- 1College of Veterinary Medicine, Henan Agricultural University, Zhengzhou, China
- 2National Research Center for Veterinary Medicine, Luoyang, China
Feline herpesvirus 1 (FHV-1) is an important pathogen causing infectious rhinotracheitis in felids, mainly infecting the upper respiratory tract and conjunctiva. Multiple vaccines are available to prevent FHV-1 infection, and the antibody levels are always used to evaluate their effectiveness. However, the cellular immunity response following immunization in cats remains unclear. This study investigated the immune responses (humoral and cellular) in cats immunized with the FHV-1 modified live virus vaccine. The results indicated that vaccination significantly reduced clinical signs, and antibody levels, including virus-neutralizing (VN) antibodies and immunoglobulin G (IgG), in the vaccine group were higher than those in the control groups. Additionally, the vaccine significantly increased cytokine secretion, indicating Th1-type cellular immune responses in cats. Moreover, cellular immune-related indicators, such as CD8+ T cells, CD4+ T cells, and interferon-gamma levels, were inversely correlated with clinical signs post-challenge by FHV-1 in vaccinated cats, highlighting its crucial role in protecting cats against FHV-1 infection. In summary, this study demonstrated the importance of cellular immune responses in protecting cats from FHV-1 infection after vaccination.
1 Introduction
Feline herpesvirus 1 (FHV-1), or felid herpesvirus 1 (FeHV-1), belongs to the Varicellovirus genus in the Alphaherpesvirinae subfamily of the Herpesviridae family (1). FHV-1 is highly contagious and an important pathogen, causing infectious rhinotracheitis in felids (2). The kittens are the most susceptible to infection, with morbidity and mortality rates up to 100 and 50%, respectively (3, 4). FHV-1 mainly infects the upper respiratory tract and conjunctiva, which clinically manifests fever, conjunctivitis, keratitis, nasal and ocular serous or mucinous discharge, and severe infection can cause lobular pneumonia and periocular skin ulcers (5, 6). Similar to other herpesviruses, the incubation period is established in the trigeminal ganglion following the acute phase of infection (7). When the cats are under stress or immunosuppression, the virus is easily reactivated, accompanied by the periodic shedding of infectious viruses and the recurrence of clinical symptoms, resulting in lifelong virus infection and recurrence (1). As part of the feline core vaccine, FHV-1 is included in a triple combination of inactivated and modified live virus (MLV) vaccines. The World Association of Small Animal Veterinarians (WSAVA) recommends vaccinating kittens with FHV-1 at 6–8 weeks of age, with at least one more dose after 2–4 weeks, followed by annual vaccination thereafter (8).
Despite widespread vaccination worldwide, current FHV-1 vaccines offer limited and short-duration protection. The diverse evasion strategies employed by herpesviruses to counteract the host’s innate immune response and their encoding of various anti-interferon response effectors often result in a limited efficacy of FHV-1 vaccination (9). FHV-1 comprises 11 distinct proteins that collaborate to facilitate immune evasion during infection (10). The subsequent viral infection outbreaks cannot be disregarded even though MLV vaccines induce more robust immune responses than inactivated vaccines (11, 12). Moreover, serum virus antibody levels are frequently applied to assess immunity to FHV-1; however, the impact of cellular immune response should not be overlooked on immunity against infections (1).
Vaccination induces a critical cellular immune response essential for preventing viral infections. Upon antigen exposure, the immune system initiates a robust response by activating T lymphocytes, specifically CD4+ helper and CD8+ cytotoxic T cells, which recognize and eliminate infected cells (13). Interferon-gamma (IFN-γ), a key cytokine secreted by activated T cells, plays a crucial role in this process. It binds to receptors on infected cells, triggering a cascade of intracellular events that effectively inhibit virus replication and dissemination (14). IFN-γ also enhances the function of other immune cells, coordinating a synchronized attack on the virus (15). This coordinated cellular immune response is essential for effective viral clearance and overall health maintenance (16). Observed that the activation of T cells to produce IFN-γ is the predominant immune response after a bovine herpesvirus 1 (BHV-1) infection. Furthermore, the bacterium-like particle (BLP) vaccine with FHV-1 gB, gC, and gD induces a specific cellular immune response in mice (17). However, the cellular immune responses induced in cats after FHV-1 infection or vaccination and the indicators associated with resistance to FHV-1 infection remain unclear.
This study focused on exploring immune responses in immunized cats with the FHV-1 MLV vaccine and subsequent challenges with FHV-1. Moreover, a correlation between humoral and cellular immune responses and protection against challenge was analyzed to obtain indicators related to protection against FHV-1 infection. Furthermore, our findings will serve as a reference for the assessment of vaccines related to FHV-1.
2 Materials and methods
2.1 Animals
A total of 13 eight-week-old cats free of feline calicivirus (FCV), feline parvovirus (FPV), FHV-1, and Feline coronavirus (FCoV) were randomly divided into three groups. Each cat was kept in a separate cage, with different groups separated into distinct spaces. The animal studies were approved by the Committee on the Ethics of Animal Experiments of the National Research Center for Veterinary Medicine (Permit Number: 202307001) and conducted in conformity with the Guide for the Care and Use of Animals in Research of the People’s Republic of China.
2.2 Cells, viruses, and vaccine preparation
Feline kidney cells (F81 cells) were maintained in Roswell Park Memorial Institute (RPMI) 1,640 medium (Gibco, Carlsbad, CA, United States), supplemented with 8% fetal bovine serum (Cegrogen Biotech, Germany), 100 U/mL penicillin, and 0.1 mg/mL streptomycin (Sigma-Aldrich, St. Louis, MO, USA) at 37°C with 5% CO2. The FHV-1 strain 64 was isolated from the eyelid and nasal swab suspension of FHV-1 infected cats in Henan Province in 2016. The eyelid and nasal swab suspension were inoculated on F81 cells for virus isolation as previously described (18). When CPE were observed in 80% of the cells, cultures were harvested and the resultant virus stocks were stored at −80°C and named as FHV-1 strain 64. Cats infected with FHV-1 strain 64 presented with ocular and nasal discharge, conjunctival congestion, and sneezing (data not shown). The serial passage was used to attenuate the FHV-1 strain 64, and the MLV vaccine was prepared by attenuating the FHV-1 strain 64 (106.0 TCID50/mL).
2.3 Experimental design
The experimental schedule is illustrated in Figure 1A. The cats were randomly divided into three groups: (1) Unvaccinated controls, n = 5, (2) vaccine immunization group, n = 5, (3) Mock group, n = 3; based on the immunization schedule of the vaccine in the published article, cats were immunized subcutaneously (SC) on days 0 and 21 (19, 20). Groups 1 and 2 were challenged with intranasal FHV-1 64 on day 35, and the mock group was inoculated with RPMI 1640 medium. Clinical symptoms and body temperatures were monitored daily, and venous blood was collected on days 0, 21, and 35 to detect the virus-neutralizing (VN) antibody and IgG levels. Serum cytokine levels were detected in venous blood collected on days 0, 21, 35, 42, and 49, and serum was stored at −20°C for further testing. On day 49, peripheral blood was collected to isolate lymphocytes, after which the cats were humanely euthanized.
