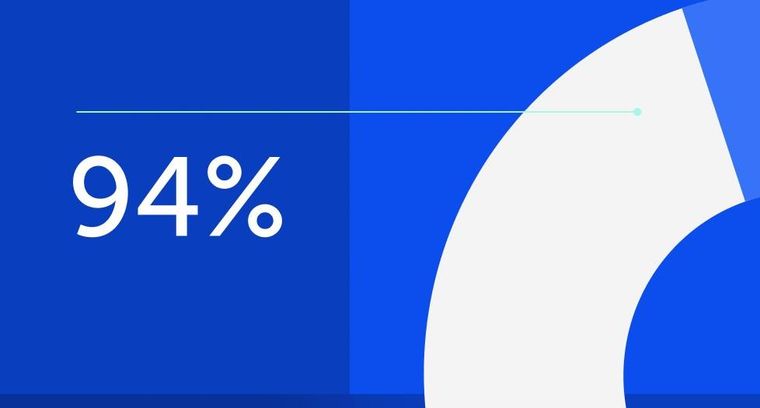
94% of researchers rate our articles as excellent or good
Learn more about the work of our research integrity team to safeguard the quality of each article we publish.
Find out more
ORIGINAL RESEARCH article
Front. Vet. Sci., 31 March 2025
Sec. Parasitology
Volume 12 - 2025 | https://doi.org/10.3389/fvets.2025.1543162
This article is part of the Research TopicInnovative Approaches for Precise Identification and Control of Ticks and Tick-Borne PathogensView all 10 articles
Introduction: Tick-borne diseases represent a major threat to both animal and human health globally. This study explores the prevalence of tick infestation and associated piroplasm infections specifically Theileria and Babesia species in cattle, in addition to evaluating the acaricidal effectiveness of Chrysanthemum extract (Dendranthema grandiflora) and neem oil emulsion (Azadirachta indica).
Methods: Among 130 cattle examined, 61 were infested with ticks and subsequently screened for piroplasm infections. Molecular analysis identified infections caused by Theileria annulata and Babesia bigemina.
Results: A strong association was found between tick infestation and Babesia species, while T. annulata infection showed a slight correlation. Hemolymph examination confirmed the critical role of ticks in the life cycle of piroplasm infection. Chrysanthemum extract and neem oil were tested for their acaricidal properties against adult ticks (Rhipicephalus annulatus). Chrysanthemum extract (0.5 mg/mL) caused tick mortality within 24 h. However, neem oil induced rapid and significant tick mortality at (20 mg/L) and (15 mg/L), achieving 100% mortality within the same time frame. Both treatments demonstrated high effectiveness, with results indicating strong dose-and time-dependent effects compared to controls. Scanning electron microscopy (SEM) revealed extensive morphological damage to treated ticks. This damage included destruction of the hypostome, loss of surface striations, wrinkling with pore formation, and cracking following exposure to neem oil and Chrysanthemum extract.
Discussion: These findings highlight the potential of D. grandiflora extract and neem oil emulsion as effective natural acaricides for controlling tick infestations and reducing tick-borne diseases.
Ticks are blood-feeding ectoparasites that pose a global health concern. The cattle tick, Rhipicephalus annulatus, is one of the most economically significant vectors affecting human and animal health (1). Distributed across many tropical and subtropical areas, where they naturally contribute to the maintenance of tick-borne diseases in both animals and humans (2). Consequently, ticks may be an effective indicator for tracking tick-borne parasites (TBPs) due to their capacity to transmit blood and pathogens from many hosts. Data on the geographical distribution of pathogens in ticks help assess the risk of exposure to tick bites and, consequently, the risk of disease transmission (3).
Tick-borne diseases such as theileriosis and babesiosis significantly threaten the cattle industry (4). Blood parasites are transmitted when infected ticks inject parasites into the host’s bloodstream while feeding (5). In Egypt, T. annulata causes tropical theileriosis, characterized by symptoms such as fever, anorexia, jaundice, tachycardia, difficulty breathing, lymphadenopathy, and general weakness, severely impacting cattle productivity (6). Different species of Babesia, (B. divergens, B. naoakii, B. bigemina, and B. bovis) cause babesiosis, which is characterized by fever, hemolytic anemia complicated with neurological and respiratory disorders, which can be fatal (7). The molecular characterization and phylogenetic analysis of Babesia and Theileria are essential for understanding the diversity, evolution, and epidemiology of these protozoan parasites (28). This knowledge is crucial for developing targeted control measures, vaccines, and diagnostic tools, which ultimately aid in managing tick-borne diseases. Additionally, genetic characterization can provide insights into host-parasite interactions and help predict how these pathogens may adapt to environmental changes or develop resistance to treatments. This underscores the importance of this research in both veterinary and human health (59).
Therefore, addressing tick control is essential for sustaining cattle health and productivity (8). Currently, the National Drug Authority has registered more than 25 acaricide brands readily available to farmers (9). Regrettably, ticks in Egypt have developed resistance to all available acaricides (10). This resistance and environmental and health concerns have driven a shift toward exploring alternative tick control methods (11). Consequently, there is upwards interest in evaluating the acaricidal efficacy of natural, environmentally sustainable, and safer biological agents, such as essential oils and plant extracts (12).
Natural products often contain bioactive compounds that exhibit insecticidal, repellent, and growth-regulating properties (13). Among these alternatives is Pyrethrum, which is recognized as a substantial reservoir of pyrethrins (14). They are derived from the flowers of Chrysanthemum cinerariaefolium and have been used as insecticides for millennia. These natural neurotoxins target the nervous system of insects, causing paralysis and death (15). They are effective against a broad spectrum of pests as they act rapidly upon contact (16). The development of synthetic pyrethroids, such as permethrin, has enhanced the insecticidal properties and environmental stability of these compounds. Consequently, they are extensively utilized in agricultural, veterinary, and household pest control products (17). Other species of Chrysanthemum may have insecticidal properties for tick control, but there is limited research on their effectiveness against R. annulatus ticks.
Neem oil, derived from the seeds of the neem tree (Azadirachta indica), is a natural source of insecticidal compounds. It contains a complex mixture of bioactive compounds. The primary compound, azadirachtin, disrupts various physiological processes in ticks, such as feeding, reproduction, and molting (18, 19). It acts as an insect growth regulator (IGR) and interferes with the synthesis and release of molting hormones, leading to disruption of the molting process and future reproduction (20). Additionally, azadirachtin reduces ticks by deterring them from attaching to and feeding on their hosts due to its anti-feedant and repellent properties. Using botanical insecticides, such as Chrysanthemum extract and neem oil, is consistent with the principles of integrated pest management (IPM). IPM underscores the use of multiple, complementary control strategies to attain effective acaricidal management while reducing its environmental impact (21). Despite the potential of Chrysanthemum extract and neem oil as tick control agents, significant knowledge gaps need addressing. Research is needed to determine the best formulations, application methods, and dosages for these insecticides to maximize their effectiveness in controlling R. annulatus while ensuring the safety of animals. This research aims to provide valuable insights for the development of alternative and eco-friendly strategies for tick control.
