- 1CAS Key Laboratory of Agro-Ecological Processes in Subtropical Region, Hunan Provincial Key Laboratory of Animal Nutritional Physiology and Metabolic Process, Institute of Subtropical Agriculture, Chinese Academy of Sciences, Changsha, Hunan, China
- 2College of Advanced Agricultural Sciences, University of Chinese Academy of Sciences, Beijing, China
- 3Department of Monogastric Animals, Institute of Animal Science, San José de Las Lajas, Mayabeque, Cuba
Introduction: The study aimed to investigate the effects of Chlorella vulgaris and lysozyme on growth performance, diarrhea rate, immune function, plasma biochemical parameters, and gut microbiota and metabolites of weaned piglets.
Methods: Thirty weaned Xiangcun black piglets (BW, 11.47 ± 1.13 kg) were randomly assigned to one of three treatment groups: corn-soybean meal-based basal diet (CON group), soybean meal replaced with 5% C. vulgaris (CHV group), and soybean meal replaced with 5% C. vulgaris and 100 mg/kg lysozyme (LYSO group).
Results: Growth performance was not affected by C. vulgaris or C. vulgaris with lysozyme supplementation, while soybean meal partially replaced by C. vulgaris without lysozyme reduced the diarrhea rate of weaned piglets. Plasma biochemical analysis showed that plasma albumin, alkaline phosphatase, and high-density lipoprotein-cholesterol (HDL-C) levels in the CHV group and the total cholesterol and HDL-C levels in the LYSO group were higher when compared with the CON group. The LYSO group had increased interleukin (IL)-10 level in the jejunum and IL-1β level in the ileum while having a decreasing IL-6 level in the jejunum of piglets. Additionally, although Firmicutes and Megashaera_A abundances and short-chain fatty acid concentrations (including acetate, propionate, butyrate, and valerate) were reduced in the CHV group, but several beneficial bacteria (such as Actinobacteroita, Faecealibacterium, and Anaerovibrio) abundances were increased in the LYSO group.
Discussion: In summary, dietary C. vulgaris or C. vulgaris with lysozyme supplementation improved health of piglets in some contexts without affecting growth performance. Therefore, soybean meal replaced by 5% C. vulgaris with or without lysozyme as sustainable feed ingredients in piglet diets could be a viable alternative approach.
1 Introduction
China is the largest pig producer in the world, and the pig industry is one of the important sources of the agricultural economy in China. Feed resources for pig production, especially corn and soybeans, are the main raw materials for high-quality feed (1). To meet the high-quality protein requirements for livestock production, China is mostly dependent on imported conventional protein sources crops such as soybeans and other feedstuffs, accounting for more than 80% of the total demands of the country, which limits the sustainable development of the pig industry. Additionally, protein sources from soybeans are also directly in competition with human nutrition. Therefore, the use of non-conventional feed resources as protein alternatives, such as agricultural by-products, insect meals, microalgae, etc., has gained the recent most important global research topic for sustainable animal production.
Microalgae has become one of the most non-conventional feed resources for livestock production due to their faster growth rate, higher biomass productivity and biodiversity, and no competition with other conventional protein resource land requirements (2). Microalgae, multicellular marine microorganisms, have gained more interest in animal nutrition due to their rich composition of essential nutrients, such as crude protein and bioactive compounds (3). Chlorella vulgaris, a green microalga, has been found as a promising feed resource for animal production with similar crude protein contents up to 67% in dry matter, with digestibility comparable to the soybean-based meal (3). In addition, C. vulgaris is also a rich source of polysaccharides, lipids, minerals, and vitamins (4, 5). In this context, the application of C. vulgaris in livestock feed resources would be a potential research target for crucial protein replacement and other bioactive compounds that can enhance animal immune response, disease inhibition, and microbiome colonization (6). However, like other microalgae such as Arthrospira platensis, C. vulgaris displays several challenges in nutrient availability when included in monogastric diets due to its recalcitrant cell wall. Indeed, several recent studies in vitro and in vivo have shown that exogenous carbohydrate-active enzymes (CAZymes) could effectively enhance the nutrient utilization of microalgae by monogastric animals (6, 7). For example, Spirulina (A. platensis) supplementation with CAZymes (such as lysozyme and Rovabio® Excel AP) increased the dry matter digestibility in weaned piglets without affecting the animal performance compared to the piglets fed without enzyme (8). Therefore, lysozyme, a well-known carbohydrate cell wall degrading enzyme, can break down insoluble mucopolysaccharides into soluble glycopeptides, inducing the damaged cell wall to release its internal contents and easily digested in the gastrointestinal tract of animals (7). However, the underlying mechanism of C. vulgaris and lysozyme in the metabolism consequences of pigs still needs to be fully uncovered.
Therefore, we hypothesize that dietary supplementation of C. vulgaris and lysozyme could exert beneficial effects in weaned piglets and thus allow them to be utilized as partial replacement for conventional feeding strategies. Thus, the present study aimed to explore the effects of partial replacement of a conventional protein source (soybean meal) from a corn-soybean meal-based diet with 5% C. vulgaris with or without lysozyme on growth performance, diarrhea rate, immunity, plasma biochemical parameters, and intestinal microbiota and metabolites in weaned Xiangcun black pigs. The outcomes of this study will provide important guiding significance for the rational use of non-conventional feed resources in pig production.
2 Materials and methods
2.1 Animals, diets, and management
Thirty weaned Xiangcun black pigs (28 days of age; half male and half female; male piglets were castrated) were selected for the feeding trial. After 7 days of the adaptation period, piglets with an average body weight (BW) of 11.47 ± 1.13 kg were randomly allocated into three groups with 10 pigs (replicates) per group. Three groups included the control group (CON, fed a corn-soybean meal-based basal diet), C. vulgaris group (CHV, fed a corn-soybean meal-based basal diet and soybean meal replaced with 5% C. vulgaris), and lysozyme group (LYSO, fed a corn-soybean meal-based basal diet and soybean meal replaced with 5% C. vulgaris and 100 mg/kg lysozyme). The feeding trial lasted 56 days. Dietary C. vulgaris and lysozyme were uniformly mixed with the basal diets. Dietary C. vulgaris was obtained from Xian Saiyang Biotechnology Co. Ltd. (Xian, China), and lysozyme was provided by the Sunson Bioscience Technology Development Co. Ltd. (Beijing, China). The supplementing dose of C. vulgaris and lysozyme was based on the previous findings (9, 10). The experimental diet’s ingredients and proximal composition were formulated (Table 1) to meet the Chinese nutrient requirements for domestic pigs in China (NY/T65-2004).
Experimental pigs were housed in individual pens (1.10 × 0.60 m) equipped with an adjusted drinking water nipple, single-hole feeder, and plastic-slatted floored room. The piggery was facilitated with forced ventilation and environmentally controlled temperature (23–26°C) and humidity (60 ± 5%). All pigs had free access to water and food at all times. All experimental animals enrolled in this study were in good health conditions, and had no antibiotic exposure or any gastrointestinal diseases before the trial.
2.2 Sample collection and processing
At the end of the feeding trial and 12 h after fasting, all pigs were selected for sampling after weighing. Blood samples (10 mL) were collected from the anterior vena cava of each pig into anticoagulated vacuum blood collection tubes, mixed upside down, and then centrifuged for 10 min at 3,500 × g and 4°C to obtain the plasma. The plasma samples were immediately stored at −80°C for further analyses of immunoglobulins and immuno-cytokines. Afterward, all pigs were anesthetized with an intramuscular injection of Zoletil® 50 (Tiletamine and zolazepam; Beijing Lab Anim. Tech. Dev. Co. Ltd., Beijing, China) and exsanguinated to collect jejunum, ileum, and colon samples. The samples (2–3 cm) of the jejunum (10 cm below the flexure of duodenum-jejunum) and ileum (10 cm above the ileo-cecal junction) were excised, flushed in phosphate buffer solution, and then scrapped by glass slides. The mucosa scrapings (~2 g) were sampled, snap-frozen in liquid nitrogen, and immediately stored at −80°C to determine intestinal immuno-cytokines. Colonic contents (mid-section) were harvested into 1.5 mL sterile tubes and stored at −80°C for colonic microbiota and metabolite analyses.
2.3 Determination of growth performance and diarrhea rate
The initial and final BW of each pig was measured to calculate the average daily gain (ADG). Feed consumption and feed refusal of each pig were recorded daily to calculate the average daily feed intake (ADFI) of pigs. The ratio of feed intake to weight gain (F/G) was calculated. The diarrhea rate of experimental pigs was evaluated daily using Hart and Dobb’s method as described previously (11, 12). Briefly, the diarrheal conditions of pigs were recorded daily as a fecal score of 0 for normal, 1 for mild diarrhea, 2 for moderate diarrhea, and 3 for severe diarrhea, respectively. The diarrhea rate (%) was calculated as follows:
Total diarrhea times/(total number of piglets × experimental days) × 100.
2.4 Determination of plasma biochemical parameters and immunoglobulins
Plasma biochemical parameters, including total protein (TP), albumin (ALB), ammonia (AMM), triglycerides (TG), total cholesterol (TC), high-density lipoprotein-cholesterol (HDL-C), low-density lipoprotein-cholesterol (LDL-C), and glucose (GLU) concentrations, as well as alpha-amylase (α-AMY), alanine aminotransferase (ALT), aspartate aminotransferase (AST), alkaline phosphatase (ALP), lactate dehydrogenase (LDH), and cholinesterase (CHE) activities were analyzed using their corresponding available kits (F. Hoffmann-La Roche Ltd., Basel, Switzerland) and instruments (Cobas c311, Basel, Switzerland). The levels of plasma immunoglobulins, including immunoglobulin A (IgA, RC-01340P2), IgG (RC-01237P2), and IgM (RC-01236P2) were determined using the commercially available ELISA kits (Haiyang Biological Co. Ltd., Changsha, China) and following the guidelines provided by the manufacturer.
2.5 Analysis of plasma and intestinal immuno-cytokines
The concentrations of plasma, jejunal, and ileal interleukin (IL)-1β (RC-01256P1), IL-2 (RC-01255P1), IL-6 (RC-01252P1), IL-10 (RC-01255P1), IL-17 (RC-01211P1), tumor necrosis factor (TNF)-α (RC-01217P1), and interferon (IFN)-γ (RC-01246P1) were evaluated with commercially available porcine-specific ELISA kits (Haiyang Biological Co. Ltd., Changsha, China) according the guidelines provided by the company. Absorbance values were read on a Multiscan Spectrophotometer (Infinite M200 PRO; TECAN, Männedorf, Switzerland).
