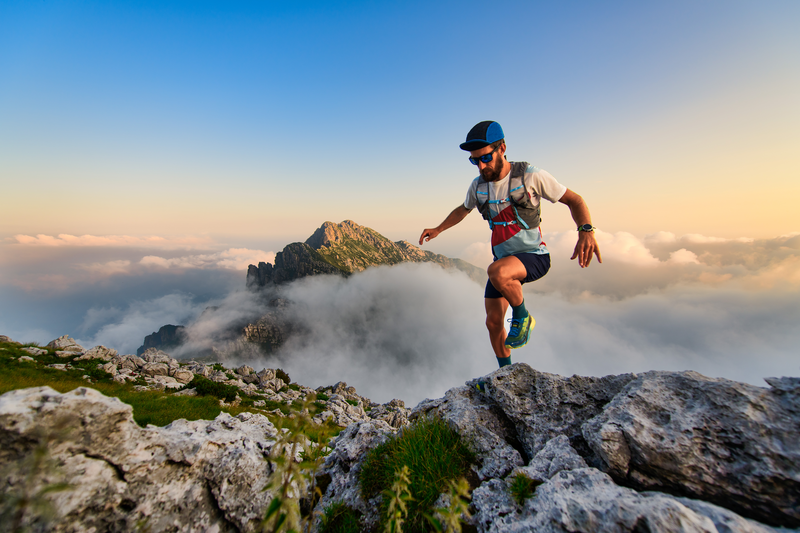
95% of researchers rate our articles as excellent or good
Learn more about the work of our research integrity team to safeguard the quality of each article we publish.
Find out more
ORIGINAL RESEARCH article
Front. Vet. Sci. , 04 February 2025
Sec. Veterinary Pharmacology and Toxicology
Volume 12 - 2025 | https://doi.org/10.3389/fvets.2025.1539448
This article is part of the Research Topic Natural Compounds in Veterinary Therapeutics View all 3 articles
Introduction: The normal butanol fraction of Polygonum hydropiper L. flavonoids (FNB) exhibits significant anti-inflammatory effects. This study investigated FNB's impact on inflammatory responses induced by Porcine circovirus type 2 (PCV2) in cell and mouse models.
Methods: An inflammatory model was established in RAW264.7 cells infected with varying PCV2 concentrations. And assigning both RAW264.7 cells and 108 SPF-grade KM mice to Control, PCV2, Rutin, and various dosages of FNB groups. Inflammatory factors such as Monocyte Chemoattractant Protein-1 (MCP-1), interleukin-6 (IL-6), IL-8, IL-10, Tumor Necrosis Factor-alpha (TNF-α), Reactive Oxygen Species (ROS), and Nitric Oxide (NO) were quantified using ELISA, RT-qPCR and immunohistochemistry.
Results: Results showed that a PCV2 titer of 104.5 TCID50/0.1 mL when applied to RAW264.7 cells effectively established an in vitro inflammatory model at 12 and 24 h post-infection. Following PCV2 infection, all the inflammatory factors displayed a significant increased both in culture supernatant and intracellular mRNA expression levels (p < 0.05 or p < 0.01), but these levels were reduced by FNB treatment (p < 0.05 or p < 0.01). In mouse sera post-PCV2 infection also showed elevated levels of IL-6, IL-8 IL-10, TNF-α, and MCP-1 (p < 0.05 or p < 0.01). Additionally, mRNA and protein levels for TNF-α, IL-8, IL-10, IL-6, and iNOS rose significantly in lung tissues (p < 0.01) but decreased with FNB treatment (p < 0.05 or p < 0.01).
Discussion: These findings suggest that FNB reduces inflammatory factor production and modulates the inflammatory response triggered by PCV2 infection, potentially enhancing host resistance against it.
Porcine circovirus type 2 (PCV2) infections inflict marked economic damage to the pig production industry (1). It can cause immunosuppression in pigs and is associated with various inflammatory and immune-related diseases (2). Studies reported that PCV2 infection can induce the upregulation of the interleukins (ILs) IL-1β, IL-6, and IL-8. This upregulation may result in excessive inflammatory responses during initial infection stages and attract neutrophils, leading to immunosuppression. Therefore, this affects the normal functioning of the immune response and facilitates the development of PCV2-related diseases (3). Therefore, controlling PCV2 infection and reducing the inflammatory responses it causes is critical to preventing and controlling PCV2-related diseases.
