- 1Animal Nutrition Institute, Sichuan Agricultural University, Chengdu, China
- 2Chongqing Academy of Animal Sciences, Chongqing, China
Rumen-protected glucose (RPG) and taurine (TAU) are crucial for the nutrition and physiology of ruminants, enhancing production performance and mitigating negative energy balance. This study aimed to assess the impact of RPG levels and TAU supplementation on the body weight, antioxidant capacity, immune function and stress responses in yaks before and after transport. Thirty-two healthy male yaks, aged 3 years and weighing 172.5 ± 10.2 kg, were randomly assigned to one of four dietary treatments: (1) low RPG and low TAU (LRLT), with 1% RPG and 5 g/d TAU; (2) low RPG and high TAU (LRHT), with 1% RPG and 15 g/d TAU; (3) high RPG and low TAU (HRLT), with 3% RPG and 5 g/d TAU; and (4) high RPG and high TAU (HRHT), with 3% RPG and 15 g/d TAU. The yaks were treated with the corresponding diet for 7 days, then received 9 h of transportation, and finally fed the same diet at their destination for 30 days. The weight was measured before and on days 0 and 30 after transport, and the plasma was collected before and on day 0, 10, and 30 after transport for analysis of biochemical, antioxidant, immune, and stress response indicators. We observed that transport increased plasma concentrations of total cholesterol, total protein, lactate dehydrogenase, creatine kinase, malondialdehyde, cortisol and lipopolysaccharides of yaks among treatments, while decreased their BW and plasma IL-10 concentration. Increasing TAU supplementation reduced weight loss (8.42 vs. 11.9 kg) and weight loss percent (4.83% vs. 6.87%) in yaks after transport. The concentration of MDA in plasma was lower in HRHT than in LRLT at day 0 after transport (p = 0.03). The activity of GSH-PX was higher in HRHT than in LRLT at day 10 after transport (p = 0.04). Concentrations of IL-10 at day 0 and 10 after transport was higher in HRHT than in LRLT and HRLT (p = 0.02, p = 0.01, respectively). With the increase of TAU supplementation, concentrations of IL-1β at day 30 after transport (p = 0.02), TNF-α at day 0 after transport (p = 0.02), COR at day 10 (p = 0.03) and 30 (p = 0.05) after transport as well as LPS at day 0 after transport (p = 0.04) decreased. In addition, concentrations of COR at day 0 after transport was lower in LRHT and HRHT than in LRLT (p = 0.03). Based on all the results, we demonstrated that increasing TAU supplementation levels reduced post-transport weight loss in yaks, improved their antioxidant capacity and immune function, and alleviated stress responses. Considering the effect of resistance to transport stress and costs, the optimal treatment identified in this study involved a diet containing 1% RPG and supplemented with 15 g/d TAU.
1 Introduction
Yak is a vital component of animal husbandry in China’s high-altitude grazing regions, providing herders with high-quality milk, meat, and fur, thus holding significant economic value. Grazing on native pasture is the traditional way of raising yaks. Yet, these pastures experience substantial seasonal fluctuations in forage availability, often leading to considerable stress on the pastures, particularly in winter (1). Typically, due to the intake of nutrients falling below the necessary maintenance levels, the weight of grazing yaks will be reduced by about 25% after a cold season, which extends the feeding cycle of yaks, reduces the slaughter yield, and seriously hinders the sustainable development of the yak industry (2, 3). Off-site fattening has increasingly become the primary feeding method for yaks during the cold season, mitigating the traditional contradiction between the forage availability and livestock demand in grazing areas, while enhancing the growth efficiency and meat quality of yaks, resulting in higher economic returns.
Some herders relocate yaks from high-altitude grazing areas to low-altitude farms for breeding. However, live transportation means yaks are exposed to multiple stressors, including noise, crowding, starvation and high temperature, which can exacerbate the adaptive and defensive responses, causing transport stress and compromising health and welfare in animals (4, 5). It has been reported that feed and water deprivation during transport caused dehydration in the body, weight loss and negative energy balance, thereby damaging organismal metabolism and reducing slaughter value (6). Furthermore, prolonged crowding and standing can increase muscle tension and fatigue, and may also promote the development of respiratory diseases (7, 8). Currently, nutritional regulation is a commonly used measure to alleviate transport stress in animals.
Rumen-protected glucose (RPG) can be released directly from the small intestine into the blood circulation, because its surface is covered with special materials like fatty acids, preventing it from being fermented and degraded in the rumen. Unlike monogastric animals, ruminants have a strong dependency on the endogenous glucose produced by gluconeogenesis, so exogenous glucose intake is relatively low. The RPG supplement can change energy supply path, improve energy utilization efficiency, and alleviate negative energy balance (9–11). Zhang et al. (12) also reported that RPG enhanced the large intestine fermentation function and mucosal immunity of transition dairy cows. Taurine (TAU) is a sulfur-containing amino acid that plays an important role in muscle growth, immune response and energy metabolism (13, 14). However, the TAU synthesized in the liver of most mammals has a limited ability to remove free radicals, while supplementation with exogenous TAU improved the antioxidant enzyme activity of animals and their liver tissues, thereby enhancing antioxidant capacity (15, 16).