2.6 Colonic microbial DNA extraction, 16S rRNA pyrosequencing, and bioinformatics analysis
Approximately 0.30 g of colonic contents of each sample were thawed and homogeneously mixed for bacterial genomic DNA extraction with a Mag-Bind® Stool DNA kit (Omega, Guangzhou, China). The concentration and quality of the extracted DNA were determined with the NanoDrop OneC miniature Volume UV–Vis spectrophotometer (Thermo Fisher Scientific, Waltham, MA, USA), and agarose gel electrophoresis was used for resulting PCR products. The hypervariable V4–V5 region of the colonic bacterial 16S rRNA genes was amplified with universal forward primer 338F (5′-ACTCCTACGGGGAGGCAGCA-3′) and reverse primer 806R (5′-GGACTACHVGGGGTWTCTAAT-3′). The PCR reaction thermal cycle conditions were followed by the manufacturer’s standard protocols (New England Biolab. Inc., Ipswich, MA, USA). The obtained PCR products were purified with Vazyme VAHTSTM DNA Clean Beads to remove non-specific products, and then the constructed DNA libraries were quantitatively analyzed using the Quant-iT PicoGreen dsDNA Detection Kit (Invitrogen, Carlsbad, CA, USA). Based on the standard protocols of Shanghai Personal Biotech. Co. Ltd. (Shanghai, China), purified amplicons were subjected to equimolar and pair-end (2 × 300) sequencing by the MiSeq Reagent kit on an Illumina MiSeq platform (Illumina, San Diego, CA, USA).
The raw sequence data obtained from the Illumina Miseq were processed using the DADA2 plugin and QIIME2 for sequence and subsequent data analysis as previously described (13). After that, using the default parameter, a representative sequence was selected from each operational taxonomic unit (OTU) and classified based on BLAST searching against the Greengenes database. The alpha-diversity indices, including Chao 1, Shannon index, Simpson index, and Observed_species, were calculated using the OTU table and QIIM2. Beta-diversity analysis was performed using principal coordinate analysis (PCoA) and non-measured multidimensional scaling (NMDS) plots based on the Bray-Curtis distance metric to identify the structural differences in microbial communities among samples. The differences in the taxonomic composition of colonic microbiota at the phylum and genus levels were evaluated using the Kruskal-Wallis test. The linear discriminant analysis size effect (LEfSe) was performed to analyze the taxonomic hierarchical distribution of marker species in each group of samples using the histograms of the distribution of linear regression analysis (LDA) values of different species and species taxonomic branching diagrams (Cladograms) to present the significantly enriched species and their levels of importance in each group of samples.
2.7 Determination of colonic microbial metabolites
The short-chain fatty acid (SCFA), including straight-chain fatty acids (acetate, propionate, butyrate, and valerate) and branched-chain fatty acids (isobutyrate and isovalerate) concentrations in the colonic contents of weaned pigs were measured with the gas chromatography (7890A; Agilent Technologies Inc., Santa Clara, CA, USA), following the previously described protocols (14). Briefly, colonic contents (1.00 g) were homogeneously mixed with ultrapure water (5 mL), and supernatants were obtained by centrifuging at 1000 × g and 4°C for 10 min. The obtained supernatants were mixed with 25% metaphosphoric acid at a 9:1 (v/v) ratio in 2 mL sterile centrifuge tubes, centrifuged at 2000 × g and 4°C for 10 min, and then filtered through a 0.45-μm polysulfone filter for gas chromatography analysis.
2.8 Statistical analysis
Data analyses of growth performance, plasma biochemical parameters, immunoglobulins, and plasma and intestinal immuno-cytokines were analyzed by one-way analysis of variance (ANOVA). Multiple comparisons among different groups were evaluated using Tukey’s post-hoc test with the SPSS 26.0 software package (IBM Inc., Chicago, IL, USA). In addition, two pigs were removed from the microbiome analysis due to experimental outliers, and the sample size was adjusted to n = 8 per group, as indicated where applicable. Colonic microbiome data were analyzed using the Kruskal-Wallis test. The individual pigs were considered the experimental unit for all analyses. All data are expressed as means with their pooled standard error of the means (SEM). Differences among groups were considered statistically significant when p < 0.05, and a trend at 0.05 ≤ p < 0.10. Correlations between abundant top 20 bacterial taxa at the genus level and plasma biochemical parameters, immunoglobulins, immuno-cytokines, and colonic SCFA concentrations were analyzed using Spearman’s rank correlation test.
3 Results
3.1 Effects of Chlorella vulgaris and lysozyme on the growth performance and diarrhea rate of weaned pigs
The effects of dietary C. vulgaris and lysozyme supplementation on the growth performance of weaned Xiangcun black pigs are presented in Table 2. The diarrhea rate was decreased (p < 0.001) in the CHV group compared with the CON and LYSO groups. Additionally, the diarrhea rate in the LYSO group was increased regarding the CON and CHV groups (p < 0.001). However, dietary C. vulgaris and lysozyme had no significant impacts (p > 0.10) on the growth performance parameters, including BW, ADG, ADFI, and F/G of weaned pigs.
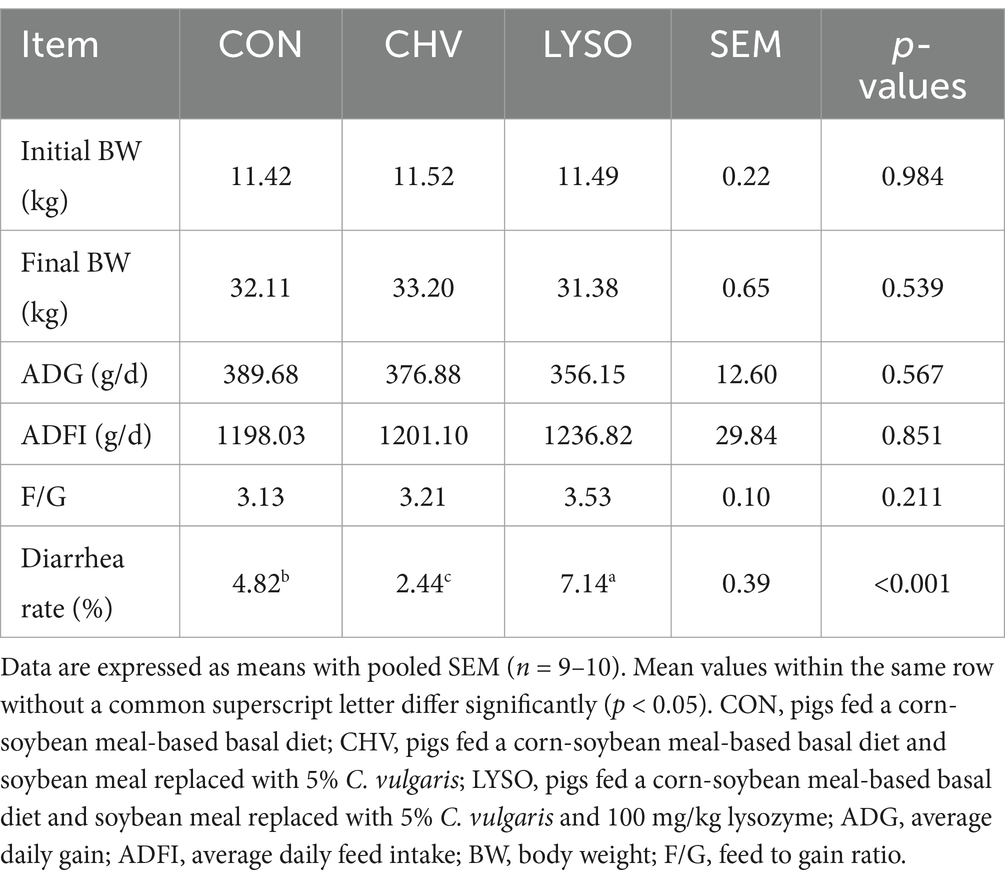
Table 2. Effects of C. vulgaris and lysozyme on the growth performance and diarrhea rate of weaned pigs.
3.2 Effects of Chlorella vulgaris and lysozyme on the plasma biochemical parameters of weaned pigs
The changes in plasma biochemical parameters of weaned Xiangcun black pigs are listed in Table 3. The plasma ALB (p = 0.052) concentration and ALP (p = 0.090) activity displayed increasing trends in the CHV group compared with the CON and LYSO groups. The plasma TC concentration was higher (p < 0.05) in the LYSO group compared with the CON and CHV groups. Moreover, plasma HDL-C concentration was higher (p < 0.05) in the CHV and LYSO groups compared with the CON group. No significant differences (p > 0.10) were observed in other plasma biochemical parameters among the three groups.
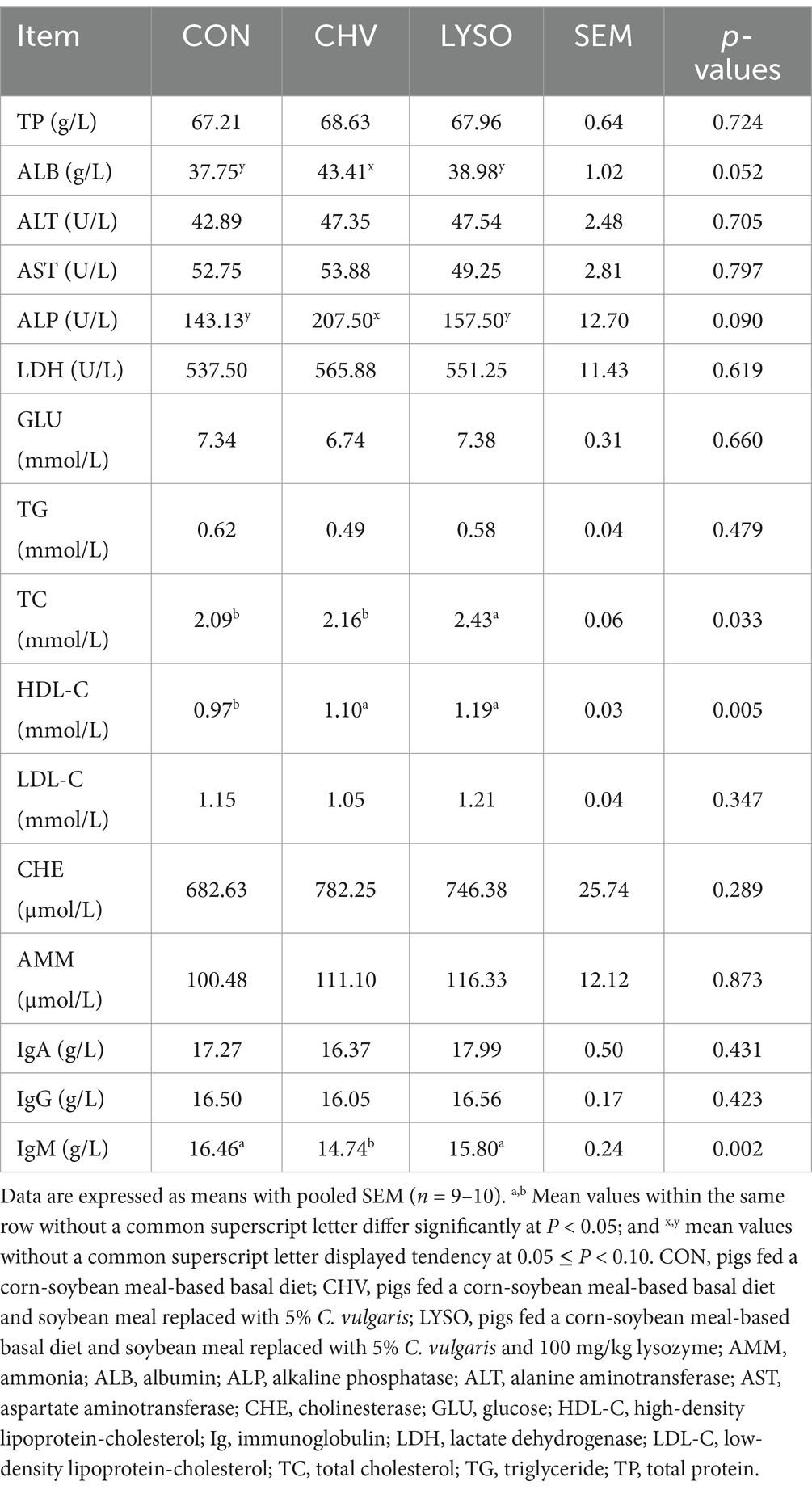
Table 3. Effects of C. vulgaris and lysozyme on the plasma biochemical parameters and immunoglobulins of weaned piglets.