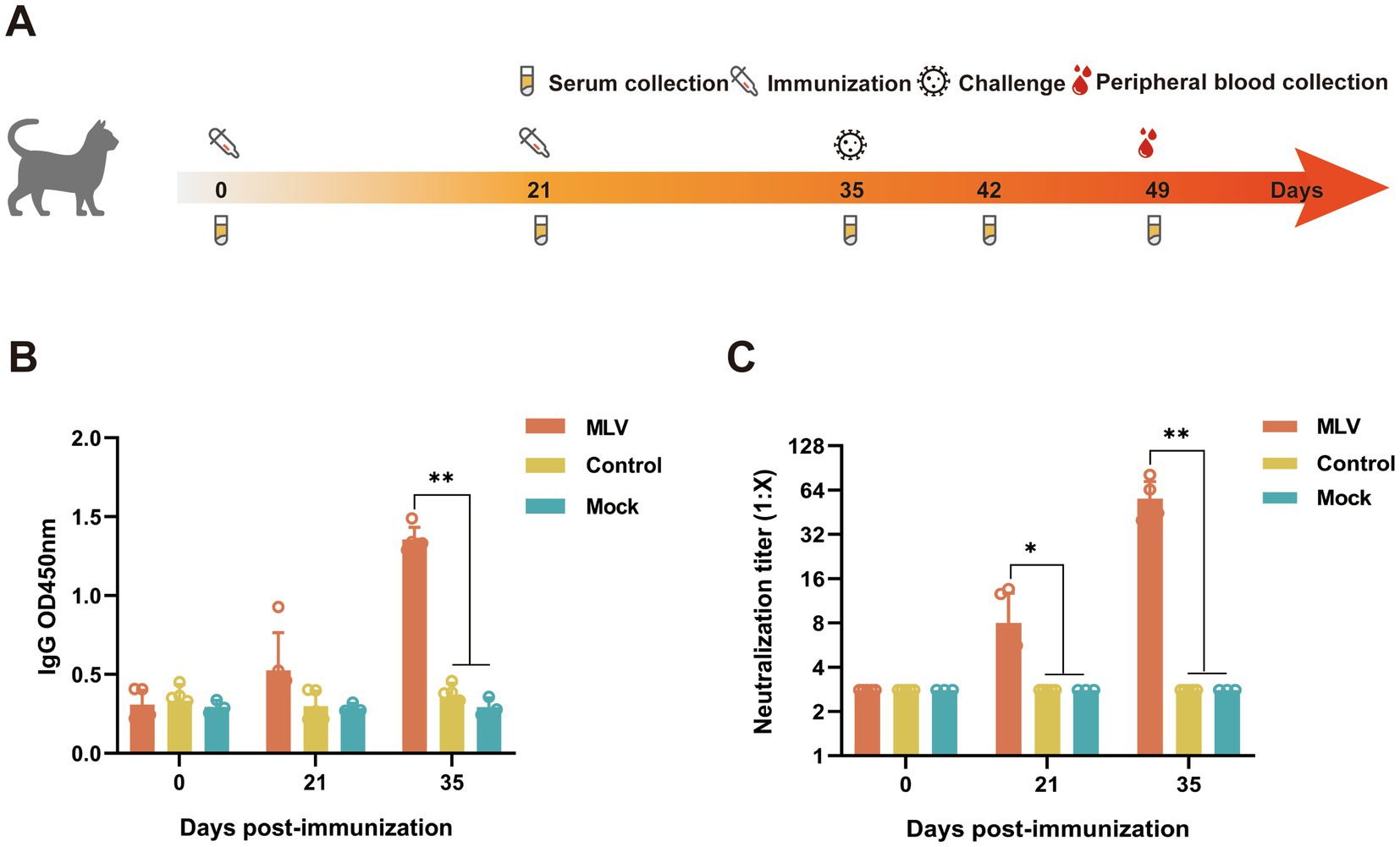
Figure 1. Detection of antibody levels in the serum from the immunized cats. (A) Schematic diagram of the experiment. (B) The serum IgG was measured using ELISA at OD450 nm (n = 13). (C) The serum FHV-1 neutralizing antibody titers were measured using FHV-1 neutralization assay (n = 13).
2.4 Virus-neutralizing antibody detection
The serum was analyzed for FHV-1 VN antibodies by heat-inactivating it at 56°C for 30 min, followed by a two-fold serial dilution ranging from 1:2 to 1:256 according to the previous method (21). A 200 TCID50 dose of FHV-1 was mixed with the serum sample in a volume ratio 1:1, incubated at 37°C for 1 h, and then added F81 cells (2 × 104 cells/100 μL). After a 4-day incubation at 37°C with 5% CO2, antibody titers were measured by CPE and expressed as the reciprocal of the highest dilution at which infection of the F81 cells was inhibited in 50% of the culture wells.
2.5 IgG antibody detection
The IgG antibodies in the serum were detected 14 days after the second immunization using an indirect enzyme-linked immunosorbent assay (ELISA) according to the previous method (17). Microtiter plates (96-well) were coated with lab-stored, purified FHV-1 (0.25 μg/well) and incubated overnight at 4°C. The plates were then blocked with a blocking buffer for 24 h at 4°C. Serum samples were heat-inactivated at 56°C for 30 min and pre-diluted (1:2,000) with phosphate buffer saline (PBS). The samples were then incubated at 37°C for 1 h, followed by incubation with HRP-conjugated goat anti-feline IgG (1,2000) at 37°C for 30 min. Tetramethyl benzidine (TMB) was added to each well, and then the reaction was stopped with 2 M H2SO4. The absorbance was measured at an optical density (OD) of 450 nm using a microplate reader (BioTek, Vermont, United States).
2.6 Clinical signs and histological analysis
Clinical scores were obtained by trained observers masked to the vaccine groups from day 0 to 14 days after the FHV-1 challenge (day 35), as described in previous studies (22, 23). Two observers recorded signs for 30 min per group each morning. Clinical signs included fever, conjunctivitis, blepharospasm, ocular discharge, sneezing, and nasal discharge. The eyelids, turbinates, tracheas, and lungs of cats were harvested 14 days after the FHV-1 challenge. All tissue samples were fixed in 4% paraformaldehyde, embedded in paraffin, and sectioned before hematoxylin and eosin staining (H&E). Monoclonal antibody 5H8 against FHV-1 gD and goat anti-mouse IgG conjugated to HRP (ThermoFisher Scientific, Waltham, MA, United States) were used for immunohistochemical (IHC) assays. Stained sections were visualized using a microscope (Leica DM2500, Leica Microsystems, Germany).
2.7 Viral shedding
After challenge, the eyelid swabs were tested daily for the FHV-1 DNA. Eyelid swabs were collected into 1 mL of PBS, and viral nucleic acid was extracted from 200 μL eyelid swab samples using the Viral Nucleic Acid Extraction Kit (Geneaid Biotech Ltd., Taiwan, P.R. China). The primers for the PCR test of FHV-1 as previously described (21). Briefly, the primers used were: Forward (5′-3′): GAC GTG GTG AAT TAT C; Reverse (5′–3′): CAA CTA GAT TTC CAC CAG GA. The reaction conditions were as follows: pre-denaturation at 95°C for 3 min, denaturation at 95°C for 30s, annealing at 58°C for 30s, elongation at 72°C for 30s, 30 cycles. Amplicons were detected on 1.5% agarose gels, with a positive reaction indicated by a 288 bp band.
2.8 Feline inflammatory cytokines detection
The feline interferon-gamma (IFN-γ) ELISA kit (Mabtech AB, Stockholm, Sweden), feline interleukin 2 (IL-2), and IL-4 ELISA kit (R&D Systems, Minneapolis, United States), and feline IL-17A ELISA kit (MyBioSource, San Diego, CA, United States) were used to measure the levels of IFN-γ, IL-2, IL-4, and IL-17A, respectively, following the manufacturer’s instructions. Each sample was analyzed in duplicate.
2.9 Flow cytometry
The peripheral blood lymphocytes were isolated with commercial kits (Tianjin Haoyang Biological Manufacture, P.R. China) following the manufacturer’s instructions and stored in liquid nitrogen. The percentages of CD4+ and CD8+ T cells in lymphocytes were determined by flow cytometry using a staining method adapted from a previously published protocol (24). The thawed lymphocytes were washed thrice with flow cytometry staining buffer by centrifugation at 2000 rpm for 5 min each. The lymphocytes were blocked by resuspending for 10 min at 4°C in flow cytometry staining buffer containing 10 μL negative cat serum. Subsequently, a total of 1 × 106cells were stained with 10 μL FITC Anti-cat CD4, RPE Anti-cat CD8, and Alexa Fluor® 647 Anti-cat CD134 antibodies (Bio-Rad Laboratories, United States) for 30 min at 4°C in the dark. After washing, the lymphocytes were stained with Hoechst 33258 (ThermoFisher Scientific, Waltham, MA, United States) for 20 min at 4°C in the dark. After three additional washes, the lymphocytes were resuspended with a flow cytometry staining buffer and analyzed using a flow cytometer (BD Biosciences, Franklin, CA, United States).
