- 1Key Laboratory of Animal Disease and Human Health of Sichuan Province, College of Veterinary Medicine, Sichuan Agricultural University, Chengdu, China
- 2College of Landscape Architecture, Sichuan Agricultural University, Chengdu, China
Background: Probiotics, which are beneficial to the host, have been shown to benefit the health of cats. Lactic acid bacteria (LAB) are commonly used probiotics, but most strains used for cats are not derived from cats, leading to reduced efficacy and poor adaptation to cats. The objective was to identify LAB with promising probiotic potential specific to cats.
Method: LABs were isolated from fecal samples of 20 healthy cats. Gram staining and the survival rate in the simulated gastrointestinal tract were used for preliminary screening. Candidate strains were identified by 16S rDNA sequencing, and further evaluated for adhesion ability, growth characteristics, antibacterial activity, antioxidant capacity, and safety.
Results: 24 Gram-positive isolates were identified, with 10 (F1-F10) showing robust viability in the simulated gastroenteric fluid. These 10 strains exhibited excellent adhesion to Caco-2 cells and strong auto-agglutination properties. They also possessed the capacity to antagonize and aggregate pathogens (Staphylococcus aureus ATCC 25923, Salmonella Braenderup H9812, Escherichia coli ATCC 25922, and Pseudomonas aeruginosa PAO1), Moreover, all strains demonstrated tolerance to H2O2 concentrations ranging from 0.5–2 mmol/L and the ability to scavenge 1, 1-diphenyl-2-picrylhydrazyl (DPPH) free radicals, indicating a certain level of antioxidant activity. Safety tests showed no hemolytic activity, and all but F6 were highly sensitive to antibiotics, with over 62.5% sensitivity to 16 antibiotics. Remarkably, F4 (Lactobacillus reuteri) and F10 (Lactobacillus brevis) exhibited exceptional viability in the simulated gastrointestinal tract, coupled with robust growth potential, enhanced adhesion efficiency, significant antibacterial and antioxidant properties.
Conclusion: Our findings revealed that F4 (Lactobacillus reuteri) and F10 (Lactobacillus brevis) hold promising potential as probiotics. This research lays a solid scientific foundation for the selection and application of probiotics tailored specifically for cats.
Introduction
As a companion animal, the health of cats has attracted more and more attention. Research has indicated a strong correlation between intestinal health and the overall well-being of the host (48, 1). Cats, in particular, are prone to experiencing diarrhea. This condition can arise from a variety of causes. Furthermore, they frequently struggling with intestinal issues that can pose significant risks to their life and health (2, 3). Preserving optimal intestinal health could empower them to resist intestinal damage caused by adverse external environments and pathogenic intestinal microbes (4). Consequently, it is crucial to identify effective strategies to safeguard the intestinal homeostasis of domestic cats.
Probiotics are defined as living microorganisms that are beneficial to the host when properly administered (5). Lactic acid bacteria (LAB), is the most commonly used probiotic, proven effective in maintaining cats’ intestinal health (6, 7). For instance, the research conducted by Kerek et al. highlights probiotics as a promising approach to decrease the reliance on antibiotics in companion animals (8); a multi-strain probiotic consisting of Saccharomyces boulardii and Pediococcus acidilactici promotes intestinal health in cats (9); Lappin’s study on cats infected with feline herpesvirus type 1 found that Enterococcus faecium SF68 preserved intestinal microbiome diversity and reduced chronic infection rates (10); Bybee showed that Enterococcus faecium SF68 decreased diarrhea incidence in cats (11), ande Additives verified L. reuteri’s benefits for cats’ intestinal health (12). Overall, LAB is beneficial for cats’ health.
Probiotics isolated from the same species of host can more effectively exhibit their probiotic characteristics (13). However, it is regrettable that most of LAB currently used in cats is not cat-specific, leading to issues such as reduced efficacy and poor adaptation to the host. To date, there is a significant gap in research on LAB derived from cats both domestically and internationally, which hinders the provision of scientific guidance for the clinical application of cat-specific probiotics. The objective of this study is to isolate LAB from the feces of healthy cats and to assess their probiotic properties. The assessment includes resistance to artificial gastric and intestinal fluids, adhesion capabilities, growth characteristics, antibacterial activity, drug resistance, antioxidant capacity, and safety. The aim is to identify LAB with promising probiotic potential specific to cats, offering a valuable reference for the application of probiotics for cats.
Materials and methods
Samples collection and isolation of LAB
Fecal samples from 20 healthy adult cats were collected. These cats were from three cattery houses in Chengdu that had not used antibiotics or probiotics for 2 months. The samples were collected from the rectum with sterile swabs and transferred into sterile 1.5 mL centrifuge tubes. It is then packed in ice and transported to the lab. The samples were diluted with sterile PBS and then placed in de Man, Rogosa, and Sharpe (MRS) Liquid medium (Hopebio, Qingdao, China) at 10% and incubated anaerobically at 37°C for 24 h. Then the culture medium was diluted by 10 times gradient, and 100 uL culture medium with different gradients was inoculated on MRS Solid medium. After culture for 24–48 h, single colonies were selected and re-plotted on the MRS Plate. Subsequently, these single colonies were screened and purified by continuous passage 3 times.
Preliminary screening
Gram stain
The isolated and purified single colonies were enriched with MRS broth. Then, subjected to Gram staining microscopy to observe the morphology and staining of the isolated strains.
Tolerance for the simulated GIT condition
Utilizing the method described by Zhang, the strains identified as Gram-positive through staining were evaluated for their tolerance to simulated gastrointestinal tract (GIT) conditions (14). 0.3 g of pepsin was dissolved in 100 mL of 0.9% sterile normal saline, with pH adjusted to 3.0 using 1 M HCL to simulate gastric fluid. Simulated intestinal fluid was prepared by dissolving 0.2 g trypsin and 0.3 g cow bile salt in 100 mL of 0.9% sterile normal saline, pH adjusted to 8.0 with 1 M NaOH. Both fluids were filtered to remove bacteria. The strains were inoculated into 10 mL MRS Broth at 1% volume and cultured for 14 h. After centrifugation (25°C, 8,000 × g, 15 min), the pellets were re-suspended in sterile saline and centrifuged under the same conditions to wash 3 times. The cells were then re-suspended in 10 mL of the prepared simulated gastric juice and incubated at 37°C for 3 h anaerobically. Following this, Centrifugal collection the pellets were transferred to 10 mL of simulated intestinal fluid and cultured at 37°C for an additional 4 h (7 h) anaerobically. The number of viable colonies at 0 h, 3 h, and 7 h was determined using the plate counting method on MRS agar. The survival rate (%) was calculated as (A0/A1) × 100%. A0 denotes the number of viable bacteria at 0 h (CFU/mL) and A1 stands for the number of viable bacteria at 3 h/7 h (CFU/mL).
