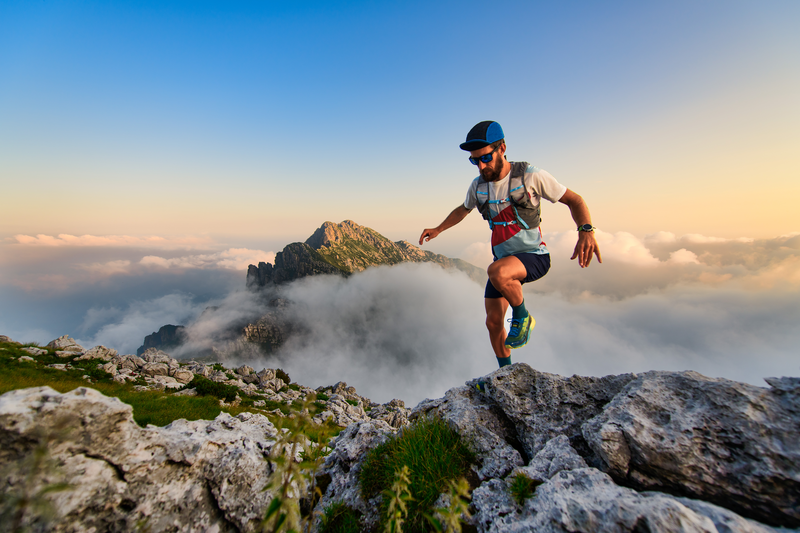
95% of researchers rate our articles as excellent or good
Learn more about the work of our research integrity team to safeguard the quality of each article we publish.
Find out more
MINI REVIEW article
Front. Vet. Sci. , 28 March 2025
Sec. Veterinary Infectious Diseases
Volume 12 - 2025 | https://doi.org/10.3389/fvets.2025.1535650
This article is part of the Research Topic Biosecurity of Infectious Diseases in Veterinary Medicine View all 8 articles
Introduction: Canine circovirus (CanineCV) is an emerging pathogen with a significant impact on animal health and potential zoonotic risks. This study addresses its characterization, epidemiology, pathogenesis, and diagnostics, emphasizing its relevance within the One Health approach.
Background: The increasing detection of CanineCV across various species and regions highlights its genetic adaptability and cross-species transmission potential. Furthermore, growing interactions among domestic animals, wildlife, and humans amplify the need to understand its public and animal health implications.
Objective: To analyze the biology, epidemiology, and diagnostic challenges of CanineCV, focusing on its genetic evolution, interactions with co-infections, and implications for control strategies.
Methods: A systematic literature review was conducted, synthesizing data from epidemiological, genomic, and clinical studies. Molecular techniques, such as PCR and qPCR, were evaluated for their efficacy in virus detection and quantification.
Results: Canine circovirus exhibits high genetic variability and has been detected in diverse species and tissues. Co-infections, including parvovirus and adenovirus, exacerbate clinical signs, primarily gastrointestinal, and respiratory. Advances in diagnostics, such as real-time PCR and in situ hybridization, have demonstrated increased sensitivity in viral detection.
Conclusion: Canine circovirus poses a growing challenge to animal health and a potential threat to public health due to its genetic plasticity and adaptability to multiple hosts. Continuous research is essential to understand its pathogenesis, develop effective control measures, and mitigate risks in diverse ecosystems.
Pets play a significant role in contemporary society, transcending their historical function as mere guardians or domestic helpers. According to the International Federation for Animal Health (IFAH), “pet” refers to any animal kept by humans for companionship, recreation, or as part of the family unit, encompassing not only dogs and cats but also birds, reptiles, fish, rodents, and other small mammals (2, 3). The human-animal relationship has evolved over the decades, shifting from a utilitarian interaction to an emotional bond, with documented benefits for the mental and physical health of their owners, such as stress reduction, increased social engagement, and improved quality of life (4). In Brazil, this scenario is widely reflected in households, where 47.9 million families own at least one pet, representing ~46.1% of all national households, according to the Brazilian Institute of Geography and Statistics [IBGE; (1)]. This figure becomes even more remarkable when considering that the country leads globally in the number of small dogs per capita and holds a prominent position in the ownership of cats and other animals. The broad definition of the term “pet” not only reflects the diversity of species that share domestic spaces with humans but also highlights the complexity of human-animal interactions, which are influenced by cultural, socioeconomic, and environmental factors (73). Thus, understanding the concept of “pet” in its entirety is essential to contextualizing health impacts, both human and animal, within the One Health approach (5–7).
Canine circovirus (CanineCV) is an emerging virus with a significant impact, particularly in the absence of vaccines. This virus often displays a variety of clinical signs, which can be further complicated by co-infections, potentially altering the clinical presentation. Additionally, the potential for zoonotic transmission cannot be excluded, as other viruses within the same genus, which includes human circovirus, are being considered for such transmission (8–10).
Canine circovirus belongs to the genus Circovirus within the family Circoviridae According to the classification of the International Committee on Taxonomy of Viruses (ICTV), species demarcation within the Circoviridae family is based on at least 80% nucleotide identity across the entire genome, along with structural and organizational characteristics. An essential criterion is the location of the replication origin (ori) relative to the coding regions. In members of the genus Circovirus, the ori is located on the same strand that encodes the replication-associated protein (Rep), while in the genus Cyclovirus, the ori is situated on the strand that encodes the capsid protein [Cap; (10, 11)]. Additionally, the genomes of these viruses exhibit an ambisense organization with two primary open reading frames (ORFs) responsible for encoding the Rep and Cap proteins, with replication occurring through a rolling circle replication mechanism.
The diversity within this family has been significantly expanded through metagenomic sequencing and degenerate PCR methods, revealing a broad distribution among mammals, birds, and even invertebrates. Phylogenetic studies indicate a closer relationship among circoviruses detected in mammals, whereas those found in birds and fish display greater genetic distance, reflecting their complex evolution. These criteria and technological advancements not only facilitate taxonomic classification but also provide a broader understanding of the biology and ecology of these viruses, which are essential for epidemiological and viral evolution studies (12, 13).
Canine circovirus was first identified in 2012 after the extraction of viral nucleic acid from a set of canine serum samples in the United States. In 2013, the complete genome was characterized in California (USA) and, after a year later, the virus was reported in a young dog in Italy. Since its identification, CanineCV has been associated with conditions such as vasculitis, hemorrhagic gastroenteritis, and diarrhea (57) and has been reported in all continents, except Oceania (14–19).
Canine circovirus, like other circovirus species, poses significant challenges in classification due to notable genetic variability. It is crucial to establish common terminology with robust classification criteria, ensuring reproducible results and promoting essential advancements in understanding diseases associated with the virus. This includes assessing the impact of coinfections on clinical signs to comprehend its effects on animal health and potential implications for public health.
Canine circovirus are non-enveloped icosahedral viruses with a single-stranded circular DNA genome of ~2 kb. As previously mentioned, they belong to the Circoviridae family. The classification of CanineCV as a new species within the circovirus genus occurred because, according to criteria set by the International Committee on Taxonomy of Viruses (10), circoviruses must share more than 75% nucleotide identity across their complete genome and more than 70% sequence identity in their capsid protein sequences to be considered the same species. Despite being genetically closer to porcine circovirus, in the study identifying the complete genome sequence of the first canine circovirus, the capsid (Cap) and replicase (Rep) proteins of CanineCV shared <25% and 50% identity, respectively, with circoviruses from other animals (8).
The CanineCV genome is a circular single-stranded DNA with 2,063 nucleotides (nt) that comprises two open reading frames (ORFs) on complementary strands oriented in opposite directions. ORF1, with 911 nt, encodes the replicase protein (303 amino acids), which is essential for viral replication. ORF2, with 811 nt, encodes the capsid protein (270 amino acids), which has a structural function. The genome also contains two intergenic non-coding regions that are 135 and 203 nucleotides long. At the replication origin (TAG TAT TACA), there is a palindromic sequence of 12 nt pairs and a 10-nucleotide open loop (CAT AGT ATT A). The amino terminus of the proposed capsid protein features a 30-amino-acid arginine-rich region, like those found in other animal circoviruses. Additionally, a third ORF (ORF-3) was identified in the antisense strand of ORF-1 from a Thailand strain, although its function is still unknown [(8, 20); Figure 1].