A total of 130 cattle, aged between one and five years old, were examined at the veterinary clinic of the Faculty of Veterinary Medicine at Assiut University in Assiut, Egypt, from January 2023 to December 2023. The examined cattle consisted of 52 males and 78 females. Only infested animals with ticks (61) were included in the study to further assess tick-piroplasm association.
Five milliliters of blood were collected from the jugular vein of all animals using sterile vacutainer tubes containing anticoagulants. The blood samples were then transported on ice to the Parasitology Department for further examination. Fresh thin blood smears were prepared, dried, and fixed in methanol to identify piroplasm infection using a light microscope (Olympus BX43F, Tokyo 163-0914, Japan). Positive samples were used for molecular analysis and species identification (22).
All positive samples with same morphological characteristics, obtained from the same host and region, underwent DNA genome extraction using the QIAamp DNA Mini Kit (Catalogue 51304, Qiagen). Two target genes were selected for amplification: tams1 for Theileria annulata and 18S rRNA for Babesia. The primer sequences for each gene were as follows: tams1 primers were 5′-GTAACCTTTAAAAACGT-3′ and 5′-GTTACGAACATGGGTTT-3′, yielding a 721 bp product (23). The 18S rRNA primers were 5′-GTCTTGTAATTGGAATGATGGTGAC-3′ and 5′-ATGCCCCCAACCGTTCCTATTA-3′, producing a 340 bp product (24). PCR amplification was carried out using the EmeraldAmp GT PCR Master Mix (Takara, Code No. RR310A). Each reaction mixture involved (12.5 μL) of 2× premix, (5.5 μL) of PCR-grade water, (1 μL) of each primer (20 pmol), and (5 μL) of a DNA template, and the total reaction volume was (25 μL). The conditions of the thermal cycling started with initial denaturation for 5 min at 94°C, followed by 35 cycles for 30 s at 94°C, annealing for 40 s at 55°C, and extension for 45 s at 72°C (for tams1), and 40 s (for 18S rRNA), final extension for 10 min at 72°C end the PCR. Ten microliters aliquots of each PCR reaction were loaded onto a 1.5% agarose gel in a horizontal gel electrophoresis system (Compact M, Biometric, Germany), stained with ethidium bromide, and visualized under UV light to confirm successful amplification (25).
The QIAquick PCR Purification Kit (Qiagen Inc., Valencia, CA) was used to purify PCR products. The PCR product was mixed with Buffer PB1 (five volumes), the mixture was added to a QIAquick spin column, washed with Buffer PE, and then the purified DNA was eluted with nuclease-free water. This procedure needs approximately 10 min to produce purified DNA suitable for subsequent sequencing applications.
PCR products were sequenced in forward and reverse directions using an automated DNA sequencer (Applied Biosystems, United States). The sequencing was performed with the BigDye Terminator v3.1 Cycle Sequencing Kit (Perkin-Elmer/Applied Biosystems). After sequencing, the NCBI BLAST tool was used to compare the sequences to those in GenBank that already existed. This comparison allowed us to identify similarities and differences between the freshly generated sequences and those already in the database.
Following sequencing, the obtained sequences were aligned using the CLUSTAL W algorithm, integrated into the MegAlign software (version 12.1) (26). MEGA11 software was used to perform Phylogenetic analyses, engaging multiple methods such as maximum likelihood, neighbor-joining, and maximum parsimony (27).
The ticks were carefully removed using soft forceps and prepared for morphological characterization. They were steeped in potassium hydroxide solution (5%) and then transferred to 2.5% an acid alcohol solution for pH regulation, dehydrated in an escalating sequence of ethanol alcohol, and finally immersed in xylene to become transparent for 24 h (28). Microscopic and molecular identification of tick’s species, Rhipicephalus annulatus, was previously reported using the coxI gene and deposited in the Gene bank under accession number (OR965090) (28).
The isolated ticks were used to prepare the hemolymph smears, to detect the presence of ookinetes of piroplasm following the method defined by Allam et al. (29). The procedure involved amputating the distal portion of one or more legs of the tick to obtain a small drop of hemolymph on a clean glass slide. The smears were air dried, fixed in absolute methanol for 10–15 min, stained with Giemsa’s stain, and examined under an oil immersion light microscope.
In May 2024, the aerial parts of the Chrysanthemum (Dendranthema grandiflora) were collected from the Floriculture Farm at the Faculty of Agriculture, Assiut University, Egypt. Chrysanthemum is an herbaceous flowering plant known for its ornamental significance. It encompasses a range of varieties displaying notable genetic diversity in traits such as flower yield, quality, and growth parameters. The specific variety under investigation is characterized by its white flowers. The plant material was air-dried, ground into a 200 g powder, and macerated in 70% methanol for 24 h. After filtration and concentration using a rotary evaporator, this process was repeated to obtain a 60 g dry residue. The Chrysanthemum extract was then diluted in distilled water and glycerol to create a series of varying concentrations: 0.125, 0.25, and 0.5 g/mL (30).
In the investigation of total phenolic acid levels, a stock solution of gallic acid was prepared in methanol at a concentration of 2 mg/mL. A series of dilutions were prepared from the stock solution to achieve concentrations of 1,000, 750, 500, 375, 250, 187.5, and 125 μg/mL. Additionally, a Chrysanthemum extract was prepared in methanol at a concentration of 10 mg/mL. Folin–Ciocalteu method was used to quantify the total phenolic content, succeeding the procedure described by Kamtekar et al. (31). Briefly, 10 μL of either the sample or standard solution was mixed with 100 μL of Folin–Ciocalteu reagent (1:10) in a 96-well microplate. After mixing, 80 μL of 1 M Na2CO3 was added to the mixture and incubated at 25°C for 20 min in the dark. The resulting blue color complex was quantified at 630 nm using a spectrophotometer. The collected data were analyzed and presented as means ± SD. We used a FluoStar Omega microplate reader to record the results.
For this purpose, the stock solution of standard rutin was prepared in methanol (CH3OH) at a concentration of 2,000 μg/mL. A series of dilutions were then made from this stock solution to achieve concentrations of 1,000, 500, 250, 125, 62.5, 31.25, and 15.625 μg/mL. Furthermore, the Chrysanthemum extract was prepared in CH3OH at a concentration of 10 mg/mL. The total flavonoid content was evaluated using the AlCl3 colorimetric assay as mentioned by Nurcholis et al. (32). For the assay, 15 μL aliquots of both the sample and standard rutin solutions were dispensed into a 96-well microplate. Then, 175 μL of CH3OH, 30 μL of 1.25% AlCl3, and 30 μL of 0.125 M CH3COONa were sequentially added. The mix was then incubated at room temperature for 5 min, the yellow color mix was measured at 420 nm using a UV/VIS spectrophotometer. The data are presented as means ± standard deviation (SD). The results were recorded using a FluoStar Omega microplate reader.
Neem oil was obtained from Centa Kind Pharmaceuticals (RC# 7797, Nefertari, Limited Co., El-Fayoum, Egypt). This oil was derived from the seeds of the neem tree (Azadirachta indica, family: Meliaceae) and is known for its high purity level of over 98%. Commercial formulations often contain standardized concentrations of active ingredients, such as azadirachtin, which enhances their effectiveness. This standardization ensures that the product delivers reliable results, making it preferable for therapeutic applications. To prepare the emulsion, the neem oil was combined with the non-ionic surfactants (soap) in a 1:3 ratio. The process began by mixing deionized water and surfactants using a stirrer, followed by the addition of neem oil. Neem oil concentrations of 10, 20, and 25% were prepared according to Gareh et al. (33).