Our previous study uncovered that supplementing with RPG and TAU reduced weight loss in yaks during transportation. However, the optimal addition of both supplements to effectively alleviate transport stress in yaks remains unclear. Our study aimed to investigate: (1) the effects of four supplemental levels on the weight of yaks before and after transportation; (2) the changes of plasma antioxidant, immune and stress response indicators before and after transportation; and (3) the impacts of continuous supplementation of RPG and TAU on the above indicators in yaks. We hypothesized that one of the four additive combinations of RPG and TAU could minimize the adverse effects of transportation on growth performance in yaks, and that sustained treatment could improve their antioxidant capacity, immunity and oxidative stress status. This study provides evidence for the use of nutritional intervention to alleviate the stress of yaks during transportation.
2 Materials and methods
2.1 Animals and experimental design
All animal procedures adhered to the Chinese Guidelines for Animal Welfare, and the study protocols were approved by the Experimental Animal Committee of the Animal Nutrition Institute at Sichuan Agricultural University (Approval no.: SCAUAC2019-36).
The study was conducted from January to March 2023. Thirty-two healthy male yaks, aged 3-years; and weighting 172.5 ± 10.2 kg, were assigned randomly into 4 different treatments: (1) low RPG and low TAU (LRLT), the level of RPG in the diet was 1%, and the addition of TAU was 5 g/d; (2) low RPG and high TAU (LRHT), the level of RPG in the diet was 1%, and the addition of TAU was 15 g/d; (3) high RPG and low TAU (HRLT), the level of RPG in the diet was 3%, and the addition of TAU was 5 g/d; and (4) high RPG and high TAU (HRHT), the level of RPG in the diet was 3%, and the addition of TAU was 15 g/d. Ingredients and chemical composition of diets offered to yaks are shown in Table 1. The RPG and TAU used in our study were purchased from Shanghai Meinong Biotechnology Co., Shanghai, China, and Qingdao Kailide Biotechnology Co., Ltd., Qingdao, China, respectively. Both had active ingredients at 50% and a small intestine release rate of 90%. Each treatment involved eight replicates of one yak each replicate. Yaks were housed individually in pens within a pasture in Hongyuan County, Sichuan Province, and were fed a total mixed ration (TMR) twice a day (0700 and 1900 h) during the trial, with a guaranteed daily feed intake of 105–110%. The feed was pushed at least 10 times daily, and yaks had unrestricted access to fresh water throughout the study period. After 7 days on different diets, the yaks were treated for 9 h of long-distance transport. The yaks were fasted for 12 h prior to transportation and loaded into a semi-trailer measuring (9 m × 2.55 m × 4 m) at 10:00 AM on the day of transport, and then transported for 9 h on expressways until they arrived at the Research Farm of Animal Nutrition Institute, Sichuan Agricultural University (Yaan, China). Fasting and water prohibition during transportation, and no feed and fresh water are also provided to yaks within 1 h after transportation. The yaks were eventually raised here for 30 days during which they were fed the same diet as prior to their transportation.
2.2 Determination of growth performance
Yaks were weighed both before transport and on days 0 and 30 post-transport to analyze changes in the body weight (BW) of the experimental yaks. The BW loss percent of yaks during transportation was calculated using the formula: BW loss percent (%) = [(BW before transport – BW after transport)/ BW before transport] × 100%. From day 0 post-transport to day 30, daily feed intake was measured to determine dry matter intake (DMI). The yaks were weighed again on d 30, and the average daily gain (ADG) and feed conversion ratio (FCR) were calculated.
2.3 Sample collection
Blood samples were collected using vacuum blood collection tubes from the jugular vein of yaks 3 h after morning feeding before transport and on d 0, 10 and 30 after transport. Blood samples were allowed to stand for 30 min and then centrifuged at 4°C and 3,000 × g for 15 min to prepare plasma. The plasma was stored at-20°C for subsequent analysis of biochemical, antioxidant, immune, and stress response indicators.
2.4 Determination of biochemical, antioxidant, immune and stress response indicators in plasma
Plasma biochemical, including total cholesterol (TC), total protein (TP), alanine aminotransferase (ALT), lactate (LAC), triglyceride (TG), glucose (GLU), lactate dehydrogenase (LDH) and creatine kinase (CK) were determined using a fully automatic bio-chemical analyzer (Hitachi 3,100, Olympus Optical, Tokyo, Japan). Plasma antioxidant indexes, including activities of catalase (CAT, A007-1-1), superoxide dismutase (SOD, A001-1-2), and glutathione peroxidase (GSH-PX, A005-1-2), as well as total antioxidant capacity (T-AOC, A015-1-2) and malondialdehyde (MDA, A003-1-2) concentrations, were evaluated with an antioxidant assay kit supplied by the Nanjing Jiancheng Bioengineering Institute (Nanjing, China). Plasma immune indexes, like IL-1β (MB-9650A), IL-4 (MB-4904A), IL-6 (MB-4905A), IL-10 (MB-4931A), TNF-α (MB-4838A) concentrations, were determined using an ELISA kit from Jiangsu Enzymebiao Bio-technology Co., Ltd. (Nanjing, China), following the manufacturer’s instructions. For plasma stress response indicators, concentrations of cortisol (COR, EB0062) and lipopolysaccharides (LPS, EU3126) were tested by ELISA kit from FineTest, Wuhan Fine Biotech Co. (Wuhan, China).