Identification of 16S rDNA
DNA extraction kit (Beijing Tiangen Biotechnology Co., LTD.) of bacteria was used to extract the DNA of the strains screened by the test of resistance to artificial gastric and intestinal fluid. The 16S rRNA gene of the strain was amplified by PCR using universal primers (F: 5’-AGAGTTTGATCCTGGCTCAG-3′; R: 5’-GGTTACCTTGTTACGACTT-3′). PCR products were then separated via 1.5% agarose gel electrophoresis, and the positive products were sent to Sangon Biotech Co. Ltd. (Shanghai, China) for sequencing. The obtained 16S rDNA sequences were compared with the Genbank database. The sequences with the highest homology were selected to construct a phylogenetic tree based on the 16S rDNA sequences by using MEGA software (version 7.0). The maximum likelihood method was employed for clustering, with 1,000 bootstrap replicates used to estimate the robustness of individual branches.
Assessment of the growth capacity
The growth performance of the 10 isolated strains was assessed following the methodology described in a previous study (15). 10 μL (1%) of each LAB culture was inoculated into 10 mL of fresh MRS broth and incubated at 37°C for 48 h. The absorbance at 600 nm was recorded every 2 h from 0–12 h and then every 4 h from 13–48 h. Additionally, MRS broth without inoculation served as the control group throughout the experiment.
Adhesion ability
According to the method reported by Anderson et al., to determine the adhesion of LAB to human Colon Colorectal adenocarcinoma cells (Caco-2) (16). Caco-2 cell line purchased from Wuhan Punos Company was cultured in DMEM medium containing 10% fetal bovine serum and 1% double antibody at 37°C Incubator with 5% carbon dioxide. Inoculate 1 mL of Caco-2 cell suspension (1.0 × 105 cells/mL, Vc) into a 12-well cell culture plate and wait until about 90% of the cells are attached. Then washed 3 times with PBS and 1 mL 1.0 × 108 CFU/mL (V0) LAB suspension (DMEM resuspension) was added to each well. After incubation at 37°C for 2 h, washed 3 times with PBS to remove unadherent bacteria. The adherent cells were digested with 0.25% pancreatic enzyme, diluted by 10 times gradient using DMEM medium, then 100 uL was applied to MRS Plate, incubated at 37°C for 24–48 h, and colony counting was performed. The adhesion rate (%) is calculated as (1 − V/V0) × 100.
Auto-aggregation and co-aggregation ability
To assess the auto-aggregation and co-aggregation abilities of the strains, the method described by Chen et al. was modified (17). The overnight cultures of LAB were adjusted to a concentration of 1 × 108 CFU/mL with sterile PBS for the ao-aggregation assay. 4 mL aliquot of the bacterial suspension was used to measure the initial OD600 nm (A0). The suspension was then incubated at 37°C for 6 h, after which the upper suspension was sampled to measure OD600 nm (A). The auto-aggregation percentage (%) was calculated using the formula (1-A/A0) × 100.
For the co-aggregation assay, 2 mL of suspension was mixed with 2 mL suspension of pathogenic bacteria (Escherichia coli ATCC 25922, Salmonella Braenderup H9812, Staphylococcus aureus ATCC 25923, Pseudomonas aeruginosa PAO1) and incubated at 37°C for 6 h. The absorbance at 600 nm (AX + Y) was measured at the end of the incubation. The co-aggregation rate (%) was calculated using the formula [1–2 AX + Y / (AX + AY)] × 100, where AX represents the OD600 of the LAB suspension and AY represents the OD600 of the pathogenic bacteria suspension.
Antagonistic activity
The antibacterial properties of the strain were evaluated using the Oxford cup method as reported (18). Following an overnight culture in MRS Liquid, the supernatant was separated by centrifugation (4°C, 8000 rpm for 10 min). To remove bacteria, the supernatant was filtered through a 0.22 μm sterile filter, and adjusted to pH = 7 using 1 M NaOH to obtain the cell-free supernatant. The pathogens were inoculated in LB broth (Escherichia coli ATCC 25922, Salmonella Braenderup H9812, Staphylococcus aureus ATCC 25923, Pseudomonas aeruginosa PAO1) was cultured at 37°C for 18 h and the concentration of the bacteria was adjusted to 1 × 107 CFU/mL by aseptic PBS. 100 μL of pathogen liquid was evenly spread on the LB plate. The Oxford cup was fixed on the plate and 100 μL cell-free supernatant of LAB was added, then placed in an incubator at 37°C for 24 h. Finally, the diameter of the inhibition zone was measured and recorded.
Antioxidative ability
Tolerance to hydrogen peroxide (H2O2)
Following the method proposed by Li et al. with modifications (19), the 2% (v/v) overnight culture of LAB (1 × 108 CFU/mL) was inoculated into MRS broth containing 0, 0.5, 1.0, 1.5, or 2.0 mmol/L H2O2. After incubation at 37°C for 8 h, the OD600 of the cultures was measured. The group with 0 mmol/L H2O2 served as the control. The resistance rate (%) was calculated as (ODtest/ODcontrol) × 100.
The ability of scavenging 1, 1-diphenyl-2-picrylhydrazyl (DPPH) free radical
Following the method reported by Lin and Chang, the DPPH free radical scavenging ability of LAB was measured (20). 2 mL of a 0.2 mmol/L DPPH solution in absolute ethanol was combined with 1 mL of either a cell-free supernatant or a bacterial suspension of the LAB strains in a centrifuge tube. The reaction was carried out in the dark at room temperature for 30 min. Then, the supernatant was collected by centrifugation (4°C, 8,000 rpm for 10 min). The OD517 of the supernatant was measured. Absolute ethanol was used in place of DPPH as the blank, and distilled water was used instead of the sample solution as the control. The DPPH free radical scavenging rate (%) was calculated as [1− (ODexperimental − ODblank) / ODcontrol] × 100.
Safety assessment
Hemolytic activity
The LAB was inoculated on the blood plate, Staphylococcus aureus ATCC 25923 was used as the positive control. The results were observed after culture at 37°C for 48 h. Hemolysis was categorized into β-hemolysis (the complete lysis of red blood cells, resulting in a clear hemolysis ring surrounding the colony), α-hemolysis (partial hydrolysis of red blood cells, forming a greenish hemolysis ring around the colony), and γ-hemolysis (no hydrolysis of red blood cells and no hemolysis zone is observed around the colony) (21).
Antibiotic susceptibility
The susceptibility of LAB to antibiotics was determined according to Zhang et al. with minor modifications (22). The sensitivity of the isolated strains to 16 antibiotics was evaluated by disk diffusion test, including cefazolin (KZ, 30 μg), erythromycin (E, 15 μg), chloramphenicol (C, 30 μg), tetracycline (TE, 30 μg), ampicillin (AMP, 10 μg), clindamycin (DA, 10 μg), penicillin G (P, 10 μg), cefotaxime (CTX, 30 μg), cefuroxim (CXM, 30 μg), amikacin (AK, 30 μg), vancomycin (VA, 30 μg), oxacillin (OX, 5 μg), norfloxacin (NOR, 5 μg), fosfomycin (S, 200 μg), rifampicin (RD, 5 μg), amoxicillin (AML, 25 μg). The suspension of LAB cultured overnight was diluted to 1 × 108 CFU/mL, and 100 μL was coated on the MRS plate and dried. After that, the paper containing antibiotics was placed on the surface of the MRS plate with tweezers. Then, cultured under anaerobic conditions at 37°C for 48 h. Antibiotic sensitivity was classified as resistance (R, ≤15 mm), intermediate (I, 16–20 mm), or sensitivity (S, ≥21 mm) by measuring the diameter of the inhibition zone (mm), according to the parameters of the Institute of Clinical and Laboratory Standards (13).