The in vitro adult immersion test (AIT) technique was used to evaluate acaricides sensitivity assay and water with four replications as described by FAO (34). Briefly, in sterile petri dishes, three concentrations were prepared from Chrysanthemum extract (0.5, 0.25, 0.125 mg/mL) and neem oil (20, 15, 10 mg/L). Phoxim (1 mL/L) was used as a positive control, deionized water, and Tween 80% (2:1) were used as the control negative group. Ten moderately engorged females (nearly the same size) were placed in 3 mL of each concentration from each treatment for 5 min. In sterile 6-well plates with filter paper, three replicates for each concentration were used to incubate the treated ticks at 28°C with a relative humidity of (80 ± 5%). Later, ticks were observed at various time intervals (3, 6, 12, 24, 48, and 72 h). Viability checks were regularly performed, if a tick did not respond to needle stimulation, it was considered dead. The mortality rate was calculated using the equation below:
R. annulatus adult ticks from the treated groups with neem oil (20 mg/L), Chrysanthemum (5 mg/L), phoxim (1 mL/L), and control negative were selected and preserved in 2.5% glutaraldehyde solution within phosphate-buffered saline at pH 7.4 for a minimum of 2 hours. The samples were prepared as previously described (35, 36). The samples were subsequently mounted on double-sided carbon adhesive tape, gold-coated, and analyzed using a scanning electron microscope (Joel, JSM-5400LV, Tokyo 1993, Japan) at Assiut University’s Electron Microscopy Unit to assess the surface morphological alterations (37).
The chi-square test was utilized to analyze the prevalence and associated risk factors concerning the sex and age of the studied animals. The in vitro investigation results were processed utilizing SPSS software (version 20). Data are presented as mean ± standard deviation (SD), and differences between experimental groups were evaluated using a one-way ANOVA, with a significance threshold of p < 0.05 (38). For multiple group comparisons, post hoc analysis was performed, specifically utilizing the LSD and Duncan tests.
Out of 130 cattle examined, 61 (46.9%) were found to be infested with ticks. Analysis of thin blood films from these infested animals revealed an infection rate of 81.9% for blood piroplasm. The identified piroplasm infections included two species: Theileria and Babesia. As shown in Table 1, the chi-square test for Theileria infection showed a value of 3.6885 with a p = 0.05479, which is marginally significant, suggesting a potential association between Theileria infection and tick infestation. In contrast, the chi-square test for Babesia infection revealed a highly significant chi-square value of 22.443 with a p = 0.00002, indicating a strong association between Babesia infection and tick infestation.
The PCR method was utilized to amplify the Theileria annulata tams1 gene, resulting in the identification of 721 bp bands observed under UV light on agarose gel (1.5%) (see Figure 1A). Sequencing of the resulted PCR product confirmed the affiliation of our sample with Theileria annulata. The sequence has been deposited in GenBank under the accession number OR987834. The phylogenetic relationship between the Tams1 nucleotide sequences of our isolate and 14 reference isolates of Theileria annulata is illustrated in Figure 2. This analysis provides insights into the genetic diversity and geographical distribution of the detected pathogen. The well-supported clade structure indicates that geographical factors significantly influence genetic variation among the isolates. Notably, there is a distinct clustering of isolates from Scotland, India, the Netherlands, Pakistan, and Egypt, highlighting the impact of geographical origins on the genetic makeup of these parasites. There is a robust subgroup, including the Egyptian isolate (OR987834.1) and the isolate from the Netherlands (AF214848.1).
Figure 1. Agarose (1.5%) gel showing (A) PCR products (721 bp) of amplified Theileria annulata samples. (B) PCR products (340 bp) of amplified Babesia bigemina. Lane (M) is the DNA size marker, lane (P) is the positive control, lane (N) is the negative control, and lane (1) is the positive sample.
Figure 2. The phylogenetic tree generated from tams1 gene sequences, revealing evident clades that define distinct genetic lineages within Theileria annulata.
The PCR method was used to amplify Babesia 18S rRNA, resulting in the detection of 340 bp bands visible under UV light on agarose gel 1.5% (Figure 1B). Sequencing of the resulted PCR product confirmed that our sample is affiliated with Babesia bigemina. The corresponding sequence has been cataloged in GenBank under the accession number OR965914. The phylogenetic relationship between our isolate (18S rRNA nucleotide sequences) and 17 reference strains of Babesia species is shown in Figure 3. The well-supported clade includes the newly identified Babesia bigemina isolates from Egypt (OR965914.1) and the isolates from Turkey (HQ197740.1 and EF446164.1). In addition, the distinct subgroup formed by the B. bigemina Colombian isolates (MH194393.1 and MH194392.1) shows regional genetic diversity within this species. The phylogenetic tree further shows distinct differences between various Babesia species. Babesia ovata and Babesia major form a separate clade, while Babesia crassa and Babesia bovis group together. There is strong support for the clade containing Babesia orientalis and Babesia occultans isolates from China.
Figure 3. The phylogenetic tree constructed from the 18S rRNA gene sequences, unveils clear clades that delineate distinct genetic lineages of Babesia bigemina.
By light microscope, the tick hemolymph appeared to contain three main groups of cells (hemocytes), which can be classified into (prohemocytes, plasmatocytes, and spherulocytes) based on their structural characteristics. Prohemocytes are small and rounded with a high nuclear-cytoplasmic ratio and deeply basophilic cytoplasm. They have a round, single nucleus with condensed homogeneous chromatin. Plasmatocytes are round or ovoid, with a single nucleus. The chromatin is punctate or granular, and the nucleus is usually eccentric in position. Finally, spherulocytes are frequently large, ovoid, or round cells with cytoplasm containing characteristic purple staining clusters of spherules. These spherules are so dense that the cytoplasm and the nucleus are obscured. The infected hemolymph contains ookinetes of piroplasms, which are banana-shaped with curved or semi-curved tails. These ookinetes have an anteriorly positioned nucleus, typically located in the middle of the cell (Figure 4).
Figure 4. Photomicrograph showing different morphological characterization of hemolymph cells and piroplasm ookinetes in the infected samples. (A) Prohaemocyte (arrow). (B) Plasmatocytes (arrow). (C) Spherule (arrow). (D) Infected hemolymph containing piroplasm ookinetes (banana-shaped) with curved or semi-curved tails (arrow).
The analysis of the total phenolic content within the hydro-methanolic extract was accomplished by referencing the gallic acid calibration curve (Figure 5A). The analysis revealed a substantial presence of phenolic compounds, with a measured content of 20.204 ± 1.75 μg gallic acid equivalent (GAE) per 1 mg of dry extract.
Figure 5. (A) Standard calibration curve showing absorbance versus gallic acid concentration for determining the total phenolic content of Chrysanthemum extract. (B) Standard calibration curve showing absorbance versus rutin concentration for determining the total phenolic content of Chrysanthemum extract.
