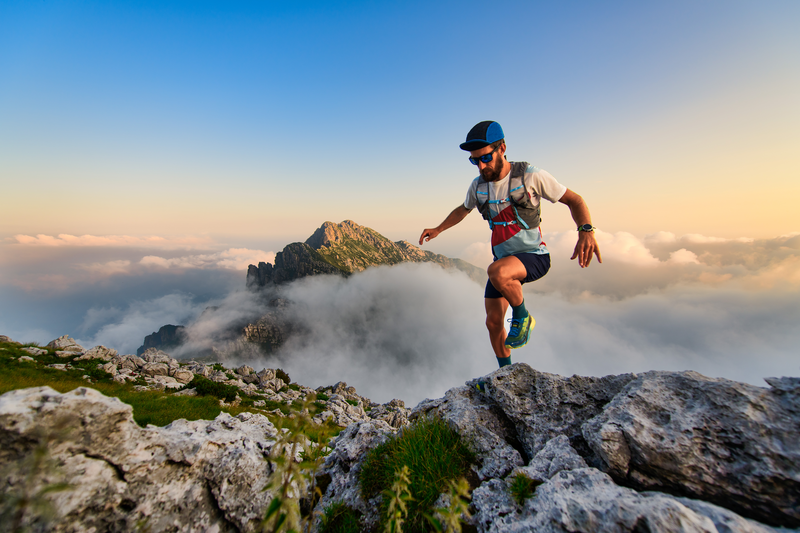
94% of researchers rate our articles as excellent or good
Learn more about the work of our research integrity team to safeguard the quality of each article we publish.
Find out more
ORIGINAL RESEARCH article
Front. Vet. Sci.
Sec. Animal Reproduction - Theriogenology
Volume 12 - 2025 | doi: 10.3389/fvets.2025.1454343
The final, formatted version of the article will be published soon.
You have multiple emails registered with Frontiers:
Please enter your email address:
If you already have an account, please login
You don't have a Frontiers account ? You can register here
Determination of the ovarian follicular reserve is of great value for predicting fertility, while circulating anti-Müllerian hormone (AMH) plays an important role in maintaining the ovarian reserve. In this study, we examined the effect of circulating AMH levels of gilts between 110 and 160 days of age on reproductive performance and evaluated the differences in the ovaries and uteruses of gilts with different AMH levels. The results indicated a significant negative correlation between circulating AMH levels from days 110 to 160 and puberty (P < 0.05). Moreover, the total born, live born and the herd retention rate of across 3 successive parities was found higher in high-AMH gilts than in low-AMH ones. Uterine morphology was assessed and it was found that high-AMH gilts had significantly increased uterine glandular density, and the median vascular area and the relative expression levels of FOXA2, VEGF, VEGFR, and CD31 were significantly increased (P < 0.05). Furthermore, we also found that high-AMH gilts had a greater number of antral follicles and higher expression level in secondary and early antral follicles than low-AMH gilts (P < 0.05).To further explain this mechanism, RNA-seq analysis was performed, which indicated that DEGs of high-AMH gilt ovaries were enriched in pathways, including steroid hormone biosynthesis, arachidonic acid metabolism, and the gonadotropin-releasing hormone signaling pathway. Our findings indicate that circulating AMH levels can possibly predict the reproductive potential of gilts, day 160 circulating AMH levels is a potential predictive indicator.
Keywords: Anti-Mullerian Hormone, Puberty, Reproductive performance, Retention rate, GILT
Received: 25 Jun 2024; Accepted: 08 Jan 2025.
Copyright: © 2025 Zhou, Xia, Linghua, Fang, Tao, Qi, Yu, Zhang, Tian, Gao, Bai, Wen, Liu and Li. This is an open-access article distributed under the terms of the Creative Commons Attribution License (CC BY). The use, distribution or reproduction in other forums is permitted, provided the original author(s) or licensor are credited and that the original publication in this journal is cited, in accordance with accepted academic practice. No use, distribution or reproduction is permitted which does not comply with these terms.
* Correspondence:
Yatian Qi, Hebei Agricultural University, Baoding, China
Yang Yu, Hebei Agricultural University, Baoding, China
Di Zhang, Hebei Agricultural University, Baoding, China
Zihao Gao, Hebei Agricultural University, Baoding, China
Jiahua Bai, Institute of Animal Husbandry and Veterinary Medicine, Beijing Academy of Agriculture and Forestry Sciences, Beijing, China
Junhui Wen, Institute of Animal Husbandry and Veterinary Medicine, Beijing Academy of Agriculture and Forestry Sciences, Beijing, China
Junjie Li, Hebei Agricultural University, Baoding, China
Disclaimer: All claims expressed in this article are solely those of the authors and do not necessarily represent those of their affiliated organizations, or those of the publisher, the editors and the reviewers. Any product that may be evaluated in this article or claim that may be made by its manufacturer is not guaranteed or endorsed by the publisher.
Research integrity at Frontiers
Learn more about the work of our research integrity team to safeguard the quality of each article we publish.