Statistical analysis
The results are expressed as mean ± standard deviation (SD) and were analyzed using the SPSS 19.0 statistical package (SPSS Inc., Chicago, USA). Significance was determined at p < 0.05 for statistically significant differences and at p < 0.01 for extremely significant differences. The figures were created using GraphPad Prism 9.5 (GraphPad Software Inc., La Jolla, USA).
Results
Preliminary screening
The isolated and purified strains were examined by microscopy after Gram staining. The staining results of 24 isolates were Gram-positive, which was consistent with the staining characteristics of LAB strains. Colony morphologies include spheres, short rods, and long rods (Figure 1).
The resilience of these 24 isolates to conditions simulating the GIT was subsequently assessed. It was found that only 10 isolates demonstrated remarkable tolerance, with a survival rate exceeding 50% under simulated GIT conditions (Figure 2). The survival rates of these 10 isolated strains varied between 59.17–84.85%. Notably, the three strains with the highest survival rates were F3 (83.91%), F4 (80.59%), and F8 (84.85%), each exhibiting survival rates exceeding 80%, which suggests a robust tolerance in the gastrointestinal tract GIT conditions. In contrast, the remaining 14 strains exhibited poor tolerance suggesting that they may not adequately survive after oral administration in a living organism. Consequently, only the 10 isolates with high survival rates in the GIT simulation were selected for further evaluation in subsequent experiments. Based on their morphological characteristics, these 10 strains were named F1 - F10.
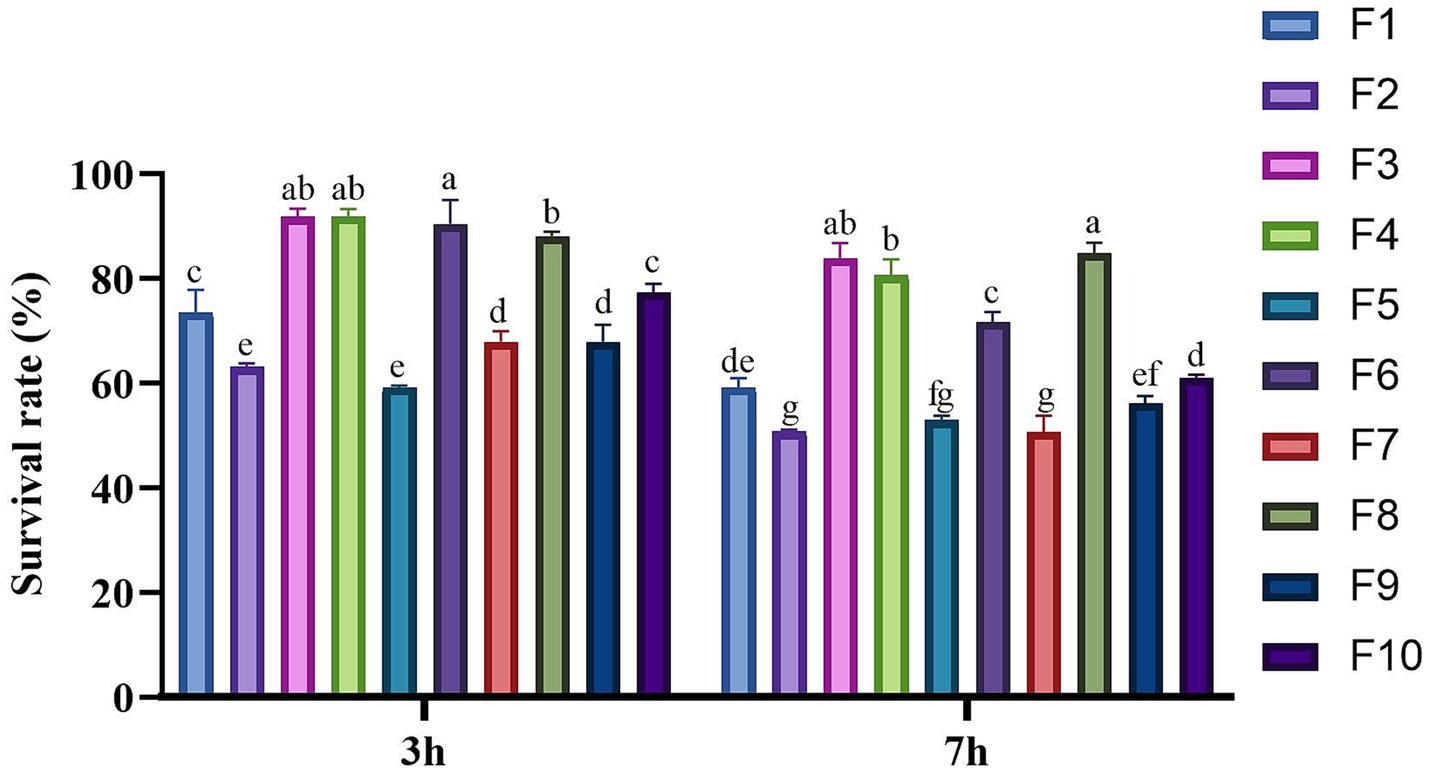
Figure 2. Survival rate of the isolates in the simulated GIT condition. Different letters marked above each column represent significant differences (Waller-Duncan, p < 0.05).
Species identification
The 16S rRNA gene sequence of the isolated strain was successfully amplified using PCR, and the sequence has been deposited in GenBank under the accession numbers PP989619 to PP989628. The most closely related reference sequence in GenBank was retrieved, and a phylogenetic tree based on the 16S gene was constructed using MEGA7.0 software (Figure 3). The analysis revealed that the 10 isolated strains comprised Lactobacillus acidophilus (F1, F2), Lactobacillus reuteri (F3, F4), Lactobacillus johnsonii (F5, F6, F7), Lactobacillus salivarius (F8, F9), and Lactobacillus brevis (F10). This classification aligns with the previous morphological clustering, indicating that strains with similar appearances are indeed the same species of LAB.
Growth ability
Analysis of the growth curves for the isolated strains in Figure 4 revealed distinct growth patterns. Notably, strains of the same species exhibited consistent growth characteristics. Lactobacillus reuteri (F3, F4) and Lactobacillus salivarius (F8, F9) exhibited rapid proliferation, reaching the stable growth phase around 12 h, especially for F8 and F9, the OD600 of these two strains exceeded 1.5, reaching 1.51 (F8) and 1.53 (F9) respectively, indicating strong growth capabilities; Lactobacillus acidophilus (F1, F2) and Lactobacillus brevis (F10) displayed a slower growth rate than the above four strains, OD600 ranging from 1–1.5, was lower than that of the four strains above. However, Lactobacillus johnsonii (F5, F6, F7) showed the poorest growth performance, entering the stable phase around 24 h. Significantly, the OD600 of these three strains were all lower than 1, which showed the lowest bacterial concentration compared with other strains.
Adhesion to Caco-2 cell line
Figure 5 illustrates the adhesion rates of the isolated strains to Caco-2 cells. The adhesion rates of the strains in this study varied between 4.87% and 18.05%. With the exception of four strains: F1 (4.87%), F5 (7.59%), F8 (5.99%) and F9 (9.91%), all other strains exhibited adhesion rates exceeding 10%. Notably, F4 demonstrated the highest adhesion capacity, reaching 18.5%, followed by F10 (13.81%) and F6 (13.16%). In contrast, F1 had the lowest adhesion rate (4.87%).