2.5 Statistical analysis
Before comparing treatments, the normality and variance homogeneity of all data were verified. Growth performance, plasma antioxidant, immune and stress response indicators of yaks were subjected to two-way ANOVA by the SPSS statistical software version 22.0 (IBM SPSS, Chicago, IL, USA) to determine the effects of RPG and TAU supplemental levels on the above indexes. When the interaction between RPG and TAU levels in diets was significant, one-way ANOVA was conducted to compare two levels of TAU supplementation within the same RPG levels and two levels of RPG in the diet within the same TAU supplementation. In addition, we also compared the changes of BW, plasma biochemistry, antioxidant, immune and stress response indicators of yaks before and after transport using ANOVA by the SPSS statistical software version 22.0 (IBM SPSS, Chicago, IL, USA). All results are reported as least squares means ± SEM. The Tukey multiple comparison test was employed to identify statistically significant differences at p ≤ 0.05.
3 Results
3.1 Weight changes in yaks before and after transportation
The effects of dietary RPG level and TAU supplementation on the changes of yak BW before and after transportation are shown in Table 2. There was no significant interaction between RPG and TAU regarding the weight of yaks pre-and post-transport, weight loss as well as weight loss percent (P > 0.05). Compared with before transportation, the weight of yaks decreased after transportation (P < 0.01). Increasing TAU supplementation reduced weight loss (p = 0.05, 11.9 and 8.42 kg in LT and HT, respectively). The weight loss percent also decreased (p = 0.04) with the increase of TAU supplementation.
3.2 Growth performance in yaks after transportation
The effects of dietary RPG level and TAU supplementation on yak growth performance from days 0 to 30 post-transportation are presented in Table 3. The level of RPG in the diet and TAU supplementation did not affect final BW, ADG, DMI and FCR in yaks (P > 0.05).
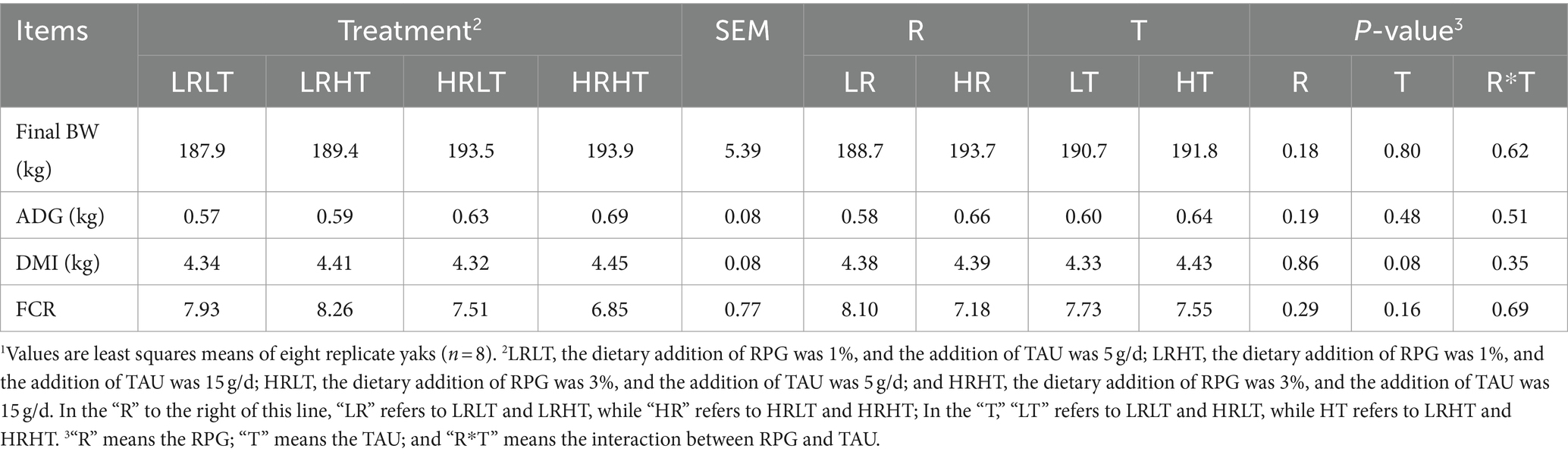
Table 3. Effects of RPG and TAU levels in the diet on the growth performance of yaks1 0–30 d after transportation.
3.3 Changes of biochemical, antioxidant, immune and stress response indicators in plasma before and after transportation
We did not observe any changes in the concentrations (P > 0.05) of ALT, LAC, TG, and GLU in plasma before and after transport or among different treatments (Table 4). Compared with before transport, the concentration of TC, TP, LDH and CK in plasma of yaks with different treatments increased after transport (P < 0.01). The plasma TP concentration before transport was higher in HRHT than in LRLT (p = 0.04). The changes of antioxidant, immune and stress response indicators in plasma before and after transportation are provided in Tables 5–7. Transport increased plasma concentrations of MDA (p = 0.05), COR (P < 0.01) and LPS (P < 0.01), while decreased plasma IL-10 concentration (P < 0.01). Increasing TAU supplementation increased plasma GSH-PX activity before (p = 0.02) and after transportation (p = 0.03). The plasma MDA concentrations after transport was lower in HRHT than in LRLT (p = 0.03, 15.1 vs. 16.7 nmol/mL). The plasma IL-4 concentration after transport was higher in HRHT than in LRLT (p = 0.03). The plasma IL-10 concentration after transport was higher in LRHT and HRHT than in LRLT and HRLT (p = 0.02). In addition, increasing TAU supplementation decreased the plasma concentration of COR before transportation (p = 0.02) as well as LPS before (p = 0.05) and after transportation (p = 0.04). The plasma COR concentration after transport was lower in LRHT and HRHT than in LRLT (p = 0.03).