Flavonoids of Polygonum hydropiper L. are a class of natural active components extracted from the Polygonaceae plant Polygonum hydropiper L., which possess various pharmacological effects, including significant anti-inflammatory activity (4). Studies have reported that Polygonum hydropiper L. flavonoids have substantial anti-inflammatory effects by blocking the production of reactive oxygen species (ROS) and nitric oxide (NO) and upregulating the anti-inflammatory IL-10 while preventing the release of IL-1β, IL-6, IL-8, Tumor Necrosis Factor-alpha (TNF-α), and pro-inflammatory factors caused by LPS exposure in Mouse Mononuclear Macrophages Cells (RAW264.7 cells) (5). The RAW264.7 cell inflammation model is a major tool for studying inflammatory responses and evaluating the effectiveness of anti-inflammatory drugs (6). This model allows researchers to investigate the effects of various substances on inflammatory mediators, offering a theoretical foundation and prospective therapeutic techniques for treating inflammation-related disorders (7).
However, there have been no known cases of developing an inflammatory model of PCV2 infection in RAW264.7 cells to date. Furthermore, there is insufficient information about the modulation of PCV2-induced inflammatory responses by the normal butanol fraction of Polygonum hydropiper L. flavonoids (FNB). The current study evaluated the effect of FNB intervention on inflammatory responses induced by PCV2 infection in both cellular and animal models. Moreover, this study established a scientific foundation for preventing and treating animal viral infections through plant flavonoids.
Phosphate-buffered saline (PBS), fetal bovine serum (FBS), and high-glucose DMEM were obtained from Gibco (USA). TRIzol was from Takara Bio (Japan). The commercial ELISA kits were from NeoBioscience Technology Co., Ltd. (China) to analyze IL-10, IL-8, IL-6, Monocyte Chemoattractant Protein-1 (MCP-1), and TNF-α. The ROS Assay Kit was acquired from Applygen (China). The Chinese company Nanjing Jiancheng Bioengineering Institute provided the Nitric Oxide Assay Kit. The HiScript III RT SuperMix for qPCR (+gDNA wiper) and ChamQ Universal SYBR qPCR Master Mix were bought from Vazyme (China). The IL-10, IL-8, IL-6, TNF-α, and iNOS antibodies were acquired from Proteintech (China). The streptavidin-peroxidase (SP) immunohistochemistry (IHC) kit was purchased from Bioss (China).
The Polygonum hydropiper L. was supplied by Tai Hua Pharmaceutical Co. Ltd. Professor Renbin Huang of Guangxi Medical University's School of Pharmacy verified the authenticity of the plant material. Tao et al.'s technique comprised the extraction and purification of the FNB in accordance with the specified protocol (5). According to the HPLC study, FNB had a total flavonoid concentration of 55.3%. Its rutin, quercetin glycoside, and quercetin concentrations were 21.9%, 8.2%, and 20.6%, respectively (5). The solution was prepared in complete DMEM, filtered using a 0.22 μm mesh, and stored at 4°C for later application.
PCV2 was supplied by Nanjing Agricultural University (China). Using the Reed-Muench assay, PCV2 titers were found to be 104.5 TCID50/0.1 mL. RAW264.7 cells were procured from the Shanghai Institute of Biochemistry and Cell Biology at the Chinese Academy of Sciences. Cells were grown in DMEM with 10% heat-inactivated FBS in an incubator at 37°C with 5% CO2.
The grouping and treatment of the model establishment experiment are shown in Table 1, with four replicates per group. RAW264.7 cells (1 × 105 cells/well) were inoculated in 12-well plates. A total of 500 μL of PCV2 dilutions at varying concentrations (MOI 1, 0.1, 0.01, and 0.001) were added to the PCV2 infection groups. At the same time, an equal volume of serum-free DMEM culture media was administered to the Control group. Following a 2-h treatment, the cells were cultured in each well with 1 mL of cell maintenance medium (DMEM culture media containing 2% fetal bovine serum) following three PBS washes. Lastly, samples were collected at various intervals.
The grouping and treatment for the experiment on the intervention effect of FNB on inflammatory responses are shown in Table 2, with 4 replicates per group. RAW264.7 cells (1 × 105 cells/well) inoculated into 12-well plates. The PCV2, FNB (high-, medium-, and low-dosage), and Rutin groups were administered 104.5 TCID50 of the viral solution. In contrast, the Control group was supplemented with equivalent serum-free DMEM culture media. Following a 2-h treatment, the cells underwent three washes with PBS. In the Control and PCV2 groups, 1 mL of cell maintenance solution (DMEM with 2% FBS) was placed in each of the wells. The FNB and the Rutin groups were each supplemented with 1 mL of DMEM containing 80, 40, and 20 μg/mL of FNB and 40 μg/mL of Rutin, respectively, and then placed in an incubator for continued culture. Subsequently, the supernatant or cells were collected from the culture medium at 4, 8, 12, and 24 h for further experiments.
