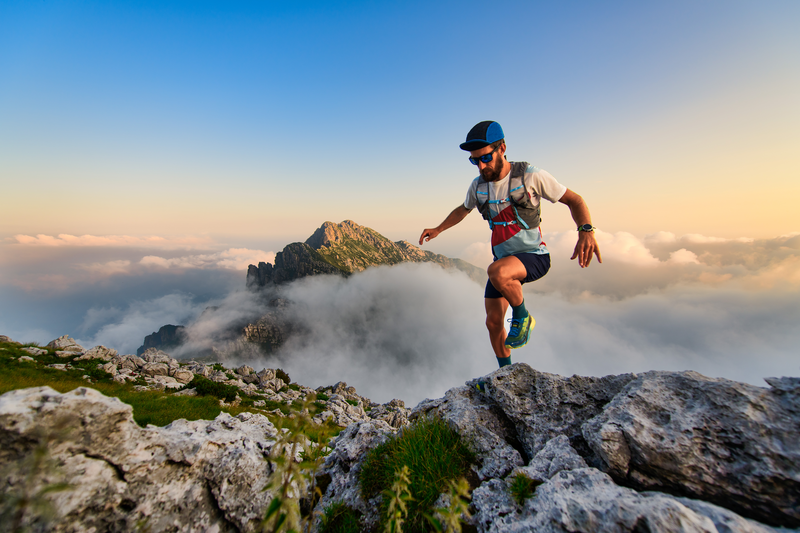
95% of researchers rate our articles as excellent or good
Learn more about the work of our research integrity team to safeguard the quality of each article we publish.
Find out more
ORIGINAL RESEARCH article
Front. Vet. Sci. , 06 January 2025
Sec. Animal Nutrition and Metabolism
Volume 11 - 2024 | https://doi.org/10.3389/fvets.2024.1523062
This article is part of the Research Topic Crosslinking of feed nutrients, microbiome and production in ruminants View all 16 articles
The aim of this experiment was to investigate the effects of intravenous infusion of lipopolysaccharide (LPS) and feeding different ratios of lysine (Lys) and methionine (Met) on feed intake, apparent digestibility, rumen fermentation and microorganisms in young Holstein bulls. Five seven-month-old Holstein bulls with similar body weights (279 ± 42 kg) were selected and subjected to a 5 × 5 Latin square experiment. The control group (CON) was fed with basal diet and the ratio of Lys to Met in the diet was adjusted to 3.0: 1. The experimental groups were received LPS infusion while being fed the basal diet (TRT1), along with LPS infusion and the addition of rumen-protected lysine (RPL) and rumen-protected methionine (RPM) to make the ratio of Lys to Met to 2.5:1 (TRT2), 3.0:1 (TRT3) and 3.5: 1 (TRT4), respectively. The LPS jugular infusion dose was set at 0.01 μg/kg body weight on days 1–3 and 0.05 μg/kg body weight on days 4–7. The trial was conducted over five periods, consisting of a 7-day trial period and a 6-day interval. The results indicated that there were no significant effects of LPS infusion on feed intake and apparent digestibility in young Holstein bulls fed different ratios of Lys and Met (p > 0.05). The treatment had no significant effects on the pH and total volatile fatty acids (p > 0.05). Compared with CON, the acetate content in the experimental groups exhibited an increasing trend (p = 0.066), while the content of NH3-N decreased significantly (p < 0.05). LPS infusion had no significant effect on rumen microorganisms at either the species or phylum level (p > 0.05). However, feeding different ratios of Lys and Met could significantly increasing the abundance of Oribacterium (p < 0.05) and tended to increase the abundance of norank_f__norank_o__RF_39 at the genus level (p = 0.087). These findings suggest that adding RPL and RPM into the diet may enhance the rumen environment in young Holstein bulls. Under the conditions of this experiment, adding RPL and RPM can mitigate the negative effects associated with LPS infusion, with an optimal ratio of Lys and Met is 3.0:1.
Lipopolysaccharide (LPS) is a common substance that induces inflammation, which can lead to weakened immunity, decreased feed intake, and substandard product quality in livestock and poultry. In the process of protein digestion and utilization of dairy cattle, the rumen characteristics lead to low protein utilization efficiency (1). Amino acids (AA) are the fundamental building blocks of proteins, and the essence of protein nutrition lies in amino acid nutrition. By reducing the protein level in the diet, an appropriate amount of AA can be provided to effectively meet the growth requirements of dairy cattle (2). The overall concentration of AA in the body is strictly regulated through transport, metabolism, and protein biosynthesis/degradation. Organs work together to achieve systemic amino acid homeostasis, and carried out through intestinal absorption and peptides and renal reabsorption. The liver metabolizes AA and muscles participate in protein biosynthesis and degradation, and the nervous and endocrine systems regulate this transport and metabolism (3). Host-rumen microbiota interaction is an important component and complex ecosystem of many physiological processes. Ruminant can consume large amounts of forage and convert it into products, mainly relying on some bacteria in the rumen to secrete cellulase, which could decompose partly cellulose as an energy source for the body (4).