3.3 Effects of Chlorella vulgaris and lysozyme on the immune function of weaned pigs
The level of plasma IgM was decreased (p < 0.05) in the CHV group compared with the CON and LYSO groups. However, supplementation of C. vulgaris and lysozyme did not affect (p > 0.10) the plasma levels of IgA and IgG in weaned pigs (Table 3).
As shown in Table 4, the IL-6 content was lower while IL-10 content was higher in the jejunum of the LYSO group (p < 0.05) compared with the CON and CHV groups. In the ileum, the IL-1β content was higher (p < 0.05) in the LYSO group compared with the CON group. Moreover, the TNF-α content was lower (p < 0.05) in the ileum of the CHV group compared with the CON and LYSO groups. However, supplementation of C. vulgaris and lysozyme did not affect (p > 0.10) the plasma immno-cytokines levels.
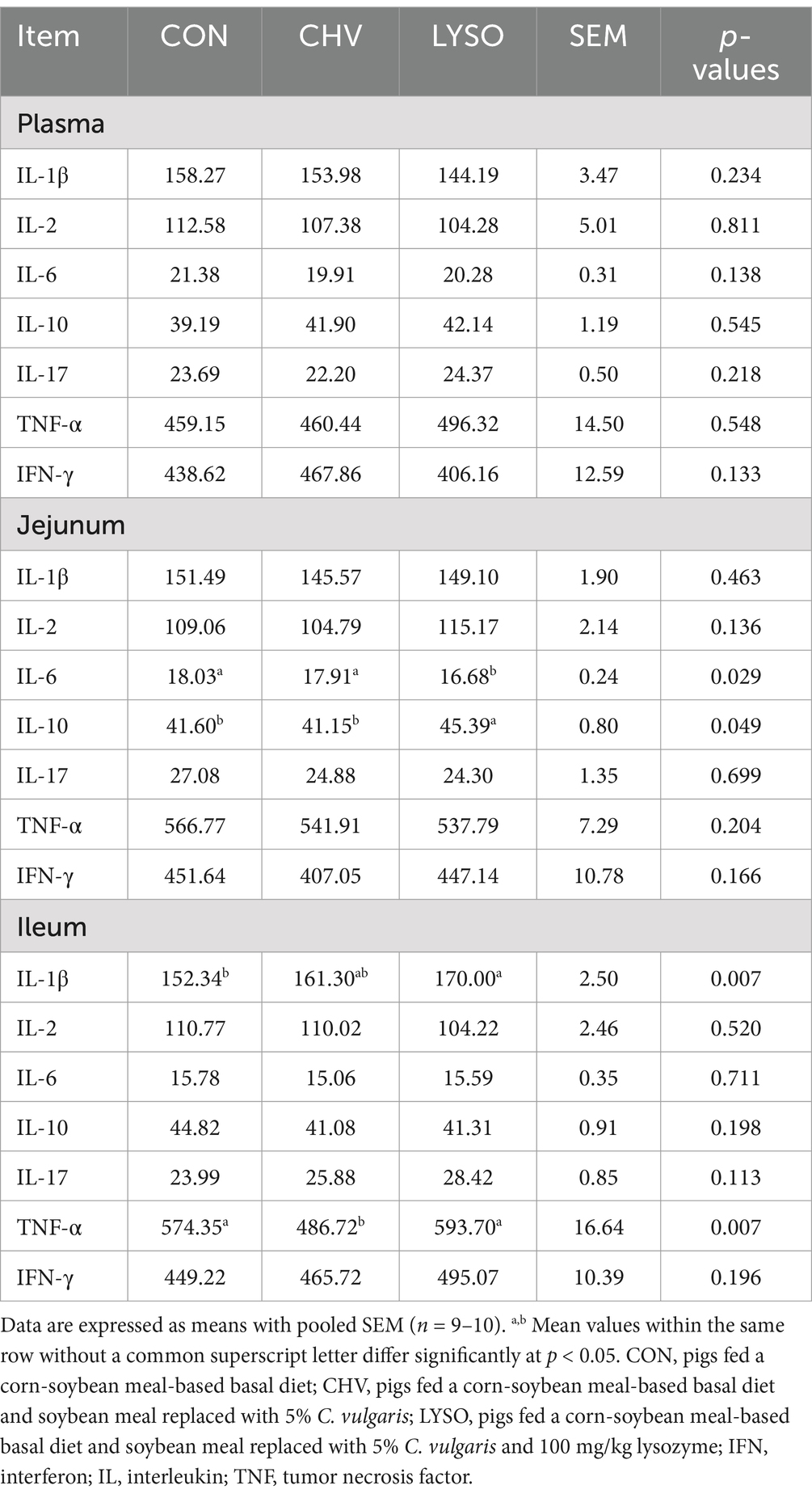
Table 4. Effects of C. vulgaris and lysozyme on plasma and intestinal immuno-cytokine levels of weaned piglets (pg/mL).
3.4 Effects of Chlorella vulgaris and lysozyme on the colonic microbiota diversity of weaned pigs
Colonic contents from 24 samples (n = 8) were assessed to evaluate the effects of C. vulgaris and lysozyme on the microbiota diversity of weaned pigs. The Venn analysis (Figure 1a) showed that there were 3,530, 3,444, and 3,040 unique OTUs in the colonic contents of the CON, CHV, and LYSO groups, respectively. Among them, there were 953 common OTUs among the three groups, of which 226, 261, and 412 common OTUs between the CON vs. CHV, CON vs. LYSO, and CHV and LYSO groups, respectively (Figure 1a).
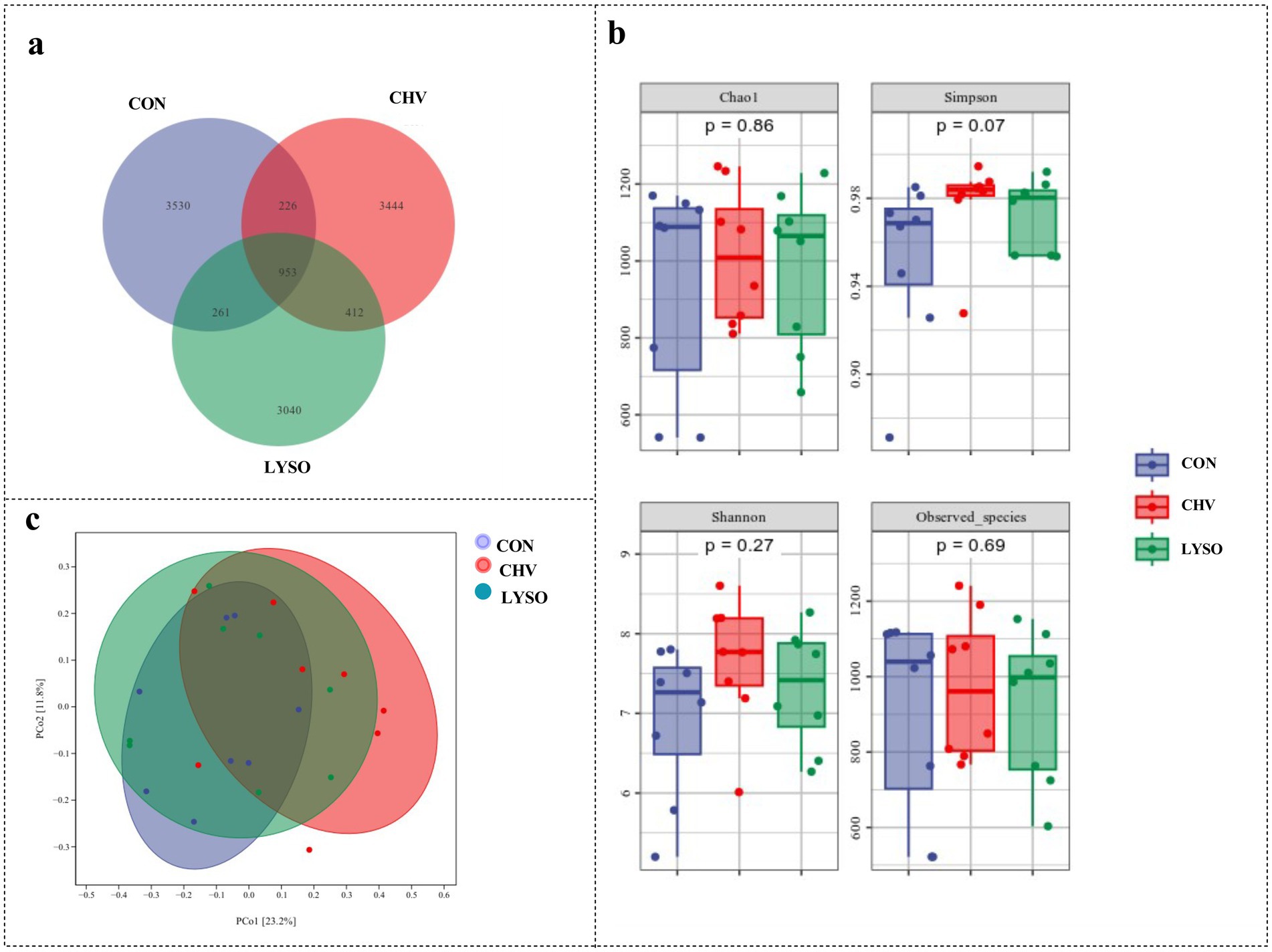
Figure 1. Effects of C. vulgaris and lysozyme on colonic microbiota diversity (n = 8). (a) The Venn, (b) alpha-diversity, and (c) principal coordinate analyses. Each dot symbol represents the gut microbiota of each piglet. CON, pigs fed a corn-soybean meal-based basal diet; CHV, pigs fed a corn-soybean meal-based basal diet and soybean meal replaced with 5% C. vulgaris; LYSO, pigs fed a corn-soybean meal-based basal diet and soybean meal replaced with 5% C. vulgaris and 100 mg/kg lysozyme.