Figure 1. Schematic representation of the CanineCV genome. Information on open reading frames [ORF1, ORF2, ORF3; (8, 19, 20)].
Although the replication of CanineCV has not been described, we can infer its replication process based on the well-documented replication mechanism of PCVs, especially PCV1 and PCV2, with the following steps (Figure 2): [1] The entry begins with the virus attaching to the host cell surface. This process is mediated by interactions between viral proteins and specific receptors on the host cell membrane. Entry primarily occurs through clathrin-mediated endocytosis, where the virus is engulfed into an endocytic vesicle and transported into the cell. [2] After entry into the cell, the viral capsid is uncoated, releasing the viral genome into the cytoplasm. The uncoating process involves the fusion of the endocytic vesicle with lysosomes, where the acidic pH facilitates the release of the single-stranded circular DNA from the protein capsid, which is transported to the cell nucleus, where replication occurs. [3] The replication of the virus genome occurs in the host cell nucleus. The Rep protein recognizes and binds to the origin of replication on the viral DNA. The Rep protein has endonuclease activity, which creates a nick in the DNA strand, producing a free 3′ OH end essential for new DNA strand synthesis. Using the 3′ OH end as a primer, the host DNA polymerase extends the DNA strand, synthesizing a new strand complementary to the original template strand. This results in the formation of a double-stranded replicative form (RF) DNA structure, which serves as a template for the synthesis of new viral single-stranded DNA through the rolling circle replication (RCR) mechanism. Replication is completed when the synthesis of the new DNA strand forms a full circle and meets the original 5′ end. The Rep protein makes another nick to release the new single-stranded DNA, which can be encapsulated into new viral particles. [4] The assembly of new viral particles occurs in the host cell nucleus. The capsid proteins (Cap), encoded by the ORF2 gene, are synthesized and transported to the nucleus, where they encapsulate the newly synthesized viral DNA. This assembly process involves forming complete viral capsids that enclose the viral DNA genome, creating new virions. [5] After assembly, the complete virions are transported out of the nucleus and accumulate in the cytoplasm before being released from the infected cell. Release can occur through cell lysis, where the host cell is destroyed, releasing virions into the extracellular environment. Alternatively, virions can be released through exocytosis, where vesicles containing virions fuse with the plasma membrane, releasing virions outside the cell without causing immediate host cell death. The replication process is carried out by cellular enzymes that are expressed during the S-phase of the host cell cycle (21–26).
There is still a lack of clarity regarding the physicochemical properties, replication process, and pathogenic characteristics of CanineCV. A study was conducted, rescuing a strain of canine circovirus in F81 cells using infectious clone plasmids, and it was discovered that the Rep protein produced by the viral packaging rescue process is associated with cytopathic effects. The Rep protein of CanineCV inhibited the activation of the type I Interferon (IFN-I) promoter, blocking the subsequent expression of interferon-stimulated genes (27).
Like observed in other circoviruses, a high evolutionary rate of 1.21 × 10−3 substitutions/site/year was described (66). This can be confirmed by the different genotypes that have been described since its first identification in 2012 (8, 17–19, 28, 29).
Phylogenetic analyses of the strains reported to date have been conducted using the complete genome sequences. These analyses also incorporate the nucleotide sequences or concatenated amino acids of the Rep and Cap proteins (24, 25, 66). Multiple efforts have been made to establish a classification system that helps understand virus origin and evolution. However, based on most recent articles where sequencing has been performed, the classification into six genotypes, i.e., CanineCV 1 to CanineCV 6, has been the most used and accepted (24, 25, 28, 30–33).
Phylogenetic analyses indicate that CanineCV likely originated from bat circovirus (BatACV). Maximum clade credibility (MCC) and maximum-likelihood (ML) trees constructed from ORF1 gene sequences suggest a close relationship between CanineCV and BatACV strains. This hypothesis is supported by the observation that circoviruses, including CanineCV, often undergo cross-species transmission, a major driver of their evolution. The genetic variations are often reflected in the virus's codon usage patterns, which have been influenced predominantly by natural selection rather than mutation pressure. This natural selection is a significant force shaping the codon usage bias (CUB) of CanineCV, enhancing its adaptability and survival in various hosts (16, 20, 33–36).
Codon adaptation index (CAI) and relative codon deoptimization index (RCDI) analyses have revealed that CanineCV exhibits the highest adaptability to red foxes, followed by domestic dogs and arctic foxes. This adaptability is attributed to the virus's ability to optimize its protein synthesis machinery to align with the host's codon usage preferences, thereby enhancing its replication efficiency and fitness. Interestingly, while CanineCV shows strong ties with wolves based on SiD analysis, the virus has developed the strongest adaptation to red foxes, indicating a complex interplay of host-specific adaptations driven by natural selection (34, 36).
Canine circovirus has been detected on every continent except Oceania (14–19).
In the United States, the virus was first identified in 2012, followed by Italy in 2014, and the United Kingdom in 2015. Subsequent detections occurred in Taiwan (2016), Germany (2017), and Thailand (2017). Brazil reported its first case in 2018, with Argentina following in 2019. China, Turkey, and Colombia all recorded their initial detections in 2020. Vietnam identified the virus in 2020, Iran in 2022, and Namibia in 2023. These findings illustrate the widespread and chronological emergence of CanineCV across multiple continents, highlighting its global distribution [(8, 15, 16, 19, 20, 31, 35, 37, 38, 41–43, 57, 60, 67); Figure 3].
Retrospective studies have shown that CanineCV was present in Latin America as early as 2012 (43). In Europe, detection of the virus dates back to samples from 1995, indicating a longer and possibly more widespread historical presence of CanineCV in canine populations across different continents (68).
Canine circovirus has been identified in various host species, demonstrating its capacity for cross-species transmission and adaptability. Most detections have been reported in dogs, starting from 2012 [(8, 16, 19, 20, 25, 31, 37–43); Figure 3].
The prevalence of CanineCV in dogs varies widely from 3.6% to 28.0%, depending on the presence and severity of clinical signs. This range indicates that clinical manifestations play a significant role in the detection rates of CanineCV among domestic dogs (30, 44).
In wild carnivores, the prevalence of CanineCV shows considerable variation across different species. In foxes, the prevalence of CanineCV ranged from 0% to 4.3%. In badgers, the prevalence was 18%. In jackals, the prevalence was notably high at 43.7%, while wolves exhibited an even higher prevalence of 50% (15, 18, 30, 45, 46).
The classification into six genotypes, has revealed various geographic and host-specific distributions. CanineCV-1 has been detected in dogs primarily in China, USA, Colombia, Argentina, Italy, Germany, and Vietnam. Additionally, it has been found in wolves in Italy. CanineCV-2 has been found in dogs exclusively in China. CanineCV-3 has been detected in dogs in China, Vietnam, and Thailand. CanineCV-4 has been observed in both wolves and dogs in Italy, as well as in dogs in China, Germany, Argentina, and Colombia. CanineCV-5 has been found in Arctic foxes (Vulpes lagopus) and red foxes (Vulpes vulpes) in the Arctic, Norway, and the United Kingdom. Finally, CanineCV-6 has been detected in dogs in Iran [(8, 18, 19, 24, 29–33, 47); Table 1].
Canine circovirus has been detected in numerous tissue types, including the brain (dog and wolves), intestine (dog, wolves, and badgers), liver (dog), spleen (dog, wolves, fox, and badgers), lymph nodes (dog and jackals), and lungs (dog, wolves, and jackals). This extensive range of sample types demonstrates the virus's ability to infect and persist in different organs and tissues, contributing to its maintenance and spread within and between species [(8, 15, 28, 30, 45, 46); Table 2]. The detection of the virus in such a wide array of tissues highlights its versatility and pathogenic potential. Understanding the tissue tropism of the virus is crucial for developing effective strategies to control its spread and mitigate its impact on both domestic and wild animal populations. Further research is necessary to elucidate the mechanisms behind the virus's tissue-specific infection and its implications for disease progression and transmission.
