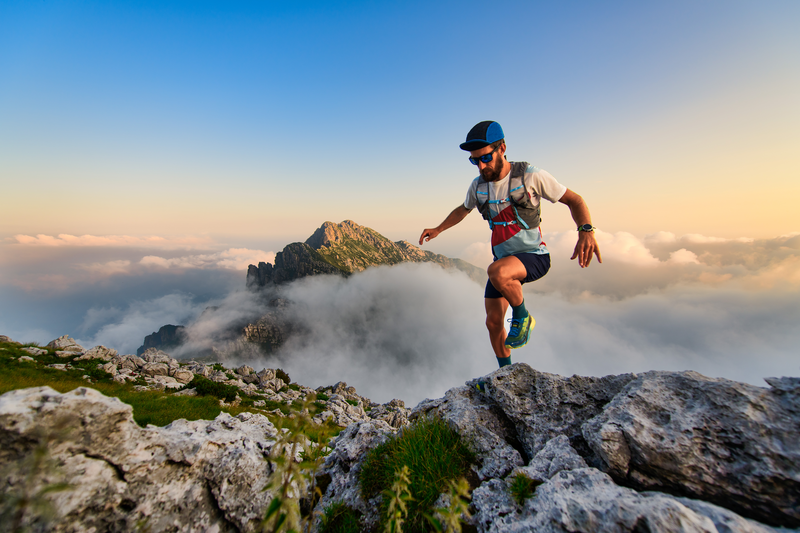
94% of researchers rate our articles as excellent or good
Learn more about the work of our research integrity team to safeguard the quality of each article we publish.
Find out more
ORIGINAL RESEARCH article
Front. Vet. Sci.
Sec. Animal Nutrition and Metabolism
Volume 12 - 2025 | doi: 10.3389/fvets.2025.1511759
The final, formatted version of the article will be published soon.
You have multiple emails registered with Frontiers:
Please enter your email address:
If you already have an account, please login
You don't have a Frontiers account ? You can register here
Coccidiosis is a significant disease with economic implications. It causes high mortality and morbidity, often associated with weight loss. This study investigated the effects of ginger supplementation on antioxidant status, biochemical parameters, and intestinal tissue histopathology in experimentally induced coccidiosis. One hundred one-day-old broiler chicks (Ross) were purchased from a local hatchery. Birds were kept in a clean, well ventilated and disinfected shed. The birds were given non-pelleted diets and water ad libitum throughout the period of the study (25 days). The aqueous ginger was administered orally to the chicks of the four dietary groups via drinking water at concentrations of 6 gm /L of water. All biosecurity measures were adopted according to standard protocol. The chicks were allocated into five groups: control negative (CN), control positive (CP) was infected with 3 x 104 sporulated oocysts at day 14th of the experiment, the third group (GO) was given ginger only for 25 days, the fourth group (GB) was given ginger from day 4 th to day 25 th of the experiment, and the fifth group (GA) was given ginger from day 5 th post infection to day 25 th of the experiment. Ginger extract treatment reduced the fecal oocyst count in the infected group, particularly on day 10 post-infection. The alterations in differential leukocyte counts due to coccidial infection were ameliorated by ginger extract administration. Biochemically, compared to the infected group, ginger (Zingiber officinale) reversed the altered biochemical parameters (total protein, albumin, MDA, and SOD enzyme) associated with cecal coccidiosis. Immunologically, ginger extract treatment increased CD4 T cell counts and overexpression of INF-γ in the cecal epithelium. Histological examination revealed a significant reduction in goblet cell number and a decrease in the villus height (VH) to crypt depth (CD) ratio in infected chicks. Restoration of normal cecal histological structure and increased absorptive function and goblet cell number were observed in ginger-treated chicks compared to control-positive chicks. In conclusion, ginger supplements have effective therapeutic uses against intestinal coccidiosis as shown in the biochemical, immunohistochemical and histopathological results.
Keywords: Amelioration, Coccidia, Ginger (Zingiber officinale), Immunity, Histopathology ETHICAL APPROVAL
Received: 15 Oct 2024; Accepted: 14 Feb 2025.
Copyright: © 2025 Saber, Mawas, Khalil, Ahmed, Shibat Elhamd, Ali, Rehan, Zigo and Salah. This is an open-access article distributed under the terms of the Creative Commons Attribution License (CC BY). The use, distribution or reproduction in other forums is permitted, provided the original author(s) or licensor are credited and that the original publication in this journal is cited, in accordance with accepted academic practice. No use, distribution or reproduction is permitted which does not comply with these terms.
* Correspondence:
Ibrahim F. Rehan, Department of Husbandry and Development of Animal Wealth, Faculty of Veterinary Medicine, Menoufia University, Shebin Alkom, Menoufia, 32511, Egypt
Disclaimer: All claims expressed in this article are solely those of the authors and do not necessarily represent those of their affiliated organizations, or those of the publisher, the editors and the reviewers. Any product that may be evaluated in this article or claim that may be made by its manufacturer is not guaranteed or endorsed by the publisher.
Research integrity at Frontiers
Learn more about the work of our research integrity team to safeguard the quality of each article we publish.