The assessment of total flavonoid content in Dendranthema grandiflora aerial parts extract revealed noteworthy findings. Using the curve rutin calibration curve (Figure 5B), the flavonoid level was quantified, showcasing a significant flavonoid content of 34.25 ± 2.55 μg rutin equivalent (RE) per 1 mg of dry extract.
Chrysanthemum extract was tested at 0.5 mg/mL, 0.25 mg/mL, and 0.125 mg/mL. The acaricidal effect was increased over time, with concentrations of 0.5 mg/mL and 0.25 mg/mL showing the most pronounced effects. These concentrations induced tick mortality within the first 3 h of application, with mortality percentages of 73.3 and 60%, respectively, reaching complete mortality after 24 h. Phoxim caused mortality in all ticks within 12 h. Significant differences were observed between all concentrations of Chrysanthemum extract and the control group (p < 0.001). No significant differences were observed between different concentrations at various time points as shown in Table 2.
Neem oil was evaluated at concentrations of 20 mg/L, 15 mg/L, and 10 mg/L. Significant differences were observed between the high and low concentrations of neem oil. The mortality rate increased over time, with concentrations of 20 mg/L and 15 mg/L exhibiting the most significant effects. These concentrations induced noticeable changes and caused tick mortality within the first 3 h of application, with mortality rates of 60%. Complete mortality was achieved after 24 h. Conversely, the minimal effect was observed in the lower concentration10 mg/L, resulted in only 13.3% mortality rate within the first 3 h, and achieved complete mortality after 72 h of treatment. Significant differences were observed between all concentrations of neem oil and the control group (p < 0.001). At various time points, significant differences were also noted between the high concentrations (20 mg/L and 15 mg/L) and the low concentrations (10 mg/L) as shown in Table 3.
The scatter plot shows the relationship between Chrysanthemum extract (0.5 mg/mL) and phoxim mortality rate (Figure 6A), the correlation coefficient is 0.67, which indicates a moderate positive linear relationship between Chrysanthemum extract and phoxim mortality rate, suggesting that higher mortality rates due to Chrysanthemum are associated with higher mortality rates due to phoxim. The correlation coefficient is 0.91 between neem oil (20 mg/L) and phoxim mortality rate, indicating a very strong positive linear relationship. As the mortality rate due to neem increases, the mortality rate due to phoxim also tends to increase. The plot contains data points representing pairs of mortality rates for neem and phoxim, most of which lie close to the fitted linear regression line, showing the data trend. Some data points lie further from the line and maybe outliers (Figure 6B). The scatter plot also demonstrates a strong positive correlation (r = 0.8) between neem (20 mg/L) and Chrysanthemum (0.5 mg/mL) treatments, indicating that as neem’s efficacy increases, Chrysanthemum’s mortality rate also rises as shown in Figure 6C.
Figure 6. (A) Scatter plot illustrating the relationship between Chrysanthemum extract 0.5 mg/mL mortality and phoxim mortality with a moderate positive correlation (r = 0.67). (B) Scatter plot showing the relationship between neem oil 20 mg/L mortality and phoxim mortality with a strong positive correlation (r = 0.91). (C) Scatter plot showing the relationship between neem oil 20 mg/L mortality and Chrysanthemum extract 0.5 mg/mL mortality with a positive correlation (r = 0.8). The blue line represents the best-fit linear regression line, and the shaded gray area indicates the confidence interval.
SEM was conducted to observe surface changes in various parts of adult female R. annulatus specifically the capitulum, dorsal surface, and ventral surface following treatments with neem oil (20 mg/L), Chrysanthemum extract (0.5 mg/mL), and phoxim (1 mL/L). In the control negative group, the capitulum maintained its intact structure (Figure 7A). In contrast, the phoxim-treated group showed complete degeneration of the capitulum (Figure 7B). Neem oil treatment caused significant damage, destroying both the hypostome and the capitulum (Figures 7C,D). Similarly, Chrysanthemum extract caused severe damage, with complete degeneration of the mouthparts and the capitulum becoming obscured (Figures 7E,F). Upon examining the dorsal surface, the control group (Figure 8A) exhibited an intact anterior region. In contrast, the phoxim-treated group (Figure 8B) revealed numerous cracks in the cuticle. The neem oil treatment resulted in cracking of the cuticle (Figures 8C,D). However, Chrysanthemum extract caused more severe damage, featuring visible pores and significant cracks in the cuticle (Figures 8E,F). On the ventral surface around the anal region, the control group exhibited a normal appearance (Figure 9A), while the phoxim-treated group showed a retracted anal region (Figure 9B). However, treatment with neem oil resulted in notable changes, including retraction of the anal region, destruction accompanied by cracks and typical distribution of striations in some area with pores (Figures 9C,D), In contrast, Chrysanthemum extract caused wrinkling of the cuticle, leading to the formation of pores or holes (Figure 9E) and a disrupted striation pattern, along with slight degeneration of the anal region (Figure 9F).
Figure 7. Acaricidal effects of the different groups on capitulum of adult female R. annulatus ticks. (A) Control group showing intact capitulum structure. (B) Phoxim-treated group with complete capitulum degeneration. (C) Neem oil-treated group displaying complete hypostome destruction. (D) Neem oil-treated group with damaged capitulum. (E) Chrysanthemum extract-treated group showing severe mouthpart damage. (F) Chrysanthemum extract-treated group with hidden capitulum.
Figure 8. Acaricidal effects of the different groups on dorsal surface of adult female R. annulatus ticks. (A) Control group with intact anterior part. (B) Phoxim-treated group displaying cuticle cracks. (C,D) Neem oil-treated group showing cuticle cracking. (E) Chrysanthemum extract-treated group showing pores in the cuticle. (F) Chrysanthemum extract-treated group with cuticle cracks.
Figure 9. Acaricidal effects of the different groups on the ventral surface of adult female R. annulatus ticks. (A) Control group maintaining normal appearance. (B) Phoxim-treated group with retracted anal region. (C) Neem oil-treated group showing the typical distribution of striations with some pores. (D) Neem oil-treated group showing destruction and cracks around the anal region. (E) Chrysanthemum extract-treated group with pore formation. (F) Chrysanthemum extract-treated group with wrinkling and slight degeneration of the anal region.
This study investigated the prevalence of tick-borne piroplasms infection (Babesia and Theileria) in cattle and explored the efficacy of Chrysanthemum extract and neem oil as potential natural acaricides. Our results showed significant associations between tick infestation and piroplasm infections, with Theileria infections being more prevalent (62.3%) than Babesia infections (19.7%). These findings align with previous studies in Egypt, where Babesia and Theileria infections were reported in 11.16 and 10.25% of cattle, respectively, with B. bigemina and T. annulata being the most common species (39). However, high prevalence of Theileria than Babesia infection were reported by Prado et al. (40) and Hossain et al. (41), whereas El-Dakhly et al. (42) reported that prevalence of Theileria infection was (9.31%) in cattle in El-Wadi El-Gadid province. Additionally, the prevalence of Theileria infection was 8.3% in Sudan (43) and Bangladesh (41). In our study, Theileria sp. has a higher prevalence than Babesia sp. due to a variety of factors, including the availability of suitable tick vectors, reservoirs, and amplification hosts. These factors all contribute to the spread and persistence of Theileria in each ecosystem (44), which lower than those reported by El-Metenawy (45) in Saudi Arabia (76.5%) and Al-Emarah et al. (46) in Iraq (69.43%). This study contributes valuable insights into the dynamics of tick-borne diseases and enhances our understanding of their epidemiology (47).