2.10 Enzyme-linked immunospot assay
The feline IFN-γ ELISpot kit (Mabtech AB, Stockholm, Sweden) and feline IL-2 ELISpot kit (R&D Systems, Minneapolis, United States) were used to evaluate the cell-mediated immune response induced by the FHV-1 vaccine. Lymphocytes from the cats on day 49 obtained as described previously were seeded into ELISpot plates (5 × 105 live cells/well) and stimulated with FHV-1 (MOI = 0.3) for 24 h. The assay was performed according to the manufacturer’s instructions. The spot-forming cells (SFCs) were counted using an ELISpot plate reader (Cellular Technology Ltd., Shaker Heights, OH, United States). The IFN-γ or IL-2 secretory cell numbers were calculated by subtracting the values from PBS-stimulated wells from FHV-1 stimulated wells.
2.11 Statistical analysis
The total clinical scores for each cat were calculated over the post-challenge (days 0–14) study periods. A Statistical Package for the Social Sciences (version 21.0) was used to conduct Pearson correlation analysis between clinical scores post-FHV-1 challenges and detection indicators. Data were analyzed by one-way analysis of variance and an independent-sample t-test at a significance level of p < 0.05.
3 Results
3.1 FHV-1 MLV vaccine immunization induces efficient antibody production in cats
The immunogenicity induced by the FHV-1 modified live virus vaccine was evaluated by administering two injections, 21 days apart, to cats (Figure 1A). The results showed that the immunized cats produced significantly higher levels of IgG compared to the control group at 14 days after the second immunization (Figure 1B). Similarly, the neutralizing antibody levels were notably higher in the immunized cats than those of non-immunized cats (Figure 1C). These data demonstrate that the FHV-1 MLV vaccine effectively induced IgG and VN antibodies production against FHV-1 infection in cats.
3.2 Proportion of CD4+ and CD8 + T cells
CD8+ and CD4+ T cells play a crucial role in clearing viral infections. To further investigate the T cell responses induced by the FHV-1 MLV vaccine, the percentages of CD8+ and CD4+ T cells within the lymphocytes of vaccinated cats were measured using flow cytometry. Figure 2A depicts the gating strategy of CD4+ T cells and CD8+ T cells in flow cytometry. The results revealed a substantial increase in the CD4+ T cells in the immunized cats compared to the control and mock group (Figure 2B; p < 0.05). The immunized group also exhibited a significantly increased number of CD8+ T cells than the control and mock group (Figure 2C; p < 0.05). Furthermore, the mean fluorescence intensity (MFI) of CD134+ T cells was also detected as a result of CD134 being expressed on activated CD4 T cells (25). However, there was no significant increase in the MFI of CD134+ T cells in the immunized group compared to the control and mock group (Figure 2D; p > 0.05).
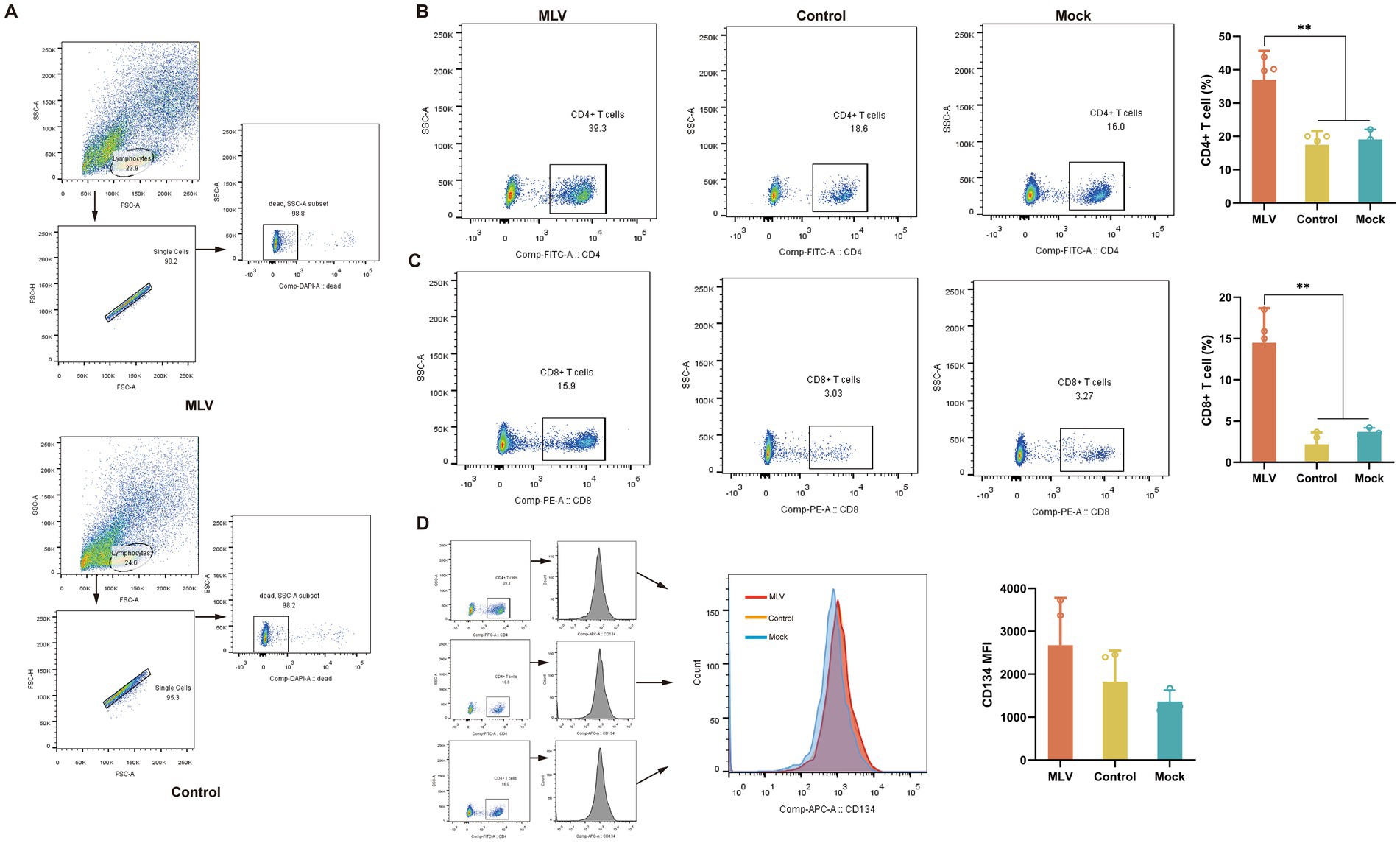
Figure 2. Analysis of T cells in lymphocytes. On the 14th day after the challenge, peripheral blood lymphocytes of cats were collected. (A) The gating strategy of CD4+ T cells and CD8+ T cells in flow cytometry. (B) Representative flow cytometric plots of lymphocytes and the percentage of CD4+ T cells (n = 11). (C) Representative flow cytometric plots of lymphocytes and the percentage of CD8+ T cells (n = 11). (D) Representative flow cytometric plots of lymphocytes and the MFI of CD4+ CD134+ T cells are representative of activated CD4+ T cells (n = 11). The numbers in the gates refer to the percentage of positive cells for each marker. The results were considered statistically significant at p < 0.05.