Table 2. Grouping and treatment for the experiment on the intervention effect of FNB on inflammatory responses.
The animal experiment referred to Chen's research (8). A total of 108 SPF-grade KM mice, each weighing 20 ± 2 g, comprising an equal distribution of males and females, were acquired from the Animal Experiment Center of Guangxi Medical University (China). Before the experiment, the mice were acclimatized in an environment maintained at 25°C and 65% humidity for 7 days to mitigate environmental stress. Table 3 indicates that the animals were randomly allocated to several groups, namely, Control, PCV2, Rutin (100 mg/kg·BW), and FNB (25, 50, 100, 200, and 400 mg/kg·BW) groups, as well as a highest dose FNB control group (not infected with the virus). Each group contained 12 mice. Mice received daily inoculations of 1 mL PCV2 via intraperitoneal injection (0.3 mL/mouse), nasal drops (0.2 mL), and gavage (0.5 mL/mouse) for 3 consecutive days. FNB was gavaged into the mice from day 4 to day 6. On day 7, the animals were euthanized via asphyxiation under the guidelines established by the Guangxi University Animal Ethics Committee. Blood and lung specimens were obtained for subsequent study.
The influence of FNB on RAW264.7 cell viability was assessed using the CCK8 assay. Briefly, a 96-well plate containing a Control group and 7 varying concentrations of FNB groups (12.5, 25, 50, 100, 200, 400, and 800 μg/mL), each with eight repetitions, was inoculated with 5 × 104 cells/mL at 100 μL/well. After adding 100 μL of FNB to each well, the wells were cultured for 24 h in a culture incubator. The growth medium was then taken out 2 h before the culture's end, 100 μL of serum-free DMEM with 10% CCK-8 was placed in each of the wells, and the culture was maintained for 2 h at 37°C with 5% CO2. Lastly, a microplate reader was used to detect the OD value at 450 nm, and the CCK8 kit's instructions were followed to determine the maximum safe concentration of FNB on RAW264.7 cells.
Following the supplier's instructions, the supernatant or serum was collected for the measurement of MCP-1, IL-10, IL-8, TNF-α, IL-6, ROS, and NO levels while employing enzyme-linked immunosorbent assay (ELISA) and related commercial assay kits.
As directed by the manufacturer, TRIzol was utilized for RNA extraction from cells and lungs. Reverse transcription was used for transcribing RNA into cDNA. Using the cDNA as the template, RT-qPCR amplification was performed through a SYRB super-mix solution and a CFX96TMReal-Time PCR Detection solution (Bio-Rad, USA). Table 4 contains primer sequences for β-actin, MCP-1, TNF-α, IL-10, IL-8, IL-6, and iNOS. The relative mRNA levels, adjusted to β-actin, were determined through the comparative 2−ΔΔct technique.
For the immunohistochemistry study, lung tissues preserved in 4% paraformaldehyde were embedded in paraffin wax and sliced into 3.5 μm thick pieces. The expression level of the relevant protein was then determined according to the IHC kits.
Data were analyzed with SPSS v.22.0, using the LSD test and one-way analysis of variance (ANOVA). Data are shown as mean ± standard deviation. p < 0.05 was considered statistically significant.
Using commercial assay kits, the concentrations of inflammatory cytokines were evaluated in vitro in RAW264.7 cells to develop an inflammatory model in RAW264 induced by PCV2 (Figure 1). At 12 and 24 h post-infection (hpi), all measured inflammatory cytokines showed a substantial increase (p < 0.05, p < 0.01) following infection with 100 PCV2 dilutions in comparison to the Control. This suggests that an in vitro inflammatory model can be established using 100 PCV2 dilutions to treat RAW264.7 cells when the PCV2 titer is 104.5 TCID50/0.1 mL. Therefore, we selected this condition to carry out subsequent experiments.
Figure 1. (A–G) Effects of PCV2 at different PCV2 dilutions on MCP-1, TNF-α, IL-6, IL-10, IL-8, ROS and NO level (mean ± SD, n = 4). *p < 0.05, **p < 0.01 vs. Control group.