Like Porcine circovirus 3 (PCV3), which infects swine, CanineCV originated from bat circovirus (BatACV). This ancestral virus may have adapted either directly to domestic dogs or through other intermediate hosts, allowing for cross-species transmission. Notably, PCV3 has been detected in ticks, and although CanineCV has not yet been described in ticks, this possibility should be considered. Figure 4 illustrates the potential transmission routes of CanineCV among different environments and species. It highlights direct contact and possible tick-mediated transmission as mechanisms through which the virus may spread among peri-domestic animals such as dogs and cats, and wild animals including foxes, badgers, jackals and wolves (9, 16, 33, 44, 48, 49). The figure underscores the complexity of CanineCV transmission dynamics and the need for further research to understand these interactions and their implications for viral maintenance and spread in diverse ecological setting (Figure 4).
Figure 4. Schematic representation of potential occurrence CanineCV transmission route across different species is a potential occurrence based on the available literature. Indicates possibility of transmission that may have started in the peridomestic or wild environment indicates a remote possibility of transmission (9, 50).
The spillover events should be closely monitored due to the high mutation rate of CanineCV and recent socio-economic changes that have increased the proximity of companion animals to wild environments. Understanding these dynamics is crucial for developing effective strategies to control the spread of CanineCV and mitigate its impact on both domestic and wild animal populations (50).
The primary route of transmission for CanineCV is fecal-oral, affecting both domestic and wild animals. Viral loads in feces have been detected at 1.8 × 103 copies of target DNA/μL of extracted DNA in dogs and 8.94 × 104 copies in foxes. Notably, animals without clinical signs also shed high viral loads in their feces, as indicated by a low cycle threshold (Ct) value of 20.7 (31, 32, 45). It must be considered that the high amount of viral particles is an important factor for viral spread in animal populations.
Special attention in the epidemiological chain should be given to the fact that some animals, such as dogs, cat, foxes, jackals, and wolves, may be infected without showing clinical signs, as they can still disseminate the virus. However, it should be considered that, like swine, the viral load shed by asymptomatic animals is lower. This highlights the importance of monitoring both symptomatic and asymptomatic carriers to effectively control the spread of CanineCV in various animal populations (15, 28, 30, 51, 52).
While the fecal-oral route is the primary mode of transmission for CanineCV, the potential for respiratory transmission also warrants attention. Although few studies have investigated this route, the virus has been detected in respiratory samples, indicating that respiratory transmission could be a significant pathway for the spread of CanineCV (28, 29). Further research is necessary to understand the extent and implications of respiratory transmission in both domestic and wild animal populations.
The prevalence of CanineCV varied significantly by age group. Among dogs aged 0–1 years, the prevalence ranged from 17.5% to 43.1%, indicating a higher susceptibility in this age group. For dogs aged 1–8 years, the prevalence ranged from 9.6% to 43.1%. In dogs older than 8 years, the prevalence was consistently reported at 0% to 13.8%. Additionally, for dogs with unreported ages, the prevalence was noted to be 13.2%. These findings highlight the significant differences in CanineCV prevalence across age groups, with the highest rates observed in the youngest dogs (14, 15, 29–32, 38, 42, 44, 53).
The prevalence of CanineCV in wolves showed significant variation across different age groups. The overall prevalence was 47.8%, with 43.5% of infected wolves being puppies (<12 months old), 30.4% being sub-adults (13–24 months), and 26.1% being adults (older than 24 months). Specifically, the prevalence was highest in puppies at 50% (5/10), followed by sub-adults at 42.9% (3/7), and adults also at 50% (3/6). These findings highlight that CanineCV affects wolves across all age groups, with a notable prevalence in both the youngest and oldest age categories, differently from what is seen in dogs (15, 30, 46).
Sex-based analysis of CanineCV infection rates revealed a higher prevalence in female dogs compared to male dogs, although this difference was not statistically significant. The prevalence in female dogs ranged from 57.1% to 67.6%, while in male dogs it ranged from 32.4% to 42.9% (31, 53).
The virus's ability to infect and persist in multiple tissues not only aids in its dissemination but also in its pathogenicity, contributing to a range of clinical manifestations in infected hosts. In CanineCV infected animals, most clinical signs are related to the digestive system but are also associated with the respiratory and nervous systems.
The prevalence of CanineCV among symptomatic and asymptomatic animals shows significant variability. On average, 10.6% of asymptomatic animals are infected, with a range between 6.9% and 28.5%. Among symptomatic animals, the average prevalence is higher, at 20.3%, with a range between 6% and 32.8%. In cats, a similar pattern is observed, with a higher prevalence of CanineCV in symptomatic animals (3.6%) compared to asymptomatic ones (1.1%). These findings show that CanineCV infection is present in both symptomatic and asymptomatic populations, with a notably higher prevalence in those showing clinical signs (14, 15, 18, 24, 28, 29, 31, 32, 38, 42, 53, 54).
Considering 16 articles describing clinical signs, a ranking of the most common clinical signs associated with CanineCV, as shown in Figure 5, indicates that enteric disturbances are the most frequently observed. Diarrhea was the most prevalent symptom, observed in 93.7% of cases, followed by hemorrhagic enteritis, which occurred in 87.5% of cases. Vomiting was documented in 43.7% of the cases, while anorexia and enteritis were less frequent, each with a prevalence of 18.7%. Lethargy and gingival hemorrhage were the least common symptoms, each observed in 6.2% of cases [(14, 20, 24, 28, 31, 32, 38, 42, 51, 53–58); Figure 5].
Figure 5. Overall prevalence (%) of clinical signs in dogs infected with CanineCV 3 based on consulted literature.
In addition to these more common signs reported in studies, there are studies associating CanineCV with respiratory (28, 59) and nervous signs (58) and lymphadenitis (31).
As observed with CanineCV involvement digestive systems clinical signs, the prevalence of the virus in animals with respiratory illness is also higher, as demonstrated in this study linking respiratory diseases to CanineCV. The overall occurrence of CanineCV infection was 8.95% (17/190), with 2.6% (2/76) in the healthy group and 13.2% (15/114) in the respiratory illness group and several factors can influence its prevalence. Age-wise, juniors (<1.5 years) exhibited the highest positivity rate at 17.5%, compared to adults (1.5–6 years) at 10.5% and seniors (≥6 years) at 1.3%. Sex-wise, females showed a higher positivity rate at 10.5% compared to males at 5.5% (29).
There are few studies that describe histopathological lesions, most of which are associated with enteric disorders. In a case report from Connecticut, United States, microscopic examination revealed major lesions in the gastrointestinal tract confined to the small intestine. These included random crypt cell necrosis and focal hemorrhage in the lamina propria and submucosa. Vascular changes comprised endothelial cell swelling and sloughing, leading to endothelial disruption. Vasculitis was noted in small arterioles of the basal mucosa and submucosa, rarely accompanied by thrombi. Hyaline degeneration and fibrinoid necrosis of small vessels were occasionally observed (32, 58, 59).
Histologically, CanineCV-positive dogs with respiratory illness exhibited varying severities of generalized hemorrhagic pyogranulomatous pneumonia, multifocal hemorrhagic pneumonia, and severe diffuse suppurative, hemorrhagic, and necrotic bronchiolitis and alveolitis (29).
Other organs such as the brain, meninges, myocardium, lung, liver, and kidney exhibited severe focal vasculitis with mononuclear cell inflammation. The spleen and lymph nodes showed significant lymphocyte necrosis and hemorrhage. Histological analysis revealed hyaline degeneration, fibrinoid necrosis of small vessels, and marked sinus histiocytosis in lymph nodes (58–60).
As observed in other animal species, such as swine, PCVs co-infected with other viruses or bacteria have been demonstrated to enhance PCV2 and PCV3 replication in target tissues. This co-infection increases the severity of induced lesions and exacerbates the clinical course of the disease. The presence of concurrent infections significantly impacts the pathogenesis, leading to more severe clinical manifestations and challenging disease management (9, 52). Studies have shown that CanineCV can co-infect with various other pathogens, the most common being canine parvovirus (CPV), canine adenovirus (CAdV), coronavirus (CCoV), and distemper virus (CDV), generally resulting in severe clinical signs.