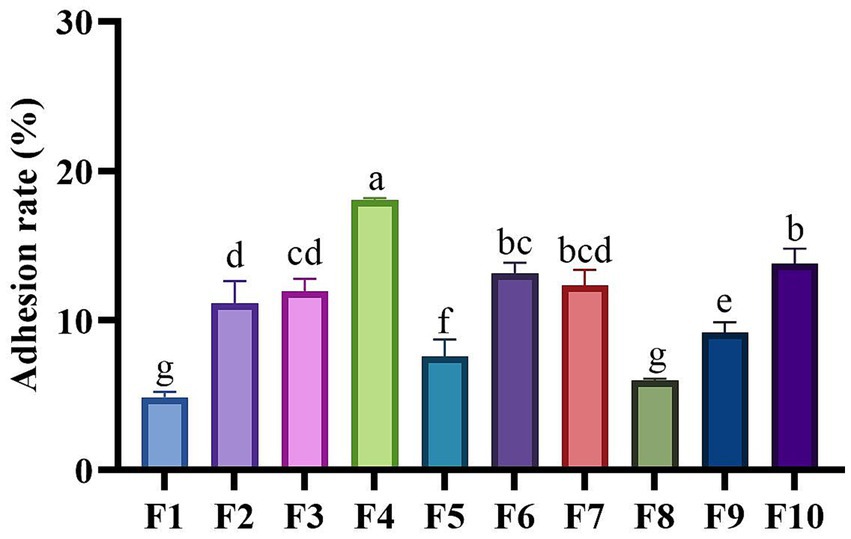
Figure 5. Adhesion capabilities of LAB isolates to Caco-2 cell line. Different letters marked above each column represent significant differences (Waller-Duncan, p < 0.05).
Auto-aggregation and Co-aggregation ability
Based on the results of auto-aggregation and co-aggregation shown in Table 1, the isolated strains exhibited varying aggregation abilities. Among the 10 LAB strains isolated in this study, the top three with the highest auto-aggregation ability were F2 (88.44%), F4 (86.79%), and F6 (81.71%), each with an auto-aggregation rate exceeding 80%. The strains demonstrating the high co-aggregation potential with Staphylococcus aureus ATCC 25923 were identified as F2 (87.44%), F5 (78.52%), and F3 (77.69%). In the case of Salmonella Braenderup H9812, the effective co-aggregating strains were F6 (69.64%), F2 (68.77%) and F9 (68.76%). For Escherichia coli ATCC 25922, the strains that exhibited the top three co-aggregation capacity were F6 (68.01%), F1 (67.23), and F2 (66.69%). Lastly, in the context of Pseudomonas aeruginosa PAO1, the strains with robust co-aggregation ability were found to be F6 (70.89%), F2 (70.12%), and F9 (69.16%).
Antimicrobial ability
Table 2 illustrates the inhibitory effects of 10 LAB isolates against four prevalent intestinal pathogens. The efficacy of these strains in inhibiting different pathogens varies significantly. Research has demonstrated that the antibacterial capacity of LAB can be classified into four levels based on the diameter of the inhibition zone: I (8 mm < zone diameters ≤12 mm), II (12 mm < zone diameters ≤16 mm), III (16 mm < zone diameters ≤20 mm), and IV (20 mm < zone diameters) (23). Compared the diameters of the inhibition zones of all strains, and the results showed that F9 (19.21 mm), F4 (19.14 mm), and F8 (17.88 mm) exhibit potent inhibitory effects against Escherichia coli ATCC 25922; F6 (30.67 mm), F1 (27.4 mm), and F2 (17.46 mm) demonstrate strong inhibition against Staphylococcus aureus ATCC 25923; F10 (23.32 mm), F3 (22.04 mm), and F2 (17.41 mm) are particularly effective against Salmonella Braenderup H9812; F10 (28.59 mm), F5 (26.36 mm), and F1 (26.13 mm) show significant inhibitory effects on Pseudomonas aeruginosa PAO1. Moreover, except for F7 (17.97 mm) and F8 (19.13 mm), whose inhibition diameters against Pseudomonas aeruginosa PAO1 were at the III level (16 mm < zone diameters ≤20 mm), the inhibition diameters of the other isolated strains were all at the IV level (20 mm < zone diameters).
Analysis of antioxidant activity
Tables 3 and Figure 6 present the findings on the isolated strains’ capacity to withstand H2O2 and neutralize DPPH free radicals, respectively. The data reveal that all 10 isolates exhibited tolerance to varying concentrations of H2O2, with their resilience diminishing as the H2O2 concentration intensified. The most tolerant strains, in descending order, were identified as F10 (6.44%), F4 (4.01%), and F6 (3.62%) in the highest concentration (2.0 mmol/L H2O2). In the scavenging of DPPH free radicals, the cell-free supernatant from the isolated strains demonstrated a superior clearance rate compared to the bacterial suspensions. The cell-free supernatant achieved clearance rates ranging from 80.62–88.12%, whereas the bacterial suspensions only managed rates between 7.18–30.21%. Notably, the cell-free supernatant of F7 (88.12%), F4 (86.71%), and F2 (85.24%) emerged as the top 3 performers in this regard.