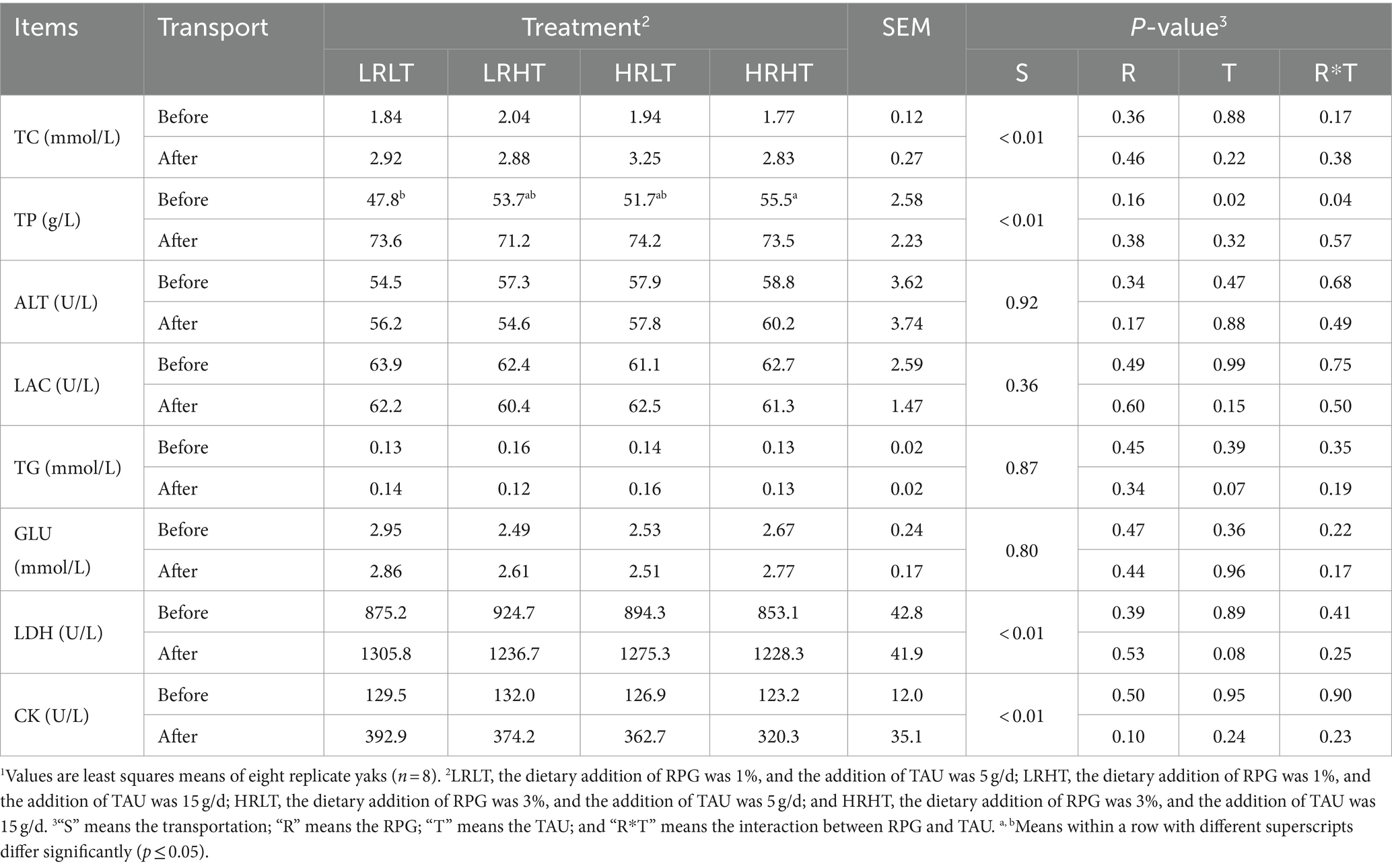
Table 4. Changes in plasma biochemical of yaks1 before and after transport under various treatments.