Lysine (Lys) and methionine (Met) are the two primary limiting essential AA for ruminant growth, playing a crucial role in supporting gastrointestinal function and the gut-related immune system. Met promotes the growth and development of immune organs, enhances the proliferation of immune cells, and facilitates the synthesis of immune-related compounds, thereby regulating the body’s immune response (5). Lys induces antioxidant enzymes to prevent oxidative stress through the Nrf2 pathway, improves the repair capabilities of proteins and DNA, and enhances the cellular redox state of intestinal cells, thus bolstering the body’s antioxidant capacity (6). Junior et al. (7) found the supplementation of rumen-protected methionine (RPM) could enhance the performance of dairy cows during peak lactation by increasing amino acid metabolism. It has been reported that RPM supplementation could alleviate heat stress (8). Moreover, the addition of Lys significantly improved immune function in dairy cows (9). Although RPM has been rumen protected, adding RPM could enhance the rumen environment of cows (10). Therefore, the purpose of this study is to evaluate the effects of lipopolysaccharide (LPS) infusion on feed intake, apparent digestibility, rumen fermentation and microorganisms in young Holstein bulls fed diets with different ratios of Lys and Met. This experiment can provide theoretical support for adjusting the amino acid structure of the diet to alleviate stress in dairy cows, which is of great significance for practical production.
This study was conducted from October to December 2023 in a dairy farm and approved by the Experimental Animal Ethics Committee of Zhejiang A&F University (ZAFUAC202448). Five seven-month-old Holstein bulls with similar body weights (279 ± 42 kg) were selected for the study. The dietary composition and nutritional components are shown in Table 1. A 5 × 5 Latin square design was employed for the experiment. The control group (CON) was fed a basal diet with Lys to Met ratio of 3.0:1, and the experimental groups received the with basal diet and infused LPS (TRT1), infused LPS and added rumen-protected lysine (RPL) and RPM in the diet, resulting in ratios of 2.5:1 (TRT2), 3.0:1 (TRT3), and 3.5:1 (TRT4), respectively. LPS (E. coli O111:B4, L2630, 297–473-0) was sourced from Sigma-Aldrich. RPL and RPM were obtained from Kemin Industries, Inc. The rumen absorption rates of RPL and RPM are 65% and 50%, respectively, while the small intestine absorption rate is greater than 80%. To avoid excessive drug stimulation, the infusion was adjusted from a low dose to a high dose. The concentration of LPS solution infused into the jugular vein was 0.01 μg/kg BW on days 1–3 and 0.05 μg/kg BW on days 4–7. The trial was conducted over five periods, each lasting 13 days, which included a 7-day experimental period followed by a 6-day rest period. During both the rest and experimental periods, the bulls were fed twice daily and had unrestricted access to water.
On the day before the start of each period, 1 L of infusion solution was prepared according to their weight and stored at 4°C. The solution was allowed to return to room temperature before infusion. A constant flow pump was used for the infusion, which lasted approximately 6 h. The bulls were arranged in order, and a central venous catheter was inserted into the jugular vein. The catheters were flushed with saline before infusion and promptly sealed with heparin sodium to prevent blockage due to blood coagulation. The surgical site for each trial was not on the same side as the previous one, allowing sufficient time for wound recovery.
Each bull was independently fed twice a day, the remaining feed was weighed, the daily feed intake of each bull was calculated, and the feed intake of each bull was calculated according to the treatment. On the last 3 days of each period, spot collection of feces every 6 h. The first collection on the second day would be collected 2 h in advance and 4 h in advance on the third day. Fecal samples would be pooled by periods and bulls. The feces were then mixed according to the cattle at the end of each period and stored at −80°C. After a 7-day experimental phase, 50 mL of rumen fluid was collected before morning feeding, discarding the first 200 mL to avoid saliva contamination. The rumen fluid was filtered through four layers of sterile gauze, and the pH was immediately measured. The sample was divided into 2 mL centrifuge tubes and stored at −80°C for microbiological testing. The rumen microbiota was analyzed by Shanghai Meiji Biomedical Technology Co., Ltd.
Total microbial genomic DNA was extracted using the E.Z.N.A.® soil DNA Kit (Omega Bio-tek, Norcross, GA, U.S.) according to manufacturer’s instructions. The quality and concentration of DNA were determined by 1.0% agarose gel electrophoresis and a NanoDrop2000 spectrophotometer (Thermo Scientific Inc., USA). The hypervariable region V3-V4 of the bacterial 16S rRNA gene were amplified with primer pairs338F (5’-ACTCCTACGGGAGGCAGCAG-3′) and 806R (5’-GGACTACHVGGGTWTCTAAT-3′), using the following conditions: 3 min of denaturation at 95°C, 27 cycles of 30 s at 95°C, 30 s of annealing at 55°C, and 45 s of elongation at 72°C, and a final extension for 10 min at 72°C (11, 12). Each PCR experiment used a 20-μL reaction mixture containing 4 μL of 5 × FastPfu buffer, 2 μL of 2.5 mM deoxynucleoside triphosphates, 0.8 μL of each primer (5 M), 0.4 μL of FastPfu polymerase, and 10 ng of template DNA. The PCR product was extracted from 2% agarose gel and purified using the PCR Clean-Up Kit (YuHua, Shanghai, China) according to manufacturer’s instructions and quantified using Qubit 4.0 (Thermo Fisher Scientific, USA). Purified amplicons were pooled in equimolar amounts and paired-end sequenced on an Illumina Nextseq2000 platform (Illumina, San Diego, USA) according to the standard protocols by Majorbio Bio-Pharm Technology Co. Ltd. (Shanghai, China).
