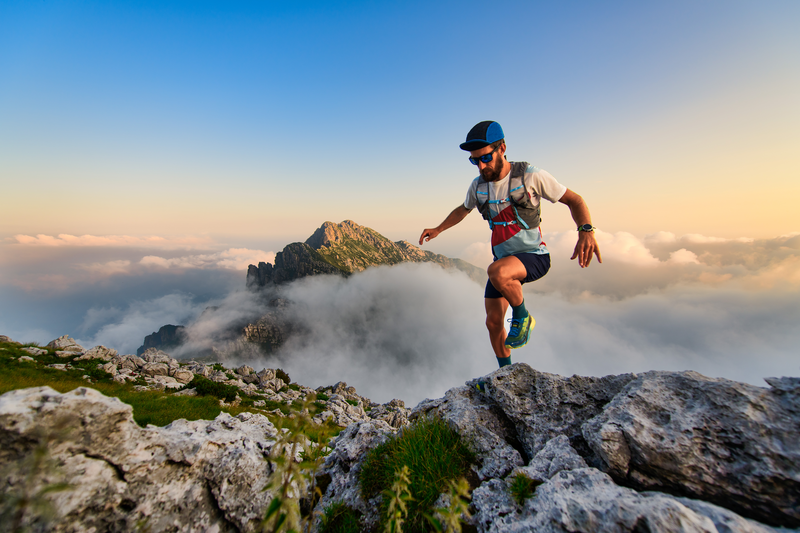
94% of researchers rate our articles as excellent or good
Learn more about the work of our research integrity team to safeguard the quality of each article we publish.
Find out more
ORIGINAL RESEARCH article
Front. Vet. Sci.
Sec. Veterinary Epidemiology and Economics
Volume 12 - 2025 | doi: 10.3389/fvets.2025.1481822
This article is part of the Research Topic Antimicrobial Resistance in Veterinary Medicine: Epidemiology, Economic Impact, and Mitigation Strategies View all 4 articles
The final, formatted version of the article will be published soon.
You have multiple emails registered with Frontiers:
Please enter your email address:
If you already have an account, please login
You don't have a Frontiers account ? You can register here
Fosfomycin (FOS) is a critical antibiotic for treating multi-drug resistant (MDR) Enterobacteriaceae infections, but its effectiveness is jeopardized by the dissemination of plasmids encoding enzymes that modify FOS. Despite the prohibition on its use in animal breeding in China, 100 strains of Escherichia coli (E. coli) exhibiting high resistance to FOS (MIC≥512 mg/L) were isolated from samples of waterfowl origin collected in Hainan, Sichuan, and Anhui. These strains commonly carried the fosA3 (88/100, 88.0%). In addition, 21 other antimicrobial resistance genes (ARGs) were detected in these strains, with high positivity rates for tetA, aphA1, sul2, folR, qnrS and bla CTX-M . It is noteworthy that there was a significant positive correlation between the fosA3 and bla CTX-M (OR=15.162, 95% CI: 1.875-122.635). The results of pulsed-field gel electrophoresis (PFGE) demonstrated the existence of multiple dispersed clonal clusters. Multilocus sequence typing (MLST) analysis identified 45 ST types, with ST48 and ST10 representing the most dominant clones. In the conjugation experiments, 49 fosA3-positive transconjugants were obtained with a conjugation frequency as high as 1.1×10 -1 , which strongly demonstrated that the fosA3 was mainly located on the plasmid and possessed an efficient transmission ability. Whole genome sequencing (WGS) analysis further showed that the fosA3 was co-localised with the bla CTX-M on plasmids that showed a high degree of similarity in genetic structure. Of particular interest is the observation that the fosA3 is frequently accompanied by IS26 on either side of the gene. This structure may play a pivotal role in the horizontal transfer of the fosA3. The study revealed the alarming prevalence of FOS resistance in E. coli of waterfowl origin and delved deeply into the genetic characteristics and transmission mechanisms of the fosA3. Detection of plasmid-mediated, transmissible FOS resistance in waterfowl E. coli poses a threat to "One Health". Urgent need for comprehensive resistance monitoring and control strategies.
Keywords: waterfowl, Escherichia coli, Fosfomycin resistance, fosA3, Plasmid -mediated
Received: 16 Aug 2024; Accepted: 31 Jan 2025.
Copyright: © 2025 Zhang, Yang, Yang, Li, Zhong, Wang, Jia, Chen, Liu, Zhu, Zhao, Wu, Yang, Huang, Ou, Sun, Tian, Wu, He and Cheng. This is an open-access article distributed under the terms of the Creative Commons Attribution License (CC BY). The use, distribution or reproduction in other forums is permitted, provided the original author(s) or licensor are credited and that the original publication in this journal is cited, in accordance with accepted academic practice. No use, distribution or reproduction is permitted which does not comply with these terms.
* Correspondence:
Shaqiu Zhang, Sichuan Agricultural University, Ya'an, China
Anchun Cheng, Sichuan Agricultural University, Ya'an, China
Disclaimer: All claims expressed in this article are solely those of the authors and do not necessarily represent those of their affiliated organizations, or those of the publisher, the editors and the reviewers. Any product that may be evaluated in this article or claim that may be made by its manufacturer is not guaranteed or endorsed by the publisher.
Research integrity at Frontiers
Learn more about the work of our research integrity team to safeguard the quality of each article we publish.