The alpha-diversity analysis was performed to identify the diversity and structure of the colonic microbiota among different groups. The Simpson index displayed an increasing trend (p = 0.07) in the CHV group compared with the CON and LYSO groups. However, there were no significant changes (p > 0.10) in the Chao1, Shannon, and Observed_species among the three groups (Figure 1b). Furthermore, the beta-diversity analysis was performed to visualize the differences in the colonic microbiota community among the three groups. However, the PCoA showed no distinct separation among the three groups (Figure 1c).
3.5 Effects of Chlorella vulgaris and lysozyme on the colonic microbiota structure of weaned pigs
At the phylum level, Firmicutes, Bacteroidota, and Proteobacteria were the top three bacterial phyla (>90%) in the colon of weaned pigs in all groups, while other phyla were shown at very low relative abundance (Figure 2a). The top three phyla (including Firmicutes, Bacteroidota, and Proteobacteria) accounted for 81.16, 15.74, and 1.69% in the CON group, 71.16, 21.88, and 4.11% in the CHV group, and 75.90, 16.66, and 3.82% in the LYSO group, respectively (Figure 2a). The relative abundance of Firmicutes was decreased, while Desulfobacterota was increased in the CHV and LYSO groups compared with the CON group (p < 0.05). Moreover, the relative abundance of Actinobacteriota was higher (p < 0.05) in the LYSO group compared with the CON and CHV groups (Figure 2b).
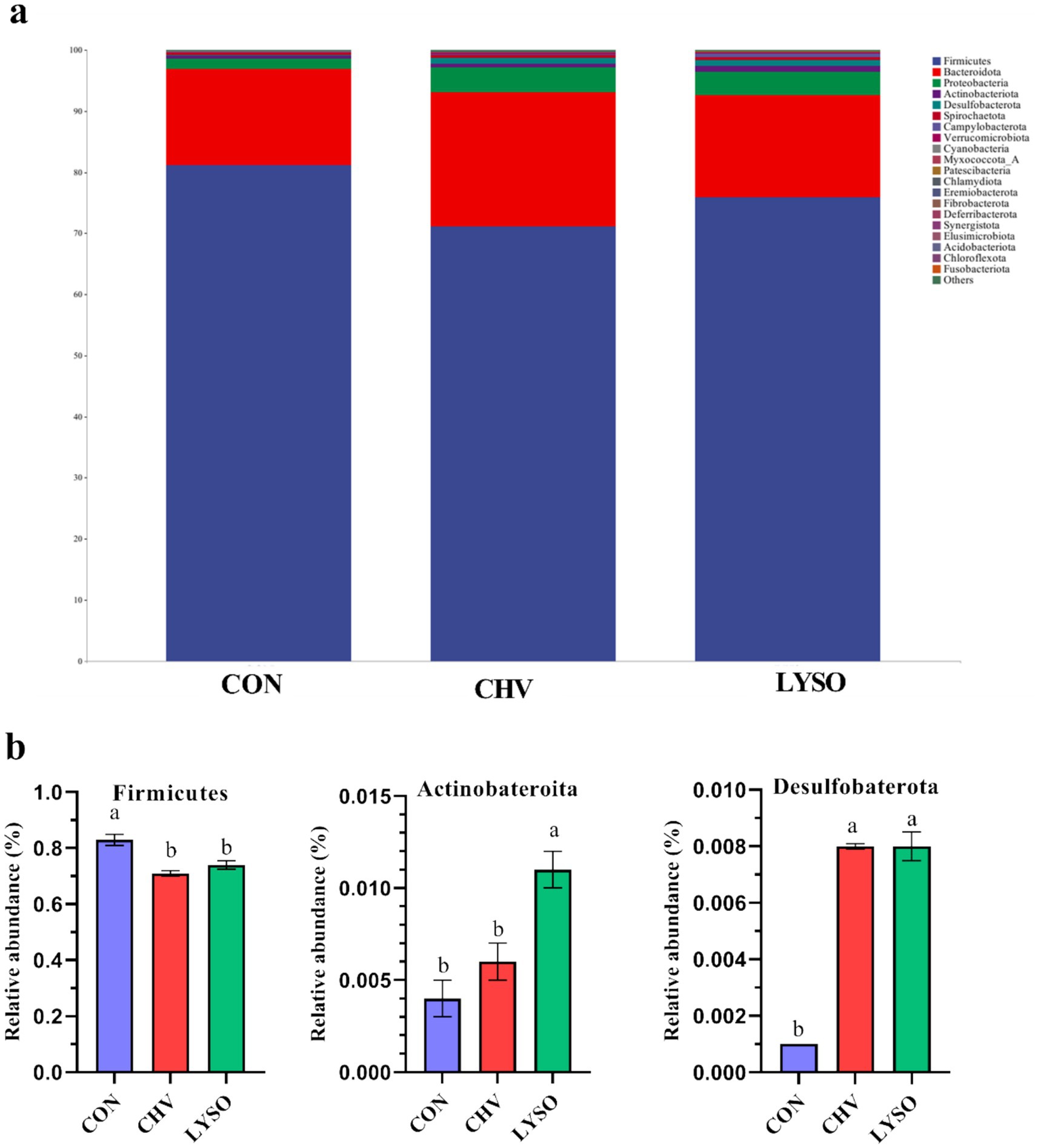
Figure 2. Effects of C. vulgaris and lysozyme on colonic microbiota composition (a) and taxonomic differences (b) at the phylum level (n = 8). Bars without a common letter indicate a significant difference (p < 0.05). CON, pigs fed a corn-soybean meal-based basal diet; CHV, pigs fed a corn-soybean meal-based basal diet and soybean meal replaced with 5% C. vulgaris; LYSO, pigs fed a corn-soybean meal-based basal diet and soybean meal replaced with 5% C. vulgaris and 100 mg/kg lysozyme.
Colonic bacterial community composition at the genus level is shown in Figure 3. Lactobacillus, Prevotella, and Megasphaera_A were the top three bacterial genera in the colon of all pigs. Lactobacillus (21.71%), Megasphaera_A (11.25%), Prevotella (9.10%), Limosilactobacillus (4.88%), Gemmiger_A (3.43%), Agathobacter (3.47%), Faecalibacterium (2.28%), and Phascolarctobacterium_A (1.74%) were the most abundant bacterial genera in the CON group. The most abundant bacterial genera in the CHV group were Lactobacillus (10.27%), Prevotella (7.75%), Sodaliphilus (4.87%), Limosilactobacillus (3.85%), Megasphaera_A (3.72%), Phascolarctobacterium_A (3.50%), and Faecalibacterium (1.45%). In addition, Lactobacillus (10.72%), Prevotella (7.75%), Megasphaera_A (5.99%), Limiosilactobacillus (3.90%), and Phascolarctobacterium_A (3.50%) were the most abundant bacterial genera in the LYSO group (Figure 3a). The relative abundance of Phascolarctobacterium_A was higher, whereas Megasphaera_A was lower in the CHV and LYSO groups compared with the CON group (p < 0.05). The relative abundance of Faecalibacterium was higher (p < 0.05) in the LYSO group compared with the CON and CHV groups, while Anaerovibrio was higher (p < 0.05) in the LYSO group compared with the CHV group (Figure 3b).
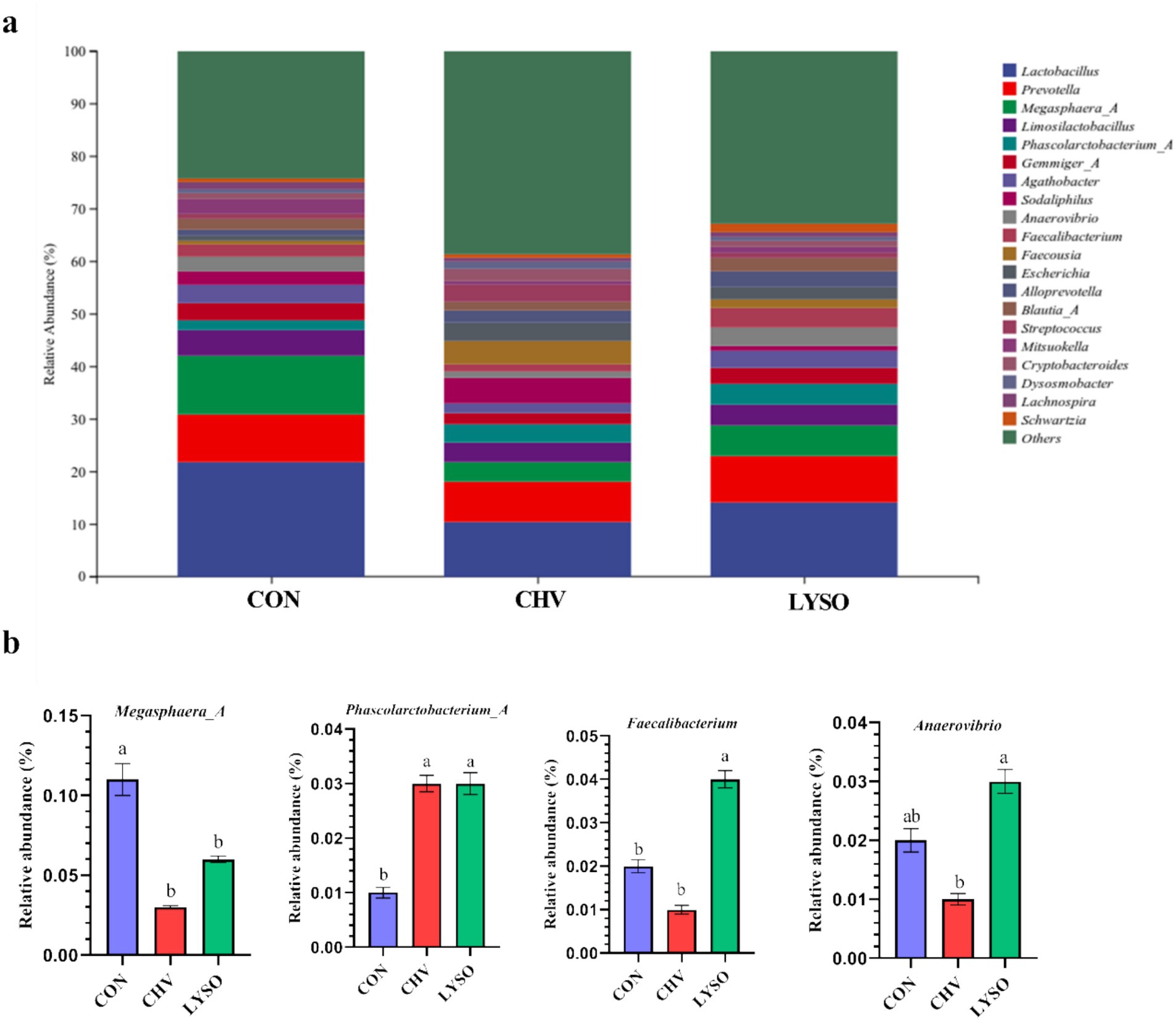
Figure 3. Effects of C. vulgaris and lysozyme on colonic microbiota composition (a) and taxonomic differences (b) at the genus level (n = 8). Bars without a common letter indicate a significant difference (p < 0.05). CON, pigs fed a corn-soybean meal-based basal diet; CHV, pigs fed a corn-soybean meal-based basal diet and soybean meal replaced with 5% C. vulgaris; LYSO, pigs fed a corn-soybean meal-based basal diet and soybean meal replaced with 5% C. vulgaris and 100 mg/kg lysozyme.