Rumen fluid was centrifuged at 500 × g at 4°C for 15 min. Subsequently, 3 mL of the supernatant was mixed with 1 mL of 77.6% trichloroacetic acid solution, vortex-mixed, and divided into two portions. One placed in an ice bath for 45 min and the other centrifuged at 27,000 × g for 15 min. Microbial protein (MCP) was measured using the Coomassie brilliant blue method with a multifunctional enzyme-labeled instrument (Tecan Infinite 200Pro, Tecan Group, Switzerland) at a wavelength of 595 nm. The collected rumen fluid was centrifuged at 1,000 × g at 4°C for 10 min. 1 mL of the supernatant was transferred into a centrifuge tube, and 0.1 mL of 25% metaphosphate was added. The mixture was then placed in an ice bath for 30 min, followed by centrifugation at 1,000 × g at 4°C for 15 min. The supernatant was analyzed for acetate, propionate, butyrate, isobutyrate, valerate, and isovalerate using a gas chromatograph (6,890 N, Agilent, USA). The concentration of NH3-N in rumen fluid was determined using indophenol colorimetry (13).
The contents of dry matter (DM) and crude protein (CP) in both feed and feces were determined using the AOAC method (1990) (14). Additionally, the levels of acid detergent fiber (ADF) and neutral detergent fiber (NDF) were assessed following the method established by Van Soest et al. (15).
The feed intake, apparent digestibility, rumen fermentation and microbial composition were all sorted by Excel 2022. Feed intake, apparent digestibility, rumen fermentation indexes and microbial composition were analyzed by PROC GLM program in SAS9.4 statistical software. Treatment and period were fixed effects in the model, while bulls were random effects. Test results are presented as mean and mean standard error (SEM). p < 0.05 indicated significant difference, and 0.05 ≤ p < 0.10 indicated trend of difference.
The effects of LPS infusion on feed intake of young Holstein bulls fed diets with different ratios of Lys and Met is shown in Table 2. During the whole period, treatment had no significant effect on feed intake of young Holstein bulls (p > 0.05).
Table 2. Effect of lipopolysaccharide infusion on feed intake of young Holstein bulls fed diets with different ratios of Lys and Met.
Effect of LPS infusion on apparent digestibility of nutrients in young Holstein bulls fed diets with different ratios of Lys and Met are shown in Table 3. Treatment had no significant effect on the apparent digestibility of DM, CP, NDF and ADF among groups (p > 0.05).
Table 3. Effects of lipopolysaccharide infusion on apparent digestibility of young Holstein bulls fed diets with different ratios of Lys and Met.
Effects of LPS infusion on rumen fermentation in young Holstein bulls fed diets with different ratios of Lys and Met are shown in Table 4. The NH3-N content of CON and TRT1 was significantly higher than that of TRT3 and TRT4 (p < 0.05), and it is the lowest in TRT3. During the whole experimental period, the acetate content in the experimental group showed an increasing trend (p = 0.066) with the highest content in the TRT3, and the treatment had no significant effect on the pH, MCP, total volatile fatty acids (TVFAs), propionate, isobutyrate, butyrate, isovalerate, valerate and the ratio of acetate and propionate of rumen fluid (p > 0.05).
Table 4. Effects of lipopolysaccharide infusion on rumen fermentation of young Holstein bulls fed diets with different ratios of Lys and Met.
As shown in Figure 1, rumen microbial dilution correlation curve tended to be stable when the measured effective sequence number reached 22,000, indicating that the data amount was reasonable.
Figure 1. Sample dilution curve in different groups. CON = basal diet, the ratio of Lys to Met is 3.0:1; TRT1 = LPS infusion and basal diet, the ratio of Lys to Met is 3.0:1; TRT2 = LPS infusion, basal diet added rumen-protected amino acids, the ratio of Lys to Met is 2.5:1; TRT3 = LPS infusion, basal diet added rumen-protected amino acids, the ratio of Lys to Met is 3.0:1; TRT4 = LPS infusion, basal diet added rumen-protected amino acids, the ratio of Lys to Met is 3.5:1.
A total of 25 rumen samples were collected for DNA extraction and 16S rRNA sequencing. As shown in Figure 2, Venn diagram showed the number of common and specific ASVs in the rumen of bulls in different groups: the five groups had 417 ASVs in common, including 38 specific ASVs in the CON, 41 specific ASVs in the TRT1, 37 specific ASVs in the TRT2, 66 specific ASVs in the TRT3 and 38 specific ASVs in the TRT4.
Figure 2. ASV level venn diagramin different groups. CON = basal diet, the ratio of Lys to Met is 3.0:1; TRT1 = LPS infusion and basal diet, the ratio of Lys to Met is 3.0:1; TRT2 = LPS infusion, basal diet added rumen-protected amino acids, the ratio of Lys to Met is 2.5:1; TRT3 = LPS infusion, basal diet added rumen-protected amino acids, the ratio of Lys to Met is 3.0:1; TRT4 = LPS infusion, basal diet added rumen-protected amino acids, the ratio of Lys to Met is 3.5:1.
Alpha diversity mainly reflects the diversity of species. As shown in Table 5, goods-coverage indexes were all above 0.9, which indicated that species diversity and community structure could be well reflected. There was no significant effect of LPS and feeding with different ratios of Lys and Met on the alpha diversity of rumen microorganisms in bulls (p > 0.05).
