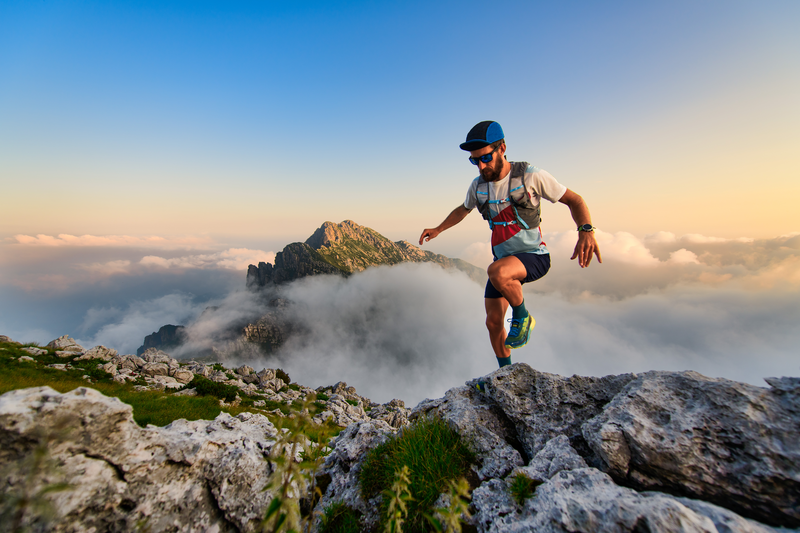
95% of researchers rate our articles as excellent or good
Learn more about the work of our research integrity team to safeguard the quality of each article we publish.
Find out more
ORIGINAL RESEARCH article
Front. Vet. Sci. , 09 January 2025
Sec. Veterinary Pharmacology and Toxicology
Volume 11 - 2024 | https://doi.org/10.3389/fvets.2024.1515977
Pasteurella multocida (Pm) is a widespread zoonotic pathogen with the ability to infect wild animals, livestock, and humans. Pm infection can cause haemorrhagic pneumonia, indicating that the pathogenesis involves serious vascular injury and inflammation. 18β-Glycyrrhetinic acid (GA) has cardiovascular protective and anti-inflammatory effects, but its effect on vascular injury caused by Pm infection is not clear. This study focused on the protective effects of GA on Pm-induced vascular inflammatory injury in mice. The results showed that GA intervention significantly improved the survival rate and the changes in haematological and biochemical parameters caused by Pm infection in mice. Haematoxylin and eosin staining revealed that GA delayed the progression of vascular injury, including abnormalities in elastic fibres, local rupture of the vascular intima, and inflammatory cell infiltration in response to Pm infection. The immunohistochemical results showed that after the GA intervention, the vascular inflammatory response in Pm-infected mice was alleviated. These protective effects may be related to the reduced expression of poly (ADP-ribose) polymerase-1, high mobility group box 1, interleukin-1β, and interleukin-18 in vascular tissue by GA. These findings suggest that GA inhibits the activation of inflammation to protect vascular injury in vivo. Hence, GA exhibits therapeutic potential in the treatment of vascular injury.
Pasteurella multocida (Pm) is an opportunistic zoonotic pathogen that has the ability to infect a diverse range of hosts, including wild and domestic animals, and humans (1–3). Pm can be categorised into five groups (A, B, D, E and F) based on the bacterial capsular characteristics (4). Among them, capsular type A mainly causes porcine pneumonia, and can also cause septicaemia and death of pigs in serious cases (5, 6). Pm infection is characterised by acute respiratory disease accompanied by hyperthermia, dyspnoea, and death within a few days. The primary lesions observed upon postmortem examination are suppurative bronchopneumonia, necrohaemorrhagic fibrinous pleuropneumonia, fibrinous pericarditis, and peritonitis (7). Haemorrhagic pneumonia caused by Pm infection involves necrosis of the vessel walls (7) and severe damage to the vascular endothelium with increased permeability (8), suggesting that there the pathogenesis involves serious vascular injury and inflammation. Healthy vascular endothelial function can provide an antioxidant, anti-inflammatory, and antithrombotic interface for the body, which plays a vital role in maintaining the health and homeostasis of multiple organs (9). The vascular response is the central link of the inflammatory process and is the key to the occurrence and development of infectious systemic inflammatory response (10–12). At present, antibiotics and vaccines are mainly used to treat and prevent Pm infection in veterinary medicine (13–16), but they cannot solve the problem of vascular inflammatory injury caused by Pm infection. Therefore, it is urgent to seek candidate drugs to control the vascular inflammatory injury associated with Pm infection in pigs.