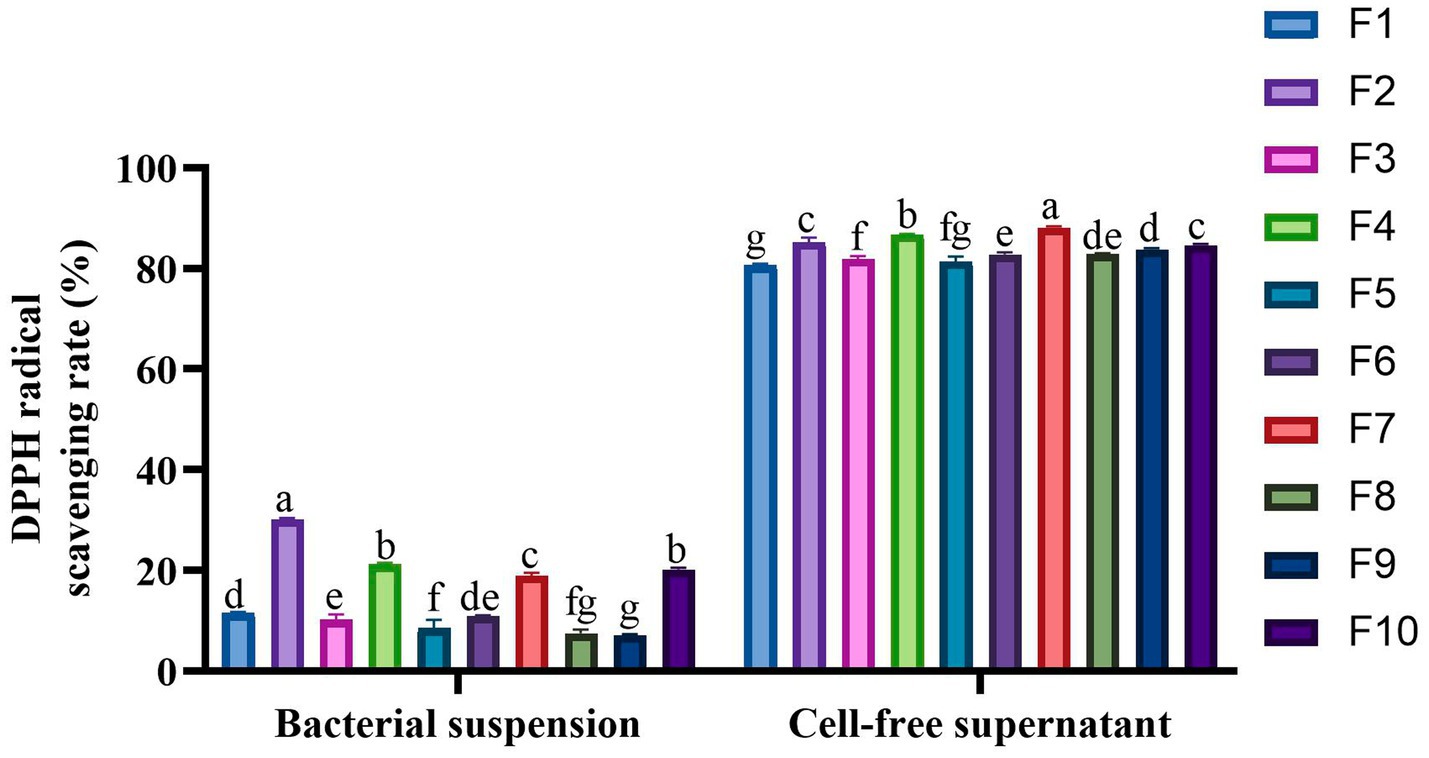
Figure 6. DPPH radical scavenging activity of the 10 LAB strains. Different letters marked above each column represent significant differences (Waller-Duncan, p < 0.05).
Safety analysis
The hemolytic test results indicated that none of the 10 LAB isolates from this study exhibited hemolytic activity. Furthermore, the antimicrobial resistance assessment of these isolates against 18 antibiotics revealed that all strains were susceptible to rifampicin (RD) but demonstrated resistance to norfloxacin (NOR). Notably, F6 displayed the highest level of drug resistance, with a sensitivity rate of only 23%, being susceptible to chloramphenicol (C), amikacin (AK), lecithin (S), and rifampicin (RD). In contrast, F2 had the highest sensitivity rate at 87.5%; followed by F4, F5, and F7, with their sensitivity rates all being 81.25% (Table 4).
Discussion
Probiotics offer multiple benefits, including improved intestinal health, enhanced antioxidant and immune functions, and pathogen inhibition, reducing antibiotic reliance and addressing resistance (24). Probiotics must be well-adapted and safe for the host (25), hence isolating them from similar hosts improves gastrointestinal tract adaptation and efficacy. Studies show probiotic benefits in cats, but most strains are not cat-derived (26, 27), limiting diversity and potential efficacy. This study isolates LAB from healthy cats to provide more appropriate and effective probiotics for cats’ health.
LAB are Gram-positive, appearing purple when subjected to Gram staining (28). Based on this characteristic, we preliminary screened the strains isolated and purified from MRS plates, ultimately identifying 24 Gram-positive strains as potential LAB candidates. For probiotics to be effective, they must survive the harsh conditions of the gastric and intestinal fluids after oral administration (29). Therefore, we initially assessed the gastrointestinal fluid tolerance of all suspected LAB isolates by simulating gastrointestinal conditions, resulting in the selection of 10 strains with strong tolerance. These strains are likely to have a high survival rate in the host after oral administration, ensuring effective utilization. Species identification through the 16S rDNA gene analysis confirmed that all 10 isolated strains were indeed LAB, consistent with the Gram staining results. These strains were classified into five species: Lactobacillus acidophilus (F1, F2), Lactobacillus reuteri (F3, F4), Lactobacillus johnsonii (F5, F6, F7), Lactobacillus salivarius (F8, F9), and Lactobacillus brevis (F10). This study has expanded the diversity of LAB isolated in cats.
As we know, probiotics are typically introduced into the host body via oral administration as living organisms to elicit their beneficial effects. Only those strains capable of adhering to the intestinal lining upon ingestion can successfully colonize and subsequently unleash their probiotic properties. Strains that fail to comply effectively are expelled from the host’s body along with the intestinal contents, leading to diminished strain efficacy, reduced probiotic impact, and potentially rendering the probiotics ineffective in the host (30). In 2018, Deng et al. reported that among strains of the same type, those with better adhesion rates had better probiotic effects, confirming that the adhesion rate of probiotics is closely related to their probiotic properties (31). In addition, probiotics can also compete with intestinal pathogens for colonization through strong adhesion, thereby exerting an antibacterial effect (32). Therefore, the ability to adhere and proliferate effectively is vital for cats’ probiotics to exert their beneficial effects and ensure high rates of utilization (33). The cell line Caco-2 is widely used as a laboratory model and is considered an important screening method for evaluating the adhesion ability of probiotics (34). The results showed that the adhesion rates of the 10 isolated strains ranged from 4.87–18.5%, consistent with the findings reported by Vidhyasagar et al., who observed adhesion rates between 9 and 17% in their isolated LAB strains from idly batter (35); Additionally, the LAB isolated from dog feces by Liu et al. exhibited a maximum adhesion rate of merely 3.62% (36), highlighting the robust adhesion capabilities of the strains in the current study. This comparison suggests that there are significant differences in the adhesion rates of LAB sourced from various origins. Of course, these disparities may be attributed to the inherent specificity of the bacterial strains themselves. Growth evaluation showed that different species of LAB had different growth abilities. While strains within the same species exhibited similar growth characteristics. Among the isolates, Lactobacillus johnsonii strains (F5, F6, and F7) demonstrated the most inferior growth performance, which suggests that LABs of the same species have comparable growth performance. In contrast, a study by Zhang et al. involved the isolation of five strains of Lactobacillus plantarum from cow’s milk, revealing distinctly different growth curves among these strains (22). When considered alongside the results of the present study, it becomes evident that the growth characteristics of LAB are not solely strain-specific but are also influenced by a range of other factors. These factors may include genetic variations within the strain’s genome, the composition of the culture environment, and the availability of nutrients, among others. Further research is necessary to fully understand the specific factors contributing to the differential growth characteristics observed in LAB strains. It is recommended that the selection of probiotics be tailored to the specific application scenarios and conditions to ensure optimal growth performance of the chosen strains.