To identify the differences in colonic microbial function among different groups, LEfSe analysis (LDA threshold score ≥ 3.0) was performed at the phylum and genus levels (Figure 4a). There was a significant enrichment of Firmicutes in the CON group and Desulfobacterota in the LYSO group at the phylum level. At the genus level, Succinivibrio was enriched in the CON group, while Eubacterium, Faecousia, CAG_238, and Peptococcus were enriched in the CHV group. Additionally, Dusulfovibrio_R, Bariatricus, Facccalibacterium, Phascolarctobacterium_A, Anaerovibrio, Bulleidia, and Holdemanella were enriched in the LYSO group (Figure 4a). Moreover, Firmicutes_C in the CON group, Peptococcia in the CHV group, and Desulfobacterota_I in the LYSO group were the most dominant microbiota (Figure 4b).
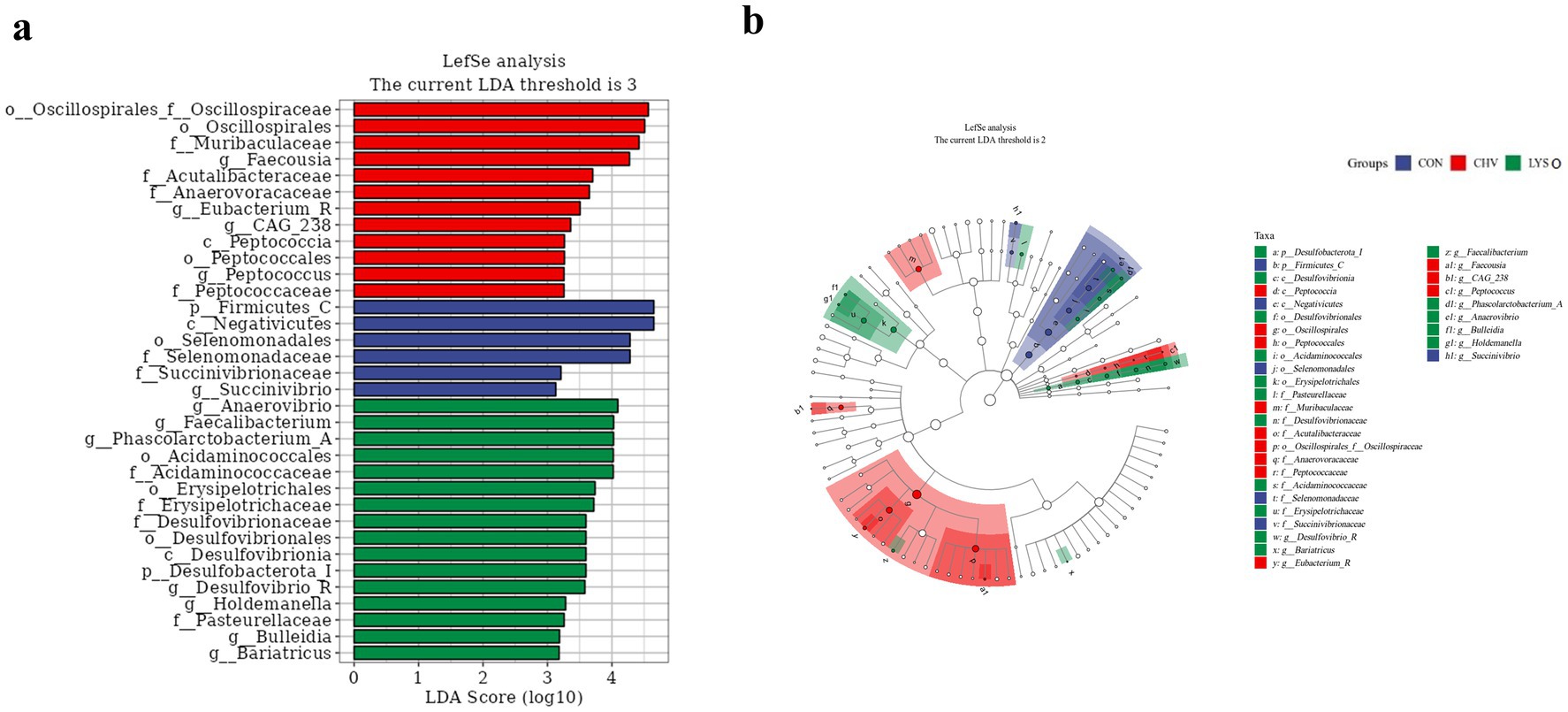
Figure 4. Linear discriminant analysis combined size effects (LEfSe) histogram (a) and species taxonomic branching diagram (b) of the colonic microbiota of weaned pigs. CON, pigs fed a corn-soybean meal-based basal diet; CHV, pigs fed a corn-soybean meal-based basal diet and soybean meal replaced with 5% C. vulgaris; LYSO, pigs fed a corn-soybean meal-based basal diet and soybean meal replaced with 5% C. vulgaris and 100 mg/kg lysozyme.
3.6 Effects of Chlorella vulgaris and lysozyme on the colonic short-chain fatty acid concentrations of weaned pigs
The effects of dietary C. vulgaris and lysozyme supplementation on the colonic SCFA concentrations of weaned pigs are presented in Table 5. Colonic acetate, propionate, butyrate, and valerate concentrations were lower (p < 0.001) in the CHV and LYSO groups compared with the CON group. However, acetate and butyrate concentrations were higher (p < 0.001) in the LYSO group compared with the CHV group. Moreover, colonic isobutyrate and isovalerate concentrations were higher (p < 0.05) in the CHV group compared with the CON and LYSO groups.
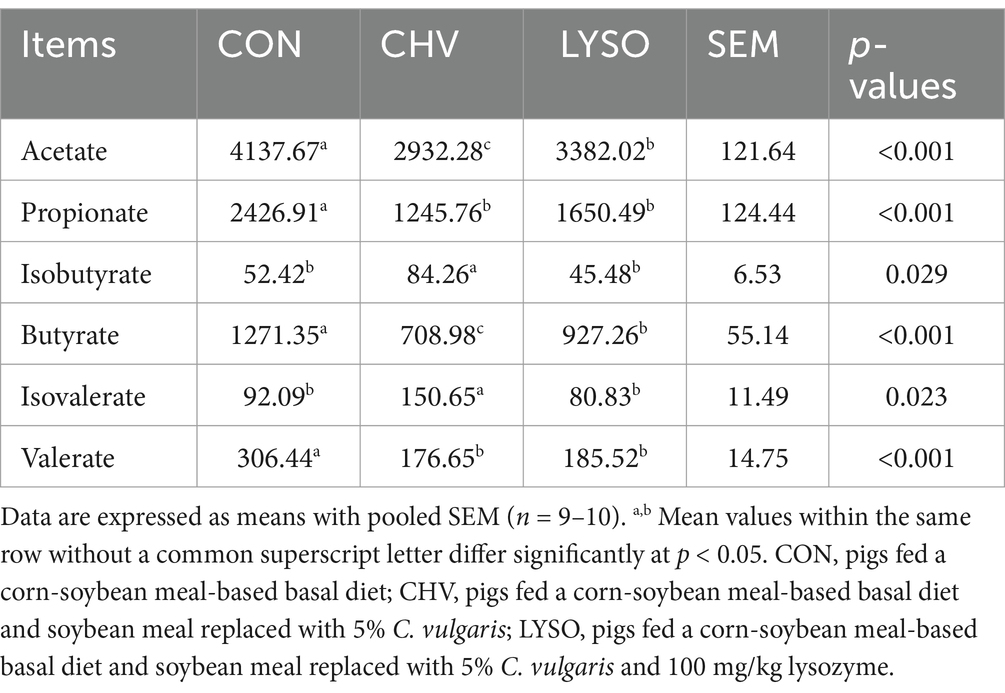
Table 5. Effects of C. vulgaris and lysozyme on the colonic short-chain fatty acid concentrations of weaned pigs (μmol/g).
3.7 Correlations between the levels of plasma biochemical parameters, immunoglobulins, immuno-cytokines, colonic SCFA, and microbiota abundances
Spearman’s correlation analysis was performed to reveal the associations between the levels of plasma biochemical parameters, immunoglobulins, immuno-cytokines, colonic SCFA, and the top 20 colonic bacterial taxa at the genus level (Figure 5). Plasma TP was negatively (p < 0.05) correlated with Lactobacillus, whereas plasma ALB was negatively correlated (p < 0.01) with Lactobacillus and Megasphaera_A and positively correlated (p < 0.05) with Faceousia, Streptococcus, and Cryptobacteroides. Plasma ALP was negatively correlated (p < 0.05) with Lactobacillus, Megasphaera_A, and Gemmiger_A, while positively correlated (p < 0.01) with Sodaliphilus and Cryptobacteroides. Plasma LDH was positively correlated with Sodaliphilus, while negatively correlated with Megasphaera_A and Agathobacter (p < 0.05). There were positive correlations between plasma HDL-C with Phascoloractobacterium_A and Alloprevotella and plasma LDL-C with Lachnospira and Schwartzia (p < 0.05). Plasma AMM was positively correlated with Dysosmobacter and negatively correlated with Limosilactobacillus (p < 0.05). Moreover, plasma IgM was positively correlated (p < 0.05) with Lactobacillus and Schwartzia and negatively correlated (p < 0.01) with Sodaliphilus (Figure 5a).