In traditional medicine, liquorice is used to treat respiratory diseases, hyperphagia, fever, gastric ulcer, rheumatism, skin diseases, haemorrhagic diseases, and jaundice (17). 18β-Glycyrrhetinic acid (GA), a major active component obtained from liquorice, shows a broad range of biological properties, including antibacterial, anti-inflammatory, antiviral, antioxidant, antiasthmatic, and anticancer activities, and hepatoprotective and cardioprotective effects (18, 19). Researchers have shown that GA can alleviate vascular injury caused by various factors. GA administration significantly improved the pathological changes in the pulmonary vasculature induced by monocrotaline in rats (20). Moreover, GA alleviated endoplasmic reticulum stress–induced inflammation in pulmonary arterial hypertension (21) and pulmonary artery injuries in rats with high-altitude pulmonary hypertension (22). In addition, poly (ADP-ribose) polymerase-1 (PARP1) is closely related to vascular endothelial dysfunction and the activation of inflammatory cells (23). In our previous study, we demonstrated that GA could alleviate Pm-induced vascular endothelial inflammatory injury by PARP1-mediated nuclear factor kappa B (NF-κB) and high mobility group box 1 (HMGB1) signalling suppression in porcine vascular endothelial cells (PIECs) (8). These studies have demonstrated that GA has beneficial effects on vascular inflammatory injury. However, whether it also protects against vascular inflammatory injury caused by Pm infection in vivo remains to be investigated.
The purpose of this study was to investigate the protective effects of GA on Pm-induced vascular inflammatory injury in vivo, and to provide additional evidence that GA could be used as a new antibiotic substitute or feed additive for adjuvant treatment of inflammatory injury. The results provide a theoretical basis for clinical relief of vascular inflammatory injury caused by Pm infection.
GA (CAS No.: 471–53-4) was purchased from MedChemExpress (USA) and Dimethyl sulfoxide (CAS No.: 67-68-5) was purchased from Sigma-Aldrich (Germany).
All animal experiments were approved by the Animal Ethics Committee of Wuhan Polytechnic University under permit number WPU202311004.
The Pm HB03 strain was kindly provided by Prof. Bin Wu (Huazhong Agricultural University, Wuhan, China) and cultured in Tryptose Soya Broth (Hopebio, China) with 5% (v/v) Newborn Calf Serum (Tianhang, China) at 37°C.
Specific pathogen–free female BALB/c mice (20–25 g in weight) were purchased from the Center of Laboratory Animals of Hubei Province, Wuhan, China. During the experiment, the temperature of the animal room was 22 ± 1°C, and the mice had free access to food and water. To avoid stress in mice, they were acclimatised for 3 days and then randomly divided into five groups, followed by drug treatment and Pm challenge. There five groups were: control (n = 10), Pm challenge (n = 10), 10 mg/kg body weight (b.w.) GA intervention (n = 10), 20 mg/kg b.w. GA intervention (n = 10) and 40 mg/kg b.w. GA intervention (n = 10). The infection was established by intraperitoneal injection of Pm. The challenge dose was 100 μL of 80 colony-forming units of Pm HB03 (24); the control group was injected with an equivalent volume of phosphate-buffered saline (PBS). The intervention group was injected with GA solution (10, 20 and 40 mg/kg b.w.) intramuscularly, and the control and challenge groups were injected with the same volume of PBS. The mice were administered GA for the first time on the day of challenge, 2 h before the Pm challenge, and then once a day for 5 consecutive days. The survival rate was assessed daily up to 5 days. Prior to euthanasia, the mice were fasted overnight.
Blood samples were collected from the mice at sacrifice to analyse haematological and serum biochemical parameters. The haematological parameters were determined using a BC-5000 vet Hematology Analyzer (Mindray, China). The following haematological parameters were determined: the number of white blood cells (WBC), neutrophils (Neu), lymphocytes (Lym), and eosinophils (Eos). For the biochemical parameters, heparinised blood samples were centrifuged for 10 min at 3500 rpm and 4°C; then, the supernatant was collected. An automatic blood biochemical analyzer (HITACHI 7100; Hitachi, Tokyo, Japan) was used to determine total bilirubin (TBIL), total protein (TP), albumin (ALB), aspartate aminotransferase (AST) activity, alanine aminotransferase (ALT) activity, triglycerides (TG), glucose (GLU), and creatinine (CRE). All haematological and biochemical parameters were measured by following standard procedures.
After collecting vascular tissue samples from the mice, they were fixed in 4% neutral buffered paraformaldehyde (Servicebio, China), dehydrated through a graded alcohol series, embedded in paraffin wax, and cut into 5-μm sections. The sections were stained with a haematoxylin and eosin staining kit (Baiqiandu, China), and the histopathological changes in the vascular tissues were examined using a microscope with and an imaging system (NIKON, Japan).