CCK-8 assays were utilized for measuring cell viability. At 24 h post-treatment (hpt), FNB at 12.5, 25, and 50 μg/mL elevated cell viability (p < 0.01; Figure 2) while FNB at 100 and 200 μg/mL had no substantial impact (p > 0.05). However, FNB at 400 and 800 μg/mL notably reduced viability (p < 0.01). Therefore, to examine their intervention effects on inflammatory responses induced by PCV2 infection, FNB concentrations of 20, 40, and 80 μg/mL were finally selected as high, medium, and low dosages, respectively.
Figure 2. Effects of varying concentrations of FNB on RAW264.7 cell viability (mean ± SD, n = 4). **p < 0.01 vs. Control group.
Commercial assay kits were used to explore the intervention impacts of FNB on inflammatory cytokine levels in cells, including MCP-1, IL-8, IL-10, TNF-α, IL-6, ROS, and NO (Figure 3). The efficient development of an in vitro inflammatory model was demonstrated by the considerable up-regulation of all evaluated inflammatory factors induced by PCV2 infection relative to the Control (p < 0.05, p < 0.01).
Figure 3. (A–G) The impact of FNB as an intervention on inflammatory cytokine levels (mean ± SD, n = 4). *p < 0.05, **p < 0.01 vs. Control group. #p < 0.05, ##p < 0.01 vs. PCV2 group.
At 4 or 8 hpt, the PCV2-induced increase in IL-6 and MCP-1 decreased considerably (p < 0.01) after exposure to 20, 40, and 80 μg/mL FNB. At 4 and 8 hpt, 40 μg/mL of FNB significantly reduced IL-10 and IL-8 levels (p < 0.01). TNF-α and NO levels were substantially lower than those of the PCV2 group after 8, 12, and 24 h following 20 μg/mL therapy (p < 0.01 or p < 0.05). At 4, 8, 12, and 24 h, ROS levels were significantly reduced (p < 0.01) by 20, 40, or 80 μg/mL FNB. An inflammatory response was triggered upon PCV2 infection, seen in raised inflammatory factor levels. Treatment with FNB significantly reduced cell TNF-α, MCP-1, IL-8, IL-6, IL-10, ROS, and NO levels, indicating that FNB could prevent inflammatory responses induced by PCV2.
Using RT-qPCR, the mRNA levels of the cytokines were further investigated. Following infection with PCV2, significant (p < 0.01) up-regulation of all six inflammatory factors was observed. Transcription was decreased by 40 and 80 μg/mL FNB administration, as evidenced by lower mRNA levels of MCP-1, IL-8, IL-10, IL-6, TNF-α, and iNOS at 12 hpt (p < 0.05, p < 0.01) than the PCV2 groups (Figure 4). These findings revealed that FNB could prevent PCV2 from activating genes and that PCV2 produced an inflammatory response by encouraging the expression of associated genes.
Figure 4. Impact of FNB on inflammatory cytokine mRNA expression levels (mean ± SD, n = 4). **p < 0.01 vs. Control group. #p < 0.05, ##p < 0.01 vs. PCV2 group.
To evaluate the effects of FNB on inflammatory responses in vivo, we infected mice with PCV2 and treated them with FNB. The levels of IL-10, IL-6, TNF-α, MCP-1, and IL-8 in mouse sera were assessed by ELISAs. As shown in Figure 5, PCV2 infection significantly increased those inflammatory cytokines (p < 0.05 or p < 0.01). FNB administration at 100, 200, and 400 mg/kg·BW substantially decreased the increase in those inflammatory cytokines (p < 0.05 or p < 0.01).
Figure 5. (A–E) Effects of FNB on inflammatory cytokine levels in mouse sera after PCV2 infection (mean ± SD, n = 6). *p < 0.05, **p < 0.01 vs. Control group. #p < 0.05, ##p < 0.01 vs. PCV2 group.
We also investigated the intervention effects of FNB on PCV2 infection-induced inflammatory responses at the gene expression level. All inflammatory cytokines listed had significantly higher mRNA expression when infected with PCV2 (p < 0.01). Moreover, FNB was able to substantially down-regulate mRNA expression at doses of 25, 50, 100, 200, and 400 mg/kg·BW (Figure 6).
Figure 6. (A–F) Impact of FNB on inflammatory cytokine mRNA expression in mouse lungs following PCV2 infection (mean ± SD, n = 6). **p < 0.01 vs. Control group. ##p < 0.01 vs. PCV2 group.