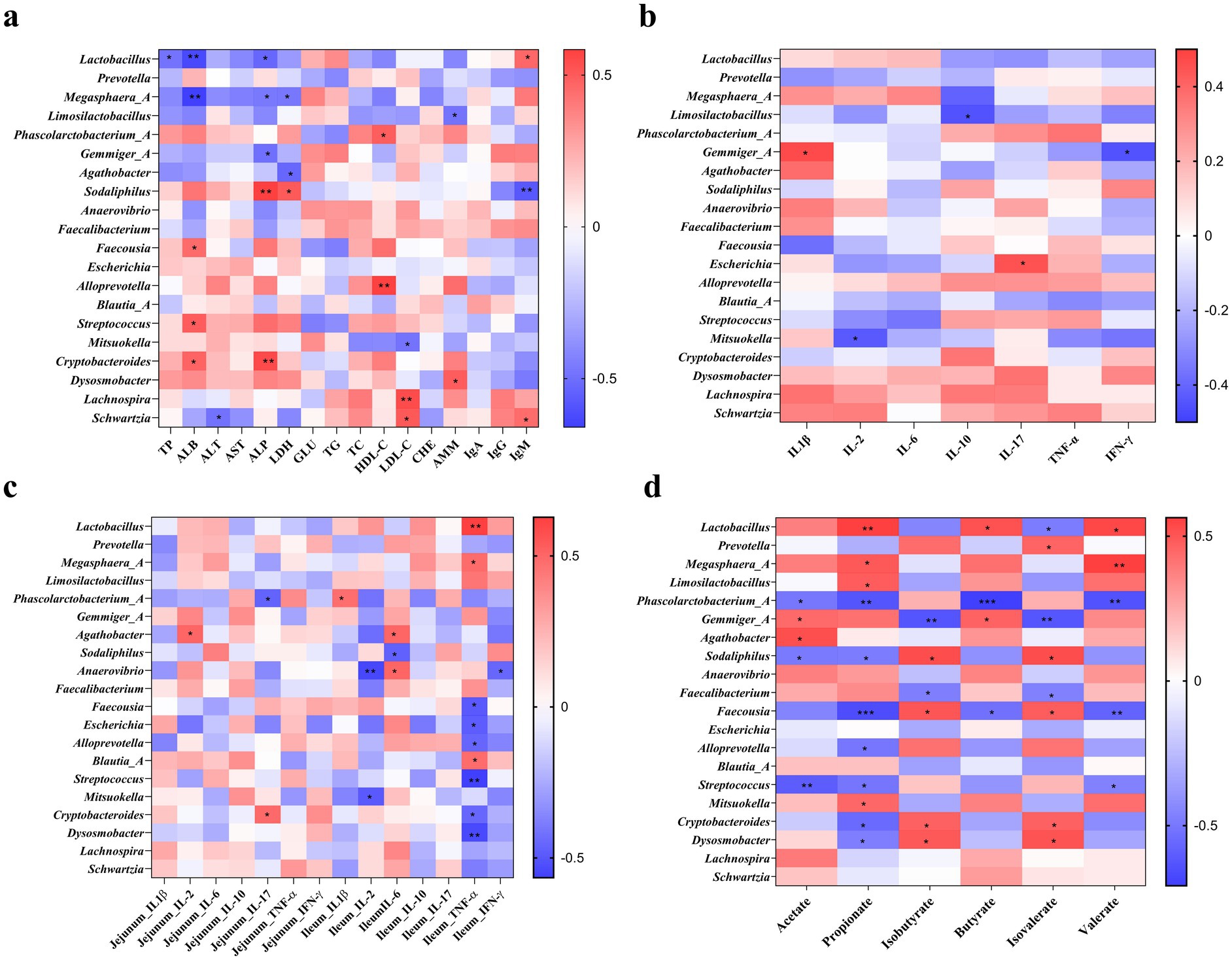
Figure 5. Spearman’s correlation analysis of different colonic microbiota and plasma biochemical parameters and immunoglobulins (a), plasma cytokines (b), intestinal cytokines (c), and colonic metabolites (d). * p < 0.05, ** p < 0.01, and *** p < 0.001.
The correlations between plasma and intestinal immuno-cytokines and colonic bacterial taxa at the genus level are shown in Figures 5b,c. The positive correlation included between plasma IL-1β with Germmiger_A and plasma IL-17 with Escherichia, while the negative correlation included between plasma IL-2 with Mitsuokella, plasma IL-10 with Limosilactobacillus, and plasma IFN-γ with Germmiger_A (Figure 5b). The IL-2 in the jejunum was positively correlated with Agathobacter, while IL-17 in the jejunum was positively correlated with Cryptobacteroides and negatively correlated with Phascoloractobacterium_A (p < 0.05). In addition, IL-1β in the ileum was positively correlated with Phascoloractobacterium_A, while IL-2 in the ileum was negatively correlated with Anaerovibrio and Mitsuokella (p < 0.05). The TNF-α in the ileum was positively correlated with Lactobacillus, Megasphaera_A, and Blautia_A, while it was negatively correlated with Faecousia, Escherichia, Alloprevotella, Streptococcus, Cryptobacteroides, and Dysosmobacter (p < 0.05). Furthermore, IFN-γ in the ileum was negatively correlated (p < 0.05) with Alloprevotella (Figure 5c).
The correlations between SCFA and colonic bacterial taxa at the genus level are shown in Figure 5d. The positive correlation included between acetate with Gemmiger_A and Agathobacter (p < 0.05), propionate with Lactobacillus (p < 0.01), Megasphaera_A (p < 0.05), Limosilactobacillus (p < 0.05),, and Mitsuokella (p < 0.05), butyrate with Lactobacillus and Gemmiger_A (p < 0.05), valerate with Lactobacillus (p < 0.05) and Megasphaera_A (p < 0.01), isobutyrate with Sodaliphilus, Faecousia, Cryptobacteroides, and Dysosmobacter (p < 0.05), and isovalerate with Prevotella, Sodaliphilus, Faecousia, Cryptobacteroides, and Dysosmobacter (p < 0.05). The negative correlation (p < 0.05) included between acetate with Phascoloractobacterium_A, Sodaliphilus, and Streptococcus, propionate (p < 0.05) with Phascoloractobacterium_A, Sodaliphilus, Faecousia (p < 0.001), Alloprevotella, Streptococcus, Cryptobacteroides, and Dysosmobacter, butyrate with Phascoloractobacterium_A (p < 0.001) and Faecousia (p < 0.05), valerate (p < 0.01) with Phascoloractobacterium_A, Faecousia, and Streptococcus (p < 0.05), isobutyrate with Germmiger_A (p < 0.01) and Faecalibacterium (p < 0.05), isovalerate with Lactobacillus (p < 0.05), Germmiger_A (p < 0.01), and Faecalibacterium (p < 0.05).
4 Discussion
Feed resources play indispensable roles in sustainable livestock production. Growing demands for protein from similar agricultural sources for both humans and animals are becoming more competitive due to the constantly increasing population. Thus, proteins from cost-effective and non-conventional sources have gained more attention for livestock production. Therefore, the present study investigated the effects of partial replacement of soybean meal with 5% C. vulgaris or 5% C. vulgaris with lysozyme on the growth performance, diarrhea rate, immune function, plasma biochemical parameters, and colonic microbiota and metabolites of weaned pigs. The findings showed that C. vulgaris supplementation reduced diarrhea rate and enhanced plasma biochemical parameters, whereas decreased IgM secretion and SCFA production. On the other hand, C. vulgaris with lysozyme supplementation showed an increased diarrhea rate, improved immune function by increasing immunoglobulin secretion and immuno-cytokines production, and increased colonic beneficial microbiome abundances and metabolites production in weaned Xiangcun black pigs compared with the CHV group.
Piglet diarrhea during the early stage of life is one of the major causes that can seriously affect the outcome of extensive animal production (15). Changes in diet structure during weaning are closely associated with piglet diarrhea and are accompanied by several health complications such as dehydration, growth retardation, and mortality of piglets (16). Consistent with a previous study (17), our results showed that soybean meal partially replaced by 5% C. vulgaris with or without lysozyme had no impact on the BW, ADG, ADFI, and F/G of weaned piglets. Moreover, several previous studies aimed to enhance growth performance and ameliorate post-weaning stress using C. vulgaris (≤1% or 385 mg/kg) as feed supplements and found no significant differences in ADFI, ADG, and feed conversion ratio in weaned piglets (18, 19). Interestingly, soybean meal partially replaced by C. vulgaris reduced diarrhea rate of piglets, while C. vulgaris supplementation with lysozyme increased the diarrhea rate of weaned piglets in the present study. The increased diarrhea rate in the LYSO group might be due to the fact that lysozyme may be involved in dissolving the cell wall of C. vulgaris to release additional nutrients (such as proteins) in the small intestine (7), causing a higher diarrhea rate when C. vulgaris is supplemented with lysozyme. However, further research is needed to understand the underlying mechanisms.
Plasma biochemical parameters have been increasingly used as physiological conditions as they represent valuable information about the host health condition of animals (20). It has been previously reported that certain microalgae species (such as Porphyra tenera and Laminaria digitata) enhance lipid metabolism through increasing lipoprotein profiles (such as lower LDL-C and higher HDL-C and lower cholesterol-enriched VLDL-C) in serum of a rat model, especially in individuals with hyperlipidemia (21). Microalgae are mainly composed of different bioactive compounds, such as polysaccharides, fibers, and lipids (i.e., omega-3 fatty acids), which are associated with lipid metabolism by lowering TG and LDL-C and increasing HDL-C levels (22, 23). Concerning the lipid metabolism biomarkers, different effects were observed by C. vulgaris and lysozyme supplementation. In the present study, soybean meal partially replaced by 5% C. vulgaris displayed an increasing trend in the plasma ALB and ALP levels compared with the pigs supplemented with the basal diet, indicating enhanced the immunity of piglets. Previous studies reported that higher level of ALB in serum can enhance the immunity and play protective role for immunoglobulins (24). Furthermore, ALP can contribute to the promotion of the differentiation of T lymphocytes and secretion of immunoglobulin to affect the humoral immune response (25). Additionally, although 5% C. vulgaris supplementation with lysozyme increased the TC level, which is in agreement with a previous study (26), as cholesterol could be partially obtained from the diet either by consumption of animal-derived products or from de-novo biosynthesis in the liver. Furthermore, the increased TC was counterbalanced by a significant increase in plasma HDL-C level in the CHV and LYSO groups, apparently leading to healthy cardiovascular functions (27). Moreover, reverse cholesterol transport can remove excess cholesterol from peripheral tissues and deliver it to the liver, while it is possibly redistributed to other tissues or removed from the key organ, as HDL-C is the main lipoprotein associated with this process. These findings indicate the beneficial effects of dietary C. vulgaris and lysozyme on fatty liver protection, as well as fatty liver-associated different metabolic disorders like obesity, diabetes, and hyperlipidemia (28).
Research evidence indicated that supplementing animal diets with microalgae has exhibited immune-enhancing functions (27). Pro- and anti-inflammatory cytokines dynamically regulate the immune function of weaned pigs. For instance, higher levels of pro-inflammatory cytokines, including IL-1β, IL-6, and TNF-α, lead to immune dysregulation, while anti-inflammatory cytokines (such as IL-10) can reduce inflammation (29). Previous studies have demonstrated that microalgae-based (such as Ulva lactua, A. platensis, and C. vulgaris) diets have significant anti-inflammatory effects in weaned pigs by regulating several immuno-cytokine profiles (19, 27). Consistent with these studies, our results showed that IL-6 level was lower, whereas IL-10 level was higher in jejunum of the LYSO group, indicating the potentiality of anti-inflammatory and immunosuppressive effects that regulated normal tissue homeostasis (30, 31). Additionally, the CHV group had a reduced the TNF-α level in the ileum of piglets. Moreover, TNF-α level in the ileum was also positively correlated with beneficial bacterial taxa, such as Lactobacillus and Blautia_A, and it was negatively correlated with Escherichia and Streptococcus. The immune structure of the jejunum, such as the higher density of immune cells and T lymphoid tissues, facilitates a more robust interaction between the lysozyme and immune cells, thus promoting the release and regulation of immuno-cytokines (32). On the other hand, the microbiome population differs between the jejunum and ileum, of which the jejunum has a comparatively lower bacterial load, while polysaccharides and bioactive compounds present in microalgae could enhance beneficial bacteria and further strengthen the immune response (33, 34). These might be the possible reasons that the anti-inflammatory and immunosuppressive repose of C. vulgaris with lysozyme was higher in the jejunum, while C. vulgaris without lysozyme exhibited a higher response in the ileum of piglets. These findings indicate that soybean meal parially replaced by 5% C. vulgaris without lysozyme may also partially contribute to the reduction of inflammation in weaned pigs.