3.3 FHV-1 MLV vaccine immunization drives Th1-based cellular immune responses in cats
To assess the activation of the cellular immune response after immunization with the FHV-1 MLV vaccine, the cytokines levels of IFN-γ, IL-2, IL-4, and IL-17A in serum were detected by ELISA. Figure 3A illustrates significantly higher levels of IL-2 in the FHV-1 MLV vaccinated group than the control group (p < 0.01), with minimal IL-2 production in the control group after the FHV-1 challenge. However, no significant difference in IFN-γ and IL-17A levels was observed between the control and vaccinated groups (Figures 3B,C; p > 0.05). Furthermore, IL-4 levels were undetectable in all groups.
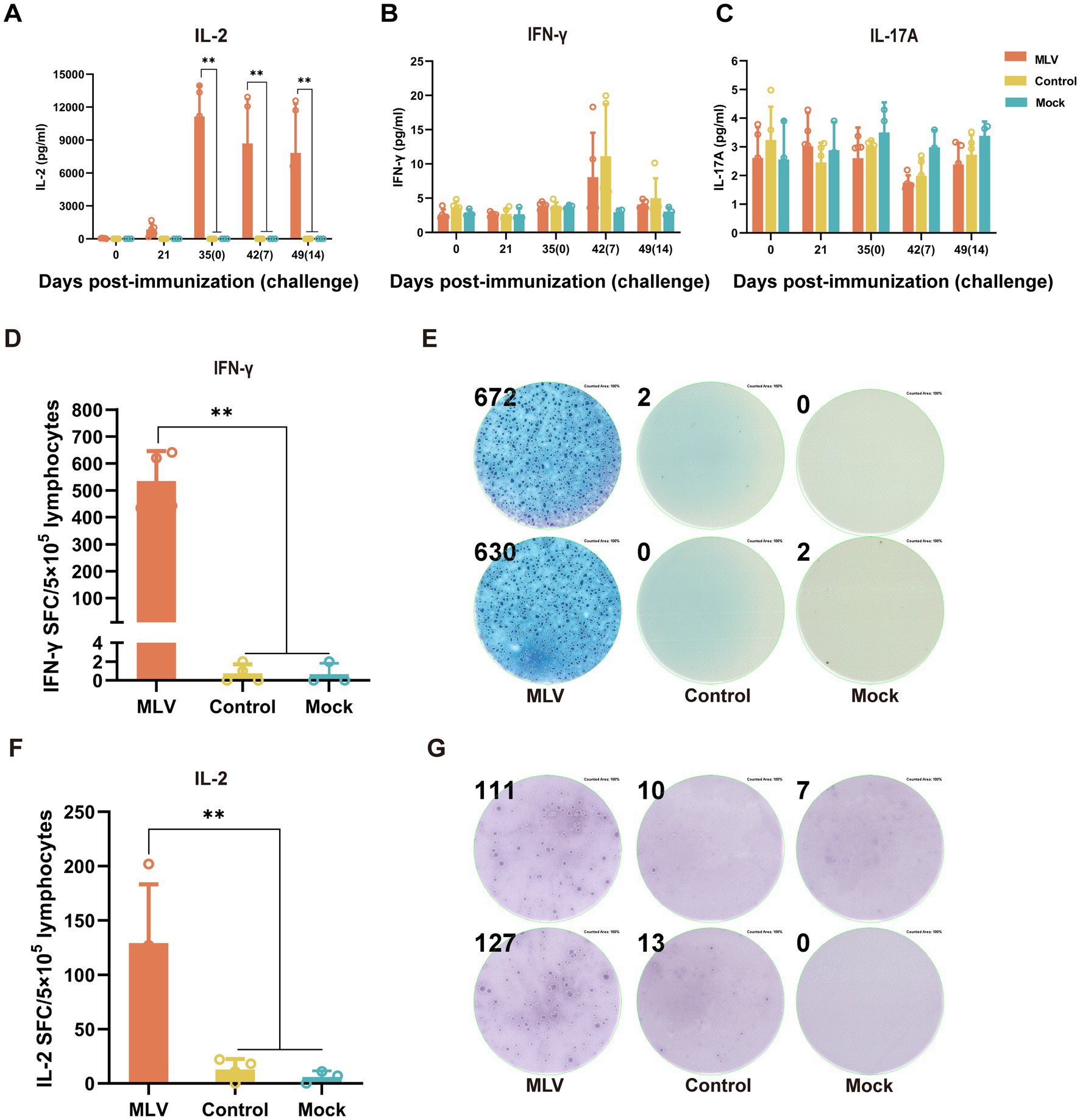
Figure 3. The FHV-1 MLV vaccine immunization induces Th1-based cellular immunity in cats. (A–C) The cytokine levels of IL-2, IFN-γ, and IL-17A in cat serum after immunization or challenge (n = 13). (D) Number of IFN-γ spot-forming counts after 24 h of stimulation with FHV-1 (n = 11). (F) Number of IL-2 spot-forming counts after 24 h of stimulation with FHV-1 (n = 11). (E,G) Representative images of ELISpot wells. The results were considered statistically significant at p < 0.05.
Based on the significant increase in percentages of CD8+ and CD4+ T cells and the elevated levels of IL-2 in the immunized group, it was hypothesized that the FHV-1 MLV vaccine may induces a Th1-type cell immune response in cats. To verify this, ELISpot assays detected significantly more SFCs secreting IFN-γ and IL-2, which are Th1-type cytokines, in the immunized groups compared to the control and mock groups (Figures 3D–G; p < 0.05). These results indicate a pronounced cellular immune response induced by the FHV-1 MLV vaccine in cats.
3.4 FHV-1MLV vaccine immunization significantly reduced clinical scores post-challenge
The protective efficacy of the FHV-1 MLV vaccine against FHV-1 was further evaluated by challenging cats with FHV-1 strain 64. As depicted in Figures 4A,B, cats in the control group exhibited severe clinical symptoms, including mouth breathing, mucoid ocular and nasal discharge, and sneezing. In contrast, vaccinated cats showed only mild clinical symptoms, and mock cats did not show any clinical symptoms. Clinical scores were evaluated as described previously (22, 23), and the results indicated a significant reduction in clinical scores in the vaccinated group compared to the control group (Table 1; Figure 4C; p < 0.05). A transient temperature increase was observed after the FHV-1 challenge in both groups, with temperatures exceeding 40°C in several control cats (Figure 4D). Moreover, as shown in Figure 4E, cats in the control group shed viral DNA in eyelid secretions for up to 2 weeks. In contrast, cats in the MLV vaccine group did not shed the virus until day 4 after infection for 5 days, and no viral shedding was detected in the mock group. These data suggest that the MLV vaccine significantly reduced clinical signs and greatly reduced FHV-1 shedding in cats.