The tissue distribution of the PARP1, HMGB1, interleukin-1β (IL-1β), and interleukin-18 (IL-18) proteins was detected using IHC analysis. In brief, paraffin sections of vascular tissues were blocked with 3% bovine serum albumin solution after antigen retrieval. Subsequently, these sections were incubated with a PARP1 polyclonal antibody (Proteintech, China), an HMGB1 polyclonal antibody (Abclonal, China), an IL-1β polyclonal antibody (Abclonal, China), or an IL-18 polyclonal antibody (Abclonal, China) overnight at 4°C. After washing with 1× PBS several times, the sections were incubated with goat anti-rabbit IgG conjugated to horseradish peroxidase (Abclonal, China) for 50 min. Finally, the sections were incubated at room temperature with a 3,3′-diaminobenzidine kit (zsbio, China), counterstained with haematoxylin (Baiqiandu, China), and encapsulated in neutral resin film (Baiqiandu, China) following conventional dewatering. The slides were evaluated using the light microscope with an imaging system (NIKON, Japan).
The results are presented as mean ± standard deviation. SPSS Statistics 18.0 for Windows (SPSS Inc., Chicago, IL, United States) was used for statistical analysis. Statistical significance was accepted for p < 0.05.
To study the protective effect of GA on Pm infection, we established a mouse model of Pm infection. Compared with the control group, the Pm challenge group showed symptoms of listlessness, crouching, anorexia, and depression, and their fur began to lose its lustre. The GA intervention groups showed marked improvement in these symptoms. Meanwhile, Figure 1 shows the survival rate of the mice. The survival rate on day 5 was lower in the Pm challenge and GA intervention groups compared with the control group, but the GA intervention groups showed a higher survival rate compared with the Pm challenge group. This suggests that GA alleviates Pm infection–induced death.
Table 1 shows the results of the haematological analysis. Compared with the control group, the Pm challenge group showed a significant increase in the number of WBC, Neu, and Eos, and a significant decrease in the number of Lym. Compared with the Pm challenge group, the 20 mg/kg b.w. GA intervention group showed a significant decrease in the number of WBC, and the 40 mg/kg b.w. GA intervention group showed a significant decrease in the number of WBC and Neu. The results indicated that Pm-infected mice developed an inflammatory injury, and GA could alleviate this abnormality.
Table 2 shows the results of the biochemical analysis. Compared with the control group, the TBIL, AST, CRE, TP and ALT levels were significantly increased in the Pm challenge group, while the ALB and GLU levels were significantly decreased. Compared with the Pm challenge group, the 20 mg/kg b.w. GA intervention group showed a significant decrease in the ALT level and a significant increase in the GLU and ALB levels; the 40 mg/kg b.w. GA intervention group showed a significant decrease in the CRE and ALT levels. The results showed that Pm infection caused liver and kidney dysfunction and abnormal glucose metabolism, and GA could alleviate these abnormalities.
Next, we evaluated the effect of GA on the Pm-induced histopathological changes in the blood vessels (Figure 2). The control showed a normal vascular structure (Figure 2A). Compared with the control group, the Pm challenge group showed some unclear elastic fibres that were disordered and partially broken (Figure 2B, black arrow), local rupture of the vascular intima (Figure 2B, green arrow) and a large number of inflammatory cells (Figure 2B, yellow arrow). Compared with the Pm challenge group, the GA intervention groups showed fewer inflammatory cells in the blood vessels and a reduction in the structural damage to the elastic fibres (Figures 2C–E). The results showed that GA could improve vascular inflammatory injury caused by Pm infection.
Figure 2. The effect of GA on vascular pathological changes in Pm-infected mice. Bar = 50 μm (A) Control group; (B) Pm infection group; (C) 10 mg/kg b.w. GA intervention group; (D) 20 mg/kg b.w. GA intervention group; (E) 40 mg/kg b.w. GA intervention group.
We further explored the effect of GA on the expression of inflammatory proteins in the blood vessels by performing IHC analysis (Figures 3–6). Compared with the control group, the Pm challenge group showed significantly increased PARP1, HMGB1, IL-1β, and IL-18 protein expression in the blood vessels, indicating that Pm infection in mice triggered vascular inflammatory injury. Compared with the Pm challenge group, the GA intervention groups showed reduced PARP1, HMGB1, IL-1β and IL-18 protein levels in vascular tissue to varying degrees depending on the GA dose. Thus, GA had a protective effect on vascular inflammatory injury in Pm-infected mice.
Figure 3. The effect of GA on PARP1 protein expression in vascular tissue from Pm-infected mice. Bar = 20 μm (A) Control group; (B) Pm infection group; (C) 10 mg/kg b.w. GA intervention group; (D) 20 mg/kg b.w. GA intervention group; (E) 40 mg/kg b.w. GA intervention group.
Figure 4. The effect of GA on HMGB1 protein expression in vascular tissue from Pm-infected mice. Bar = 20 μm (A) Control group; (B) Pm infection group; (C) 10 mg/kg b.w. GA intervention group; (D) 20 mg/kg b.w. GA intervention group; (E) 40 mg/kg b.w. GA intervention group.