To identify piroplasm species, the Tams1 gene for Theileria and the 18S rRNA gene for Babesia were amplified and sequenced. Phylogenetic analysis revealed genetic diversity within T. annulata and B. bigemina isolates, suggesting a need for region-specific control measures. Phylogenetic analysis of T. annulata revealed significant genetic diversity influenced by geography, with isolates from different regions such as Scotland, India, the Netherlands, Pakistan, and Egypt forming distinct clusters. There are multiple Egyptian isolates in different clades highlighting high genetic variability within the local population aligning with another study in upper Egypt that estimated the presence of T. annulata infection in cattle (48). On the other hand, the phylogenetic tree of B. bigemina showed a close genetic relationship between the Egyptian isolate and those from Turkey. This indicates regional genetic diversity, with distinct clades for various Babesia species and clear evolutionary relationships observed.
In this study, morphological characteristics of piroplasm ookinetes were examined in tick hemolymph. The presence of ookinetes, crucial for active infections, was confirmed using their distinct banana shape, curved tails, and central nucleus. These results align with findings from Martínez-García et al. (49), and are key to recognizing piroplasm ookinetes, which play a crucial role in active infections (49). Our results contribute to the broader understanding of piroplasm morphology and the role of ticks as vectors of hemoprotozoan parasites, offering valuable insights into infection dynamics.
Integrating botanical insecticides into tick management programs can contribute to a holistic approach to animal health, addressing ectoparasite control and overall well-being. Given the growing resistance of ticks to commercial acaricides, this study evaluated Chrysanthemum extract and neem oil as potential natural eco-friendly alternatives. Our results demonstrate that both extracts had significant acaricidal activity against ticks. Chrysanthemum extract, particularly at high concentrations (0.5 mg/mL), showed high efficacy in inducing tick mortality, resulting in 73.3% mortality within the first 3 h, reaching complete mortality at 24 h. Our findings aligned with a study that demonstrated that the effects of Chrysanthemum roseum extracts achieved 100% mortality against Rhipicephalus microplus at a 5% concentration (50). In this study, the statistical analysis showed a moderate correlation between Chrysanthemum extract (0.5 mg/mL) and phoxim mortality rates with significant differences between all concentrations of Chrysanthemum extract and the control group. Organic repellent utilizing Chrysanthemum oil on 5 mL, resulted in the killing of insects such as ants, cockroaches, and flies after 2.81 min (51). Notably, pyrethrins, derived from Pyrethrum, laid the foundation for the synthesis of more potent synthetic pyrethroids. The insecticidal activity of Pyrethrum, with its rapid biodegradation and its relatively low mammalian toxicity, makes it one of the most widely used non-synthetic insecticides in certified organic agriculture (17). In the present study, the Chrysanthemum extract has abundant phenolic and flavonoid contents, which contribute to its potential bioactivity and therapeutic value. In addition, the high flavonoid content in the Chrysanthemum extract suggests potential therapeutic value and health benefits. Phenolic compounds are well-known for their antioxidant properties, anti-inflammatory, antimicrobial, and anti-carcinogenic activities (52–54). Flavonoids are renowned for their diverse pharmacological effects, including antioxidant and anti-inflammatory properties, and anti-cancer effects, among others (55).
Neem oil, at concentrations of 20, 15, and 10 mg/L, exhibited significant acaricidal effects. The highest concentration (20 mg/L) resulted in 60% mortality within the first 3 h and reaching complete mortality after 24 h. Significant differences were observed between all concentrations of neem oil and the control group, as well as between higher and lower concentrations of neem oil at different time points. These results are consistent with the study by Gareh et al. (33), which revealed that 100% mortality of adult ticks on the 1st day post-treatment. The correlation analysis between the high concentration of neem oil (20 mg/L) and phoxim mortality rates showed a strong positive linear relationship, while the mortality rate due to neem oil increased, the phoxim mortality rate also increased. This indicates that Chrysanthemum and neem oil could be a powerful alternative to phoxim, as it has also shown insecticidal effects on numerous insect species, such as mosquitoes (56).
SEM results confirmed that all treatments (Chrysanthemum extract, neem oil, and phoxim) caused notable damage to the tick cuticle, including wrinkling, pores, loss of striation, and cracks in some areas. The destruction of the hypostome was particularly evident when neem oil was used. This indicates that neem oil has the potential to interfere with the survival and reproduction of ticks. This effect may be due to the active components of neem, salannin, which have insect growth-regulating and antifeedant activity (33). The destruction of mouthparts with neem oil is similar to the effects noted with Melia azedarach and Artemisia herba-alba extracts on Hyalomma dromedarii (57). On the other hand, Chrysanthemum extract induced pronounced damage to the tick cuticle, marked by cracks and pores. Phoxim was effective in causing complete degeneration of the capitulum and extensive cuticle cracks. Also, cracks resulting from Chrysanthemum and phoxim were similar to those caused by Cymbopogon citratus oil on Haemaphysalis longicornis ticks (58). In the neem oil-treated group, the ventral surface and anal region of ticks showed retraction and destruction. In contrast, Chrysanthemum extract caused loss of striations and wrinkling with pore formation. These findings suggest that Chrysanthemum extract and neem oil demonstrate effective acaricidal effects similar to phoxim, confirming their potential as eco-friendly alternatives in integrated pest management strategies.
Our research highlights the strong connection between tick infestations and piroplasm infection in cattle, specifically Babesia species. Molecular identification confirmed the presence of T. annulata and B. bigemina. Phylogenetic analyses revealed significant genetic diversity influenced by geographical factors. Chrysanthemum extract, as well as neem oil, demonstrated high acaricidal efficacy, resulting in substantial mortality rates in R. annulatus ticks. Both treatments showed time-and dose-dependent effectiveness, causing severe morphological damage to the ticks, as demonstrated by SEM analysis. Overall, the findings support the use of Chrysanthemum extract and neem oil as effective natural alternatives for tick control, providing a sustainable solution for livestock health management.
The datasets presented in this study can be found in online repositories. The names of the repository/repositories and accession number(s) can be found in the article/supplementary material.
The animal study was approved by Research Ethical Committee, Faculty of Veterinary Medicine at Assiut University (Approval number: 06/2024/0257). The study was conducted in accordance with the local legislation and institutional requirements.
SA-E: Conceptualization, Data curation, Formal analysis, Investigation, Methodology, Validation, Writing – original draft, Writing – review & editing. FK: Data curation, Formal analysis, Investigation, Methodology, Writing – review & editing. SA-H: Data curation, Formal analysis, Validation, Writing – review & editing. AK: Data curation, Investigation, Validation, Writing – original draft. SM: Methodology, Writing – review & editing. AAb: Investigation, Methodology, Writing – review & editing. MD: Data curation, Validation, Writing – review & editing. AA-H: Funding acquisition, Validation, Writing – original draft. AAl: Funding acquisition, Investigation, Writing – review & editing. AD: Conceptualization, Data curation, Writing – review & editing.