Consistent with the earlier studies, we investigated the intervention effects of FNB on inflammatory responses produced by PCV2 infection using immunohistochemistry methods. The proteins IL-8, IL-10, TNF-α, IL-6, and iNOS were expressed in the lung tissues of both the PCV2 group and the Control group of mice, demonstrating a diffuse distribution in the lungs and localized expression in the cytoplasm of cells, alveolar spaces, blood vessels, and tiny bronchial epithelial cells (Figure 7A). Relative to the controls, the protein iNOS, IL-10, IL-8, TNF-α, and IL-6 expression levels in the lung tissue of PCV2-infected mice were substantially higher (p < 0.01). Administration of 25, 50, 100, 200, and 400 mg/kg·BW FNB markedly reduced the protein iNOS, IL-10, IL-8, TNF-α, and IL-6 expressions levels in the alveolar space, fine bronchi, and cell cytoplasm of mice (p < 0.01; Figure 7B).
Figure 7. (A, B) Effects of FNB on inflammatory cytokine protein levels in mouse lungs following PCV2 infection (mean ± SD, n = 6). **p < 0.01 vs. Control group. ##p < 0.01 vs. PCV2 group.
Interleukin-6 is a multifunctional cytokine essential for inflammatory responses and immunological control (9). In reports related to COVID-19, elevated levels of IL-6 are considered a predictive parameter for poor disease progression and the need for invasive oxygen support (10). IL-8 is a crucial inflammatory marker; as a chemokine, it attracts and activates neutrophils, regulates inflammatory responses, and participates in viral replication. It is reported that coronavirus porcine epidemic diarrhea virus utilizes IL-8 to facilitate viral replication by regulating Ca2+ flux (11). The levels of IL-8 are significantly elevated in various tumor tissues and patient serum, showing a clear correlation with patient prognosis and tumor staging (12). IL-10 is anti-inflammatory and promotes immune tolerance and regulates immune responses (13). However, in some cases, it may promote the survival of pathogens and the immune escape of tumors, and elevated IL-10 may be associated with the activity of viral infections (14). The CC chemokine family includes MCP-1, often referred to as Chemokine (CC motif) Ligand 2 (CCL2), which is essential for inflammatory reactions (15). It has been found that in COVID-19 patients, raised MCP-1 levels are associated with disease severity, and it can be used to monitor disease progression (16). One crucial pro-inflammatory cytokine necessary for apoptosis, inflammatory reactions, and immunological responses in several physiological processes is TNF-α (17). Inhibitors targeting TNF-α, such as infliximab, adalimumab, and etanercept, have been used clinically to treat multiple inflammatory diseases and have achieved significant therapeutic effects (18). ROS plays a complex and critical role in viral infections and inflammatory responses; excessive production of ROS can exacerbate inflammatory responses and enhance the replication ability of viruses (19). For example, the Porcine reproductive and respiratory syndrome virus utilizes ROS to enhance its replication (20). NO is also an important biological regulatory factor, and changes in NO levels can also serve as an inflammatory marker to determine whether an inflammatory response has occurred (21). NO levels can rise substantially in viral infections, particularly respiratory infections; in COVID-19 patients, for example, high NO levels are positively linked to disease severity (22). In this study, infection of RAW264.7 cells with PCV2 at a titer of 104.5 TCID50/0.1 mL for 12 and 24 h resulted in a significant increase of the levels of IL-6, IL-8, IL-10, MCP-1, TNF-α, ROS, and NO. Hence, the in vitro inflammatory model was established successfully.
On the other hand, studies have shown that flavonoid compounds possess significant antioxidant, anti-inflammatory, and antiviral properties. They delay the progression of viral infections by alleviating oxidative stress and inflammation by targeting pathways such as NF-κB, MAPK, ERK, and Akt (23). IFN-γ, IL-17, IL-8, IL-1β, IL-6, and TNF-α are inflammatory factors many flavonoids can decrease (24). Sargassum polysaccharide has been shown by Chen et al. to decrease TNF-α, IL-6, IL-1β, IL-8, IL-10, and MCP-1 and mRNA expression levels resulting from PCV2 infection in RAW264.7 cells (25). Chen et al. also showed that Spatholobus suberectus Dunn's total flavonoids may decrease the elevated ROS and NO generation in RAW264.7 cells by PCV2 infection (26). According to a study by Ren et al., in Pseudorabies virus-infected RAW264.7 cells, the ethyl acetate fraction of flavonoids from Polygonum hydropiper L. can lower the levels of iNOS, NO, ROS, and other inflammatory markers (27). Ren et al. also found that the ethyl acetate flavonoid fraction from Polygonum hydropiper L. can inhibit increases in PCV2-induced TNF-α, IL-1β, IL-8, and NF-κB mRNA levels (28). Thus, our data are consistent with these studies, further showing the intervention impacts of FNB on PCV2-induced in vitro inflammatory responses.