Figure 5. The effect of GA on IL-1β protein expression in vascular tissue from Pm-infected mice. Bar = 20 μm (A) Control group; (B) Pm infection group; (C) 10 mg/kg b.w. GA intervention group; (D) 20 mg/kg b.w. GA intervention group; (E) 40 mg/kg b.w. GA intervention group.
Figure 6. The effect of GA on IL-18 protein expression in vascular tissue from Pm-infected mice. Bar = 20 μm (A) Control group; (B) Pm infection group; (C) 10 mg/kg b.w. GA intervention group; (D) 20 mg/kg b.w. GA intervention group; (E) 40 mg/kg b.w. GA intervention group.
Pm is a gram-negative bacterium that can infect both domestic animals and humans, leading to large economic losses to the livestock industry (25). Haemorrhagic pneumonia caused by Pm infection characterised by the haemorrhagic multifocal areas, necrosis of the vessel walls, and associated with suppurative inflammatory exudation (7), which means that Pm infection can induce vascular inflammatory injury. In veterinary clinical practice, vaccination and antibiotics are mainly used to prevent and treat Pm infection (26). However, this approach cannot solve the problem of vascular inflammatory injury caused by Pm infection, and the widespread illegal use of antibiotics can easily lead to the development of drug resistance (27–29). Moreover, natural products have the potential to serve as candidate drugs, thereby exerting a relieving effect on vascular inflammatory injury (30, 31). Therefore, it is of great clinical significance to study the vascular inflammatory injury caused by Pm infection and the regulatory effects of natural products. In the present study, we used Pm to construct a mouse model of vascular injury, and explored the role of GA in alleviating this vascular inflammatory injury. We found that the GA intervention significantly improved the vascular pathological changes and reduced the expression of vascular inflammatory proteins caused by Pm infection in mice (Figure 7). Elucidating the regulatory role of GA in vascular inflammatory injury will provide a theoretical basis for the prevention and control of Pm infection and the development of alternative antibiotic therapies and feed additives.
Figure 7. Schematic representation of the proposed protective effects of GA on Pm-induced vascular inflammatory injury in mice. In this study, vascular injury was established in mice via Pm infection, and the ability of GA to reduce this injury was explored. GA could significantly ameliorate the abnormal haematological and biochemical parameters and vascular pathological changes in mice caused by Pm infection, and reduce the expression of vascular inflammatory proteins.
Previous studies have shown that GA alleviates the inflammatory injury of PIECs caused by Pm infection (8). Mice are a useful animal model to study Pm infection (32), and to explore whether GA has protective effect on vascular inflammatory injury caused by Pm infection in vivo. Mice infected by Pm show a dull coat, depression, anorexia, wrinkles, and reluctance to move, and the lungs are congested and bleed (33). In this study, Pm-infected mice also showed similar clinical symptoms to those of Pm-infected mice, such as appetite loss, messy fur, and depression (32). Compared with the control group, the Pm challenge group presented significantly increased mortality (24, 34), a finding consistent with the fact that the Pm HB03 strain is classified as highly virulent. Natural extracts have therapeutic effects on animals infected by Pm (35). We found that GA, isolated from liquorice, could improve the survival rate of mice compared with the Pm challenge group, demonstrating the potential therapeutic effect of GA.
When a host is infected by a pathogen, the body responds to the invading pathogen, and there are changes in haematological and biochemical parameters. Praveena et al. (36) reported that after Pm infection in mice, the number of Neu increased significantly, the number of Lym decreased significantly, and the TP level in the blood increased, changes that are consistent with the results of the present study. Meanwhile, biomarkers of liver and kidney injury (AST, ALT, and CRE) increase significantly in the Pm-infected mice (37). We noted similar results changes in the blood biochemical parameters in the present study, indicating that Pm infection in mice impairs liver and kidney function. In a previous study, researchers reported elevated serum CRE and lactate dehydrogenase levels in BALB/c mice with renal injury; treatment with 10, 25, or 50 mg/kg b.w. GA significantly decreased these biochemical parameters (38). In the present study, slightly difference GA concentrations alleviated the abnormal haematological and biochemical parameters in mice after Pm infection, suggesting that GA may alleviate the inflammatory reaction in mice caused by Pm infection.