The author(s) declare that financial support was received for the research and/or publication of this article. The authors extend their appreciation to the Deanship of Research and Graduate Studies at King Khalid University, KSA, for funding this work through Small Research Group under Grant Number (RGP1/343/45). Small Research Group, the Deanship of Research and Graduate Studies.
The authors declare that the research was conducted in the absence of any commercial or financial relationships that could be construed as a potential conflict of interest.
The authors declare that no Gen AI was used in the creation of this manuscript.
All claims expressed in this article are solely those of the authors and do not necessarily represent those of their affiliated organizations, or those of the publisher, the editors and the reviewers. Any product that may be evaluated in this article, or claim that may be made by its manufacturer, is not guaranteed or endorsed by the publisher.
1. Pereira, DFS, Ribeiro, HS, Gonçalves, AAM, da Silva, AV, Lair, DF, de Oliveira, DS, et al. Rhipicephalus microplus: an overview of vaccine antigens against the cattle tick. Ticks Tick Borne Dis. (2022) 13:101828. doi: 10.1016/j.ttbdis.2021.101828
2. Magesa, WS, Haji, I, Kinimi, E, Nzalawahe, JS, and Kazwala, R. Distribution and molecular identification of ixodid ticks infesting cattle in Kilombero and Iringa Districts, Tanzania. BMC Vet Res. (2023) 19:121. doi: 10.1186/s12917-023-03652-x
3. Roome, A, Gouli, S, Yodsuwan, R, Victory, J, Collins, C, Jenkins, P, et al. Tick magnets: the occupational risk of tick-borne disease exposure in forestry workers in New York. Health Sci Rep. (2022) 5:e509. doi: 10.1002/hsr2.509
4. Satti, RA, Awadelkareem, EA, Suganuma, K, Salim, B, Inoue, N, Xuan, X, et al. Cattle anaplasmosis and babesiosis: major tick-borne diseases affecting the cattle industry in Khartoum State, Sudan. Vet Parasitol Reg Stud Reports. (2021) 26:100632. doi: 10.1016/j.vprsr.2021.100632
5. Stuen, S. Haemoparasites-challenging and wasting infections in small ruminants: a review. Animals. (2020) 10:2179. doi: 10.3390/ani10112179
6. Selim, A, Weir, W, and Khater, H. Prevalence and risk factors associated with tropical theileriosis in Egyptian dairy cattle. Vet World. (2022) 15:919–24. doi: 10.14202/vetworld.2022.919-924
7. Azhar, M, Gadahi, JA, Bhutto, B, Tunio, S, Vistro, WA, Tunio, H, et al. Babesiosis: current status and future perspectives in Pakistan and chemotherapy used in livestock and pet animals. Heliyon. (2023) 9:e17172. doi: 10.1016/j.heliyon.2023.e17172
8. Makwarela, TG, Nyangiwe, N, Masebe, T, Mbizeni, S, Nesengani, LT, Djikeng, A, et al. Tick diversity and distribution of hard (Ixodidae) cattle ticks in South Africa. Microbiol Res. (2023) 14:42. doi: 10.3390/microbiolres14010004
9. Tayebwa, DS, Vudriko, P, Tuvshintulga, B, Guswanto, A, Nugraha, AB, Gantuya, S, et al. Molecular epidemiology of Babesia species, Theileria parva, and Anaplasma marginale infecting cattle and the tick control malpractices in Central and Eastern Uganda. Ticks Tick Borne Dis. (2018) 9:1475–83. doi: 10.1016/j.ttbdis.2018.06.012
10. Rodriguez-Vivas, RI, Jonsson, NN, and Bhushan, C. Strategies for the control of Rhipicephalus microplus ticks in a world of conventional acaricide and macrocyclic lactone resistance. Parasitol Res. (2018) 117:3–29. doi: 10.1007/s00436-017-5677-6
11. Tiffin, HS, Rajotte, EG, Sakamoto, JM, and Machtinger, ET. Tick control in a connected world: challenges, solutions, and public policy from a United States border perspective. Trop Med Infect Dis. (2022) 7:388. doi: 10.3390/tropicalmed7110388
12. Bhowmick, B, and Han, Q. Understanding tick biology and its implications in anti-tick and transmission blocking vaccines against tick-borne pathogens. Front Vet Sci. (2020) 7:319. doi: 10.3389/fvets.2020.00319
13. Salman, M, Abbas, RZ, Israr, M, Abbas, A, Mehmood, K, Khan, MK, et al. Repellent and acaricidal activity of essential oils and their components against Rhipicephalus ticks in cattle. Vet Parasitol. (2020) 283:109178. doi: 10.1016/j.vetpar.2020.109178
14. Showler, AT, and Saelao, P. Integrative alternative tactics for ixodid control. Insects. (2022) 13:302. doi: 10.3390/insects13030302
15. Farag, MR, Alagawany, M, Bilal, RM, Gewida, AGA, Dhama, K, Abdel-Latif, HMR, et al. An overview on the potential hazards of pyrethroid insecticides in fish, with special emphasis on cypermethrin toxicity. Animals. (2021) 11:1880. doi: 10.3390/ani11071880
16. Aznar-Alemany, Ò, and Eljarrat, E. Introduction to pyrethroid insecticides: chemical structures, properties, mode of action and use In: E Eljarrat, editor. Pyrethroid insecticides. The handbook of environmental chemistry. Cham: Springer (2020). 1–16.