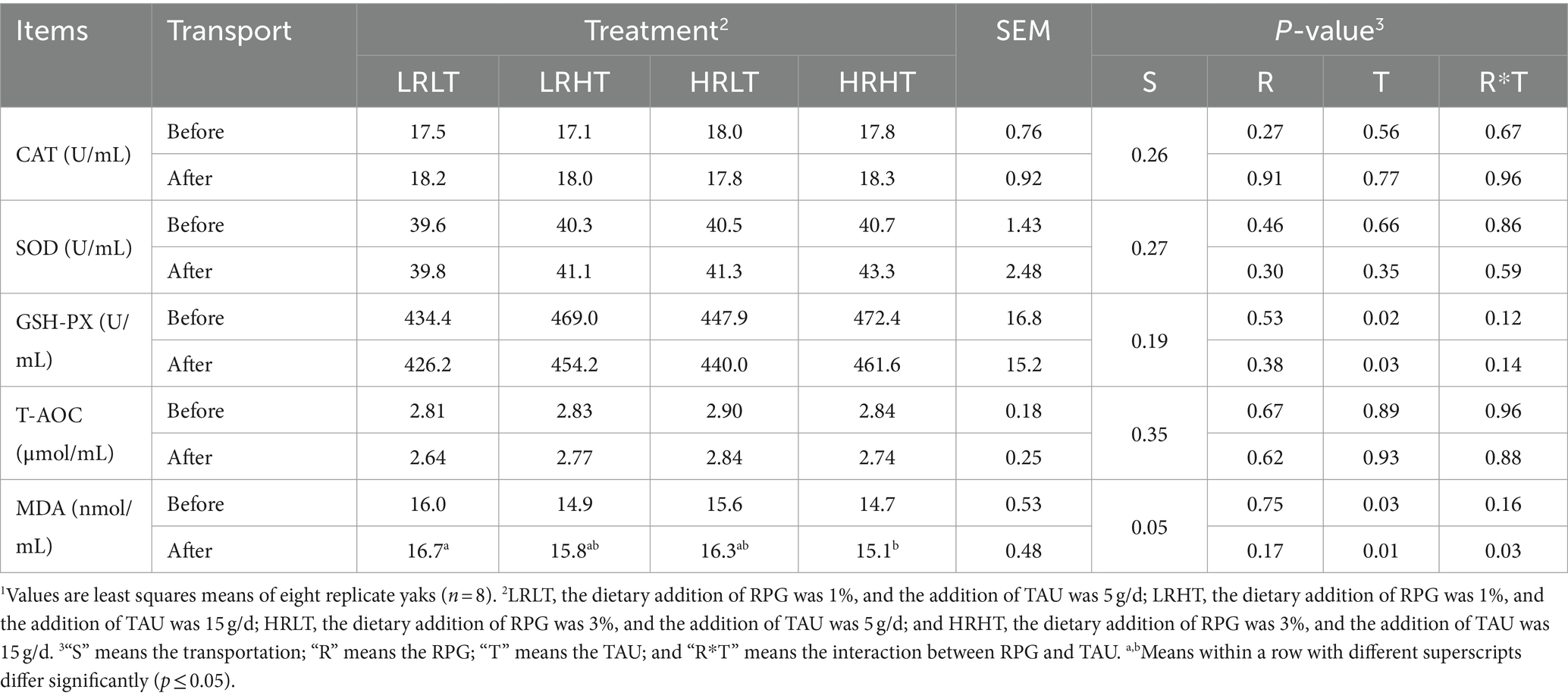
Table 5. Changes in plasma antioxidant capacity of yaks1 before and after transport under various treatments.
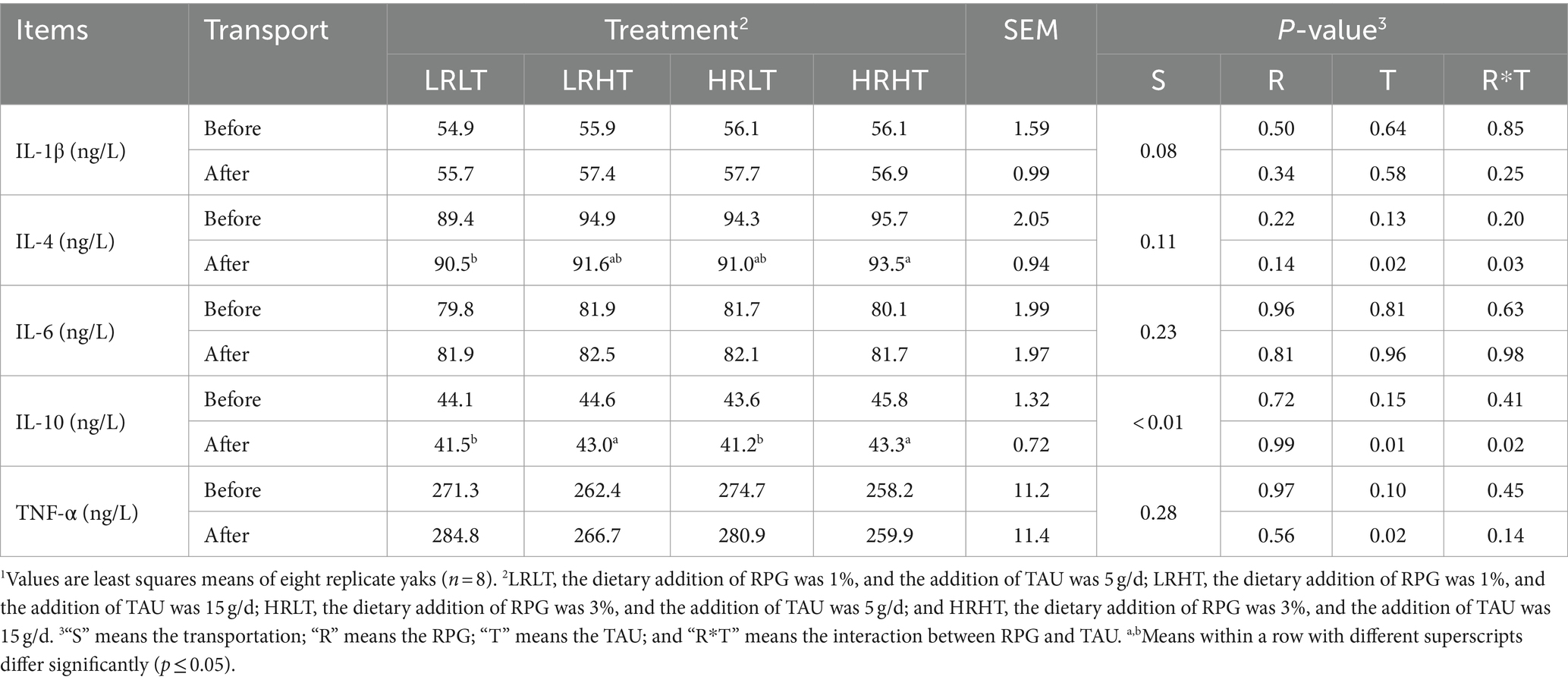
Table 6. Changes in plasma immune index of yaks1 before and after transport under various treatments.