Table 5. Effects of lipopolysaccharide infusion on alpha diversity of young Holstein bulls fed diets with different ratios of Lys and Met.
As shown in Table 6, there was no significant effect of LPS infusion on the species difference of rumen microorganisms at the phylum level in bulls which fed diets with different ratios of Lys and Met (p > 0.05). Table 7 showed that feeding diets with different proportions of Lys and Met could significantly increase the content of Oribacterium in the rumen (p < 0.05), with that highest Oribacterium content in bulls treated with TRT3. Additionly, norank_f__norank_o__RF39 showed an increasing trend (p = 0.078) at genus level.
Table 6. Comparison of species differences in rumen microorganisms at phylum level in young Holstein bulls infused lipopolysaccharide and fed diets with different Lys and Met ratios (relative abundance >0.5%).
Table 7. Comparison of species differences in rumen microorganisms at genus level in young Holstein bulls fed diets with different ratios of Lys and Met by lipopolysaccharide infusion.
The aim of this experiment is to explore effects of lipopolysaccharide infusion on feed intake, apparent digestibility, rumen fermentation and microorganisms of young Holstein bulls fed diets with different ratios of Lys to Met. The experimental results can provide theoretical support for exploring the amino acid structure of dairy cows’ diets under chronic stress.
Feed intake is the primary factor of determining efficiency in production, and whether it is normal or not significantly impacts animal production (16). Stress is one of the main contributors to decreased feed intake in animals. Low feed intake adversely affects the rumen condition of ruminants (17–19). It has been discovered that a state of stress could lead to a significant reduction in feed intake among mice (20). It has been demonstrated that a variety of stresses including oxidative stress can lead to low feed intake in ruminants (21). Meanwhile, Kang et al. (22) reported that intraperitoneal injection of a high dose of LPS inhibited feed intake. Similar results have been found in studies of dairy cows (23). However, no difference in feed intake obtained by LPS infusion may causing by the dose in this study, which lead to varying doses of RPL and RPM did not significantly effect of young Holstein bulls. The LPS dose selected in this experiment was relatively low, as well as in order to avoid acute stress on the bulls. And a concentration gradient was used to gradually increase the infusion dose, giving the bulls enough time to adapt. This may be the reason why the feed intake decreased to a certain extent while no difference among groups.
Apparent digestibility reflects the efficiency with animals utilize feed. It has been found that incorporating RPL and RPM into the diet of yaks increased nutrient digestibility (24). Dietary addition of RPL and RPM have also been shown to promote the digestibility of CP and NDF in other ruminants (25, 26), likely because methionine or its analogs increased the abundance of cellulose-decomposing bacteria in the rumen (27), there are differences from our experimental results, which also may be related to the rumen passage rate of AA and the level of feeding management. However, the same trend has not been shown in studies of dairy cows (28, 29), which aligns with the findings of this study. Zhao et al. (30) and Lee et al. (31) found that RPM and other essential amino AA did not influence the apparent digestibility of nutrients in dairy cows’ diets, consistent with our research findings. Similarly, Odedra et al. (32) reported that supplementing RPL into the basal diet of goats did not significantly affect the apparent digestibility of DM, ADF, and NDF, consistent with the results of this study. The discrepancies in these research findings may be attributed to the use of different types of rumen-protected AA across studies, which exhibit distinct rumen protection rates, rumen microbial metabolism rates, and apparent digestibility rates.
In the normal physiological range, a lower rumen pH value is beneficial for the rumen development of growing cattle (33). However, when the pH exceeds this range, whether it becomes too acidic or too alkaline, it can lead to a decline in animal production performance, directly negatively impacting rumen fermentation. In severe cases, subacute acidosis may occur, potentially resulting in the death of cattle (34, 35). In this study, the treatments had no significant effect on the rumen pH of young Holstein bulls. Ruminants cannot directly utilize ammonia nitrogen, however, rumen bacteria possess the ability to capture free ammonia nitrogen in the rumen for MCP synthesis (36). The rumen and intestinal microflora serve as intermediaries in nutrient absorption. Through a symbiotic relationship with rumen microorganisms, all forms of dietary nitrogen sources are ultimately degraded into peptides, AA, and ammonia in the rumen. Increasing MCP yield is an effective strategy for enhancing ruminant performance and reducing protein feed waste (37). Macelline et al. (38) reported that broilers fed a low-protein diet supplemented with synthetic AA could sustain growth performance and intestinal integrity while reducing nitrogen excretion. In ruminant, low-protein diet supplemented with RPM promoted rumen digestibility and the production of volatile fatty acids (39). Additionally, Van et al. (40) discovered that supplementing RPM and RPL in a low-protein diet can improve production performance, maintain nitrogen balance, and enhance nitrogen utilization efficiency in dairy cows. However, some studies also showed that the addition of Lys and Met had no effect on rumen fermentation parameters and only improved nitrogen use efficiency (29, 41). Under these experimental conditions, feeding different proportions of RPL and RPM decreased the concentration of NH3-N in the rumen, corroborating the aforementioned findings. Löest et al. (42) found that cattle exposed to LPS exhibited reduced recyclable nitrogen, leading to increased AA oxidation. The observed rise in ruminal NH3-N concentration 24 h after LPS infusion may be attributed to an imbalance between tissue AA supply and immune system AA demand, or due to increased AA oxidation to support other metabolic functions (43). It indicated supplementation of ruminal AA helps maintain this balance and improves nitrogen utilization (44, 45). This suggests that under LPS infusion conditions, incorporating RPL and RPM into the diet of young Holstein bulls can enhance nitrogen utilization efficiency, reduce nitrogen excretion, improve NH3-N utilization efficiency, and optimize their rumen fermentation status, and the optimal ratio of Lys and Met in diet is 3.0: 1.