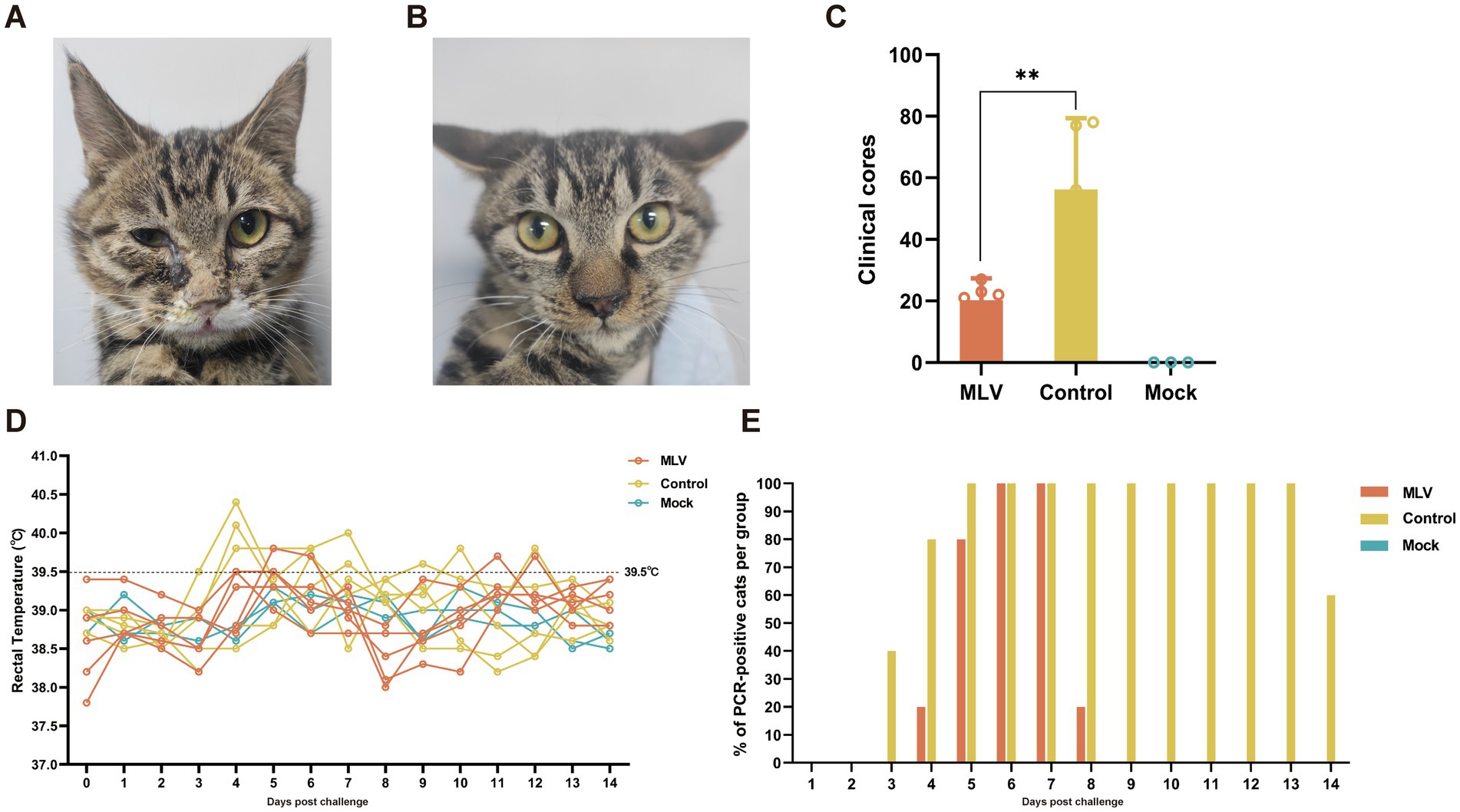
Figure 4. Clinical symptoms and clinical scores post FHV-1 challenge. (A) Cats infected with FHV-1 showed mucoid nasal or ocular discharge and blepharospasm in the control group. (B) Immunized cats infected with FHV-1 showed mild nasal discharge. (C) Statistical analysis of cumulative clinical scores in cats after FHV-1 infection, from day 36 to 49 (n = 13), the results were considered statistically significant at p < 0.05. (D) Rectal temperature of cats after challenge. Fever is defined as rectal temperature > 39.4°C. (E) The percentage of PCR-positive cats per group.
Pathological examination 14 days post-infection revealed minimal lesions in immunized cats, while in control cats, lesions were limited to eyelids, turbinates, tracheas, and lungs, characterized by focal necrosis and desquamation of epithelial cells. Moreover, FHV-1 inclusion bodies were observed in the epithelial cells of the nasal mucosa (Figure 5A). Similarly, immunohistochemical (IHC) assays against FHV-1 showed positive staining in all control samples and negative staining in the MLV vaccine group (Figure 5B). Given that the cats in the mock group exhibited no clinical symptoms and no viral DNA was detected in their eyelid secretions, pathological examinations were not conducted on this group in consideration of animal welfare. These results indicated that the MLV vaccine confers potent protection against FHV-1 infection in cats.
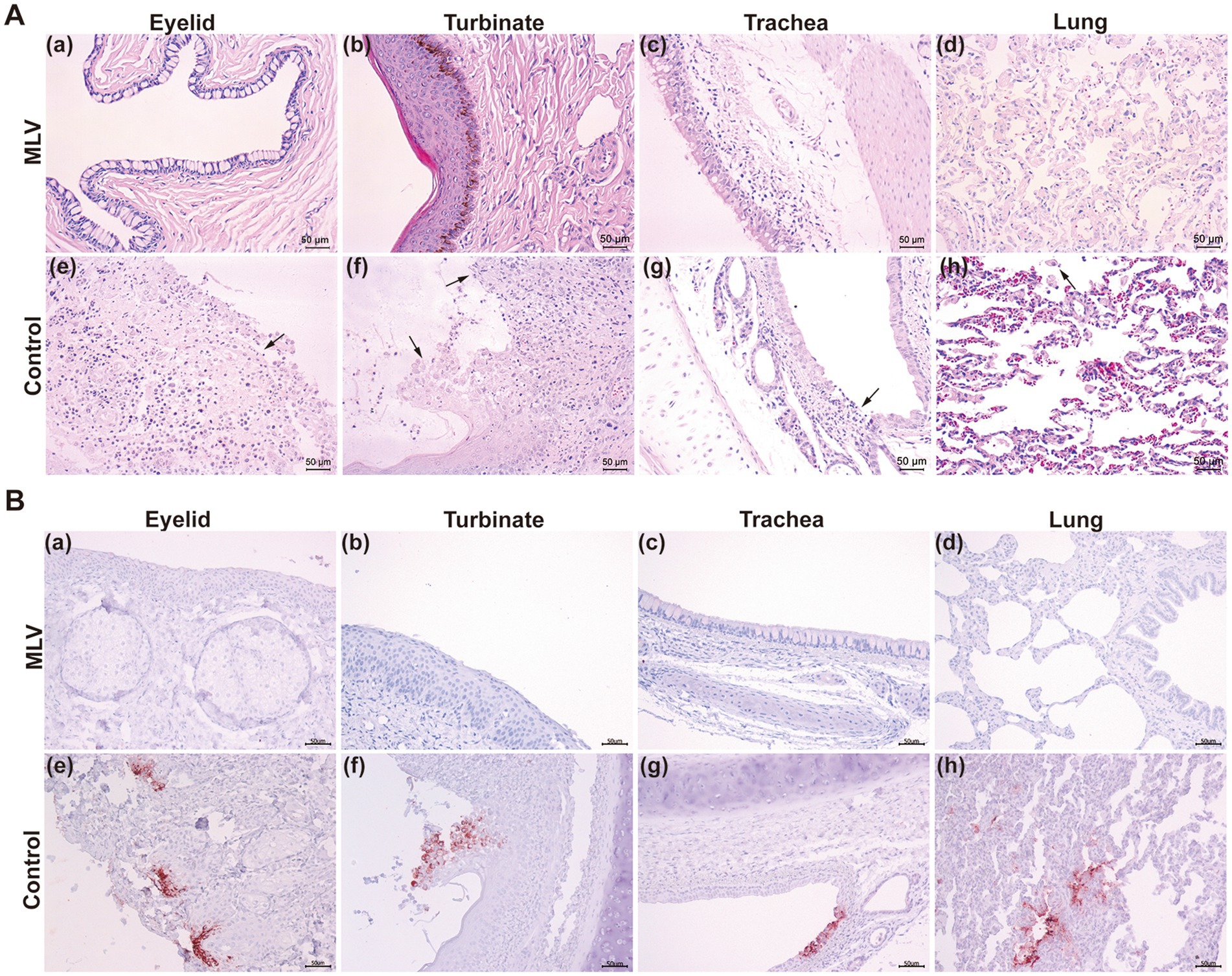
Figure 5. Histological changes post FHV-1 challenge. (A) Histopathological lesions of cats after challenge. Necropsy was performed on all cats. The eyelids, turbinates, tracheas, and lungs were collected and subjected to H&E staining. The black arrows represent tissue lesions. (B) IHC assays of cats after challenge.