The auto-aggregation capability of probiotics aids in the adherence of bacterial cells to the intestinal epithelial wall, while their co-aggregation ability helps prevent pathogen colonization in the intestine (37). The auto-aggregation rates of the strains isolated in this study ranged from 36.66–88.44%, with their co-aggregation potential reaching 25.82–87.44%. Significant differences were noted among the strains, yet overall, they outperformed the LAB strains isolated from panda feces by Wang et al., which had self-aggregation rates of 11.83–41.61% and co-aggregation rates of 7.89–30.15% (38). This demonstrates the robust self-aggregation and co-aggregation abilities of the LAB isolated here. Research indicates that LAB hold promise as an alternative to antibiotics in combating pathogen infections. This study evaluated the antibacterial capacity of the isolated strains against four common intestinal pathogens (Escherichia coli ATCC 25922, Salmonella Braenderup H9812, Staphylococcus aureus ATCC 25923, Pseudomonas aeruginosa PAO1). The results revealed that all strains exhibited excellent antibacterial properties, the majority of their antibacterial diameters fall into III level (16 mm < zone diameters≤20 mm) or IV level (20 mm < zone diameters), which is considerably superior to the antibacterial capabilities of LAB isolated in other reports (23, 39). In their studies, the most antibacterial diameters were classified as level II (12 mm < zone diameters ≤16 mm). Moreover, Among the four pathogens, the strain isolated in this study had the best inhibitory effect on Pseudomonas aeruginosa PAO1. This suggests that cat-derived LAB may produce substances that can effectively antagonize the Pseudomonas aeruginosa PAO1, which may be specific bacteriocins or antibacterial enzymes (32, 40). However, the specific antibacterial substances and mechanisms need further study. In conjunction with the inhibition results against the four pathogens, F1, F4, and F10 demonstrated strong comprehensive antibacterial abilities. This result provides a reference for the selection of clinical antibacterial probiotics for cats, which is beneficial to reduce the use of antibiotics and the problem of drug resistance.
An imbalance between pro-oxidants and antioxidants in the body leads to an overproduction of reactive oxygen species (ROS), causing oxidative stress. This can lead to inflammation, organ damage, and other diseases (41). Research indicates that certain LAB strains possess significant antioxidant potential, which can reduce the risk of oxidative damage to host cells and lower the incidence of chronic diseases, qualifying them as effective natural antioxidants (42). The evaluation results of the antioxidant capacity of the isolated strains in this study showed that all strains showed tolerance to H2O2 concentrations of 0.5-2 mmol/L, among which F10 showed the highest tolerance, with the survival rate of 6.44%. In contrast, in Wang’s report, only 2 of the 5 LAB isolated from panda feces could tolerate H2O2 at 2 mmol/L (38). Moreover, our study found that the supernatant of the isolated strains had a significantly higher DPPH free radical clearance rate (80.62–88.12%) than the bacterial suspension (7.17–30.21%), which is aligns with Zhou et al.’s report (43). This could be attributed to the fact that the supernatant of LAB is abundant in antioxidant metabolites, such as Exopolysaccharides (EPS) (44). The metabolites are secreted by the strains as they grow. To guarantee that the strains demonstrate superior antioxidant properties, probiotic strains must possess outstanding growth and proliferation abilities within the body. This ensures the sustained and consistent production of beneficial metabolites, thereby enhancing the probiotics’ exceptional antioxidant capabilities. However, the precise substances responsible for the antioxidant effects in our isolated strains require further experimental confirmation. Notably, F7 had the strongest free radical scavenging ability, reaching 88.12%, which, although lower than the 95.98% clearance rate of Lactobacillus plantarum GXL94 isolated from capsicum by Zhou (43), but significantly higher than the 57–66% rate of two LAB strains isolated from dog feces by Zhao et al. (13). These differences may stem from variations in strain origin or complex antioxidant mechanisms (45). In conclusion, the strains isolated in this study demonstrated robust antioxidant capacity, highlighting their potential in cats as natural antioxidants and their suitability for reducing oxidative stress.
The hemolysis test and antibiotic resistance profiling are crucial for assessing the safety of probiotics. Fortunately, none of the 10 strains isolated in this study exhibited hemolytic activity. In this study, the least sensitive isolate, F6, was only susceptible to 4 of the 16 tested antibiotics, indicating a relatively lower safety profile compared to the other strains, which all exhibited higher sensitivities, exceeding 62.4%. Notably, all strains showed resistance to norfloxacin, which is hypothesized to be an inherent resistance trait of these strains (46), unrelated to the presence of resistance genes. Additionally, all 10 LABs isolated here belonged to the Lactobacillus genus, a group within LAB that is generally recognized as safe (GRAS) (47). In summary, all the isolates in this study can be considered safe.
Conclusion
In this study, 24 strains of LAB were isolated from the feces of 20 healthy cats, and 10 strains with high survival rates in the simulated GIT environment were selected to further study. Comprehensive analysis of these metrics indicated that F4 (Lactobacillus reuteri) and F10 (Lactobacillus brevis) demonstrated excellent performance and hold significant potential as probiotics. This study provides a scientific basis for the selection and application of cat-derive probiotics. However, it is important to note that these in vitro evaluations are not sufficient to confirm the prebiotic properties of these LAB strains in vivo, and further research is needed to determine whether their effects in cats align with the in vitro findings. Additionally, there are hundreds of LAB species, this study only isolates 5 species of Lactobacillus. Consequently, the findings are subject to certain limitations. Future research can enhance the scope by increasing the sample size to isolate a wider array of cat-derived LAB, thereby expanding our understanding of their diversity and probiotic potential.
Data availability statement
The datasets presented in this study can be found in online repositories. The names of the repository/repositories and accession number(s) can be found in the article/supplementary material.
Ethics statement
The animal study was approved by The Animal Ethical and Welfare Committee of Sichuan Agricultural University. The study was conducted in accordance with the local legislation and institutional requirements. The cell lines present in this study were obtained from Wuhan Punos Company.
Author contributions
JW: Data curation, Formal analysis, Writing – original draft, Methodology. XY: Data curation, Writing – original draft. YP: Data curation, Formal analysis, Writing – original draft. JZ: Data curation, Validation, Writing – review & editing. YH: Methodology, Supervision, Writing – review & editing. ZhZ: Formal analysis, Project administration, Writing – review & editing. HL: Funding acquisition, Validation, Writing – review & editing. HF: Data curation, Project administration, Supervision, Writing – review & editing. ZiZ: Conceptualization, Supervision, Writing – review & editing. GP: Conceptualization, Supervision, Writing – review & editing.
Funding
The author(s) declare that financial support was received for the research, authorship, and/or publication of this article. This study was financially supported by the National Science and Technology Department’s “13th Five-Year” Special Subproject of China (No. 2016YFD0501009).
Acknowledgments
Thanks to the cattery staff for helping collect healthy cat fecal samples. Moreover, we appreciated to the editors and reviewers for their valuable contributions, which significantly enhanced the quality of the manuscript.
Conflict of interest
The authors declare that the research was conducted in the absence of any commercial or financial relationships that could be construed as a potential conflict of interest.
Publisher’s note
All claims expressed in this article are solely those of the authors and do not necessarily represent those of their affiliated organizations, or those of the publisher, the editors and the reviewers. Any product that may be evaluated in this article, or claim that may be made by its manufacturer, is not guaranteed or endorsed by the publisher.