LPS can cause rumen microbial dysbiosis in cows (46). One of the purposes of this study is to evaluate the effects of LPS infusion on rumen microorganisms in young Holstein bulls fed diets with different ratios of Lys and Met. In this study, feeding diets with different proportions of Lys and Met had no significant effect on rumen microbial diversity at the phylum level and the alpha diversity of rumen microbiota, which aligns with the results reported by the previous studies (47, 48). At the genus level, different proportions of Lys and Met were found to increase the abundance of norank_f__norank_o__RF39 and Oribacterium, maintained rumen homeostasis. The abundance of norank_f__norank_o__RF39 showed a significant positive correlation with NDF digestibility (49). Therefore, the higher NDF in TRT3 compared to other groups may be related to the increase in norank_f__norank_o__RF39. Oribacterium is a strict anaerobic bacterium that plays a crucial role in synthesizing acetate, propionate, and butyrate for its host (50, 51). Additionally, enhanced fiber degradation by the action of Oribacterium was reported in the previous study (52). These TVFAs can be secreted and absorbed by the intestinal tract as nutrients and are also essential for maintaining the host’s health. These changes may lead to the degradation of nutrients and improve utilization efficiency in the rumen. The increase in rumen acetic acid concentration in TRT3 may be related to the increase in Oribacteria abundance.
In conclusion, adding RPL and RPM in diets for young Holstein bulls which infused by LPS has no effect on feed intake and apparent digestibility, but can reduce the concentration of NH3-N in the rumen, the lowest one is when Lys to Met is 3.0:1. Meanwhile, it can increase the abundance of norank_f__norank_o__RF39 and Oribacterium at genus level, the highest one is when Lys to Met is 3.0:1, helping to maintain a stable state of rumen microflora. Thus, it has the best improvement effect on the rumen of young Holstein bulls infused by LPS whenthe ratio of Lys to Met in the diet was 3.0:1.
The raw data supporting the conclusions of this article will be made available by the authors, without undue reservation.
The animal study was approved by Experimental Animal Ethics Committee of Zhejiang A&F University. The study was conducted in accordance with the local legislation and institutional requirements.
HW: Data curation, Writing – original draft. HL: Resources, Supervision, Visualization, Writing – review & editing, Methodology. SP: Data curation, Methodology, Writing – original draft. ZM: Investigation, Writing – original draft. YW: Investigation, Resources, Writing – original draft. JL: Project administration, Supervision, Writing – review & editing. CW: Funding acquisition, Writing – review & editing, Project administration, Supervision, Validation. ZA: Writing – review & editing, Data curation, Project administration, Software, Visualization.
The author(s) declare that financial support was received for the research, authorship, and/or publication of this article. This research was funded by National Natural Science Foundation of China (32172742), Zhejiang Provincial Science and Technology Plan Project (2022C04038), Zhejiang Animal Husbandry Industry Technology Project, Zhejiang Support Xinjiang Science and Technology Project, and Naqu Science and Technology Plan Project (NQKJ-2023-08), the modern agricultural industry technology system (CARS-36).
YW was employed by Kemin (China) Technologies Co. Ltd.
The remaining authors declare that the research was conducted in the absence of any commercial or financial relationships that could be construed as a potential conflict of interest.
The authors declare that no Gen AI was used in the creation of this manuscript.
All claims expressed in this article are solely those of the authors and do not necessarily represent those of their affiliated organizations, or those of the publisher, the editors and the reviewers. Any product that may be evaluated in this article, or claim that may be made by its manufacturer, is not guaranteed or endorsed by the publisher.