3.5 Correlation analysis between clinical scores post-FHV-1 challenges and detection indicators
Correlation analysis revealed significant negative correlations between clinical scores and VN titers (p < 0.05), IgG antibody titers (p < 0.05), IFN-γ SFC (p < 0.05), CD4 + T cells (p < 0.05), and CD8 + T cells (p < 0.05). Moderate inverse correlations were observed between clinical scores and IL-2 SFC, approaching significance (p > 0.05) (Figure 6).
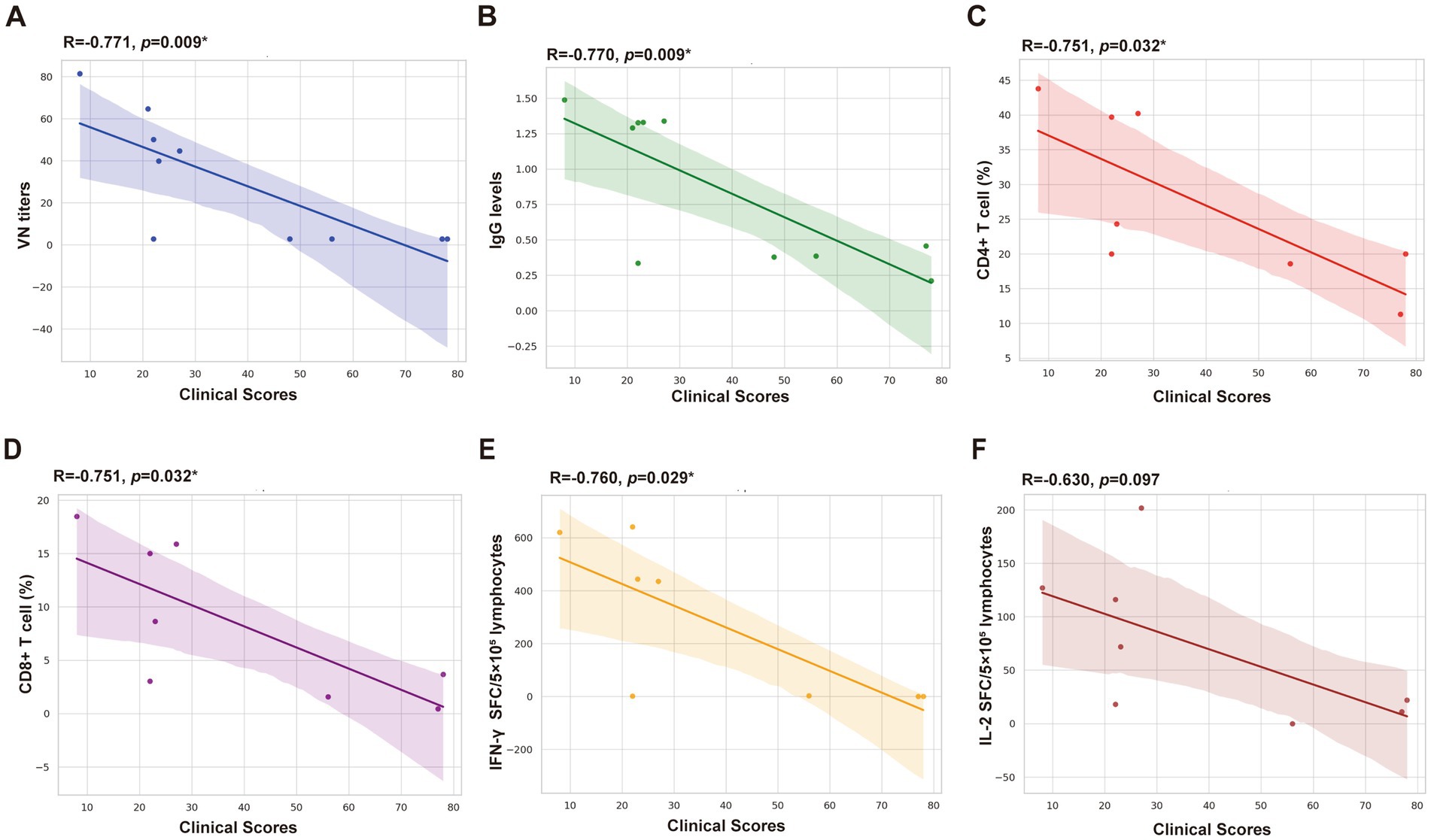
Figure 6. Correlation analysis between clinical scores and detection indicators. (A) VN titers. (B) IgG levels (OD 450 nm). (C) CD4+ T cell (%). (D) CD8+ T cell (%). (E) IFN-γ SFC/5 × 105 lymphocytes. (F) IL-2 SFC/5 × 105 lymphocytes.
4 Discussion
FHV-1 infection has become an important infectious disease that seriously endangers cats (21). Despite numerous studies on novel vaccines for FHV-1 and the availability of commercial options, the immune effects of these vaccines are inconsistent, and the disease can still develop after immunization (26). Although the FHV-1 MLV or inactivated vaccine may counteract viral infection, like FHV-1 infection, their detectable FHV-1 neutralizing antibodies after vaccination only occur in a limited number of cats with low titers (1). Several studies have confirmed this, including (27), who reported that only 22% of cats had a titer increase, with the magnitude of titer increases generally being low.
In the current study, serum neutralization antibodies and IgG levels were measured to identify the antibodies that neutralize or bind harmful particles and prevent infection. Consistent with (28), the FHV-1 MLV vaccine elicited a serological response in cats that received two doses. Typically, multiple immunizations are required to produce detectable seroconversion, regardless of whether the vaccine was attenuated or inactivated (29).
The challenge assay results align with previous research, demonstrating the vaccine’s significant clinical protection compared to the unvaccinated control group (28, 30). The vaccinated cats exhibited remarkably low clinical scores after the challenge, with symptoms limited to mild nasal or ocular discharge, mild nasal congestion, and sneezing for 2–3 days. In contrast, unvaccinated cats showed severe clinical signs. Analysis revealed a significant correlation between the clinical signs and antibodies, including VN titers and IgG levels, indicating that the humoral immune response generated by vaccination offered protection. The relationship between elevated antibody levels and reduced clinical symptoms post-FHV-1 challenge suggests that humoral immunity plays a vital role in eliminating non-bound viruses and preventing host cell invasion, thereby defending against infection (31). Consistent with other alpha herpesviruses such as equine herpesvirus-1 (EHV-1) and varicella zoster virus (VZV), it is essential to consider other immune mechanisms in preventing FHV-1 infections (32).
CD8+ T cells, or cytotoxic T lymphocytes (CTLs), are crucial in combating viral infections. Following antigen presentation, CD8+ T cells undergo clonal expansion and differentiate into effector cells, producing cytokines like IFN-γ. Meanwhile, CD4+ T cells differentiate into various subsets, such as Th1 cells, which produce IFN-γ and stimulate B cells to produce antibodies (33). As EHV-1 efficiently enters host cells and evades antibody neutralization in horses, T cells play a vital role in clearing replicating herpesviruses (34). CD8+ T lymphocytes primarily contribute to limiting the spread of infectious agents by recognizing and eliminating infected cells and secreting antiviral cytokines, while CD4+ T cells also play a role in this (35, 36). Consequently, the percentage of CD4+ and CD8 + T cells in the lymphocytes of immune-challenged, challenged-only, and mock cats was analyzed, which revealed a significant increase in the quantity of CD4+ T cells and CD8+ T cells in the immunized group compared to the control and mock group. These results indicate a robust immunological response induced by the FHV-1 MLV vaccine. The elevated CD8+ T cell levels suggest an effective cytotoxic response against FHV-1 infection, which is crucial for eliminating infected cells and preventing pathogen dissemination (37). The concurrent increase in both T cell populations highlights the comprehensive nature of the immune response elicited by the FHV-1 MLV vaccine, validating its effectiveness in inducing a robust cellular immune response.