According to Wang et al.'s in vivo studies, Panax notoginseng saponins can lower MPO and iNOS activity significantly while also lowering ROS and NO levels in the splenic lymphocytes of PCV2-infected mice (29). The total flavonoid extract from Spatholobus suberectus Dunn has been shown by Fu et al. to lower MPO and iNOS activity, improve thymus and spleen indices in PCV2-infected mice, alter immunological function, and lessen oxidative stress induced by viral infection (30). These findings thus support our previous research, demonstrating the impact of FNB as an intervention on PCV2-induced inflammatory reactions in vivo. However, the specific molecular mechanisms underlying the intervention of FNB are still not fully understood. For instance, through which signaling pathways does FNB exert its anti-inflammatory effects, are there any non-coding RNAs involved, and what role do histone acetylation modifications play? These questions will be clarified in further studies conducted in our laboratory.
In conclusion, this study effectively created an in vitro PCV2 infection model in RAW264.7 cells and demonstrated that FNB could affect the inflammatory responses induced through PCV2 in vivo and in vitro. Moreover, the findings offer a foundation for the application of plant flavonoids in the prevention and management of viral infections in animals.
The original contributions presented in the study are included in the article/supplementary material, further inquiries can be directed to the corresponding author/s.
Approval was obtained from the Ethics Committee of Guangxi University. The procedures used in this study adhere to the tenets of the Declaration of Helsinki. The study was conducted in accordance with the local legislation and institutional requirements.
Y-hW: Data curation, Writing – original draft, Conceptualization, Methodology. S-mZ: Data curation, Writing – original draft, Conceptualization, Methodology. WZ: Visualization, Writing – review & editing, Software. QC: Writing – review & editing, Investigation, Supervision. Q-hW: Formal Analysis, Writing – review & editing, Data curation. M-lY: Data curation, Resources, Writing – review & editing. Y-yW: Investigation, Validation, Writing – review & editing. T-jH: Conceptualization, Funding acquisition, Project administration, Supervision, Writing – review & editing.
The author(s) declare financial support was received for the research, authorship, and/or publication of this article. This work was funded by the National Natural Science Foundation of China (Grant No. 32072907) and the Innovation Project of Guangxi Graduate Education (Grant No. YCBZ2024068).
We thank Dr. Huang KH, a member of the College of Veterinary Medicine at Nanjing Agricultural University, for providing PCV2.
The authors declare that the research was conducted in the absence of any commercial or financial relationships that could be construed as a potential conflict of interest.
The author(s) declare that no Gen AI was used in the creation of this manuscript.
All claims expressed in this article are solely those of the authors and do not necessarily represent those of their affiliated organizations, or those of the publisher, the editors and the reviewers. Any product that may be evaluated in this article, or claim that may be made by its manufacturer, is not guaranteed or endorsed by the publisher.