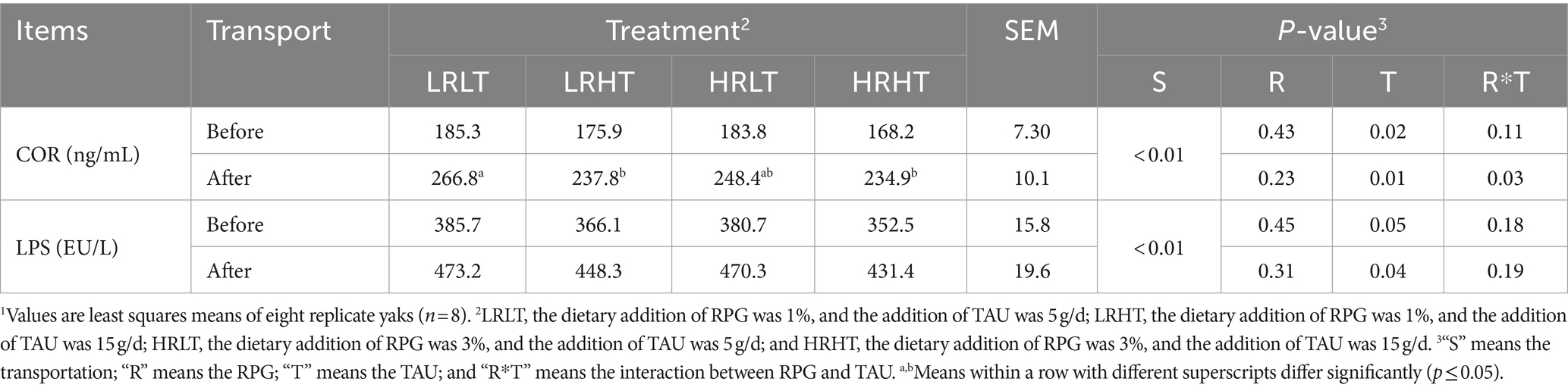
Table 7. Changes in plasma stress response indicators of yaks1 before and after transport under various treatments.
3.4 Plasma antioxidant in yaks after transportation
We found no significant changes (p > 0.05) in the activities of CAT and SOD, T-AOC as well as MDA concentrations in plasma on days 10, and 30 post-transport across treatments (Table 8). The TAU (p = 0.03) and the interaction between RPG and TAU (p = 0.04) had significant effects on plasma GSH-PX activities at day 10 d after transport, which was lower in HRHT than in LRLT.
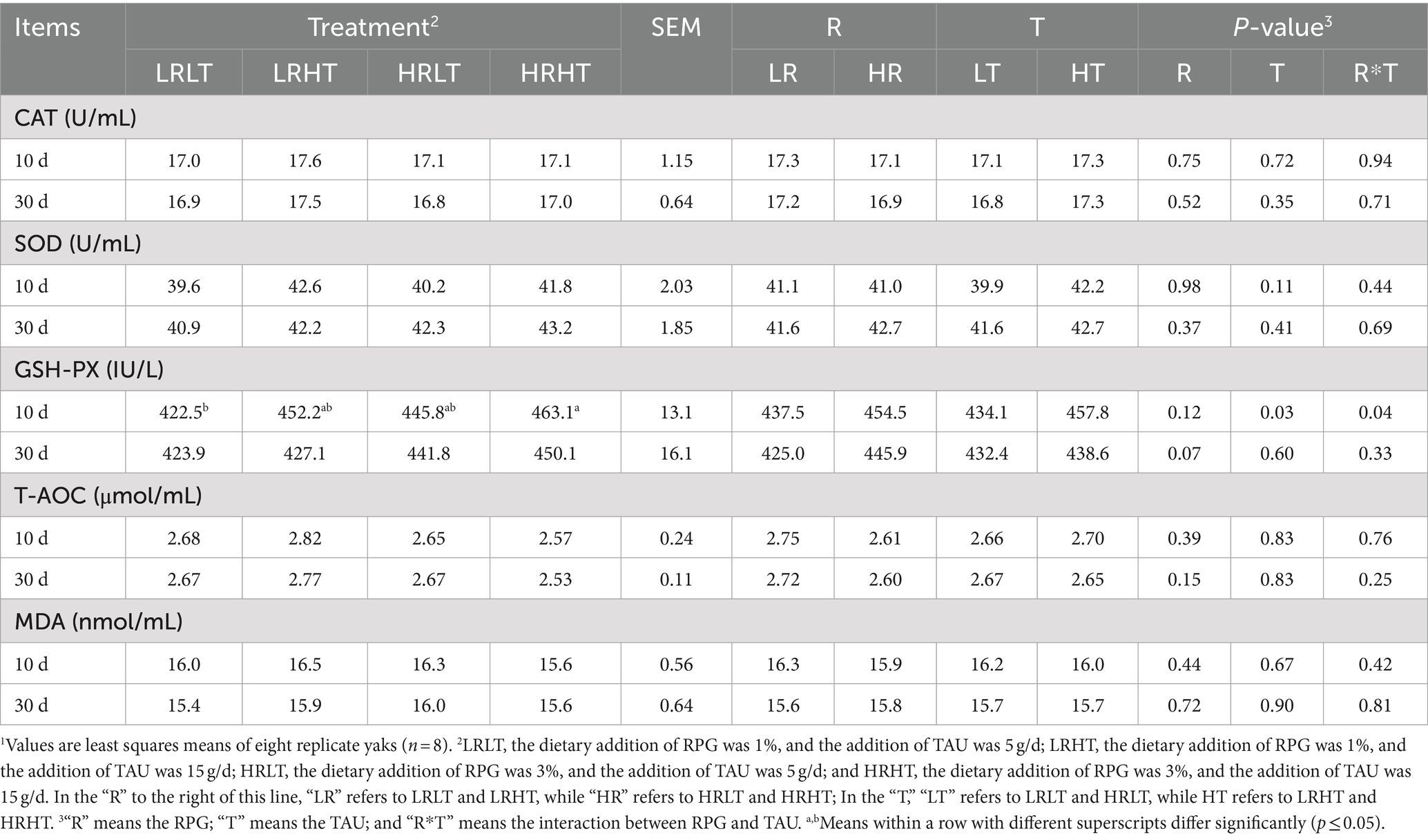
Table 8. Effects of RPG and TAU levels in the diet on the plasma antioxidant capacity of yaks1 after transportation.