17. Hodoșan, C, Gîrd, CE, Ghica, MV, Dinu-Pîrvu, C-E, Nistor, L, Bărbuică, IS, et al. Pyrethrins and pyrethroids: a comprehensive review of natural occurring compounds and their synthetic derivatives. Plants. (2023) 12:4022. doi: 10.3390/plants12234022
18. Sarkar, S, Singh, RP, and Bhattacharya, G. Exploring the role of Azadirachta indica (neem) and its active compounds in the regulation of biological pathways: an update on molecular approach. 3 Biotech. (2021) 11:178. doi: 10.1007/s13205-021-02745-4
19. Denardi, SE, Bechara, GH, Oliveira, PRD, and Camargo-Mathias, MI. Azadirachta indica a. Juss (neem) induced morphological changes on oocytes of Rhipicephalus sanguineus (Latreille, 1806) (Acari: Ixodidae) tick females. Exp Parasitol. (2010) 126:462–70. doi: 10.1016/j.exppara.2010.05.017
20. Chaudhary, S, Kanwar, RK, Sehgal, A, Cahill, DM, Barrow, CJ, Sehgal, R, et al. Progress on Azadirachta indica based biopesticides in replacing synthetic toxic pesticides. Front Plant Sci. (2017) 8:610. doi: 10.3389/fpls.2017.00610
21. Galli, M, Feldmann, F, Vogler, UK, and Kogel, K-H. Can biocontrol be the game-changer in integrated pest management? A review of definitions, methods and strategies. J Plant Dis Prot. (2024) 131:265–91. doi: 10.1007/s41348-024-00878-1
22. Kamel, FA, Dyab, AK, Khedr, AA, and Abd-Elrahman, SM. Epidemiological and clinical manifestations of blood parasitic infections in cattle in Assiut Governorate Egypt. Assiut Vet Med J. (2024) 70:8–16. doi: 10.21608/avmj.2024.246898.1203
23. Paliwal, S, Shanker, D, Sudan, V, Kumar, S, Srivastava, M, and Gupta, KK. Comparison of different PCR protocols and respective primer sets from pool of TAMS 1 gene for diagnosis of calf theileriosis from semi arid India. Biologicals. (2019) 57:50–4. doi: 10.1016/j.biologicals.2018.12.004
24. Ahmed, AS, Ahmed, AE, Mohamed, AE-MM, and Ali, AOA. Clinical and laboratory diagnosis of some blood parasites in dairy cows in Qena Governorate. SVU Int J Vet Sci. (2022) 5:68–82. doi: 10.21608/svu.2022.90304.1140
25. Abdel-Hakeem, SS, Fadladdin, YA, El-Sagheer, AM, and Adel, A. New host record, Sclerophrys regularis (Bufonidae), for Rhabdias africanus (Rhabdiasidae, Kuzmin, 2001) based on molecular and morphological evidence. Saudi Journal of Biological Sciences. (2022) 29:103366. doi: 10.1016/j.sjbs.2022.103366
26. Yousef, AM, Abdel-Radi, S, Dyab, AK, Khedr, AA, and Abd-Elrahman, SM. A study on ectoparasites infesting domestic cats in Giza Governorate, Egypt. Assiut Vet Med J. (2024) 70:192–207. doi: 10.21608/avmj.2024.198094.1235
27. Hassan, FA, Tolba, M, Abed, GH, Omar, H, and Abdel-Hakeem, SS. Contact lenses contamination by Acanthamoeba spp. in Upper Egypt. PLoS One. (2021) 16:e0259847. doi: 10.1371/journal.pone.0259847
28. Abd-Elrahman, SM, Dyab, AK, Kamel, FA, Khedr, AA, Khalifa, MM, Mohamed, SM, et al. Assessment of cattle tick infestation: molecular insights into Rhipicephalus annulatus and the efficacy of garlic oil and nanoemulsion as acaricidal agents. Vet Parasitol. (2024) 329:110211. doi: 10.1016/j.vetpar.2024.110211
29. Allam, NAT, El Moghazy, FM, and Abdel-Baky, SMM. Molecular epidemiological updates on spotted fever rickettsioses in animal species and their hard ticks settling Egyptian desert. J Adv Pharm Educ Res. (2018) 8:64–74.
30. Nong, X, Ren, Y-J, Wang, J-H, Fang, C-L, Xie, Y, Yang, D-Y, et al. Clinical efficacy of botanical extracts from Eupatorium adenophorum against the scab mite, Psoroptes cuniculi. Vet Parasitol. (2013) 192:247–52. doi: 10.1016/j.vetpar.2012.10.005
31. Kamtekar, S, Keer, V, and Patil, V. Estimation of phenolic content, flavonoid content, antioxidant and alpha amylase inhibitory activity of marketed polyherbal formulation. J Appl Pharm Sci. (2014) 4:061–5. doi: 10.7324/JAPS.2014.40911
32. Nurcholis, W, Sya'bani Putri, DN, Husnawati, H, Aisyah, SI, and Priosoeryanto, BP. Total flavonoid content and antioxidant activity of ethanol and ethyl acetate extracts from accessions of Amomum compactum fruits. Ann Agric Sci. (2021) 66:58–62. doi: 10.1016/j.aoas.2021.04.001
33. Gareh, A, Hassan, D, Essa, A, Kotb, S, Karmi, M, Mohamed, AEH, et al. Acaricidal properties of four neem seed extracts (Azadirachta indica) on the camel tick Hyalomma dromedarii (Acari: Ixodidae). Front Vet Sci. (2022) 9:946702. doi: 10.3389/fvets.2022.946702
34. FAO. Resistance management and integrated parasites control in ruminants-guidelines In: Module 1: ticks: acaricide resistance, diagnosis, management and prevention. Rome: Food and Agriculture Organization, Animal Production and Health Division (2004)
35. Abd-Elrahman, SM, Dyab, AK, Mahmoud, AE, Mostafa, SM, and Elossily, NA. Antiparasitic activity of myrrh crude extract and myrrh volatile oil compared to albendazole against Trichinella spiralis muscular larvae in vitro. J Egypt Soc Parasitol. (2020) 50:307–14. doi: 10.21608/jesp.2020.113052
36. Abdel-Zaher, M, Abed, G, and Abdel-Hakeem, S. Ultrastructural changes of Schistosoma mansoni worms associated with administration of its polyvalent vaccine. J Zool Stud. (2016) 3:09–20.
37. Abd-Elrahman, SM, Dyab, AK, Mahmoud, AE, Alsharif, FM, Mohamed, SM, Abomughaid, MM, et al. Influence of chemically and biosynthesized silver nanoparticles on in vitro viability and infectivity of Trichinella spiralis muscle larvae. Ann Parasitol. (2021) 67:591–602. doi: 10.17420/ap6704.375
38. Abdel-Hakeem, SS, Abdel-Samiee, MA, Youssef, MSE, Abd-Elsadek, SH, Abd-Elrahman, SM, and Abdel-Hakeem, SS. Nanocurcumin: a promising therapeutic candidate for experimental Trichinellosis. Microsc Microanal. (2024) 30:368–81. doi: 10.1093/micmic/ozae007
39. Rizk, MA, Salama, A, El-Sayed, SA-E-S, Elsify, A, El-ashkar, M, Ibrahim, H, et al. Animal level risk factors associated with Babesia and Theileria infections in cattle in Egypt. Acta Parasitol. (2017) 62:796–804. doi: 10.1515/ap-2017-0096
40. Prado, ICB, Capuno, LXB Jr, Collera, PD, Cabralda, APD, De Ramos, KAS, Bernardo, JMG, et al. Molecular detection and characterization of Babesia and Theileria in cattle and water buffaloes from southern Luzon, Philippines. Microorganisms. (2022) 10:678. doi: 10.3390/microorganisms10040678
41. Hossain, MJ, Raut, S, Singh, RP, Mishra, P, Hossain, MS, Dey, AR, et al. Molecular detection of Babesia and Theileria from crossbred cattle in Sirajganj and Rangpur districts of Bangladesh. Vet Med Sci. (2023) 9:899–906. doi: 10.1002/vms3.989
42. El-Dakhly, KM, Arafa, W, Ghanem, S, Abdel-Fatah, O, and Wahba, A. Microscopic and molecular detection of Theileria annulata infection of cattle in Egypt. J Adv Parasitol. (2018) 5:29–34. doi: 10.17582/journal.jap/2018/5.2.29.34
43. Abaker, IA, Salih, DA, El Haj, LM, Ahmed, RE, Osman, MM, and Ali, AM. Prevalence of Theileria annulata in dairy cattle in Nyala, South Darfur State, Sudan. Vet World. (2017) 10:1475–80. doi: 10.14202/vetworld.2017.1475-1480
44. Remesar, S, Díaz, P, Prieto, A, Markina, F, Cao, JMD, López-Lorenzo, G, et al. Prevalence and distribution of Babesia and Theileria species in roe deer from Spain. Int J Parasitol Parasites Wildl. (2019) 9:195–201. doi: 10.1016/j.ijppaw.2019.05.005
45. El-Metenawy, T. Prevalence of blood parasites among cattle at the central area of Saudi Arabia. Vet Parasitol. (2000) 10:6–13.