Th1 T-cells (characteristically secrete IFN-γ, TNF-α, and IL-2) and CD8+ T cells contribute to the control of the alpha herpesviruses, and Th1-related cytokines like IFN-γ and IL-2 have been detected in responses to other alpha herpesviruses vaccines (24). Consistent with this, the current study depicted that the MLV vaccine induced significant levels of IL-2 in cats, with undetectable IL-4 in the serum, representing a Th1-biased cellular immune response. Although this study indicated no significant increase in IFN-γ levels compared to the control group, this may be attributed to the ELISA assay’s limited sensitivity, low vaccine-induced IFN-γ levels, or the short duration of IFN-γ in serum. To further investigate this, we employed ELISpot assay, which revealed that the MLV vaccine enhanced the secretion of IFN-γ and IL-2, associated with Th1 cells that mediate cellular immune responses and protect cells from pathogenic microorganisms (38, 39). Similarly, the live attenuated shingles vaccine Zostavax has been shown to induce a Th1-type cellular immune response that prevents VZV infection (35).
The discussed data revealed various differential factors after the immunization with the FHV-1 MLV vaccine, prompting further analysis of the correlation between clinical scores and detection indicators. The results depict a significant correlation between clinical scores and CD8+ T cells, CD4+ T cells, and IFN-γ SFC. These findings suggest that the MLV vaccine primarily activates the Th1-type cellular immune response, leading to CTLs differentiation and release of the IFN-γ, which clears the pathogen and confers resistance to FHV-1 infection (16, 39). The analysis of VN antibodies, IgG levels, and cytokines indicates that the FHV-1 MLV vaccine induces both humoral and cellular immune responses in cats, protecting against FHV-1 infection.
5 Conclusion
The findings of the study substantiate the immunological efficacy of the FHV-1 MLV vaccine. Immunized cats exhibited a Th1-type cellular immune response, as evidenced by the high levels of VN antibodies, IgG levels, and elevated levels of IFN-γ and IL-2. Furthermore, the clinical scores were substantially negatively correlated with increased IFN-γ levels that protected cats from FHV-1 infection. Overall, the FHV-1 MLV vaccine displays effective immunogenicity, and this study serves as a foundation for future FHV-1 vaccine research.
Data availability statement
The original contributions presented in the study are included in the article/supplementary material, further inquiries can be directed to the corresponding authors.
Ethics statement
The animal study was approved by the Animal Experiments of the National Research Center for Veterinary Medicine. The study was conducted in accordance with the local legislation and institutional requirements.
Author contributions
HW: Methodology, Writing – original draft, Writing – review & editing. PQ: Data curation, Methodology, Writing – original draft, Writing – review & editing. YC: Methodology, Writing – review & editing. CL: Methodology, Supervision, Writing – review & editing. NH: Methodology, Writing – review & editing. HD: Writing – review & editing, Resources. XW: Methodology, Writing – review & editing. LW: Methodology, Writing – review & editing. XX: Methodology, Writing – review & editing. YL: Project administration, Resources, Writing – review & editing. KT: Project administration, Resources, Writing – review & editing, Supervision.
Funding
The author(s) declare that no financial support was received for the research, authorship, and/or publication of this article.
Acknowledgments
We thank Feifei Tan for her help and advice with flow cytometry and ELISpot assay. We additionally thank Xiuling Yu, and Zhe Sun for excellent technical assistance with histological analysis.
Conflict of interest
The authors declare that the research was conducted in the absence of any commercial or financial relationships that could be construed as a potential conflict of interest.
Generative AI statement
The authors declare that no Generative AI was used in the creation of this manuscript.
Publisher’s note
All claims expressed in this article are solely those of the authors and do not necessarily represent those of their affiliated organizations, or those of the publisher, the editors and the reviewers. Any product that may be evaluated in this article, or claim that may be made by its manufacturer, is not guaranteed or endorsed by the publisher.
References
1. Gaskell, R, Dawson, S, Radford, A, and Thiry, E. Feline herpesvirus. Vet Res. (2007) 38:337–54. doi: 10.1051/vetres:2006063
2. Maes, R. Felid herpesvirus type 1 infection in cats: a natural host model for alphaherpesvirus pathogenesis. ISRN Vet Sci. (2012) 2012:495830:1–14. doi: 10.5402/2012/495830
3. Veir, JK, Ruch-Gallie, R, Spindel, ME, and Lappin, MR. Prevalence of selected infectious organisms and comparison of two anatomic sampling sites in shelter cats with upper respiratory tract disease. J Feline Med Surg. (2008) 10:551–7. doi: 10.1016/j.jfms.2008.04.002
4. Sykes, JE. Pediatric feline upper respiratory disease. Vet Clin North Am Small Anim Pract. (2014) 44:331–42. doi: 10.1016/j.cvsm.2013.10.005
5. Friedl, Y, Schulz, B, Knebl, A, Helps, C, Truyen, U, and Hartmann, K. Efficacy of passively transferred antibodies in cats with acute viral upper respiratory tract infection. Vet J. (2014) 201:316–21. doi: 10.1016/j.tvjl.2014.05.002
6. Nelli, RK, Maes, R, Kiupel, M, and Hussey, GS. Use of a feline respiratory epithelial cell culture system grown at the air-liquid interface to characterize the innate immune response following feline herpesvirus 1 infection. Virus Res. (2016) 214:39–48. doi: 10.1016/j.virusres.2016.01.006
7. Gaskell, R, and Willoughby, K. Herpesviruses of carnivores. Vet Microbiol. (1999) 69:73–88. doi: 10.1016/S0378-1135(99)00092-9
8. Day, MJ, Horzinek, MC, Schultz, RD, and Squires, RA. WSAVA guidelines for the vaccination of dogs and cats. J Small Anim Pract. (2016) 57:E1–e45. doi: 10.1111/jsap.2_12431
9. Capozza, P, Pratelli, A, Camero, M, Lanave, G, Greco, G, Pellegrini, F, et al. Feline coronavirus and alpha-herpesvirus infections: innate immune response and immune escape mechanisms. Animals. (2021) 11:11. doi: 10.3390/ani11123548
10. Tian, J, Liu, Y, Liu, X, Sun, X, Zhang, J, and Qu, L. Feline herpesvirus 1 US3 blocks the type I interferon signal pathway by targeting interferon regulatory factor 3 dimerization in a kinase-independent manner. J Virol. (2018) 92:e00047–18. doi: 10.1128/JVI.00047-18
11. Rivas, AE, Langan, JN, Colegrove, KM, Terio, K, and Adkesson, MJ. Herpesvirus and calicivirus infection in a black-footed cat (Fels nigripes) family group following vaccination. J Zoo Wildlife Med. (2015) 46:141–5. doi: 10.1638/2013-0216R1.1
12. Pennings, A, Seeley, K, Mathieu, A, Foust, A, Garner, MM, and Ramer, J. Feline herpesvirus infection in four captive cheetahs (Acinonyx Jubatus) postvaccination. J Zoo Wildlife Med. (2020) 51:210–6. doi: 10.1638/2019-0041