References
1. Lee, W, and Hase, K. Gut microbiota–generated metabolites in animal health and disease. Nat Chem Biol. (2014) 10:416–24. doi: 10.1038/nchembio.1535
2. Cook, AK. Feline infectious diarrhea. Top Companion Anim Med. (2008) 23:169–76. doi: 10.1053/j.tcam.2008.07.001
3. Sui, Y, Song, P, Chen, G, Zuo, S, Liu, H, Guo, J, et al. Gut microbiota and tritrichomonas foetus infection: a study of prevalence and risk factors based on pet cats. Prev Vet Med. (2024) 226:106162. doi: 10.1016/j.prevetmed.2024.106162
4. Zha, M, Zhu, S, and Chen, Y. Probiotics and cat health: a review of progress and prospects. Microorganisms. (2024) 12:1080. doi: 10.3390/microorganisms12061080
5. Hill, C, Guarner, F, Reid, G, Gibson, GR, Merenstein, DJ, Pot, B, et al. Expert consensus document: the international scientific association for probiotics and prebiotics consensus statement on the scope and appropriate use of the term probiotic. Nat Rev Gastroenterol Hepatol. (2014) 11:506–14. doi: 10.1038/nrgastro.2014.66
6. Bourdichon, F, Casaregola, S, Farrokh, C, Frisvad, JC, Gerds, ML, Hammes, WP, et al. Food fermentations: microorganisms with technological beneficial use. Int J Food Microbiol. (2012) 154:87–97. doi: 10.1016/j.ijfoodmicro.2011.12.030
7. Yang, Q, and Wu, Z. Gut probiotics and health of dogs and cats: benefits, applications, and underlying mechanisms. Microorganisms. (2023) 11:2452. doi: 10.3390/microorganisms11102452
8. Kerek, A, Szabó, E, Szabó, Á, Papp, M, Bányai, K, Kardos, G, et al. Investigating antimicrobial resistance genes in probiotic products for companion animals. Front Vet Sci. (2024) 11:1464351. doi: 10.3389/fvets.2024.1464351
9. Li, Y, Ali, I, Lei, Z, Li, Y, Yang, M, Yang, C, et al. Effect of a multistrain probiotic on feline gut health through the fecal microbiota and its metabolite scfas. Meta. (2023) 13:228. doi: 10.3390/metabo13020228
10. Lappin, MR, Veir, JK, Satyaraj, E, and Czarnecki-Maulden, G. Pilot study to evaluate the effect of oral supplementation of Enterococcus faecium sf68 on cats with latent feline herpesvirus 1. J Feline Med Surg. (2009) 11:650–4. doi: 10.1016/j.jfms.2008.12.006
11. Bybee, SN, Scorza, AV, and Lappin, MR. Effect of the probiotic Enterococcus faecium sf68 on presence of diarrhea in cats and dogs housed in an animal shelter. J Vet Intern Med. (2011) 25:856–60. doi: 10.1111/j.1939-1676.2011.0738.x
12. On Additives, EP, Bampidis, V, Azimonti, G, de Lourdes Bastos, M, Christensen, H, Dusemund, B, et al. Safety and efficacy of Lactobacillus reuteri nbf-2 (dsm 32264) as a feed additive for cats. EFSA J. (2019) 17:5526. doi: 10.2903/j.efsa.2019.5526
13. Zhao, M, Liu, K, Zhang, Y, Li, Y, Zhou, N, and Li, G. Probiotic characteristics and whole-genome sequence analysis of Pediococcus acidilactici isolated from the feces of adult beagles. Front Microbiol. (2023) 14:1179953. doi: 10.3389/fmicb.2023.1179953
14. Zhang, B, Wang, Y, Tan, Z, Li, Z, Jiao, Z, and Huang, Q. Screening of probiotic activities of lactobacilli strains isolated from traditional tibetan qula, a raw yak milk cheese. Asian Australas J Anim Sci. (2016) 29:1490–9. doi: 10.5713/ajas.15.0849
15. Liu, W, Chen, M, Duo, L, Wang, J, Guo, S, Sun, H, et al. Characterization of potentially probiotic lactic acid bacteria and bifidobacteria isolated from human colostrum. J Dairy Sci. (2020) 103:4013–25. doi: 10.3168/jds.2019-17602
16. Anderson, RC, Cookson, AL, McNabb, WC, Kelly, WJ, and Roy, NC. Lactobacillus plantarum dsm 2648 is a potential probiotic that enhances intestinal barrier function. FEMS Microbiol Lett. (2010) 309:184–92. doi: 10.1111/j.1574-6968.2010.02038.x
17. Chen, X, Xu, J, Shuai, J, Chen, J, Zhang, Z, and Fang, W. The s-layer proteins of Lactobacillus crispatus strain zj001 is responsible for competitive exclusion against Escherichia coli o157: h7 and Salmonella typhimurium. Int J Food Microbiol. (2007) 115:307–12. doi: 10.1016/j.ijfoodmicro.2006.11.007
18. Fontana, C, Cocconcelli, PS, Vignolo, G, and Saavedra, L. Occurrence of antilisterial structural bacteriocins genes in meat borne lactic acid bacteria. Food Control. (2015) 47:53–9. doi: 10.1016/j.foodcont.2014.06.021
19. Li, S, Zhao, Y, Zhang, L, Zhang, X, Huang, L, Li, D, et al. Antioxidant activity of Lactobacillus plantarum strains isolated from traditional Chinese fermented foods. Food Chem. (2012) 135:1914–9. doi: 10.1016/j.foodchem.2012.06.048
20. Lin, M, and Chang, F. Antioxidative effect of intestinal bacteria Bifidobacterium longum atcc 15708 and Lactobacillus acidophilus atcc 4356. Dig Dis Sci. (2000) 45:1617–22. doi: 10.1023/A:1005577330695
21. Reuben, RC, Roy, PC, Sarkar, SL, Alam, R, and Jahid, IK. Isolation, characterization, and assessment of lactic acid bacteria toward their selection as poultry probiotics. BMC Microbiol. (2019) 19:1–20. doi: 10.1186/s12866-019-1626-0
22. Zhang, Q, Wang, M, Ma, X, Li, Z, Jiang, C, Pan, Y, et al. In vitro investigation on lactic acid bacteria isolatedfrom yak faeces for potential probiotics. Front Cell Infect Microbiol. (2022) 12:984537. doi: 10.3389/fcimb.2022.984537
23. Zhang, W, Lai, S, Zhou, Z, Yang, J, Liu, H, Zhong, Z, et al. Screening and evaluation of lactic acid bacteria with probiotic potential from local Holstein raw milk. Front Microbiol. (2022) 13:918774. doi: 10.3389/fmicb.2022.918774
24. Suez, J, Zmora, N, Segal, E, and Elinav, E. The pros, cons, and many unknowns of probiotics. Nat Med. (2019) 25:716–29. doi: 10.1038/s41591-019-0439-x
25. Dunne, C, O’Mahony, L, Murphy, L, Thornton, G, Morrissey, D, O’Halloran, S, et al. In vitro selection criteria for probiotic bacteria of human origin: correlation with in vivo findings. Am J Clin Nutr. (2001) 73:386s–92s. doi: 10.1093/ajcn/73.2.386s
26. Rossi, G, Jergens, A, Cerquetella, M, Berardi, S, Di Cicco, E, Bassotti, G, et al. Effects of a probiotic (slab51™) on clinical and histologic variables and microbiota of cats with chronic constipation/megacolon: a pilot study. Benef Microbes. (2018) 9:101–10. doi: 10.3920/BM2017.0023