1. Schuba, J, Südekum, K-H, Pfeffer, E, and Jayanegara, A. Excretion of faecal, urinary urea and urinary non-urea nitrogen by four ruminant species as influenced by dietary nitrogen intake: a meta-analysis. Livest Sci. (2017) 198:82–8. doi: 10.1016/j.livsci.2017.01.017
2. Sinclair, KD, Garnsworthy, PC, Mann, GE, and Sinclair, LA. Reducing dietary protein in dairy cow diets: implications for nitrogen utilization, milk production, welfare and fertility. Animal. (2014) 8:262–74. doi: 10.1017/S1751731113002139
3. Coleman, DN, Lopreiato, V, Alharthi, A, and Loor, JJ. Amino acids and the regulation of oxidative stress and immune function in dairy cattle. J Anim Sci. (2020) 98:S175–93. doi: 10.1093/jas/skaa138
4. Zeineldin, M, Barakat, R, Elolimy, A, Salem, AZM, Elghandour, MMY, and Monroy, JC. Synergetic action between the rumen microbiota and bovine health. Microb Pathog. (2018) 124:106–15. doi: 10.1016/j.micpath.2018.08.038
5. Long, X, Liu, Q, Chan, M, Wang, Q, and Sun, SSM. Metabolic engineering and profiling of rice with increased lysine. Plant Biotechnol J. (2013) 11:490–501. doi: 10.1111/pbi.12037
6. Gresner, N, Rodehutscord, M, and Südekum, KH. Amino acid pattern of rumen microorganisms in cattle fed mixed diets-an update. J Anim Physiol Anim Nutr. (2022) 106:752–71. doi: 10.1111/jpn.13676
7. Junior, VC, Lopes, F, Schwab, CG, Toledo, MZ, and Collao-Saenz, EA. Effects of rumen-protected methionine supplementation on the performance of high production dairy cows in the tropics. PLoS One. (2021) 16:e0243953. doi: 10.1371/journal.pone.0243953
8. Coleman, DN, Totakul, P, Onjai-Uea, N, Aboragah, A, Jiang, Q, Vailati-Riboni, M, et al. Rumen-protected methionine during heat stress alters mTOR, insulin signaling, and 1-carbon metabolism protein abundance in liver, and whole-blood transsulfuration pathway genes in Holstein cows. J Dairy Sci. (2022) 105:7787–804. doi: 10.3168/jds.2021-21379
9. Guadagnin, AR, Fehlberg, LK, Thomas, B, Sugimoto, Y, Shinzato, I, and Cardoso, FC. Effect of feeding rumen-protected lysine through the transition period on postpartum uterine health of dairy cows. J Dairy Sci. (2022) 105:7805–19. doi: 10.3168/jds.2022-21934
10. Gu, F, Liang, S, Zhu, S, Liu, J, and Sun, HZ. Multi-omics revealed the effects of rumen-protected methionine on the nutrient profile of milk in dairy cows. Food Res Int. (2021) 149:110682. doi: 10.1016/j.foodres.2021.110682
11. Liu, C, Zhao, D, Ma, W, Guo, Y, Wang, A, Wang, Q, et al. Denitrifying sulfide removal process on high-salinity wastewaters in the presence of Halomonas sp. Appl Microbiol Biotechnol. (2016) 100:1421–6. doi: 10.1007/s00253-015-7039-6
12. Zhang, F, Xie, Y, Peng, R, Ji, X, and Bai, L. Heavy metals and nutrients mediate the distribution of soil microbial community in a typical contaminated farmland of South China. Sci Total Environ. (2024) 947:174322. doi: 10.1016/j.scitotenv.2024.174322
13. Broderick, GA, and Kang, JH. Automated simultaneous determination of ammonia and total amino acids in ruminal fluid and in vitro media. J Dairy Sci. (1980) 63:64–75. doi: 10.3168/jds.S0022-0302(80)82888-8
14. AOAC. Official methods of analysis. Arlington, VA: Association of Official Analytical Chemists (1990).
15. Van Soest, PJ, Robertson, JB, and Lewis, BA. Methods for dietary fiber, neutral detergent fiber, and nonstarch polysaccharides in relation to animal nutrition. J Dairy Sci. (1991) 74:3583–97. doi: 10.3168/jds.S0022-0302(91)78551-2
16. Assan, D, Huang, Y, Mustapha, UF, Addah, MN, Li, G, and Chen, H. Fish feed intake, feeding behavior, and the physiological response of apelin to fasting and refeeding. Front Endocrinol. (2021) 12:798903. doi: 10.3389/fendo.2021.798903
17. Goopy, JP, Korir, D, Pelster, D, Ali, AIM, Wassie, SE, Schlecht, E, et al. Severe below-maintenance feed intake increases methane yield from enteric fermentation in cattle. Br J Nutr. (2020) 123:1239–46. doi: 10.1017/S0007114519003350
18. Ali, DN, and Hennessy, DR. The effect of feed intake on the rate of flow of digesta and the disposition and activity of oxfendazole in sheep. Int J Parasitol. (1993) 23:477–84. doi: 10.1016/0020-7519(93)90036-X
19. Adams-Progar, AL, Friend, TH, Holub, GA, Krenek, AJ, Garey, SM, and Terrill, CL. Effects of repeated transport on Holstein calf post-transport behavior and feed intake. J Anim Sci. (2015) 93:731–6. doi: 10.2527/jas.2014-7724
20. Xiao, Y, Kronenfeld, JM, and Renquist, BJ. Feed intake-dependent and -independent effects of heat stress on lactation and mammary gland development. J Dairy Sci. (2020) 103:12003–14. doi: 10.3168/jds.2020-18675
21. Bühler, S, Frahm, J, Tienken, R, Kersten, S, Meyer, U, Huber, K, et al. Effects of energy supply and nicotinic acid supplementation on serum anti-oxidative capacity and on expression of oxidative stress-related genes in blood leucocytes of periparturient primi- and pluriparous dairy cows. J Anim Physiol Anim Nutr. (2018) 102:e87–98. doi: 10.1111/jpn.12705