The invasion of a host by pathogenic bacteria can lead to congestion, swelling, and even bleeding at the infection site, accompanied by an inflammatory reaction. Researchers have found that mice infected by Pm show pulmonary congestion, obvious vasodilation, haemorrhage, serum protein exudation, leucocyte recruitment, and other phenomena (33, 39). Pm-infected mice show a marked inflammatory cell infiltration in the alveolar wall and a large number of red blood cells in the alveolar cavity, indicating local haemorrhage (40). Our histopathological examination revealed that the intima of blood vessels in the Pm-infected mice was damaged, accompanied by marked inflammatory cell infiltration. GA could effectively alleviate this vascular inflammatory injury caused by Pm infection. In chickens, the inflammatory factors HMGB1 and IL-6 are widely expressed in the lungs after Pm infection (41). Moreover, bacterial infection can promote the expression of PARP1 and the activation of inflammation related pathways (42, 43), and the inhibition of PARP1 has been repeatedly confirmed by experimental models to improve the inflammatory response and increase the survival rate (44, 45). In addition, our previous study showed that GA could alleviate Pm-induced vascular endothelial inflammation via PARP1-mediated HMGB1 signalling suppression in PIECs (8). Consistent with those prior findings, we found that Pm infection increased PARP1, HMGB1, IL-1β, and IL-18 protein expression in vascular tissue, while GA reduced the expression of these inflammatory factors. These data suggest that GA might ameliorate vascular inflammatory injury in mice via a PARP1–HMGB1 signalling pathway.
In conclusion, we showed that in a mouse model of Pm infection, GA significantly reduced Pm-induced vascular inflammatory injury, and decreased PARP1, HMGB1, IL-1β, and IL-18 protein expression in vascular tissue. PARP1-mediated HMGB1 signalling suppression may represent a critical pathway for treating Pm-induced vascular inflammatory injury. We have provided data that should be useful for preclinical and clinical studies that evaluate the potential of GA to prevent vascular inflammatory injury caused by Pm infection.
The original contributions presented in the study are included in the article/supplementary material, further inquiries can be directed to the corresponding authors.
The animal study was approved by all animal experiments were approved by the Animal Ethics Committee of Wuhan Polytechnic University. The study was conducted in accordance with the local legislation and institutional requirements.
QL: Conceptualization, Funding acquisition, Investigation, Methodology, Project administration, Supervision, Writing – original draft. LW: Formal analysis, Investigation, Methodology, Validation, Writing – original draft. XJ: Investigation, Methodology, Validation, Writing – original draft. WH: Formal analysis, Validation, Visualization, Writing – original draft. PG: Data curation, Visualization, Writing – review & editing. YL: Conceptualization, Formal analysis, Project administration, Writing – original draft. SF: Data curation, Writing – review & editing. JX: Data curation, Writing – review & editing. ZW: Data curation, Writing – review & editing. YQ: Conceptualization, Formal analysis, Funding acquisition, Project administration, Writing – review & editing.
The author(s) declare financial support was received for the research, authorship, and/or publication of this article. This research was funded by National Natural Science Foundation of China (NSFC) (32202867), Key Research and Development Plan of Hubei Province, China (2023BBB069) and the Open Project of Hubei Key Laboratory of Animal Nutrition and Feed Science (202404).
We thank the Professor Bin Wu at Huazhong Agricultural University (Wuhan, China) for the gift of P. multocida HB03 strain.
The authors declare that the research was conducted in the absence of any commercial or financial relationships that could be construed as a potential conflict of interest.
The author(s) declare that no Gen AI was used in the creation of this manuscript.
All claims expressed in this article are solely those of the authors and do not necessarily represent those of their affiliated organizations, or those of the publisher, the editors and the reviewers. Any product that may be evaluated in this article, or claim that may be made by its manufacturer, is not guaranteed or endorsed by the publisher.
ALB, albumin; ALT, alanine aminotransferase; AST, aspartate aminotransferase; CRE, creatinine; Eos, eosinophils; GA, 18β-glycyrrhetinic acid; GLU, glucose; HMGB1, high mobility group box 1; IL-1β, interleukin-1β; IL-18, interleukin-18; Lym, lymphocytes; Neu, neutrophils; NF-κB, nuclear factor kappa B; PARP1, poly (ADP-ribose) polymerase-1; PBS, phosphate-buffered saline; PIECs, porcine vascular endothelial cells; Pm, Pasteurella multocida; TBIL, total bilirubin; TG, triglycerides; TP, total protein; WBC, white blood cells.