13. Hou, J, Wang, S, Li, D, Carpp, LN, Zhang, T, Liu, Y, et al. Early pro-inflammatory signal and T-cell activation associate with vaccine-induced anti-vaccinia protective neutralizing antibodies. Front Immunol. (2021) 12:737487. doi: 10.3389/fimmu.2021.737487
14. Schoenborn, JR, and Wilson, CB. Regulation of interferon-gamma during innate and adaptive immune responses. Adv Immunol. (2007) 96:41–101. doi: 10.1016/S0065-2776(07)96002-2
15. Danastas, K, Miranda-Saksena, M, and Cunningham, AL. Herpes simplex virus type 1 interactions with the interferon system. Int J Mol Sci. (2020) 21:21. doi: 10.3390/ijms21145150
16. Levings, RL, and Roth, JA. Immunity to bovine herpesvirus 1: II. Adaptive immunity and vaccinology. Anim Health Res Rev. (2013) 14:103–23. doi: 10.1017/S1466252313000054
17. Jiao, C, Jin, H, Zhang, M, Liu, D, Huang, P, Bai, Y, et al. A bacterium-like particle vaccine displaying protective feline herpesvirus 1 antigens can induce an immune response in mice and cats. Vet Microbiol. (2023) 287:109898. doi: 10.1016/j.vetmic.2023.109898
18. Wu, Q, Wu, H, He, S, Liu, Y, Chen, Y, Qi, X, et al. Feline herpesvirus infection and pathology in captive snow leopard. Sci Rep. (2022) 12:4989. doi: 10.1038/s41598-022-08994-4
19. Brunner, C, Kanellos, T, Meli, ML, Sutton, DJ, Gisler, R, Gomes-Keller, MA, et al. Antibody induction after combined application of an adjuvanted recombinant FeLV vaccine and a multivalent modified live virus vaccine with a chlamydial component. Vaccine. (2006) 24:1838–46. doi: 10.1016/j.vaccine.2005.10.030
20. Kanellos, T, Sutton, DJ, Salisbury, CF, and Chalmers, WS. Combined administration in a single injection of a feline multivalent modified live vaccine against FHV, FCV, and FPLV together with a recombinant FeLV vaccine is both safe and efficacious for all four major feline viral pathogens. J Feline Med Surg. (2008) 10:346–54. doi: 10.1016/j.jfms.2008.02.001
21. Liu, C, Liu, Y, Qian, P, Cao, Y, Wang, J, Sun, C, et al. Molecular and serological investigation of cat viral infectious diseases in China from 2016 to 2019. Transbound Emerg Dis. (2020) 67:2329–35. doi: 10.1111/tbed.13667
22. Summers, SC, Ruch-Gallie, R, Hawley, JR, and Lappin, MR. Effect of modified live or inactivated feline herpesvirus-1 parenteral vaccines on clinical and laboratory findings following viral challenge. J Feline Med Surg. (2017) 19:824–30. doi: 10.1177/1098612X16659333
23. Lee, Y, Maes, RK, Kruger, JM, Kiupel, M, Giessler, KS, and Soboll, HG. Safety and efficacy of felid Herpesvirus-1 deletion mutants in cats. Viruses. (2021) 13:13. doi: 10.3390/v13020163
24. Ren, X, Cao, N, Tian, L, Liu, W, Zhu, H, Rong, Z, et al. A self-assembled nanoparticle vaccine based on pseudorabies virus glycoprotein D induces potent protective immunity against pseudorabies virus infection. Vet Microbiol. (2023) 284:109799. doi: 10.1016/j.vetmic.2023.109799
25. Croft, M, So, T, Duan, W, and Soroosh, P. The significance of OX40 and OX40L to T-cell biology and immune disease. Immunol Rev. (2009) 229:173–91. doi: 10.1111/j.1600-065X.2009.00766.x
26. Gourkow, N, Lawson, JH, Hamon, SC, and Phillips, CJ. Descriptive epidemiology of upper respiratory disease and associated risk factors in cats in an animal shelter in coastal western Canada. Can Vet J. (2013) 54:132–8.
27. Bergmann, M, Speck, S, Rieger, A, Truyen, U, and Hartmann, K. Antibody response to feline herpesvirus-1 vaccination in healthy adult cats. J Feline Med Surg. (2020) 22:329–38. doi: 10.1177/1098612X19845702
28. Jas, D, Aeberlé, C, Lacombe, V, Guiot, AL, and Poulet, H. Onset of immunity in kittens after vaccination with a non-adjuvanted vaccine against feline panleucopenia, feline calicivirus and feline herpesvirus. Vet J. (2009) 182:86–93. doi: 10.1016/j.tvjl.2008.05.025
29. Jas, D, Frances-Duvert, V, Vernes, D, Guigal, PM, and Poulet, H. Three-year duration of immunity for feline herpesvirus and calicivirus evaluated in a controlled vaccination-challenge laboratory trial. Vet Microbiol. (2015) 177:123–31. doi: 10.1016/j.vetmic.2015.03.009
30. Tang, A, Zhu, M, Zhu, J, Zhang, D, Zhu, S, Wang, X, et al. Pathogenicity and immunogenicity of gI/gE/TK-gene-deleted felid herpesvirus 1 variants in cats. Virol J. (2023) 20:87. doi: 10.1186/s12985-023-02053-8
31. Umehashi, M, Imamura, T, Akiyama, S, Matsuda, J, Tokiyoshi, S, Tohya, Y, et al. Pre-exposure treatment of cats with anti-FHV-1 and anti-FCV mouse-cat chimeric antibodies. J Vet Med Sci. (2003) 65:563–6. doi: 10.1292/jvms.65.563
32. Zhang, J, Liu, H, and Wei, B. Immune response of T cells during herpes simplex virus type 1 (HSV-1) infection. J Zhejiang Univ Sci B. (2017) 18:277–88. doi: 10.1631/jzus.B1600460
33. Laidlaw, BJ, Craft, JE, and Kaech, SM. The multifaceted role of CD4(+) T cells in CD8(+) T cell memory. Nat Rev Immunol. (2016) 16:102–11. doi: 10.1038/nri.2015.10
34. van der Meulen, KM, Favoreel, HW, Pensaert, MB, and Nauwynck, HJ. Immune escape of equine herpesvirus 1 and other herpesviruses of veterinary importance. Vet Immunol Immunopathol. (2006) 111:31–40. doi: 10.1016/j.vetimm.2006.01.006
35. Sullivan, NL, Eberhardt, CS, Wieland, A, Vora, KA, Pulendran, B, and Ahmed, R. Understanding the immunology of the Zostavax shingles vaccine. Curr Opin Immunol. (2019) 59:25–30. doi: 10.1016/j.coi.2019.02.005
36. Li, S, Sullivan, NL, Rouphael, N, Yu, T, Banton, S, Maddur, MS, et al. Metabolic phenotypes of response to vaccination in humans. Cell. (2017) 169:862–77.e17. doi: 10.1016/j.cell.2017.04.026
37. Zhou, S, Ou, R, Huang, L, Price, GE, and Moskophidis, D. Differential tissue-specific regulation of antiviral CD8+ T-cell immune responses during chronic viral infection. J Virol. (2004) 78:3578–600. doi: 10.1128/JVI.78.7.3578-3600.2004
38. Ruterbusch, M, Pruner, KB, Shehata, L, and Pepper, M. In vivo CD4(+) T cell differentiation and function: revisiting the Th1/Th2 paradigm. Annu Rev Immunol. (2020) 38:705–25. doi: 10.1146/annurev-immunol-103019-085803
Keywords: feline herpesvirus 1, MLV vaccine, cats, cellular immune responses, IFNγ
Citation: Wu H, Qiao P, Chen Y, Liu C, Huo N, Ding H, Wang X, Wang L, Xi X, Liu Y and Tian K (2025) Cellular and humoral immune responses in cats vaccinated with feline herpesvirus 1 modified live virus vaccine. Front. Vet. Sci. 11:1516850. doi: 10.3389/fvets.2024.1516850
Edited by:
Simon Paul Graham, The Pirbright Institute, United KingdomReviewed by:
Rory Cristiane Fortes De Brito, The Pirbright Institute, United KingdomNattawooti Sthitmatee, Chiang Mai University, Thailand
Xinming Tang, Chinese Academy of Agricultural Sciences, China
Copyright © 2025 Wu, Qiao, Chen, Liu, Huo, Ding, Wang, Wang, Xi, Liu and Tian. This is an open-access article distributed under the terms of the Creative Commons Attribution License (CC BY). The use, distribution or reproduction in other forums is permitted, provided the original author(s) and the copyright owner(s) are credited and that the original publication in this journal is cited, in accordance with accepted academic practice. No use, distribution or reproduction is permitted which does not comply with these terms.
*Correspondence: Yuxiu Liu, cWluZ3Fpbmd5dXhpdTFAMTYzLmNvbQ==; Kegong Tian, dmV0dmFjQDEyNi5jb20=