22. Kang, A, Xie, T, Zhu, D, Shan, J, Di, L, and Zheng, X. Suppressive effect of ginsenoside Rg3 against lipopolysaccharide-induced depression-like behavior and neuroinflammation in mice. J Agric Food Chem. (2017) 65:6861–9. doi: 10.1021/acs.jafc.7b02386
23. Lippolis, KD, Cooke, RF, Schubach, KM, Marques, RS, and Bohnert, DW. Effects of intravenous lipopolysaccharide administration on feed intake, ruminal forage degradability, and liquid parameters and physiological responses in beef cattle. J Anim Sci. (2017) 95:2859–70. doi: 10.2527/jas.2017.1502
24. Wang, H, Fu, J, Wu, X, Wang, Y, Li, W, Huang, Y, et al. Effects of dietary protein level and rumen-protected methionine and lysine on growth performance, rumen fermentation and serum indexes for yaks. Animals. (2024) 14:1751. doi: 10.3390/ani14121751
25. Liu, YQ, Wang, C, Liu, C, Zhang, J, and Liu, Q. Effects of coated folic acid and coated methionine on growth performance, nutrient digestibility and rumen fermentation in Simmental bulls. Anim Feed Sci Tech. (2023) 298:115596. doi: 10.1016/j.anifeedsci.2023.115596
26. Chen, J, Niu, X, Li, FD, and Guo, L. Replacing soybean meal with distillers dried grains with solubles plus rumen-protected lysine and methionine: effects on growth performance, nutrients digestion, rumen fermentation, and serum parameters in Hu sheep. Animals. (2021) 11:2428. doi: 10.3390/ani11082428
27. Soto, RC, Muhammed, SA, Newbold, CJ, Stewart, CS, and Wallace, RJ. Influence of peptides, amino acids and urea on microbial activity in the rumen of sheep receiving grass hay and on the growth of rumen bacteria in vitro. Anim Feed Sci Tech. (1994) 49:151–61. doi: 10.1016/0377-8401(94)90088-4
28. Li, Y, Wei, J, Dou, M, Liu, S, Yan, B, Li, C, et al. Effects of rumen-protected methionine supplementation on production performance, apparent digestibility, blood parameters, and ruminal fermentation of lactating Holstein dairy cows. Front Vet Sci. (2022) 9:981757. doi: 10.3389/fvets.2022.981757
29. Wei, J, Cao, Z, and Li, Y. 294 effect of supplementing rumen-protected methionine on production, apparent digestibility and economic effectiveness in lactating Holstein dairy cows. J Anim Sci. (2021) 99:159–9. doi: 10.1093/jas/skab235.292
30. Zhao, K, Liu, W, Lin, XY, Hu, ZY, Yan, ZG, Wang, Y, et al. Effects of rumen-protected methionine and other essential amino acid supplementation on milk and milk component yields in lactating Holstein cows. J Dairy Sci. (2019) 102:7936–47. doi: 10.3168/jds.2018-15703
31. Lee, C, Giallongo, F, Hristov, AN, Lapierre, H, Cassidy, TW, Heyler, KS, et al. Effect of dietary protein level and rumen-protected amino acid supplementation on amino acid utilization for milk protein in lactating dairy cows. J Dairy Sci. (2015) 98:1885–902. doi: 10.3168/jds.2014-8496
32. Odedra, MD, Ravikala, K, Bhadaniya, AR, and Gajera, AP. Effect of supplemental rumen bypass lysine and methionine on digestibility and efficiency of nutrients in growing jaffrabadi heifers. Indian J Vet Sci Biotechnol. (2015) 10:54–9.
33. Villot, C, Meunier, B, Bodin, J, Martin, C, and Silberberg, M. Relative reticulo-rumen pH indicators for subacute ruminal acidosis detection in dairy cows. Animal. (2018) 12:481–90. doi: 10.1017/S1751731117001677
34. Cerrato-Sánchez, M, Calsamiglia, S, and Ferret, A. Effects of time at suboptimal pH on rumen fermentation in a dual-flow continuous culture system. J Dairy Sci. (2007) 90:1486–92. doi: 10.3168/jds.S0022-0302(07)71634-X
35. Mensching, A, Hummel, J, and Sharifi, AR. Statistical modeling of ruminal pH parameters from dairy cows based on a meta-analysis. J Dairy Sci. (2020) 103:750–67. doi: 10.3168/jds.2019-16802
36. Pengpeng, W, and Tan, Z. Ammonia assimilation in rumen bacteria: a review. Anim Biotechnol. (2013) 24:107–28. doi: 10.1080/10495398.2012.756402
37. Lu, ZY, Xu, ZH, Shen, ZM, and Tian, YC. Dietary energy level promotes rumen microbial protein synthesis by improving the energy productivity of the ruminal microbiome. Front Microbiol. (2019) 10:847. doi: 10.3389/fmicb.2019.00847
38. Macelline, SP, Wickramasuriya, SS, Cho, HM, Kim, E, Shin, TK, Hong, JS, et al. Broilers fed a low protein diet supplemented with synthetic amino acids maintained growth performance and retained intestinal integrity while reducing nitrogen excretion when raised under poor sanitary conditions. Poultry Sci. (2020) 99:949–58. doi: 10.1016/j.psj.2019.10.035
39. Abbasi, IHR, Abbasi, F, Abd El-Hack, ME, Swelum, AA, Yao, J, and Cao, Y. Post-ruminal effects of rumen-protected methionine supplementation with low protein diet using long-term simulation and in vitro digestibility technique. AMB Express. (2018) 8:36. doi: 10.1186/s13568-018-0566-7