In double co-infections, co-infection with CPV varied between 18.7% and 57.7% (54, 61), while CPV2 co-infection rates were 15.6% (15/96) and 16.6% (33, 41). CCoV was found in 12.67% of cases (54). The frequencies of triple co-infections involving CPV, CCoV, and CanineCV ranged from 3.2% to 9.8%. A retrospective study analyzing 95 samples of enteritis caused by parvovirus found that 8 (8.9%) were positive for CanineCV (30).
In addition to viral co-infections, double and triple infections with others agents were also related with enterotoxigenic Escherichia coli, Shiga toxin-producing Escherichia coli, Salmonella, Cryptosporidium spp., C. perfringens α toxin, Giardia spp., Campylobacter jejuni, and Campylobacter coli were also described, although their frequencies were not reported (59, 60, 62).
Co-infection was also observed in animals in studies investigating the association between CanineCV and respiratory diseases. Among CanineCV-positive dogs, nine (52.94%) were co-detected with other pathogens: canine herpesvirus 1 (CaHV-1; n = 2), canine distemper virus (CDV; n = 2), canine respiratory coronavirus (CRCoV; n = 2), canine parainfluenza virus (CPIV; n = 1), canine adenovirus type 2 (CAdV-2; n = 1), and triple-detected with CaHV-1 and CRCoV [n = 1; (29)].
The association between viral load and disease severity has been described in PCV2 infections in swine, where a threshold viral load correlates with clinical signs. There for, 107 or greater PCV2 genomic copies per milliliter of serum were associated with severe PCV2-associated disease (PCVAD), and poor prognosis. Consequently, PCR results are reported as negative, positive with no PCVAD (<106 PCV2 DNA copies), positive with PCVAD suspect (106 PCV2 DNA copies), or positive with PCVAD [107 PCV2 DNA copies or greater; (52)].
In CanineCV infections, viral loads varied between 3.57 × 101 and 8.37 × 108 (30, 54). Another method used to determine viral load in studies was Cycle Threshold (Ct) values, which are inversely proportional to viral load; lower Ct values indicate higher viral loads. Ct values ranged from <13 to 30 (18, 32). Although there is no standardization of viral load and disease severity for CanineCV, Ct < 13 in intestinal samples of three dogs from a study were associated with severe clinical signs such as anorexia, vomiting, and severe bloody diarrhea during outbreaks in a Papillon breeding colony in Michigan in March 2013 and February 2014 (32). The association between viral load and disease severity was also observed, with CanineCV loads generally low, ranging from 3.57 × 101 to 8.37 × 108 (mean of 1.03 × 103) and from 8.60 × 101 to 5.38 × 105 viral DNA copies/μL (mean of 2.45 × 102) for clinical cases and control animals, respectively (54).
Different studies have confirmed the presence of CanineCV in wild carnivores, including wolves, foxes, badgers, and jackals, with wolves being the most studied. These studies are concentrated in Italy and Africa. In wolves, the prevalence of CanineCV varies between 26.4% and 50% of the animals tested, using tissue samples such as intestine and spleen. The overall median quantity of CanineCV DNA was 6.8 × 102 copies of the target DNA per microliter of template [range: 8.2 × 10°−3.7 × 107; (18, 30, 46)].
In wolves, co-infection with CanineCV and other pathogens has been reported in only two studies. One study identified co-infection in 47.8% (11/23) of wolves, with 72.7% (8/11) involving Carnivore protoparvovirus 1 and CanineCV. Additionally, 18.2% (2/11) tested positive for three viruses: one case with Carnivore protoparvovirus 1, CAdV-2, and CanineCV, and another with Carnivore protoparvovirus 1, CAdV-1, and CanineCV (30). Another study found that CanineCV was detected alongside CDV in 77.8% (7/9), CPV-2 in 44.4% (4/9), and Trichinella britovi in 22.2% (2/9) of the cases. Co-infection with two or three agents, in addition to CanineCV, was observed in 22.2% (2/9; CPV-2 + CDV), 11.1% (1/9; CDV + Trichinella britovi), and 22.2% (2/9; CPV-2 + CDV + Trichinella britovi) (46).
In foxes, the prevalence of CanineCV varied from 0% (0/232) to 4.3% (5/115) (17, 66). The viral load ranged from 1.96 to 8.94 × 104 copies of DNA/mL of tissue homogenate (pool of organs or spleen), reflecting variations in viral replication or the stage of infection at the time of sampling. The only CanineCV-positive animal that did not die from trauma (1/5) presented neurological symptoms (46).
The only study involving jackals was conducted on samples (lung and lymph node) collected during predator control operations in 2021, and the prevalence was 18%. Therefore, no clinical signs or diseases were associated with CanineCV infection in this study. The prevalence of CanineCV in jackals was 43.75% [14/32; (15)].
Recently, a novel circovirus was identified in Iberian lynxes (Lynx pardinus), one of the most endangered feline species in the world and a symbol of wildlife conservation in Europe. Study conducted by Castro-Scholten et al. (63) identified the Iberian lynx-associated circovirus-1 (ILCV-1) in 57.8% of spleen samples analyzed, collected from both wild and captive populations. The high positivity rate observed suggests a systemic infection that may have significant implications for the immunological and overall health of this species. Iberian lynxes, which already face substantial challenges due to habitat loss, prey scarcity, and infectious diseases such as bovine tuberculosis and feline leukemia virus, now confront a new potential pathogen that could further complicate conservation efforts (63). The discovery of ILCV-1 highlights the urgent need for additional studies to better understand the epidemiology, clinical impact, and potential transmission mechanisms of this virus, as well as to evaluate management strategies to mitigate the risks associated with its circulation in already vulnerable populations. This identification also broadens our understanding of viral diversity in large felines and underscores the importance of systematic virological investigations in endangered species.
One of the most widely used techniques to detect the CanineCV genome is the Polymerase Chain Reaction [PCR; (14, 20, 24)] particularly real-time quantitative PCR (qPCR). PCR has also been employed for sequencing purposes (14, 15, 25, 29–31, 41, 45, 53, 57, 60).
The systems utilized for qPCR include SYBR Green (18.5%) and Taqman (81.5%). Several studies have utilized qPCR qualitatively due to its ability to be up to 1,000 times more sensitive than traditional PCR, while others have used qPCR to quantify CanineCV DNA in various types of samples (15, 17, 19, 31, 33, 38, 46, 48, 55, 64).
The advantage of qPCR lies in its ability to establish the absolute quantification of viral nucleic acid. As indicated above, the quantification of CanineCV in canine tissues (18, 29, 30, 32, 57), wolves (30), and foxes (45) allows for determining the target tissues for replication that may be related to the virus's pathogenesis. Additionally, the quantification of CanineCV in feces (32, 43, 54, 68) and nasal secretions (29) lays a significant role in understanding viral dissemination (Table 3).
Table 3. Summary of methods and sample types used for CanineCV detection across different species and regions.
The primer sets used in both PCR and qPCR reactions target the cap gene (60) the Rep gene (29, 60), and intergenic region (65). The Rep region and intergenic region, due to their lower mutation rates compared to the cap region, should be considered in diagnostics as they enhance the detection of viruses that may have undergone mutations.
Although several PCR techniques are currently in use, considerable research is still being conducted to standardize and validate these methods to optimize the diagnosis of CanineCV.
Hao et al. (27) developed multiplex PCR (mPCR) method demonstrated superior results compared to traditional PCR techniques, offering simultaneous detection of multiple canine viruses, including canine adenovirus type 2 (CAV-2), canine influenza virus (CIV), CD, CPIV, CanineCV, CCoV, and CPV, with high sensitivity and specificity. The mPCR method's detection limit was established at 1 × 104 viral copies for both respiratory and enteric viruses, significantly enhancing diagnostic accuracy in clinical samples. The ability to detect up to seven different viruses in a single reaction not only streamlines the diagnostic process but also improves the reliability of detecting co-infections. This method, therefore, presents a valuable tool for comprehensive epidemiological surveillance and the rapid, precise diagnosis of canine viral infections.