1. Lin, L, Bi, H, Yang, J, Shang, Y, Lv, Q, Zhang, D, et al. Pasteurella multocida infection induces blood-brain barrier disruption by decreasing tight junctions and adherens junctions between neighbored brain microvascular endothelial cells. Vet Res. (2024) 55:104. doi: 10.1186/s13567-024-01351-5
2. Piorunek, M, Brajer-Luftmann, B, and Walkowiak, J. Pasteurella Multocida infection in humans. Pathogens. (2023) 12:1210. doi: 10.3390/pathogens12101210
3. Guan, LJ, Song, JJ, Xue, Y, Ai, X, Liu, ZJ, Si, LF, et al. Immune protective efficacy of China's traditional inactivated and attenuated vaccines against the prevalent strains of Pasteurella multocida in mice. Vaccines (Basel). (2021) 9:1155. doi: 10.3390/vaccines9101155
4. Peng, Z, Wang, X, Zhou, R, Chen, H, Wilson, BA, and Wu, B. Pasteurella multocida: genotypes and genomics. Microbiol Mol Biol Rev. (2019) 83:e00014–9. doi: 10.1128/MMBR.00014-19
5. Kim, J, Kim, JW, Oh, SI, So, B, Kim, WI, and Kim, HY. Characterisation of Pasteurella multocida isolates from pigs with pneumonia in Korea. BMC Vet Res. (2019) 15:119. doi: 10.1186/s12917-019-1861-5
6. de Oliveira, JX, Morés, MAZ, Rebelatto, R, Agnol, AMD, Plieski, CLA, Klein, CS, et al. Pasteurella multocida type a as the primary agent of pneumonia and septicaemia in pigs. Pesqui Vet Bras. (2015) 35:716–24. doi: 10.1590/S0100-736X2015000800003
7. Oliveira Filho, JX, Morés, MAZ, Rebellato, R, Kich, JD, Cantão, ME, Klein, CS, et al. Pathogenic variability among Pasteurella multocida type a isolates from Brazilian pig farms. BMC Vet Res. (2018) 14:244. doi: 10.1186/s12917-018-1565-2
8. Lu, Q, Han, W, Wen, D, Guo, P, Liu, Y, Wu, Z, et al. 18β-Glycyrrhetinic acid alleviates P. multocida-induced vascular endothelial inflammation by PARP1-mediated NF-κB and HMGB1 signalling suppression in PIEC cells. Infect Drug Resist. (2023) 16:4201–12. doi: 10.2147/IDR.S413242
9. Xu, S, Ilyas, I, Little, PJ, Li, H, Kamato, D, Zheng, X, et al. Endothelial dysfunction in atherosclerotic cardiovascular diseases and beyond: from mechanism to pharmacotherapies. Pharmacol Rev. (2021) 73:924–67. doi: 10.1124/pharmrev.120.000096
10. Hellenthal, KEM, Brabenec, L, and Wagner, NM. Regulation and dysregulation of endothelial permeability during systemic inflammation. Cells. (2022) 11:1935. doi: 10.3390/cells11121935
11. Sprague, AH, and Khalil, RA. Inflammatory cytokines in vascular dysfunction and vascular disease. Biochem Pharmacol. (2009) 78:539–52. doi: 10.1016/j.bcp.2009.04.029
12. Hattori, Y, Hattori, K, Machida, T, and Matsuda, N. Vascular endotheliitis associated with infections: its pathogenetic role and therapeutic implication. Biochem Pharmacol. (2022) 197:114909. doi: 10.1016/j.bcp.2022.114909
13. Chen, Y, Ji, X, Zhang, S, Wang, W, Zhang, H, and Ding, H. Pharmacokinetic/pharmacodynamic integration of tilmicosin against Pasteurella multocida in a piglet tissue cage model. Front Vet Sci. (2023) 10:1260990. doi: 10.3389/fvets.2023.1260990
14. Wu, MC, Wu, HC, Lee, JW, Chang, WC, and Chu, CY. A protein-based subunit vaccine with biological adjuvants provides effective protection against Pasteurella multocida in pigs. Vet Res. (2023) 54:17. doi: 10.1186/s13567-023-01150-4
15. Somogyi, Z, Mag, P, Simon, R, Kerek, Á, Makrai, L, Biksi, I, et al. Susceptibility of Actinobacillus pleuropneumoniae, Pasteurella multocida and Streptococcus suis isolated from pigs in Hungary between 2018 and 2021. Antibiotics (Basel). (2023) 12:1298. doi: 10.3390/antibiotics12081298
16. Guan, LJ, Yang, JQ, Xu, QY, Feng, YF, Zhang, XC, Tang, B, et al. Immunogenicity and efficacy of serogroup a and D bacterins against Pasteurella multocida in mice. Front Vet Sci. (2023) 10:1132536. doi: 10.3389/fvets.2023.1132536
17. El-Saber Batiha, G, Magdy Beshbishy, A, El-Mleeh, A, Abdel-Daim, MM, and Prasad Devkota, H. Traditional uses, bioactive chemical constituents, and pharmacological and toxicological activities of Glycyrrhiza glabra L. (Fabaceae). Biomolecules. (2020) 10:352. doi: 10.3390/biom10030352
18. Kowalska, A, and Kalinowska-Lis, U. 18β-Glycyrrhetinic acid: its core biological properties and dermatological applications. Int J Cosmet Sci. (2019) 41:325–31. doi: 10.1111/ics.12548