46. Al-Emarah, GY, Khudor, M, and Daham, H. Clinical, haematological and biochemical study to cattle naturally infected with Theileria annulata in north of Basrah province. Al-Qadisiyah J Vet Med Sci. (2012) 11:54. doi: 10.29079/vol11iss1art171
47. Abdel-Shafy, S, Abdullah, H, Elbayoumy, MK, Elsawy, BSM, Hassan, MR, Mahmoud, MS, et al. Molecular epidemiological investigation of piroplasms and anaplasmataceae bacteria in Egyptian domestic animals and associated ticks. Pathogens. (2022) 11. doi: 10.3390/pathogens11101194
48. Al-Hosary, A, Răileanu, C, Tauchmann, O, Fischer, S, Nijhof, AM, and Silaghi, C. Tick species identification and molecular detection of tick-borne pathogens in blood and ticks collected from cattle in Egypt. Ticks Tick Borne Dis. (2021) 12:101676. doi: 10.1016/j.ttbdis.2021.101676
49. Martínez-García, G, Santamaría-Espinosa, RM, Lira-Amaya, JJ, and Figueroa, JV. Challenges in tick-borne pathogen detection: the case for Babesia spp. identification in the tick vector. Pathogens. (2021). 10.
50. Dhabale, AR, Macha, V, Jadhav, ND, Choudhary, SK, Sharma, AK, Gudewar, JG, et al. (2024). In vitro acaricidal properties of extracts from Carica papaya seeds and Chrysanthemum roseum leaves against Rhipicephalus microplus. Research Square. (2024). doi: 10.3390/pathogens10020092
51. Yunos, YFM, Tuah, KH, and Ibrahim, MY. Extraction of chrysanthemum oil to produce organic insect repellent. E3S Web of Conf. 479:224. doi: 10.1051/e3sconf/202447905005
52. Maheshwari, N, and Sharma, MC. Anticancer properties of some selected plant phenolic compounds: future leads for therapeutic development. J Herb Med. (2023) 42:100801. doi: 10.21203/rs.3.rs-4443263/v1
53. Chen, X, Lan, W, and Xie, J. Natural phenolic compounds: antimicrobial properties, antimicrobial mechanisms, and potential utilization in the preservation of aquatic products. Food Chem. (2024) 440:138198. doi: 10.1016/j.foodchem.2023.138198
54. Etiang, P, Musoba, A, Nalumenya, D, Ndekezi, C, Bbira, J, Ochwo, S, et al. Distribution and prevalence of ixodid tick species (Acari: Ixodidae) infesting cattle in Karamoja region of northeastern Uganda. BMC Vet Res. (2024) 20:50. doi: 10.1186/s12917-023-03802-1
55. Okoduwa, SIR, Abdulwaliyu, I, Igiri, BE, Arekemase, SO, Okoduwa, UJ, Itiat, JF, et al. Multi-therapeutic potential of flavonoids as an essential component in nutraceuticals for the treatment and management of human diseases. Phytomed Plus. (2024) 4:100558. doi: 10.1016/j.phyplu.2024.100558
56. Chandramohan, B, Murugan, K, Panneerselvam, C, Madhiyazhagan, P, Chandirasekar, R, Dinesh, D, et al. Characterization and mosquitocidal potential of neem cake-synthesized silver nanoparticles: genotoxicity and impact on predation efficiency of mosquito natural enemies. Parasitol Res. (2016) 115:1015–25. doi: 10.1007/s00436-015-4829-9
57. Abdel-Ghany, HSM, Fahmy, MM, Abuowarda, MM, Abdel-Shafy, S, El-Khateeb, RM, and Hoballah, EM. In vitro acaricidal effect of Melia azedarach and Artemisia herba-alba extracts on Hyalomma dromedarii (Acari: Ixodidae): embryonated eggs and engorged nymphs. J Parasit Dis. (2019) 43:696–710. doi: 10.1007/s12639-019-01149-9
58. Agwunobi, DO, Pei, T, Wang, K, Yu, Z, and Liu, J. Effects of the essential oil from Cymbopogon citratus on mortality and morphology of the tick Haemaphysalis longicornis (Acari: Ixodidae). Exp Appl Acarol. (2020) 81:37–50. doi: 10.1007/s10493-020-00485-3
Keywords: Theileria annulata , Babesia bigemina , Rhipicephalus annulatus , hemolymph, acaricides, scanning electron microscope
Citation: Abd-Elrahman SM, Kamel FA, Abdel-Hakeem SS, Khedr AA, Mohamed SM, Abdelgaber AA, Darwish M, Al-Hakami AM, Alqahtani AJ and Dyab AK (2025) Piroplasm infestations in cattle: exploring tick control using Chrysanthemum extract and neem oil emulsion. Front. Vet. Sci. 12:1543162. doi: 10.3389/fvets.2025.1543162
Received: 10 December 2024; Accepted: 07 March 2025;
Published: 31 March 2025.
Edited by:
Mourad Ben Said, University of Manouba, TunisiaReviewed by:
Elizabeth Salinas Estrella, Instituto Nacional de Investigaciones Forestales, Agrícolas y Pecuarias (INIFAP), MexicoCopyright © 2025 Abd-Elrahman, Kamel, Abdel-Hakeem, Khedr, Mohamed, Abdelgaber, Darwish, Al-Hakami, Alqahtani and Dyab. This is an open-access article distributed under the terms of the Creative Commons Attribution License (CC BY). The use, distribution or reproduction in other forums is permitted, provided the original author(s) and the copyright owner(s) are credited and that the original publication in this journal is cited, in accordance with accepted academic practice. No use, distribution or reproduction is permitted which does not comply with these terms.
*Correspondence: Sara Salah Abdel-Hakeem, c2FyYV9hc3NpdXQ4NkBhdW4uZWR1LmVn
†ORCID: Salwa Mahmoud Abd-Elrahman, https://orcid.org/0000-0002-0319-7713
Sara Salah Abdel-Hakeem, https://orcid.org/0000-0003-1069-5806
Abeer A. Khedr, https://orcid.org/0000-0003-3186-7427
Shaymaa M. Mohamed, https://orcid.org/0000-0001-7768-0890
Ahmed Kamal Dyab, https://orcid.org/0000-0002-4021-7904
Disclaimer: All claims expressed in this article are solely those of the authors and do not necessarily represent those of their affiliated organizations, or those of the publisher, the editors and the reviewers. Any product that may be evaluated in this article or claim that may be made by its manufacturer is not guaranteed or endorsed by the publisher.
Research integrity at Frontiers
Learn more about the work of our research integrity team to safeguard the quality of each article we publish.