1. Guo J, Hou L, Zhou J, Wang D, Cui Y, Feng X, et al. Porcine circovirus type 2 vaccines: commercial application and research advances. Viruses. (2022) 14:2005. doi: 10.3390/v14092005
2. Yang X, Du Q, Wang X, Shi J, Wang T, Li P, et al. Porcine circovirus type 2 infection inhibits macrophage M1 polarization induced by other pathogens via viral capsid protein and host gC1qR protein. Vet Microbiol. (2023) 285:109871. doi: 10.1016/j.vetmic.2023.109871
3. Choi CY, Rho SB, Kim HS, Han J, Bae J, Lee SJ, et al. The ORF3 protein of porcine circovirus type 2 promotes secretion of IL-6 and IL-8 in porcine epithelial cells by facilitating proteasomal degradation of regulator of G protein signalling 16 through physical interaction. J Gen Virol. (2015) 96:1098–108. doi: 10.1099/vir.0.000046
4. Shen N, Wang T, Gan Q, Liu S, Wang L, Jin B. Plant flavonoids: classification, distribution, biosynthesis, and antioxidant activity. Food Chem. (2022) 383:132531. doi: 10.1016/j.foodchem.2022.132531
5. Tao J, Wei Y, Hu T. Flavonoids of Polygonum hydropiper L. attenuates lipopolysaccharide-induced inflammatory injury via suppressing phosphorylation in MAPKs pathways. BMC Complement Altern Med. (2016) 16:25. doi: 10.1186/s12906-016-1001-8
6. Li P, Hao Z, Wu J, Ma C, Xu Y, Li J, et al. Comparative proteomic analysis of polarized human THP-1 and mouse RAW2647 macrophages. Front Immunol. (2021) 12:700009. doi: 10.3389/fimmu.2021.700009
7. Castaneda OA, Lee SC, Ho CT, Huang TC. Macrophages in oxidative stress and models to evaluate the antioxidant function of dietary natural compounds. J Food Drug Anal. (2017) 25:111–8. doi: 10.1016/j.jfda.2016.11.006
8. Chen HL, Tan HL, Yang J, Song ML, Feng HY, Kuang N, et al. Inhibitory effect of polysaccharide of Sargassum weizhouense on PCV2 induced inflammation in mice by suppressing histone acetylation. Biomed Pharmacother. (2019) 112:108741. doi: 10.1016/j.biopha.2019.108741
9. Kaur S, Bansal Y, Kumar R, Bansal G. A panoramic review of IL-6: structure, pathophysiological roles and inhibitors. Bioorg Med Chem. (2020) 28:115327. doi: 10.1016/j.bmc.2020.115327
10. Kishimoto T, Kang S. IL-6 revisited: from rheumatoid arthritis to CAR T cell therapy and COVID-19. Annu Rev Immunol. (2022) 40:323–48. doi: 10.1146/annurev-immunol-101220-023458
11. Guo X, Feng Y, Zhao X, Qiao S, Ma Z, Li Z, et al. Coronavirus porcine epidemic diarrhea virus utilizes chemokine interleukin-8 to facilitate viral replication by regulating Ca2+ flux. J Virol. (2023) 97:e0029223. doi: 10.1128/jvi.00292-23
12. Bakouny Z, Choueiri TK. IL-8 and cancer prognosis on immunotherapy. Nat Med. (2020) 26:650–1. doi: 10.1038/s41591-020-0873-9
13. Saraiva M, Vieira P, O'Garra A. Biology and therapeutic potential of interleukin-10. J Exp Med. (2020) 217:e20190418. doi: 10.1084/jem.20190418
14. Alagarasu K, Kaushal H, Shinde P, Kakade M, Chaudhary U, Padbidri V, et al. TNFA and IL10 polymorphisms and IL-6 and IL-10 levels influence disease severity in influenza A(H1N1)pdm09 virus infected patients. Genes. (2021) 12:1914. doi: 10.3390/genes12121914
15. Singh S, Anshita D, Ravichandiran V. MCP-1: function, regulation, and involvement in disease. Int Immunopharmacol. (2021) 101:107598. doi: 10.1016/j.intimp.2021.107598
16. Chen Y, Wang J, Liu C, Su L, Zhang D, Fan J, et al. IP-10 and MCP-1 as biomarkers associated with disease severity of COVID-19. Mol Med. (2020) 26:97. doi: 10.1186/s10020-020-00230-x
17. Dömling A, Li X. TNF-α: the shape of small molecules to come? Drug Discov Today. (2022) 27:3–7. doi: 10.1016/j.drudis.2021.06.018
18. Jang DI, Lee AH, Shin HY, Song HR, Park JH, Kang TB, et al. The role of tumor necrosis factor alpha (TNF-α) in autoimmune disease and current TNF-α inhibitors in therapeutics. Int J Mol Sci. (2021) 22:2719. doi: 10.3390/ijms22052719
19. Wang L, Cao Z, Wang Z, Guo J, Wen J. Reactive oxygen species associated immunoregulation post influenza virus infection. Front Immunol. (2022) 13:927593. doi: 10.3389/fimmu.2022.927593