40. Van den Bossche, T, Goossens, K, Ampe, B, Haesaert, G, De Sutter, J, De Boever, JL, et al. Effect of supplementing rumen-protected methionine, lysine, and histidine to low-protein diets on the performance and nitrogen balance of dairy cows. J Dairy Sci. (2023) 106:1790–802. doi: 10.3168/jds.2022-22041
41. Ao, R, Liang, C, Zhiwei, K, Bin, Z, ChuanShe, Z, Zhiliang, T, et al. Effects of dietary methionine and lysine supplementation on growth performance, meat composition and rumen fermentation pattern in goats. Indian J Anim Res. (2019) 53:1629–34. doi: 10.18805/ijar.B-1073
42. Löest, CA, Gilliam, GG, Waggoner, JW, and Turner, JL. Post-ruminal branched-chain amino acid supplementation and intravenous lipopolysaccharide infusion alter blood metabolites, rumen fermentation, and nitrogen balance of beef steers. J Anim Sci. (2018) 96:2886–906. doi: 10.1093/jas/sky168
43. Reeds, PJ, and Jahoor, F. The amino acid requirements of disease. Clin Nutr. (2001) 20:15–22. doi: 10.1054/clnu.2001.0402
44. Reeds, PJ, Fjeld, CR, and Jahoor, F. Do the differences between the amino acid compositions of acute-phase and muscle proteins have a bearing on nitrogen loss in traumatic states? J Nutr. (1994) 124:906–10. doi: 10.1093/jn/124.6.906
45. Calder, PC. Branched-chain amino acids and immunity. J Nutr. (2006) 136:288S–93S. doi: 10.1093/jn/136.1.288S
46. Hu, X, Li, S, Mu, R, Guo, J, Zhao, C, Cao, Y, et al. The rumen microbiota contributes to the development of mastitis in dairy cows. Microbiol Spectr. (2022) 10:e0251221. doi: 10.1128/spectrum.02512-21
47. Guo, Y, Fan, Z, Li, M, Xie, H, Peng, L, and Yang, C. Effects of sodium nitrate and coated methionine on lactation performance, rumen fermentation characteristics, amino acid metabolism, and microbial communities in lactating buffaloes. Microorganisms. (2023) 11:675. doi: 10.3390/microorganisms11030675
48. Hassan, FU, Guo, Y, Li, M, Tang, Z, Peng, L, Liang, X, et al. Effect of methionine supplementation on rumen microbiota, fermentation, and amino acid metabolism in in vitro cultures containing nitrate. Microorganisms. (2021) 9:1717. doi: 10.3390/microorganisms9081717
49. Jiang, F, Gao, Y, Peng, Z, Ma, X, You, Y, Hu, Z, et al. Isoacids supplementation improves growth performance and feed fiber digestibility associated with ruminal bacterial community in yaks. Front Microbiol. (2023) 14:1175880. doi: 10.3389/fmicb.2023.1175880
50. Tian, Y, Gu, C, Yan, F, Gu, Y, Feng, Y, Chen, J, et al. Alteration of skin microbiome in CKD patients is associated with pruritus and renal function. Front Cell Infect Microbiol. (2022) 12:923581. doi: 10.3389/fcimb.2022.923581
51. Carlier, JP, Bedora-Faure, M, K’ouas, G, Alauzet, C, and Mory, F. Proposal to unify Clostridium orbiscindens winter et al. 1991 and Eubacterium plautii (Séguin 1928) Hofstad and Aasjord 1982, with description of Flavonifractor plautii gen. Nov., comb. nov., and reassignment of Bacteroides capillosus to Pseudoflavonifractor capillosus gen. Nov., comb. Nov. Int J Syst Evol Microbiol. (2010) 60:585–90. doi: 10.1099/ijs.0.016725-0
Keywords: lipopolysaccharide, lysine, methionine, young Holstein bulls, rumen microorganism
Citation: Wang H, Liu H, Pan S, Ma Z, Wang Y, Liu J, Wang C and An Z (2025) Effects of lipopolysaccharide infusion on feed intake, apparent digestibility, rumen fermentation and microorganisms of young Holstein bulls fed diets with different ratios of lysine and methionine. Front. Vet. Sci. 11:1523062. doi: 10.3389/fvets.2024.1523062
Received: 05 November 2024; Accepted: 11 December 2024;
Published: 06 January 2025.
Edited by:
Shengguo Zhao, Chinese Academy of Agricultural Sciences, ChinaReviewed by:
Xianjun Dai, China Jiliang University, ChinaCopyright © 2025 Wang, Liu, Pan, Ma, Wang, Liu, Wang and An. This is an open-access article distributed under the terms of the Creative Commons Attribution License (CC BY). The use, distribution or reproduction in other forums is permitted, provided the original author(s) and the copyright owner(s) are credited and that the original publication in this journal is cited, in accordance with accepted academic practice. No use, distribution or reproduction is permitted which does not comply with these terms.
*Correspondence: Chong Wang, d2FuZ2Nvbmc5OTJAMTYzLmNvbQ==; Zhigao An, emdhbkB6YWZ1LmVkdS5jbg==
†These authors have contributed equally to this work
Disclaimer: All claims expressed in this article are solely those of the authors and do not necessarily represent those of their affiliated organizations, or those of the publisher, the editors and the reviewers. Any product that may be evaluated in this article or claim that may be made by its manufacturer is not guaranteed or endorsed by the publisher.
Research integrity at Frontiers
Learn more about the work of our research integrity team to safeguard the quality of each article we publish.