Still with the aim of diagnosing agents involved in CanineCV co-infections, Wang et al. (36) developed a duplex SYBR Green I-based real-time PCR assay developed for the simultaneous detection of CanineCV and CaAstV demonstrated high sensitivity and specificity. The assay's detection limits were 9.25 × 101 copies/μL for CanineCV and 6.15 × 101 copies/μL for CaAstV, making it significantly more sensitive than traditional PCR methods. The duplex PCR also showed no cross-reactivity with other common canine viruses, such as CPV, CCoV, CDV, and canine kobuvirus (CaKoV), underscoring its specificity. Additionally, the reproducibility of the assay was confirmed through low intra- and inter-assay variation. This method offers a rapid, reliable, and cost-effective tool for detecting co-infections in clinical samples, significantly improving the accuracy of diagnosis in cases where CanineCV and CaAstV are suspected.
Chip digital PCR (cdPCR) is a cutting-edge PCR method that involves encapsulating nanoliter-sized volumes of liquid in high-throughput microcells or microchannels for PCR amplification, followed by direct interpretation of fluorescence signals. This technique allows for the absolute quantification of nucleic acids without the need for external standards, calibration curves, or Ct values. cdPCR excels in precisely detecting and measuring even very small amounts of DNA, making it especially useful for samples with low DNA concentrations or those that contain inhibitors that could interfere with traditional PCR methods. The technique is also known for its high sensitivity and specificity, significantly minimizing the chances of false positives or negatives. This method was used for the detection of CanineCV and exhibited a detection limit of 6.62 copies/μL, making it ~10 times more sensitive than qPCR, which had a detection limit of 6.62 × 101 copies/μL. This increased sensitivity allows for more accurate detection, especially in samples with low viral loads. Furthermore, the cdPCR method showed excellent specificity, with no cross-reactivity observed with other common canine viruses and demonstrated high repeatability with low intra-assay and inter-assay coefficients of variation (36).
Another widely used technique for viral detection is in situ hybridization (ISH), which labels viral DNA within tissue samples, enabling precise localization of the virus. This technique is crucial for identifying target tissues and understanding the lesions associated with viral infections. By determining the exact location of the virus in the tissue, ISH provides valuable insights into the pathogenesis of the infection and its impact on specific tissues. In this technique, the Rep gene has been used as the target for detecting CanineCV DNA within various tissues of infected dogs (29, 32, 59).
In situ hybridization was particularly effective in identifying the presence of viral nucleic acid within specific lymphoid tissues, such as the spleen, mesenteric lymph nodes, and Peyer's patches. The ISH method produced strong positive signals in these lymphoid tissues, especially within epithelioid macrophages located in regions of granulomatous inflammation (32, 59). Additionally, ISH located CanineCV DNA within the pulmonary tissues, notably within the alveolar lining cells, endothelial cells of capillary blood vessels, and lymphoid cells within the follicles of the tracheobronchial lymph nodes. The technique demonstrated high sensitivity, successfully detecting viral DNA within the nuclei and cytoplasm of histiocytes and macrophages. This precise localization of the virus in both lymphoid and pulmonary tissues highlights the direct association between CanineCV and the pathological lesions observed in these areas (69). By providing detailed insights into the specific tissues affected and the cellular localization of the virus, ISH proves to be a critical tool in understanding the pathogenesis of CanineCV infections.
The One Health concept, defined as the collaborative effort of multiple disciplines working locally, nationally, and globally to attain optimal health for people, animals, and the environment, has gained significant recognition. This approach acknowledges the interconnectedness of human, domestic animal, and wildlife health within the broader context of ecosystem health. By providing a holistic framework, One Health facilitates the development of comprehensive solutions to global health challenges. The emergence of infectious diseases, whether novel or known, exemplifies the dynamic interplay between pathogens, hosts, and their environments, highlighting the necessity of an integrated approach to health (70–72).
The proximity between wild and domestic hosts plays a crucial role in the transmission of viruses. As human populations expand and urbanize, the interactions between humans, domestic animals, and wildlife increase, heightening the risk of pathogen transmission and the emergence of novel disease outbreaks. Factors such as wildlife trade and the introduction of domestic species decrease the geographical and behavioral separation between donor and recipient hosts, promoting viral emergence. These interactions create opportunities for cross-host exposures, a critical step in the transference to new hosts, and facilitate the establishment of epidemics by enabling sufficient contact for virus transfer and adaptation (71, 74–76).
RNA and single-stranded DNA (ssDNA) viruses exhibit high mutation and nucleotide substitution rates, allowing rapid evolution and adaptation to new environments. This high variability, coupled with error-prone replication and the lack of a proofreading mechanism, enhances their ability to infect new hosts. For instance, RNA viruses have rapid replication, short generation times, and large populations, which increase the likelihood of adaptation to new hosts. In contrast, most DNA viruses are less variable, often showing virus-host co-speciation. However, ssDNA viruses like Circoviridae can exhibit mutation rates like RNA viruses, suggesting their potential for rapid evolution and cross-species transmission. These rapid evolutionary capabilities are particularly concerning when considering the increased interactions between humans, domestic animals, and wildlife (77–80).
The behavior of CanineCV and PCVs highlights the complexities of viral adaptation and cross-species transmission. PCV3, a member of the Circoviridae family, has been shown to infect multiple hosts, with a high possibility of infecting baboons, demonstrating its capability for trans-species transmission. CanineCV, with its high mutation rate, can adapt to various hosts, like the behavior observed in PCV (9, 24, 25).
Adaptation to interhost transmission by droplet spread, and fecal-oral transmission, which occur with the CanineCV, represent different adaptational challenges due to host differences and variation in environmental exposure, therefore the capacity of the virus in the environment is very important (75). There is no study that shows the viability of CanineCV in the environment, but there is with PCV. PCV2 were detected in wastewater from manure treatment systems consisting of an equalization tank, a settling tank, an anaerobic reactor, an aerobic reactor, and a secondary settling tank, showing its stability in the environment (81). The survival of the virus in the environment is a crucial factor in the spread of the virus and increases the possibility of the virus contacting new hosts.
Another important factor that must be considered is the dog meat feeding habits in some countries. Additionally, serum from these animals may has a high viral load, with a Ct ranging from 28 to 35, making a possible human route of infection, not only through ingestion but also during handling of the animals during slaughter. t should also be noted that depending on the moment of infection, the amount of virus may be even greater, with a Ct range of 13–30 (18, 32).
The detection of CanineCV across these various host species highlights the virus's adaptability and potential for cross-species transmission. Further research is necessary to understand the mechanisms behind this adaptability and the implications for disease management in both domestic and wild animal populations.
In conclusion, CanineCV represents a significant emerging pathogen with the ability to infect various species, including domestic dogs, wild carnivores, and potentially other hosts. The high genetic variability and adaptability of CanineCV, as evidenced by its widespread detection across different regions and host species, underscore the importance of continued surveillance and research. Diagnostic advancements, including the use of techniques such as qPCR, ISH, and multiplex PCR, have significantly enhanced our ability to detect and quantify the virus, thereby improving our understanding of its epidemiology and pathogenicity. These tools, combined with detailed phylogenetic analyses, are crucial in monitoring the virus's evolution and in developing strategies to mitigate its impact on animal health. The observed associations between CanineCV infections and co-infections with other pathogens highlight the complex interplay between the virus and host immune responses, which can exacerbate disease severity.
Therefore, ongoing research into the virus's transmission dynamics, tissue tropism, and interactions with co-infecting agents is essential for developing effective control measures and understanding the broader implications of CanineCV infections for both domestic and wild animal populations.