20. Zhang S, Zeng L, Su B, Yang G, Wang J, Ming S, et al. The glycoprotein 5 of porcine reproductive and respiratory syndrome virus stimulates mitochondrial ROS to facilitate viral replication. MBio. (2023) 14:e0265123. doi: 10.1128/mbio.02651-23
21. Facchin BM, Dos Reis GO, Vieira GN, Mohr ETB, da Rosa JS, Kretzer IF, et al. Inflammatory biomarkers on an LPS-induced RAW 2647 cell model: a systematic review and meta-analysis. Inflamm Res. (2022) 71:741–58. doi: 10.1007/s00011-022-01584-0
22. Hussain M, Khurram Syed S, Fatima M, Shaukat S, Saadullah M, Alqahtani AM, et al. Acute respiratory distress syndrome and COVID-19: a literature review. J Inflamm Res. (2021) 14:7225–42. doi: 10.2147/JIR.S334043
23. Al-Khayri JM, Sahana GR, Nagella P, Joseph BV, Alessa FM, Al-Mssallem MQ. Flavonoids as potential anti-inflammatory molecules: a review. Molecules. (2022) 27:2901. doi: 10.3390/molecules27092901
24. Mahmoud AM, Sayed AM, Ahmed OS, Abdel-Daim MM, Hassanein EHM. The role of flavonoids in inhibiting IL-6 and inflammatory arthritis. Curr Top Med Chem. (2022) 22:746–68. doi: 10.2174/1568026622666220107105233
25. Chen HL, Tan HL, Yang J, Wei YY, Hu TJ. Sargassum polysaccharide inhibits inflammatory response in PCV2 infected-RAW2647 cells by regulating histone acetylation. Carbohydr Polym. (2018) 200:633–40. doi: 10.1016/j.carbpol.2018.06.060
26. Chen HL, Yang J, Fu YF, Meng XN, Zhao WD, Hu TJ. Effect of total flavonoids of Spatholobus suberectus Dunn on PCV2 induced oxidative stress in RAW2647 cells. BMC Complement Altern Med. (2017) 17:244. doi: 10.1186/s12906-017-1764-6
27. Ren CZ, Hu WY, Li JC, Xie YH, Jia NN, Shi J, et al. Ethyl acetate fraction of flavonoids from Polygonum hydropiper L. modulates pseudorabies virus-induced inflammation in RAW2647 cells via the nuclear factor-kappa B and mitogen-activated protein kinase pathways. J Vet Med Sci. (2020) 82:1781–92. doi: 10.1292/jvms.20-0263
28. Ren CZ, Hu WY, Song ML, Wei YY, Hu TJ. An ethyl acetate fraction of flavonoids from Polygonum hydropiper L. exhibits an anti-inflammatory activity in PCV2-infected porcine alveolar macrophages via PI3K/Akt and NF-κB pathways. Vet Res Forum. (2022) 13:339–47. doi: 10.30466/vrf.2021.137675.3070
29. Wang QH, Kuang N, Hu WY, Yin D, Wei YY, Hu TJ. The effect of Panax notoginseng saponins on oxidative stress induced by PCV2 infection in immune cells: in vitro and in vivo studies. J Vet Sci. (2020) 21:e61. doi: 10.4142/jvs.2020.21.e61
Keywords: porcine circovirus type 2, normal butanol fraction of Polygonum hydropiper L. flavonoids, inflammatory response, RAW264.7 cells, model establishment
Citation: Wei Y-h, Zhou S-m, Zhao W, Chen Q, Wang Q-h, Yu M-l, Wei Y-y and Hu T-j (2025) Normal butanol fraction of Polygonum hydropiper L. flavonoids reduces inflammation caused by PCV2 infections in cell and mouse models. Front. Vet. Sci. 12:1539448. doi: 10.3389/fvets.2025.1539448
Received: 04 December 2024; Accepted: 13 January 2025;
Published: 04 February 2025.
Edited by:
Shuaiyu Wang, China Agricultural University, ChinaCopyright © 2025 Wei, Zhou, Zhao, Chen, Wang, Yu, Wei and Hu. This is an open-access article distributed under the terms of the Creative Commons Attribution License (CC BY). The use, distribution or reproduction in other forums is permitted, provided the original author(s) and the copyright owner(s) are credited and that the original publication in this journal is cited, in accordance with accepted academic practice. No use, distribution or reproduction is permitted which does not comply with these terms.
*Correspondence: Ting-jun Hu, dGluZ2p1bmh1QDEyNi5jb20=
Disclaimer: All claims expressed in this article are solely those of the authors and do not necessarily represent those of their affiliated organizations, or those of the publisher, the editors and the reviewers. Any product that may be evaluated in this article or claim that may be made by its manufacturer is not guaranteed or endorsed by the publisher.
Research integrity at Frontiers
Learn more about the work of our research integrity team to safeguard the quality of each article we publish.