19. Shinu, P, Gupta, GL, Sharma, M, Khan, S, Goyal, M, Nair, AB, et al. Pharmacological features of 18β-glycyrrhetinic acid: a pentacyclic triterpenoid of therapeutic potential. Plants (Basel). (2023) 12:1086. doi: 10.3390/plants12051086
20. Zhang, M, Chang, Z, Zhao, F, Zhang, P, Hao, YJ, Yan, L, et al. Protective effects of 18β-glycyrrhetinic acid on monocrotaline-induced pulmonary arterial hypertension in rats. Front Pharmacol. (2019) 10:13. doi: 10.3389/fphar.2019.00013
21. Wang, JL, Liu, H, Jing, ZC, Zhao, F, and Zhou, R. 18β-Glycyrrhetinic acid ameliorates endoplasmic reticulum stress-induced inflammation in pulmonary arterial hypertension through PERK/eIF2α/NF-κB signaling. Chin J Physiol. (2022) 65:187–98. doi: 10.4103/0304-4920.354801
22. Yang, T, Zhou, J, Fang, L, Wang, M, Dilinuer, M, and Ainiwaer, A. Protection function of 18β-glycyrrhetinic acid on rats with high-altitude pulmonary hypertension based on (1)H NMR metabonomics technology. Anal Biochem. (2021) 631:114342. doi: 10.1016/j.ab.2021.114342
23. Safdar, R, Mishra, A, Shah, GM, and Ashraf, MZ. Poly (ADP-ribose) Polymerase-1 modulations in the genesis of thrombosis. J Thromb Thrombolysis. (2024) 57:743–53. doi: 10.1007/s11239-024-02974-3
24. Chen, Y, Sun, E, Yang, L, Song, J, and Wu, B. Therapeutic application of bacteriophage PHB02 and its putative depolymerase against Pasteurella multocida capsular type a in mice. Front Microbiol. (2018) 9:1678. doi: 10.3389/fmicb.2018.01678
25. Elsayed, M, Eldsouky, SM, Roshdy, T, Said, L, Thabet, N, Allam, T, et al. Virulence determinants and antimicrobial profiles of Pasteurella multocida isolated from cattle and humans in Egypt. Antibiotics (Basel). (2021) 10:480. doi: 10.3390/antibiotics10050480
26. Wang, Y, Zeng, Z, Ran, J, Peng, L, Wu, X, Ye, C, et al. The critical role of potassium efflux and Nek7 in Pasteurella multocida-induced NLRP3 inflammasome activation. Front Microbiol. (2022) 13:849482. doi: 10.3389/fmicb.2022.849482
27. Dayao, DA, Gibson, JS, Blackall, PJ, and Turni, C. Antimicrobial resistance in bacteria associated with porcine respiratory disease in Australia. Vet Microbiol. (2014) 171:232–5. doi: 10.1016/j.vetmic.2014.03.014
28. Truswell, A, Laird, TJ, Jones, S, O'Dea, M, Blinco, J, Abraham, R, et al. Antimicrobial resistance of and genomic insights into Pasteurella multocida strains isolated from Australian pigs. Microbiol Spectr. (2023) 11:e0378422. doi: 10.1128/spectrum.03784-22
29. Petrocchi-Rilo, M, Gutiérrez-Martín, CB, Pérez-Fernández, E, Vilaró, A, Fraile, L, and Martínez-Martínez, S. Antimicrobial resistance genes in porcine Pasteurella multocida are not associated with its antimicrobial susceptibility pattern. Antibiotics (Basel). (2020) 9:614. doi: 10.3390/antibiotics9090614
30. Jiang, F, and Dusting, GJ. Natural phenolic compounds as cardiovascular therapeutics: potential role of their antiinflammatory effects. Curr Vasc Pharmacol. (2003) 1:135–56. doi: 10.2174/1570161033476736
31. Deng, ZY, Hu, MM, Xin, YF, and Gang, C. Resveratrol alleviates vascular inflammatory injury by inhibiting inflammasome activation in rats with hypercholesterolemia and vitamin D2 treatment. Inflamm Res. (2015) 64:321–32. doi: 10.1007/s00011-015-0810-4
32. Fu, Q, Jiang, J, Li, X, Zhai, Z, Wang, X, Li, C, et al. Activation of MyD88-dependent TLR signaling modulates immune response of the mouse heart during Pasteurella multocida infection. Microorganisms. (2023) 11:400. doi: 10.3390/microorganisms11020400
33. Priya, GB, Nagaleekar, VK, Milton, AAP, Saminathan, M, Kumar, A, Sahoo, AR, et al. Genome wide host gene expression analysis in mice experimentally infected with Pasteurella multocida. PLoS One. (2017) 12:e0179420. doi: 10.1371/journal.pone.0179420
34. Zhou, G, Tian, J, Tian, Y, Ma, Q, Li, Q, Wang, S, et al. Recombinant-attenuated Salmonella enterica serovar Choleraesuis vector expressing the PlpE protein of Pasteurella multocida protects mice from lethal challenge. BMC Vet Res. (2023) 19:128. doi: 10.1186/s12917-023-03679-0