DS: Investigation, Methodology, Writing – original draft, Writing – review & editing. MC: Investigation, Methodology, Writing – original draft, Writing – review & editing. VL: Investigation, Methodology, Writing – original draft, Writing – review & editing. DM: Writing – original draft. TO: Writing – original draft. AC: Conceptualization, Data curation, Formal analysis, Funding acquisition, Investigation, Methodology, Project administration, Resources, Software, Supervision, Validation, Visualization, Writing – original draft, Writing – review & editing.
The author(s) declare that no financial support was received for the research and/or publication of this article.
The authors declare that the research was conducted in the absence of any commercial or financial relationships that could be construed as a potential conflict of interest.
The author(s) declare that no Gen AI was used in the creation of this manuscript.
All claims expressed in this article are solely those of the authors and do not necessarily represent those of their affiliated organizations, or those of the publisher, the editors and the reviewers. Any product that may be evaluated in this article, or claim that may be made by its manufacturer, is not guaranteed or endorsed by the publisher.
1. Felipetto LG, Fernandes FD, Vogel FSF, Flores EF, Botton SA, Sangioni LA. Demographics and health care profiles of dogs and cats associated with the socioeconomic profile of their tutors in areas assisted by Family Health Strategies in Brazil. Arq Bras Med Vet Zootec. (2022) 74:1007–16. doi: 10.1590/1678-4162-12708
2. Albert A, Bulcroft K. Pets, families, and the life course. J Marr Fam. (1988) 50:543. doi: 10.2307/352019
3. Solhjoo N, Krtalić M, Goulding A. Pets and people: information experience of multispecies families. J Document. (2022) 78:1092–108. doi: 10.1108/JD-03-2021-0052
4. Barker SB, Wolen AR. The benefits of human–companion animal interaction: a review. J Vet Med Educ. (2008) 35:487–95. doi: 10.3138/jvme.35.4.487
5. Queiroz FCBP, Lima NC, da Silva CL, da Silva CL, Queiroz J, de Souza GHS. Purchase intentions for brazilian recycled PET products—circular economy opportunities. Recycling. (2021) 6:75. doi: 10.3390/recycling6040075
6. Defelipe R, Savalli C, Otta E. Demographics and self-reported well-being of Brazilian adults as a function of pet ownership: a pilot study. Heliyon. (2020) 6:e04069. doi: 10.1016/j.heliyon.2020.e04069
7. Mazon MS, De Moura WG. Cachorros e humanos: mercado de rações pet em perspectiva sociológica. Civitas Rev Ciências Sociais. (2017) 17:138. doi: 10.15448/1984-7289.2017.1.25292
8. Kapoor A, Dubovi EJ, Henriquez-Rivera JA, Lipkin WI. Complete genome sequence of the first canine circovirus. J Virol. (2012) 86:7018. doi: 10.1128/JVI.00791-12
9. da Silva RR, da Silva DF, da Silva VH, de Castro AMM. Porcine circovirus 3: a new challenge to explore. Front Vet Sci. (2024) 10:1266499. doi: 10.3389/fvets.2023.1266499
10. Lefkowitz EJ, Dempsey DM, Hendrickson RC, Orton RJ, Siddell SG, Smith DB. Virus taxonomy: the database of the International Committee on Taxonomy of Viruses (ICTV). Nucleic Acids Res. (2018) 46:D708–17. doi: 10.1093/nar/gkx932
11. Rosario K, Breitbart M, Harrach B, Segalés J, Delwart E, Biagini P, et al. Revisiting the taxonomy of the family Circoviridae: establishment of the genus Cyclovirus and removal of the genus Gyrovirus. Arch Virol. (2017) 162:1447–63. doi: 10.1007/s00705-017-3247-y
12. Cheung AK. Identification of an octanucleotide motif sequence essential for viral protein, DNA, and progeny virus biosynthesis at the origin of DNA replication of Porcine circovirus type 2. Virology. (2004) 324:28–36. doi: 10.1016/j.virol.2004.03.037
13. Mankertz A, Persson F, Mankertz J, Blaess G, Buhk HJ. Mapping and characterization of the origin of DNA replication of porcine circovirus. J Virol. (1997) 71:2562–6. doi: 10.1128/jvi.71.3.2562-2566.1997
14. Cardoso BTM, de Deus DR, Sousa EC, Pinheiro KC, da Costa JN, Maués MAC, et al. Molecular analysis of canine circovirus in dogs from animal shelters in Belém, Pará, northern Brazil: first detection at the amazon region. Braz J Vet Med. (2023) 45:e000723. doi: 10.29374/2527-2179.bjvm000723
15. de Villiers L, Molini U, Coetzee LM, Visser L, Spangenberg J, de Villiers M, et al. Molecular epidemiology of canine circovirus in domestic dogs and wildlife in Namibia, Africa. Infect Genet Evol. (2023) 112:105458. doi: 10.1016/j.meegid.2023.105458
16. Kotsias F, Bucafusco D, Nuñez DA, Borisovsky LAL, Rodriguez M, Bratanich AC. Genomic characterization of canine circovirus associated with fatal disease in dogs in South America. PLoS ONE. (2019) 14:e0218735. doi: 10.1371/journal.pone.0218735
17. Ndiana LA, Lanave G, Vasinioti V, Desario C, Martino C, Colaianni ML, et al. Detection and genetic characterization of canine adenoviruses, circoviruses, and novel cycloviruses from wild carnivores in Italy. Front Vet Sci. (2022) 9:851987. doi: 10.3389/fvets.2022.851987
18. Ndiana LA, Lanave G, Desario C, Odigie AE, Madubuike KG, Lucente MS, et al. Detection of selected canine viruses in Nigerian free-ranging dogs traded for meat consumption. Animals. (2023) 13:1119. doi: 10.3390/ani13061119
19. Tuong NM, Piewbang C, Rungsipipat A, Techangamsuwan S. Detection and molecular characterization of two canine circovirus genotypes co-circulating in Vietnam. Vet Q. (2021) 41:232–41. doi: 10.1080/01652176.2021.1967511
20. Piewbang C, Jo WK, Puff C, van der Vries E, Kesdangsakonwut S, Rungsipipat A, et al. Novel canine circovirus strains from Thailand: evidence for genetic recombination. Sci Rep. (2018) 8:7524. doi: 10.1038/s41598-018-25936-1
21. Cheung AK. Porcine circovirus: transcription and DNA replication. Virus Res. (2012) 164:46–53. doi: 10.1016/j.virusres.2011.10.012
22. Faurez F, Dory D, Grasland B, Jestin A. Replication of porcine circoviruses. Virol J. (2009) 6:60. doi: 10.1186/1743-422X-6-60
23. Fehér E, Jakab F, Bányai K. Mechanisms of circovirus immunosuppression and pathogenesis with a focus on Porcine circovirus 2: a review. Vet Q. (2023) 43:1–18. doi: 10.1080/01652176.2023.2234430
24. Gomez-Betancur D, Rendon-Marin S, Giraldo-Ramírez S, Jaime J, Ruiz-Saenz J. Canine circovirus genomic characterization in dogs with and without diarrheal syndrome in Medellín, Colombia. Front Vet Sci. (2023) 10:1204214. doi: 10.3389/fvets.2023.1204214
25. Gomez-Betancur D, Vargas-Bermudez DS, Giraldo-Ramírez S, Jaime J, Ruiz-Saenz J. Canine circovirus: an emerging or an endemic undiagnosed enteritis virus? Front Vet Sci. (2023) 10:1150636. doi: 10.3389/fvets.2023.1150636
26. Mankertz A. Molecular interactions of porcine circoviruses type 1 and type 2 with its host. Virus Res. (2012) 164:54–60. doi: 10.1016/j.virusres.2011.11.001