Accordingly, the present study also evaluated the effects of C. vulgaris and lysozyme on the plasma immunoglobulins of weaned pigs. Although there were no significant differences in the plasma IgA and IgG concentrations, but plasma IgM was significantly lower in the CHV group compared with the other two groups. Moreover, plasma IgM was positively correlated with colonic Lactobacillus and Schwartzia abundances. These findings are consistent with a previous study by Matrins et al. (26), who reported that the IgM level could rise in the short-term and then begin to drop as the secretion of other immunoglobulins increases, thereby providing long-term protection for the immune organs. Overall, our findings suggest that soybean meal partially replaced by C. vulgaris exhibited lower immunity in piglets, while C. vulgaris supplemented with lysozyme recovered that effect. This indicates that C. vulgaris supplementation with lysozyme has significantly better immune effects than C. vulgaris alone.
The intestinal microbiota and microbiota-derived metabolites play crucial roles in various physiological and pathophysiological processes to regulate the gastrointestinal and immune function of the host. Microbial diversity indicators are commonly known as the representative of the gut ecosystem stability and the health of the host. The lower intestinal microbiota diversity indicates the dysbiosis of microbiota, suggesting that the host is affected by pathogen or pathogenic bacteria (35). In the present study, dietary C. vulgaris or C. vulgaris supplemented with lysozyme had no impact on the colonic microbiota diversity of weaned pigs. However, C. vulgaris supplementation without lysozyme showed an increasing trend in the Simpson index, indicating partial beneficial effects on maintaining the intestinal homeostasis of weaned pigs (36).
At the phylum level, our findings revealed that the most abundant bacterial phyla in all groups were Firmicutes, Bacteroidota, and Actinobacteria, which are in agreement with previous studies (17, 37). The taxonomic difference indicated that Firmicutes abundance was decreased, while Desulfobacterota abundance was increased in the CHV and LYSO groups compared with the CON group. Firmicutes are mainly associated with energy absorption from nutrient substances and SCFA production by the fermentative metabolism and degradation of carbon sources, proteins, and amino acids (38). Therefore, the decreased Firmicutes abundance in the CHV and LYSO groups affected the SCFA production, as evidenced by lower SFCA concentrations in the colon of weaned pigs in the present study. This decrease in both Firmicutes and SCFA (including acetate, butyrate, propionate, and valerate) indicates the presence of lower levels of fermentable carbohydrates in the colon of weaned pigs, which may be due to the recalcitrant wall of C. vulgaris that inhibited fermentation of carbohydrates in the digestive compartment. Previously, it has also been reported that diets consisting of insoluble dietary fiber affected the fermentation and the production and absorption of SCFA in the large intestine of pigs (39). In the present study, C. vulgaris supplementation with lysozyme increased Actinobacteriota abundance in the colon of weaned piglets. Several studies have found that Actinobacteriota is related to the production of antibiotics and immunomodulatory metabolites, which possess intestinal inflammation and immunity (40, 41), as evidenced by reduced IL-6 and elevated IL-10 levels in the jejunum of the LYSO group in the present study.
At the genus level, microbiome analyses showed that Megasphaera_A abundance was decreased and Phascolarctobacterium_A abundance was increased in the CHV and LYSO groups compared with the CON group. The higher abundance of Phascolarctobacterium leads to the promotion of the growth of symbiotic bacteria in the intestine to protect against intestinal diseases (42). A previous study indicated that Megasphaera is a potential bacterial genus that converts lactate to acetate, butyrate, propionate, and valerate and plays essential roles in gut health (43). This might be one of the possible reasons that the SCFA concentrations were lower in the CHV and LYSO groups.
The correlation analysis also revealed that colonic Megasphaera_A abundance was positively correlated with propionate and valerate concentrations. Furthermore, C. vulgaris with lysozyme supplementation increased the abundances of Faecalibacterium and Anaerovibrio in the colon. These findings are consistent with LEfSe results, which revealed that Faecalibacterium and Anaerovibrio were enriched in the LYSO group. Research evidence indicated that reduced Faecalibacterium abundance is associated with certain disorders such as celiac disease, chronic diarrhea, and inflammatory bowel diseases (44). Anaerovibrio are considered a group of lipid-degrading bacteria, which can inhibit the oxidation reactions during gastrointestinal digestion and protect against gastrointestinal inflammation (42). Taken together, although diets supplemented with C. vulgaris without lysozyme displayed causal effects of several pathogenic bacteria associated with SCFA production due to the presence of the recalcitrant wall of microalgae while C. vulgaris supplemented with lysozyme had beneficial effects in some contexts to enhance the plasma biochemical parameters and immune function of weaned pigs.
5 Conclusion
In this study, we found that partially replacing soybean meal with C. vulgaris reduces the diarrhea rate without affecting the growth performance of weaned piglets. Additionally, soybean meal replaced with C. vulgaris and lysozyme partially improves intestinal immunity by reducing pro-inflammatory and enhancing anti-inflammatory cytokines in the jejunum of weaned pigs. Moreover, C. vulgaris with lysozyme enhances several beneficial gut bacteria and metabolites related to lipid degradation and host immunity in comparison to C. vulgaris alone. Nevertheless, partially replacing soybean meal with C. vulgaris and lysozyme could be a promising non-conventional dietary supplemental approach for cost-effective swine production.
Data availability statement
The datasets presented in this study can be found in online repositories. The names of the repository/repositories and accessions number(s) can be found at the Science Data Bank under accession number https://doi.org/10.57760/sciencedb.16959.
Ethics statement
The animal study was approved by the present study was conducted in accordance with the ARRIVE guidelines and the Laboratory Animal Ethics Commission of the Chinese Academy of Sciences. The experimental protocol was reviewed and approved by the Animal Care and Use Committee of the Institute of Subtropical Agriculture, Chinese Academy of Sciences (Approval no. 20210035). The study was conducted in accordance with the local legislation and institutional requirements.
Author contributions
MA: Conceptualization, Data curation, Formal analysis, Funding acquisition, Investigation, Methodology, Project administration, Resources, Software, Validation, Visualization, Writing – original draft, Writing – review & editing. BL: Formal analysis, Investigation, Methodology, Writing – original draft. TY: Data curation, Formal analysis, Investigation, Methodology, Writing – review & editing. BQ: Data curation, Formal analysis, Investigation, Methodology, Software, Writing – review & editing. QZ: Data curation, Formal analysis, Methodology, Software, Writing – review & editing. YM: Data curation, Investigation, Software, Writing – review & editing. XK: Conceptualization, Resources, Supervision, Validation, Writing – review & editing.
Funding
The author(s) declare financial support was received for the research, authorship, and/or publication of this article. This study was supported by the Hunan Province Natural Science Fund (2022JJ30643), National Natural Science Fund of China (32350410424), and the Future Partner Special Fund of the Chinese Academy of Sciences (092GJHZ2022044FN).
Acknowledgments
The authors would like to thank the staff and postgraduate students from the Hunan Provincial Key Laboratory of Animal Nutritional Physiology and Metabolic Process for collecting samples and technicians from the CAS Key Laboratory of Agro-Ecological Processes in Subtropical Region for proving technical assistance.
Conflict of interest
The authors declare that the research was conducted in the absence of any commercial or financial relationships that could be construed as a potential conflict of interest.
The author(s) declared that they were an editorial board member of Frontiers, at the time of submission. This had no impact on the peer review process and the final decision.
Generative AI statement
The author(s) declare that no Gen AI was used in the creation of this manuscript.
Publisher’s note
All claims expressed in this article are solely those of the authors and do not necessarily represent those of their affiliated organizations, or those of the publisher, the editors and the reviewers. Any product that may be evaluated in this article, or claim that may be made by its manufacturer, is not guaranteed or endorsed by the publisher.
References
1. Zhang, X, Li, Z, Yang, H, Liu, D, Cai, G, Li, G, et al. Novel transgenic pigs with enhanced growth and reduced environmental impact. eLife. (2018) 7:e34286. doi: 10.7554/eLife.34286
2. Costa, M, Coelho, D, Alfaia, C, Pestana, J, Lopes, PA, and Prates, JAM. Microalgae application in feeds for monogastrics. In: E Jacob-Lopes, MI Queiroz, MM Maroneze, and LQ Zepka, editors. Handbook of food and feed from microalgae : Academic Press, Cambridge, MA, United States. (2023). 411–20.
3. Valente, LMP, Cabrita, ARJ, Maia, MRG, Valente, IM, Engrola, S, Fonseca, AJM, et al. Microalgae as feed ingredients for livestock production and aquaculture. In: CM Galanakis, editor. Microalgae : Academic Press, Cambridge, MA, United States. (2021). 239–312.