35. El-Sheikh, SMA, Youssef, FM, Mohamed, HI, El-Saber Batiha, G, Albrakati, A, and Galal, AAA. Efficacy of grape seed hydro-alcoholic extract in the treatment of experimentally Pasteurella multocida infected rabbits. Vet Med Sci. (2021) 7:923–34. doi: 10.1002/vms3.446
36. Praveena, PE, Periasamy, S, Kumar, AA, and Singh, N. Cytokine profiles, apoptosis and pathology of experimental Pasteurella multocida serotype A1 infection in mice. Res Vet Sci. (2010) 89:332–9. doi: 10.1016/j.rvsc.2010.04.012
37. Zhao, G, Tang, Y, Liu, X, Li, P, Zhang, T, Li, N, et al. Pasteurella multocida activates Rassf1-hippo-yap pathway to induce pulmonary epithelial apoptosis. Vet Res. (2024) 55:31. doi: 10.1186/s13567-024-01285-y
38. Wu, CH, Chen, AZ, and Yen, GC. Protective effects of glycyrrhizic acid and 18β-glycyrrhetinic acid against cisplatin-induced nephrotoxicity in BALB/c mice. J Agric Food Chem. (2015) 63:1200–9. doi: 10.1021/jf505471a
39. Wu, Q, Yu, L, Qiu, J, Shen, B, Wang, D, Soromou, LW, et al. Linalool attenuates lung inflammation induced by Pasteurella multocida via activating Nrf-2 signaling pathway. Int Immunopharmacol. (2014) 21:456–63. doi: 10.1016/j.intimp.2014.05.030
40. Cheng, Y, Wang, K, Lin, L, Zhao, X, Pan, Z, and Zhou, Z. Differences in pathogenicity and virulence-associated gene expression among Pasteurella multocida strains with high and low virulence in a lung tissue model. Microb Pathog. (2020) 140:103911. doi: 10.1016/j.micpath.2019.103911
41. Li, W, Tang, Q, Dai, N, Feng, W, Xie, C, Cheng, G, et al. Receptor-interacting serine/threonine kinase 1- and 3-dependent inflammation induced in lungs of chicken infected with Pasteurella multocida. Sci Rep. (2020) 10:6340. doi: 10.1038/s41598-020-62042-7
42. Nossa, CW, Jain, P, Tamilselvam, B, Gupta, VR, Chen, LF, Schreiber, V, et al. Activation of the abundant nuclear factor poly(ADP-ribose) polymerase-1 by Helicobacter pylori. Proc Natl Acad Sci USA. (2009) 106:19998–20003. doi: 10.1073/pnas.0906753106
43. Altmeyer, M, Barthel, M, Eberhard, M, Rehrauer, H, Hardt, WD, and Hottiger, MO. Absence of poly(ADP-ribose) polymerase 1 delays the onset of Salmonella enterica serovar typhimurium-induced gut inflammation. Infect Immun. (2010) 78:3420–31. doi: 10.1128/IAI.00211-10
44. Wasyluk, W, and Zwolak, A. PARP inhibitors: an innovative approach to the treatment of inflammation and metabolic disorders in sepsis. J Inflamm Res. (2021) 14:1827–44. doi: 10.2147/JIR.S300679
Keywords: 18β-glycyrrhetinic acid, inflammatory response, Pasteurella multocida , protective effects, vascular injury
Citation: Lu Q, Wang L, Jiang X, Han W, Guo P, Liu Y, Fu S, Xiong J, Wu Z and Qiu Y (2025) Protective effects of 18β-glycyrrhetinic acid on Pasteurella multocida–induced vascular inflammatory injury in mice. Front. Vet. Sci. 11:1515977. doi: 10.3389/fvets.2024.1515977
Received: 23 October 2024; Accepted: 26 December 2024;
Published: 09 January 2025.
Edited by:
Fan Yang, Henan University of Science and Technology, ChinaReviewed by:
Jianzhong Wang, Shanxi Agricultural University, ChinaCopyright © 2025 Lu, Wang, Jiang, Han, Guo, Liu, Fu, Xiong, Wu and Qiu. This is an open-access article distributed under the terms of the Creative Commons Attribution License (CC BY). The use, distribution or reproduction in other forums is permitted, provided the original author(s) and the copyright owner(s) are credited and that the original publication in this journal is cited, in accordance with accepted academic practice. No use, distribution or reproduction is permitted which does not comply with these terms.
*Correspondence: Yu Liu, bHl5d2Z5QHdocHUuZWR1LmNu; Yinsheng Qiu, cWl1eWluc2hlbmc2NDA1QHdocHUuZWR1LmNu
†These authors have contributed equally to this work and share first authorship
Disclaimer: All claims expressed in this article are solely those of the authors and do not necessarily represent those of their affiliated organizations, or those of the publisher, the editors and the reviewers. Any product that may be evaluated in this article or claim that may be made by its manufacturer is not guaranteed or endorsed by the publisher.
Research integrity at Frontiers
Learn more about the work of our research integrity team to safeguard the quality of each article we publish.