27. Hao X, Liu R, He Y, Xiao X, Xiao W, Zheng Q, et al. Multiplex PCR methods for detection of several viruses associated with canine respiratory and enteric diseases. PLoS ONE. (2019) 14:e0213295. doi: 10.1371/journal.pone.0213295
28. Xiao X, Li YC, Xu FP, Hao X, Li S, Zhou P. Canine circovirus among dogs and cats in China: first identification in cats. Front Microbiol. (2023) 14:1252272. doi: 10.3389/fmicb.2023.1252272
29. Dankaona W, Nooroong P, Poolsawat N, Srionrod N, Techangamsuwan S, Anuracpreeda P. Molecular characterization of canine circovirus based on the Capsid gene in Thailand. BMC Vet Res. (2024) 20:312. doi: 10.1186/s12917-024-04120-w
30. Balboni A, Urbani L, Delogu M, Musto C, Fontana MC, Merialdi G, et al. Integrated use of molecular techniques to detect and genetically characterise DNA viruses in Italian wolves (Canis lupus italicus). Animals. (2021) 11:2198. doi: 10.3390/ani11082198
31. Beikpour F, Ndiana LA, Sazmand A, Capozza P, Nemati F, Pellegrini F, et al. Detection and genomic characterization of canine circovirus in Iran. Animals. (2022) 12:507. doi: 10.3390/ani12040507
32. Thaiwong T, Wise AG, Maes RK, Mullaney T, Kiupel M. Canine circovirus 1 (CaCV-1) and canine parvovirus 2 (CPV-2). Vet Pathol. (2016) 53:1204–9. doi: 10.1177/0300985816646430
33. Niu L, Wang Z, Zhao L, Wang Y, Cui X, Shi Y, et al. Detection and molecular characterization of canine circovirus circulating in northeastern China during 2014–2016. Arch Virol. (2020) 165:137–43. doi: 10.1007/s00705-019-04433-4
34. Wang Z, Shi Y, Wang Y, Zhao L, Cui X, Wen S, et al. Detection of antibodies against canine circovirus in naturally and experimentally infected canines by recombinant capsid enzyme-linked immunosorbent assay. Front Vet Sci. (2020) 7:294. doi: 10.3389/fvets.2020.00294
35. Sun P, Ye Y, Li Y, Cui Y, Zhou T, Li Y, et al. Establishment of hydrolysis probe system real-time PCR assay for rapid detection of canine circovirus. 3 Biotech. (2021) 11:472. doi: 10.1007/s13205-021-03031-z
36. Wang Y, Li Y, Cui Y, Jiang S, Liu G, Wang J, et al. Establishment of a duplex SYBR green I-based real-time polymerase chain reaction assay for the rapid detection of canine circovirus and canine astrovirus. Mol Cell Probes. (2020) 54:101666. doi: 10.1016/j.mcp.2020.101666
37. Weber MN, Cibulski SP, Olegário JC, da Silva MS, Puhl DE, Mósena ACS, et al. Characterization of dog serum virome from Northeastern Brazil. Virology. (2018) 525:192–9. doi: 10.1016/j.virol.2018.09.023
38. Hsu H-S, Lin T-H, Wu H-Y, Lin LS, Chung CS, Chiou MT, et al. High detection rate of dog circovirus in diarrheal dogs. BMC Vet Res. (2016) 12:116. doi: 10.1186/s12917-016-0722-8
39. Castro AMMG, Cortez A, Heinemann MB, Brandão P, Richtzenhain LJ. Circovírus suíno tipo 2 (PCV-2). Arq Inst Biol. (2007) 74:281–91. doi: 10.1590/1808-1657v74p2812007
40. Duque PR, Silva ASA da, Barros Júnior MR, Sergio AMT, Barbosa CN. Serological survey of Porcine circovirus 2 antibodies in Northeastern Brazil by immunoperoxidase monolayer assay. Semin Cienc Agrar. (2020) 41:345–50. doi: 10.5433/1679-0359.2020v41n1p345
41. Giraldo-Ramirez S, Rendon-Marin S, Vargas-Bermudez DS, Jaime J, Ruiz-Saenz J. First detection and full genomic analysis of canine circovirus in CPV-2 infected dogs in Colombia, South America. Sci Rep. (2020) 10:17579. doi: 10.1038/s41598-020-74630-8
42. Gentil M, Gruber A, Müller E. Nachweishäufigkeit von Dog circovirus bei gesunden und an Durchfall erkrankten Hunden. Tierärztliche Praxis Ausgabe K Kleintiere Heimtiere. (2017) 45:89–94. doi: 10.15654/TPK-160701
43. Cruz TF, Batista TN, Vieira EN, Portela LMF, Baccarin AM, Gradiz JJ, et al. Genomic characterization of Canine circovirus detected in a dog with intermittent hemorrhagic gastroenteritis in Brazil. Ciênc Rural. 50:e20190909. doi: 10.1590/0103-8478cr20190909
44. Wang Y, Noll L, Lu N, Porter E, Stoy C, Zheng W, et al. Genetic diversity and prevalence of Porcine circovirus type 3 (PCV3) and type 2 (PCV2) in the Midwest of the USA during 2016–2018. Transb Emerg Dis. (2020) 67:1284–94. doi: 10.1111/tbed.13467
45. Franzo G, Segalés J. Porcine circovirus 2 (PCV-2) genotype update and proposal of a new genotyping methodology. PLoS ONE. (2018) 13:e0208585. doi: 10.1371/journal.pone.0208585
46. Zaccaria G, Malatesta D, Scipioni G, Di Felice E, Campolo M, Casaccia C, et al. Circovirus in domestic and wild carnivores: an important opportunistic agent? Virology. (2016) 490:69–74. doi: 10.1016/j.virol.2016.01.007
47. Wu J, He J, Tian X, Zhong J, Li H, Sun X. Activation of the Hedgehog pathway promotes recovery of neurological function after traumatic brain injury by protecting the neurovascular unit. Transl Stroke Res. (2020) 11:720–33. doi: 10.1007/s12975-019-00771-2
48. Kwon T, Yoo SJ, Park C-K, Lyoo YS. Prevalence of novel Porcine circovirus 3 in Korean pig populations. Vet Microbiol. (2017) 207:178–80. doi: 10.1016/j.vetmic.2017.06.013
49. Feng C, Wang C, Zhang Y, Du F, Zhang Z, Xiao F, et al. Establishment of a sensitive TaqMan-based real-time PCR assay for Porcine circovirus type 3 and its application in retrospective quarantine of imported boars to China. Vet Med Sci. (2019) 5:168–75. doi: 10.1002/vms3.141
50. Letko M, Seifert SN, Olival KJ, Plowright RK, Munster VJ. Bat-borne virus diversity, spillover and emergence. Nat Rev Microbiol. (2020) 18:461–71. doi: 10.1038/s41579-020-0394-z
51. Ge M, Ren J, Xie Y-L, Zhao D, Fan F-C, Song X-Q, et al. Prevalence and genetic analysis of Porcine circovirus 3 in China from 2019 to 2020. Front Vet Sci. (2021) 8:773912. doi: 10.3389/fvets.2021.773912
52. Opriessnig T, Meng X-J, Halbur PG. Porcine circovirus type 2–associated disease: update on current terminology, clinical manifestations, pathogenesis, diagnosis, and intervention strategies. J Vet Diagn Investig. (2007) 19:591–615. doi: 10.1177/104063870701900601
53. Faraji R, Sadeghi M, Mozhgani S-H, Vasinioti V, Ndiana LA, Desario C, et al. Detection of canine circovirus in dogs infected with canine parvovirus. Acta Trop. (2022) 235:106646. doi: 10.1016/j.actatropica.2022.106646
54. Dowgier G, Lorusso E, Decaro N, Desario C, Mari V, Lucente MS, et al. A molecular survey for selected viral enteropathogens revealed a limited role of canine circovirus in the development of canine acute gastroenteritis. Vet Microbiol. (2017) 204:54–58. doi: 10.1016/j.vetmic.2017.04.007
55. Anderson A, Hartmann K, Leutenegger CM, Proksch AL, Mueller RS, Unterer S. Role of canine circovirus in dogs with acute haemorrhagic diarrhoea. Vet Rec. (2017) 180:542–2. doi: 10.1136/vr.103926
56. da Cruz TF. Padronização e Aplicação da Técnica de ELISA (Enzyme-Linked Immunosorbent Assay) Indireto com Anticorpo de Captura para a Detecção de Anticorpos contra o Circovírus Suíno Tipo 2. Universidade Estadual Paulista da Faculdade de Medicina Veterinária e Zootecnia de Botucatu, 2010 (2010).