27. Torres-Henderson, C, Summers, S, Suchodolski, J, and Lappin, MR. Effect of Enterococcus faecium strain sf68 on gastrointestinal signs and fecal microbiome in cats administered amoxicillin-clavulanate. Top Companion Anim Med. (2017) 32:104–8. doi: 10.1053/j.tcam.2017.11.002
28. Wyszyńska, A, Kobierecka, P, Bardowski, J, and Jagusztyn-Krynicka, EK. Lactic acid bacteria—20 years exploring their potential as live vectors for mucosal vaccination. Appl Microbiol Biotechnol. (2015) 99:2967–77. doi: 10.1007/s00253-015-6498-0
29. Zommiti, M, Bouffartigues, E, Maillot, O, Barreau, M, Szunerits, S, Sebei, K, et al. In vitro assessment of the probiotic properties and bacteriocinogenic potential of Pediococcus pentosaceus mzf16 isolated from artisanal tunisian meat “dried ossban”. Front Microbiol. (2018) 9:2607. doi: 10.3389/fmicb.2018.02607
30. Nishiyama, K, Sugiyama, M, and Mukai, T. Adhesion properties of lactic acid bacteria on intestinal mucin. Microorganisms. (2016) 4:34. doi: 10.3390/microorganisms4030034
31. Deng, Z, Dai, T, Zhang, W, Zhu, J, Luo, XM, Fu, D, et al. Glyceraldehyde-3-phosphate dehydrogenase increases the adhesion of Lactobacillus reuteri to host mucin to enhance probiotic effects. Int J Mol Sci. (2020) 21:9756. doi: 10.3390/ijms21249756
32. Van Zyl, WF, Deane, SM, and Dicks, LM. Molecular insights into probiotic mechanisms of action employed against intestinal pathogenic bacteria. Gut Microbes. (2020) 12:1831339. doi: 10.1080/19490976.2020.1831339
33. De Melo Pereira, GV, de Oliveira Coelho, B, Júnior, AIM, Thomaz-Soccol, V, and Soccol, CR. How to select a probiotic? A review and update of methods and criteria. Biotechnol Adv. (2018) 36:2060–76. doi: 10.1016/j.biotechadv.2018.09.003
34. Vasiee, A, Falah, F, Behbahani, BA, and Tabatabaee-Yazdi, F. Probiotic characterization of pediococcus strains isolated from iranian cereal-dairy fermented product: interaction with pathogenic bacteria and the enteric cell line caco-2. J Biosci Bioeng. (2020) 130:471–9. doi: 10.1016/j.jbiosc.2020.07.002
35. Vidhyasagar, V, and Jeevaratnam, K. Evaluation of Pediococcus pentosaceus strains isolated from idly batter for probiotic properties in vitro. J Funct Foods. (2013) 5:235–43. doi: 10.1016/j.jff.2012.10.012
36. Liu, Y, Wang, J, Zheng, H, Xin, J, Li, G, Zhong, Z, et al. Multi-functional properties of lactic acid bacteria strains derived from canine faeces. Front. Vet. Sci. (2024) 11:1404580. doi: 10.3389/fvets.2024.1404580
37. Janković, T, Frece, J, Abram, M, and Gobin, I. Aggregation ability of potential probiotic Lactobacillus plantarum strains. Int J Sanitary Eng Res. (2012) 6:19–24.
38. Wang, J, Pu, Y, Zeng, Y, Chen, Y, Zhao, W, Niu, L, et al. Multi-functional potential of five lactic acid bacteria strains derived from giant panda (Ailuropoda melanoleuca). Probiotics Antimicrob Proteins. (2023) 15:668–81. doi: 10.1007/s12602-021-09881-6
39. Coman, MM, Verdenelli, MC, Cecchini, C, Belà, B, Gramenzi, A, Orpianesi, C, et al. Probiotic characterization of lactobacillus isolates from canine faeces. J Appl Microbiol. (2019) 126:1245–56. doi: 10.1111/jam.14197
40. Özogul, F, and Hamed, I. The importance of lactic acid bacteria for the prevention of bacterial growth and their biogenic amines formation: a review. Crit Rev Food Sci Nutr. (2018) 58:1660–70. doi: 10.1080/10408398.2016.1277972
41. Pisoschi, AM, and Pop, A. The role of antioxidants in the chemistry of oxidative stress: a review. Eur J Med Chem. (2015) 97:55–74. doi: 10.1016/j.ejmech.2015.04.040
42. Mishra, V, Shah, C, Mokashe, N, Chavan, R, Yadav, H, and Prajapati, J. Probiotics as potential antioxidants: a systematic review. J Agric Food Chem. (2015) 63:3615–26. doi: 10.1021/jf506326t
43. Zhou, Y, Gong, W, Xu, C, Zhu, Z, Peng, Y, and Xie, C. Probiotic assessment and antioxidant characterization of Lactobacillus plantarum gxl94 isolated from fermented chili. Front Microbiol. (2022) 13:997940. doi: 10.3389/fmicb.2022.997940
44. Sharma, P, Sharma, A, and Lee, H. Antioxidant potential of exopolysaccharides from lactic acid bacteria: a comprehensive review. Int J Biol Macromol. (2024) 281:135536. doi: 10.1016/j.ijbiomac.2024.135536
45. Feng, T, and Wang, J. Oxidative stress tolerance and antioxidant capacity of lactic acid bacteria as probiotic: a systematic review. Gut Microbes. (2020) 12:1801944. doi: 10.1080/19490976.2020.1801944
46. Liasi, SA, Azmi, TI, Hassan, MD, Shuhaimi, M, Rosfarizan, M, and Ariff, AB. Antimicrobial activity and antibiotic sensitivity of three isolates of lactic acid bacteria from fermented fish product, budu. Malays J Microbiol. (2009) 5:33–7.
47. Dahiya, D, and Nigam, PS. The gut microbiota influenced by the intake of probiotics and functional foods with prebiotics can sustain wellness and alleviate certain ailments like gut-inflammation and colon-cancer. Microorganisms. (2022) 10:665. doi: 10.3390/microorganisms10030665
Keywords: cat, lactic acid bacteria strains, probiotics, antimicrobial activity, antioxidant capacity
Citation: Wang J, Yang X, Peng Y, Zhang J, Huang Y, Zhong Z, Liu H, Fu H, Zhou Z and Peng G (2024) Isolation and in vitro investigation on lactic acid bacteria for potential probiotic properties from cat feces. Front. Vet. Sci. 11:1495745. doi: 10.3389/fvets.2024.1495745
Edited by:
Gulbeena Saleem, University of Veterinary and Animal Sciences, PakistanReviewed by:
Shakira Ghazanfar, National Institute for Genomics and Advanced Biotechnology (NIGAB), PakistanZahida Fatima, Pakistan Agricultural Research Council, Pakistan
Copyright © 2024 Wang, Yang, Peng, Zhang, Huang, Zhong, Liu, Fu, Zhou and Peng. This is an open-access article distributed under the terms of the Creative Commons Attribution License (CC BY). The use, distribution or reproduction in other forums is permitted, provided the original author(s) and the copyright owner(s) are credited and that the original publication in this journal is cited, in accordance with accepted academic practice. No use, distribution or reproduction is permitted which does not comply with these terms.
*Correspondence: Ziyao Zhou, enpob3VAc2ljYXUuZWR1LmNu; Guangneng Peng, cGduLnNpY2F1QDE2My5jb20=
†These authors have contributed equally to this work