3.5 Plasma immune in yaks after transportation
Table 9 shows the effects of dietary RPG level and TAU supplementation on the plasma immune index of yak after transportation. Increasing TAU supplementation decreased plasma IL-1β concentrations at day 30 after transport (p = 0.02), while increased plasma IL-4 concentrations at day 10 after transport (p = 0.02). Besides that, plasma immune index did not differ at all treatments (P > 0.05).
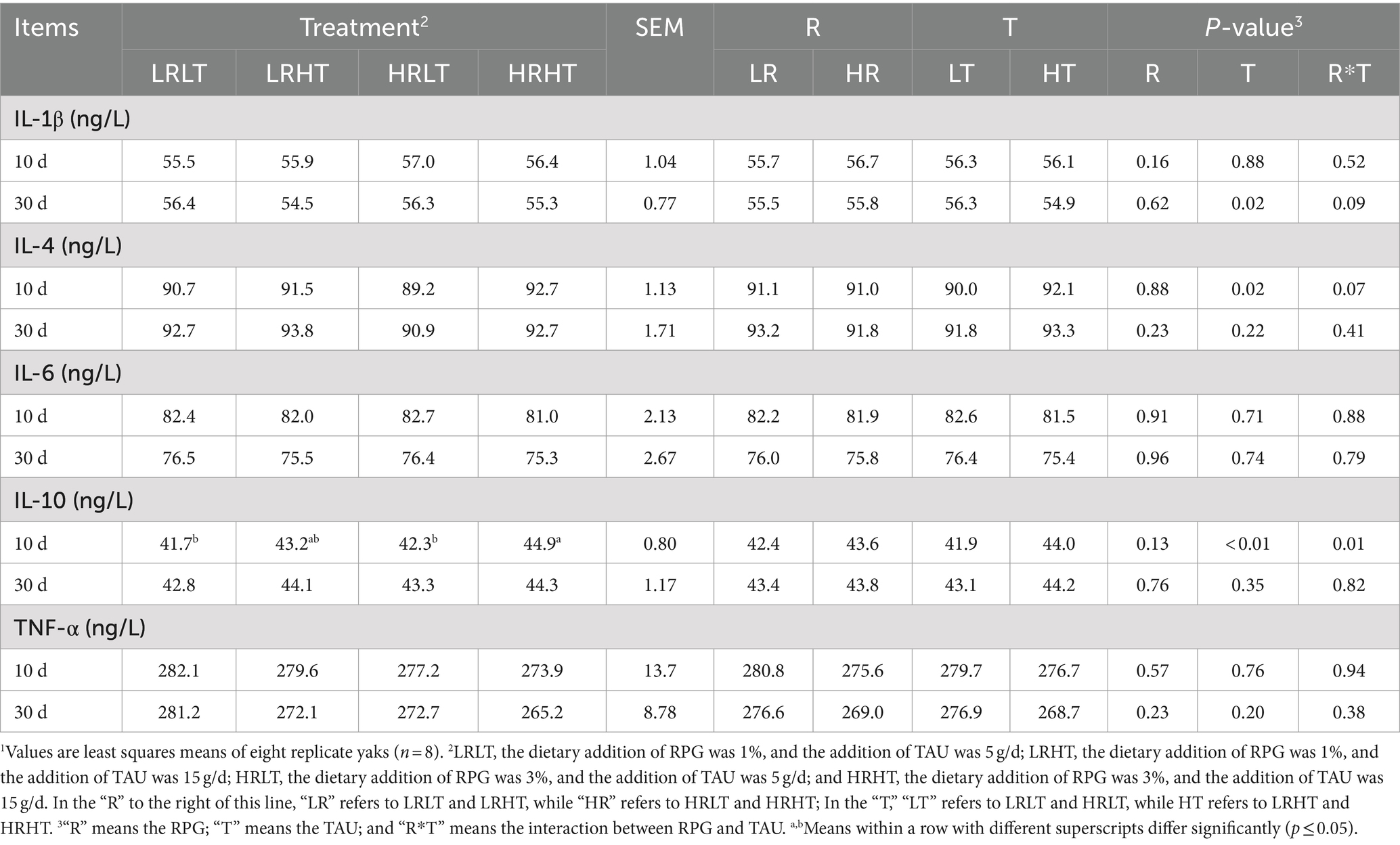
Table 9. Effects of RPG and TAU levels in the diet on the plasma immune index of yaks1 after transportation.