57. Decaro N, Martella V, Desario C, Lanave G, Circella E, Cavalli A, et al. Genomic characterization of a circovirus associated with fatal hemorrhagic enteritis in dog, Italy. PLoS ONE. (2014) 9:e105909. doi: 10.1371/journal.pone.0105909
58. Van Kruiningen HJ, Heishima M, Kerr KM, Garmendia AE, Helal Z, Smyth JA. Canine circoviral hemorrhagic enteritis in a dog in Connecticut. J Vet Diagn Investig. (2019) 31:732–6. doi: 10.1177/1040638719863102
59. Lv Z-H, Lian C-Y, Li C-L, Chui W-T, Yao X-Y, Shao J-W, et al. Epidemiology and genotypic diversity of canine circovirus identified in pet dogs in Harbin, China. Acta Trop. (2023) 245:106978. doi: 10.1016/j.actatropica.2023.106978
60. Li L, McGraw S, Zhu K, Leutenegger CM, Marks SL, Kubiski S, et al. Circovirus in tissues of dogs with vasculitis and hemorrhage. Emerg Infect Dis. (2013) 19:534–41. doi: 10.3201/eid1904.121390
61. Delling C, Daugschies A. Literature review: coinfection in young ruminant livestock—Cryptosporidium spp. and its companions. Pathogens. (2022) 11:103. doi: 10.3390/pathogens11010103
62. Wang Y, Noll L, Porter E, Stoy C, Dong J, Anderson J, et al. Development of a differential multiplex real-time PCR assay for Porcine circovirus type 2 (PCV2) genotypes PCV2a, PCV2b and PCV2d. J Virol Methods. (2020) 286:113971. doi: 10.1016/j.jviromet.2020.113971
63. Castro-Scholten S, Vasinioti VI, Caballero-Gómez J, García-Bocanegra I, Pellegrini F, Salvaggiulo A, et al. Identification and characterization of a novel circovirus in Iberian lynx in Spain. Res Vet Sci. (2024) 176:105336. doi: 10.1016/j.rvsc.2024.105336
64. Mortari APG, Masuda EK, Flores MM, Flores EF, Cargnelutti JF, Vogel FSF. Coinfection with canine distemper virus and canine circovirus in a dog in Brazil. Braz J Microbiol. (2022) 53:2315–20. doi: 10.1007/s42770-022-00803-4
65. Opriessnig T, Karuppannan AK, Halbur PG, Calvert JG, Nitzel GP, Matzinger SR, et al. Porcine circovirus type 2a or 2b based experimental vaccines provide protection against PCV2d/porcine parvovirus 2 co-challenge. Vaccine. (2020) 38:1975–81. doi: 10.1016/j.vaccine.2020.01.013
66. Franzo G, Drigo M, Legnardi M, Grassi L, Menandro ML, Pasotto D, et al. Porcine gammaherpesviruses in Italian commercial swine population: frequent but harmless. Pathogens. (2021) 10:47. doi: 10.3390/pathogens10010047
67. Sindicato Nacional Da Indústria De Produtos Para Saúde Animal (SINDAN). Annual Report Sindan 2020. São Paulo: SINDAN (2020). Available at: https://www.sindan.org.br/wp-content/uploads/2021/02/RELATORIO-SINDAN-2020.pdf (Accessed January 26, 2025).
68. Balboni A, Terrusi A, Urbani L, Troia R, Stefanelli SAM, Giunti M, et al. Canine circovirus and Canine adenovirus type 1 and 2 in dogs with parvoviral enteritis. Vet Res Commun. (2022) 46:223–32. doi: 10.1007/s11259-021-09850-y
69. Dankaona W, Mongkholdej E, Satthathum C, Piewbang C, Techangamsuwan S. Epidemiology, genetic diversity, and association of canine circovirus infection in dogs with respiratory disease. Sci Rep. (2022) 12:15445. doi: 10.1038/s41598-022-19815-z
70. Gummow B. Challenges posed by new and re-emerging infectious diseases in livestock production, wildlife and humans. Livest Sci. (2010) 130:41–6. doi: 10.1016/j.livsci.2010.02.009
71. Jones KE, Patel NG, Levy MA, Storeygard A, Balk D, Gittleman JL, et al. Global trends in emerging infectious diseases. Nature. (2008) 451:9903. doi: 10.1038/nature06536
72. Sleeman JM, DeLiberto T, Nguyen N. Optimization of human, animal, and environmental health by using the One Health approach. J Vet Sci. (2017) 18:263–8. doi: 10.4142/jvs.2017.18.S1.263
73. Hall S, Dolling L, Bristow K, Fuller T, Mills D. Companion Animal Economics: The Economic Impact of Companion Animals in the UK. Research report (2017).
74. Morse SS. Factors in the emergence of infectious diseases. Emerg Infect Dis. (1995) 1, 7–15. doi: 10.3201/eid0101.950102
75. Parrish CR, Holmes EC, Morens DM, Park EC, Burke DS, Calisher CH, et al. Cross-species virus transmission and the emergence of new epidemic diseases. Microbiol Mol Biol Rev. (2008) 72:457–70. doi: 10.1128/MMBR.00004-08
76. Wolfe ND, Daszak P, Kilpatrick AM, Burke DS. Bushmeat hunting, deforestation, and prediction of zoonoses emergence. Emerg Infect Dis. (2005) 11:1822–7. doi: 10.3201/eid1112.040789
77. Faillace CA, Lorusso NS, Duffy S. Overlooking the smallest matter: viruses impact biological invasions. Ecol Lett. (2017) 20:524–38. doi: 10.1111/ele.12742
78. Longdon B, Brockhurst MA, Russell CA, Welch JJ, Jiggins FM. The evolution and genetics of virus host shifts. PLoS Pathog. (2014) 10:e1004395. doi: 10.1371/journal.ppat.1004395
79. McCarthy J. From here to human-level AI. Artif Intellig. (2007) 171:1174–82. doi: 10.1016/j.artint.2007.10.009
80. Sanjuán R, Nebot MR, Chirico N, Mansky LM, Belshaw R. Viral mutation rates. J Virol. (2010) 84:9733–48. doi: 10.1128/JVI.00694-10
81. Viancelli A, Garcia LA, Kunz A, Steinmetz R, Esteves PA, Barardi CR. Detection of circoviruses and porcine adenoviruses in water samples collected from swine manure treatment systems. Res Vet Sci. (2012) 93:538–43. doi: 10.1016/j.rvsc.2011.07.022
Keywords: CanineCV, Circoviridae, Porcine circovirus, public health, emerging, pig disease
Citation: Silva DFd, Ciola M, Lopes VdO, Matias DRM, Oliveira TS and Castro AMMGd (2025) Canine circovirus: emergence, adaptation, and challenges for animal and public health. Front. Vet. Sci. 12:1535650. doi: 10.3389/fvets.2025.1535650
Received: 27 November 2024; Accepted: 13 January 2025;
Published: 28 March 2025.
Edited by:
Joel Fernando Soares Filipe, University of Milan, ItalyReviewed by:
Gianvito Lanave, University of Bari Aldo Moro, ItalyCopyright © 2025 Silva, Ciola, Lopes, Matias, Oliveira and Castro. This is an open-access article distributed under the terms of the Creative Commons Attribution License (CC BY). The use, distribution or reproduction in other forums is permitted, provided the original author(s) and the copyright owner(s) are credited and that the original publication in this journal is cited, in accordance with accepted academic practice. No use, distribution or reproduction is permitted which does not comply with these terms.
*Correspondence: Diego Ferreira da Silva, ZmVycmVpcmEuZGllZ29AdXNwLmJy
Disclaimer: All claims expressed in this article are solely those of the authors and do not necessarily represent those of their affiliated organizations, or those of the publisher, the editors and the reviewers. Any product that may be evaluated in this article or claim that may be made by its manufacturer is not guaranteed or endorsed by the publisher.
Research integrity at Frontiers
Learn more about the work of our research integrity team to safeguard the quality of each article we publish.