4. Dineshbabu, G, Goswami, G, Kumar, R, Sinha, A, and Das, D. Microalgae–nutritious, sustainable aqua- and animal feed source. J Funct Foods. (2019) 62:103545. doi: 10.1016/j.jff.2019.103545
5. Spínola, MP, Costa, MM, and Prates, JAM. Enhancing digestibility of Chlorella vulgaris biomass in monogastric diets: Strategies and insights. Animals. (2023) 13:1017. doi: 10.3390/ani13061017
6. Martins, CF, Ribeiro, DM, Costa, M, Coelho, D, Alfaia, CM, Lordelo, M, et al. Using microalgae as a sustainable feed resource to enhance quality and nutritional value of pork and poultry meat. Food Secur. (2021) 10:2933. doi: 10.3390/foods10122933
7. Coelho, D, Lopes, PA, Cardoso, V, Ponte, P, Brás, J, Madeira, MS, et al. Novel combination of feed enzymes to improve the degradation of Chlorella vulgaris recalcitrant cell wall. Sci Rep. (2019) 9:5382. doi: 10.1038/s41598-019-41775-0
8. Martins, CF, Pestana, J, Ribeiro, D, Madeira, MSMDS, Alfaia, CMRPM, Lopes, PAAB, et al. Effect of dietary inclusion of Spirulina on production performance, nutrient digestibility and meat quality traits in post-weaning piglets. J Anim Physiol Anim Nutr. (2021) 105:247–59. doi: 10.1111/jpn.13470
9. Martins, CF, Pestana, JM, Alfaia, CM, Costa, M, Ribeiro, DM, Coelho, D, et al. Effects of Chlorella vulgaris as a feed ingredient on the quality and nutritional value of weaned piglets’ meat. Food Secur. (2021) 10:1155. doi: 10.3390/foods10061155
10. Xiong, X, Zhou, J, Liu, H, Tang, Y, Tan, B, and Yin, Y. Dietary lysozyme supplementation contributes to enhanced intestinal functions and gut microflora of piglets. Food Funct. (2019) 10:1696–706. doi: 10.1039/c8fo02335b
11. Hart, GK, and Dobb, GJ. Effect of a fecal bulking agent on diarrhea during enteral feeding in the critically ill. J Parenter Enteral Nutr. (1988) 12:465–8. doi: 10.1177/0148607188012005465
12. Liu, Y, Azad, MAK, Zhao, X, Zhu, Q, and Kong, X. Dietary crude protein levels alter diarrhea incidence, immunity, and intestinal barrier function of Huanjiang mini-pigs during different growth stages. Front Immunol. (2022) 13:908753. doi: 10.3389/fimmu.2022.908753
13. Zhu, Q, Song, M, Azad, MAK, Cheng, Y, Liu, Y, Liu, Y, et al. Probiotics or synbiotics addition to sows’ diets alters colonic microbiome composition and metabolome profiles of offspring pigs. Front Microbiol. (2022) 13:934890. doi: 10.3389/fmicb.2022.934890
14. Ding, H, Zhao, X, Azad, MAK, Ma, C, Gao, Q, He, J, et al. Dietary supplementation with Bacillus subtilis and xylo-oligosaccharides improves growth performance and intestinal morphology and alters intestinal microbiota and metabolites in weaned piglets. Food Funct. (2021) 12:5837–49. doi: 10.1039/d1fo00208b
15. Zhou, X, Liang, J, Xiong, X, and Yin, Y. Amino acids in piglet diarrhea: Effects, mechanisms and insights. Anim Nutr. (2024) 16:267–74. doi: 10.1016/j.aninu.2023.07.009
16. Tang, Q, Lan, T, Zhou, C, Gao, J, Wu, L, Wei, H, et al. Nutrition strategies to control post-weaning diarrhea of piglets: From the perspective of feeds. Anim Nutr. (2024) 17:297–311. doi: 10.1016/j.aninu.2024.03.006
17. Martins, CF, Trevisi, P, Coelho, DF, Correa, F, Ribeiro, DM, Alfaia, CM, et al. Influence of Chlorella vulgaris on growth, digestibility and gut morphology and microbiota of weaned piglet. Sci Rep. (2022) 12:6012. doi: 10.1038/s41598-022-10059-5
18. Furbeyre, H, van Milgen, J, Mener, T, Gloaguen, M, and Labussière, E. Effects of dietary supplementation with freshwater microalgae on growth performance, nutrient digestibility and gut health in weaned piglets. Animal. (2017) 11:183–92. doi: 10.1017/S1751731116001543
19. Furbeyre, H, van Milgen, J, Mener, T, Gloaguen, M, and Labussière, E. Effects of oral supplementation with Spirulina and Chlorella on growth and digestive health in piglets around weaning. Animal. (2018) 12:2264–73. doi: 10.1017/S1751731118000125
20. Ding, S, Azad, MAK, Fang, J, Zhou, X, Xu, K, Yin, Y, et al. Impact of sulfur-containing amino acids on the plasma metabolomics and intestinal microflora of the sow in late pregnancy. Food Funct. (2019) 10:5910–21. doi: 10.1039/c9fo01456j
21. Bocanegra, A, Bastida, S, Benedí, J, Nus, M, Sánchez-Montero, JM, and Sánchez-Muniz, FJ. Effect of seaweed and cholesterol-enriched diets on postprandial lipoproteinaemia in rats. Br J Nutr. (2009) 102:1728–39. doi: 10.1017/S000711450999105X
22. Gabbia, D, Roverso, M, Zanotto, I, Colognesi, M, Sayaf, K, Sarcognato, S, et al. A nutraceutical formulation containing brown algae reduces hepatic lipid accumulation by modulating lipid metabolism and inflammation in experimental models of NAFLD and NASH. Mar Drugs. (2022) 20:572. doi: 10.3390/md20090572
23. Czech, A, Woś, K, Muszyński, S, and Tomaszewska, E. Nutritional and antioxidative benefits of dietary macroalgae supplementation in weaned piglets. Animals. (2024) 14:549. doi: 10.3390/ani14040549
24. Zhao, K, Yin, H, Yan, H, Tang, W, Diao, H, Wang, Q, et al. Dietary supplementation of Lactobacillus johnsonii Rs-7 improved antioxidant and immune function of weaned piglets. Animals. (2023) 13:1595. doi: 10.3390/ani13101595
25. Hou, G, Peng, W, Wei, L, Li, R, Huang, X, and Yin, Y. Probiotics and Achyranthes bidentata polysaccharides improve growth performance via promoting intestinal nutrient utilization and enhancing immune function of weaned pigs. Animals. (2021) 11:2617. doi: 10.3390/ani11092617
26. Martins, CF, Lopes, PA, Palma, M, Pinto, RMA, Costa, M, Alfaia, CM, et al. Impact of dietary Chlorella vulgaris and feed enzymes on health status, immune response and liver metabolites in weaned piglets. Sci Rep. (2022) 12:16816. doi: 10.1038/s41598-022-21238-9
27. Ribeiro, DM, Lopes, PA, Pinto, RMA, Pestana, JM, Costa, MM, Alfaia, CM, et al. Dietary Ulva lactuca and CAZyme supplementation improve serum biochemical profile and hepatic composition of weaned piglets. Sci Rep. (2023) 13:8784. doi: 10.1038/s41598-023-36008-4
28. Yki-Järvinen, H, Luukkonen, PK, Hodson, L, and Moore, JB. Dietary carbohydrates and fats in nonalcoholic fatty liver disease. Nat Rev Gastroenterol Hepatol. (2021) 18:770–86. doi: 10.1038/s41575-021-00472-y
29. Azad, MAK, Wang, H, Yang, H, Tan, B, Zhou, S, and Guan, G. Effects of dietary carboxymethyl pachyman on oxidative stress and inflammation in weaned piglets challenged with diquat. Anim Feed Sci Technol. (2021) 276:114922. doi: 10.1016/j.anifeedsci.2021.114922
30. York, AG, Skadow, MH, Oh, J, Qu, R, Zhou, QD, Hsieh, WY, et al. IL-10 constrains sphingolipid metabolism to limit inflammation. Nature. (2024) 627:628–35. doi: 10.1038/s41586-024-07098-5
31. Grebenciucova, E, and Van Haerents, S. Interleukin 6: At the interface of human health and disease. Front Immunol. (2023) 14:1255533. doi: 10.3389/fimmu.2023.1255533
32. Mahapatro, M, Erkert, L, and Becker, C. Cytokine-mediated crosstalk between immune cells and epithelial cells in the gut. Cells. (2021) 10:111. doi: 10.3390/cells10010111
33. Isaacson, R, and Kim, HB. The intestinal microbiome of the pig. Anim Health Res Rev. (2012) 13:100–9. doi: 10.1017/S1466252312000084
34. Kim, SW, and Duarte, ME. Understanding intestinal health in nursery pigs and the relevant nutritional strategies. Anim Biosci. (2021) 34:338–44. doi: 10.5713/ab.21.0010
35. Sekirov, I, Russell, SL, Antunes, LCM, and Finlay, BB. Gut microbiota in health and disease. Physiol Rev. (2010) 90:859–904. doi: 10.1152/physrev.00045.2009
36. Zhang, YJ, Bousvaros, A, Docktor, M, Kaplan, AL, Rufo, PA, Leier, M, et al. Higher alpha diversity and Lactobacillus blooms are associated with better engraftment after fecal microbiota transplant in inflammatory bowel disease. Sci Rep. (2024) 14:18188. doi: 10.1038/s41598-024-68619-w
37. Rose, EC, Blikslager, AT, and Ziegler, AL. Porcine models of the intestinal microbiota: The translational key to understanding how gut commensals contribute to gastrointestinal disease. Front Vet Sci. (2022) 9:834598. doi: 10.3389/fvets.2022.834598
38. Lu, J, Zhang, X, Liu, Y, Cao, H, Han, Q, Xie, B, et al. Effect of fermented corn-soybean meal on serum immunity, the expression of genes related to gut immunity, gut microbiota, and bacterial metabolites in grower-finisher pigs. Front Microbiol. (2019) 10:2620. doi: 10.3389/fmicb.2019.02620
39. Wang, J, Qin, C, He, T, Qiu, K, Sun, W, Zhang, X, et al. Alfalfa-containing diets alter luminal microbiota structure and short chain fatty acid sensing in the caecal mucosa of pigs. J Anim Sci Biotechnol. (2018) 9:11. doi: 10.1186/s40104-017-0216-y
40. Matsui, T, Tanaka, J, Namihira, T, and Shinzato, N. Antibiotics production by an actinomycete isolated from the termite gut. J Basic Microbiol. (2012) 52:731–5. doi: 10.1002/jobm.201100500
41. Cui, C, Wei, Y, Wang, Y, Ma, W, Zheng, X, Wang, J, et al. Dietary supplementation of benzoic acid and essential oils combination enhances intestinal resilience against LPS stimulation in weaned piglets. J Anim Sci Biotechnol. (2024) 15:4. doi: 10.1186/s40104-023-00958-6
42. Sun, T, Miao, H, Zhang, C, Wang, Y, Liu, S, Jiao, P, et al. Effect of dietary Bacillus coagulans on the performance and intestinal microbiota of weaned piglets. Animal. (2022) 16:100561. doi: 10.1016/j.animal.2022.100561
43. Liang, J, Kou, S, Chen, C, Raza, SHA, Wang, S, Ma, X, et al. Effects of Clostridium butyricum on growth performance, metabonomics and intestinal microbial differences of weaned piglets. BMC Microbiol. (2021) 21:85. doi: 10.1186/s12866-021-02143-z
44. Lopez-Siles, M, Enrich-Capó, N, Aldeguer, X, Sabat-Mir, M, Duncan, SH, Garcia-Gil, LJ, et al. Alterations in the abundance and co-occurrence of Akkermansia muciniphila and Faecalibacterium prausnitzii in the colonic mucosa of inflammatory bowel disease subjects. Front Cellul Infect Microbiol. (2018) 8:281. doi: 10.3389/fcimb.2018.00281
Keywords: alternative feed, immunity, microalgae, gut health, Xiangcun black pigs
Citation: Azad MAK, Li B, Ye T, Qin B, Zhu Q, Martinez Y and Kong X (2025) Effects of partial replacement of soybean meal with Chlorella vulgaris and lysozyme on diarrheal incidence, plasma biochemical parameters, and immunity of weaned piglets. Front. Vet. Sci. 11:1505540. doi: 10.3389/fvets.2024.1505540
Edited by:
Ismoyowati Ismoyowati, Jenderal Soedirman University, IndonesiaReviewed by:
Young Dal Jang, University of Georgia, United StatesDongsheng Che, Jilin Agriculture University, China
Copyright © 2025 Azad, Li, Ye, Qin, Zhu, Martinez and Kong. This is an open-access article distributed under the terms of the Creative Commons Attribution License (CC BY). The use, distribution or reproduction in other forums is permitted, provided the original author(s) and the copyright owner(s) are credited and that the original publication in this journal is cited, in accordance with accepted academic practice. No use, distribution or reproduction is permitted which does not comply with these terms.
*Correspondence: Xiangfeng Kong, bm5reGZAaXNhLmFjLmNu
†These authors have contributed equally to this work