3.6 Plasma stress response indicators in yaks after transportation
As shown in Table 10, the RPG and TAU did not change the plasma LPS concentration of yaks after transport (P > 0.05). However, the plasma COR concentration in yaks decreased at 10 (p = 0.03) and 30 (p = 0.05) days after transport with the increase of TAU addition.
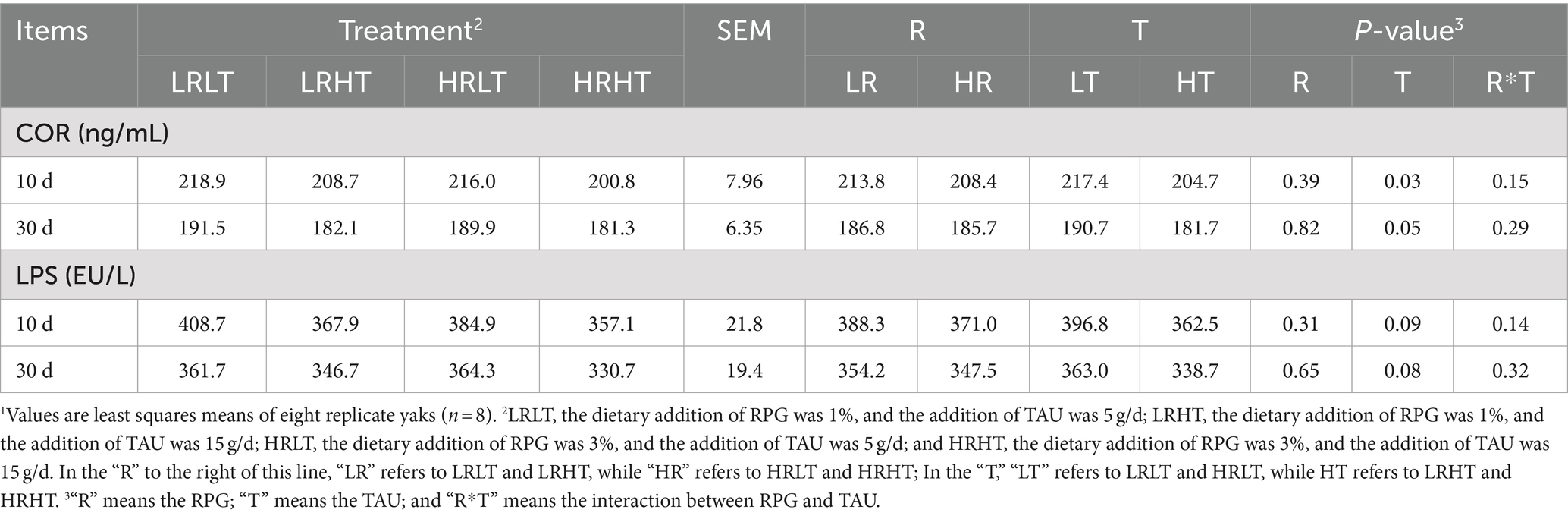
Table 10. Effects of RPG and TAU levels in the diet on the plasma stress response indicators of yaks1 after transportation.
4 Discussion
Harsh conditions during transportation, like noise and overcrowding, can induce oxidative stress in animals, resulting in weight loss. Lendrawati et al. (17) reported that transport reduces the weight of sheep, and the weight loss increases with the longer the transport time. González et al. (18) reported that while rearing patterns, age, transportation time, and truck facilities all affect the weight of cattle transported long distances, these tests all show a decrease in weight. In our study, all yaks experienced weight loss after 9 h of long-distance transport, and even supplementation with high levels of RPG and TAU could not reverse this outcome. However, yaks fed high supplemental TAU had lower weight loss (8.42 vs. 11.9 kg) and lower weight loss percent (4.83 vs. 6.87%), which was closely related to the biological function of TAU. Mice with inadequate TAU intake reportedly exhibited low body weight, slower responses to stimuli, and exercise intolerance, indicating that TAU was crucial for maintaining body weight and muscle endurance (19). Kim et al. (20) demonstrated the fatigue resistance of TAU following acute exercise, which were attributed to increased oxygen uptake and enhanced lipid oxidation in a mouse model. Taurine is also a promoter of energy metabolic balance (13) and may help increase energy supply of yak during transport. In addition, feeding TAU can improve the performance of animals (16, 21). However, we found that the growth performance of yaks within 30 days after transport did not change due to the increase in TAU and RPG. One possible reason is that this study focused more on the dosage of RPG and TAU rather than whether they were added, which may limit the comparison of this work with other studies and will be refined in future work.
Plasma TP is clinically significant for assessing liver synthesis function, nutritional status, and water balance. Mufarrej et al. (22) observed that transport increased the levels of TP and albumin in plasma of lambs. In our study, long-term transport may promote the fluid loss in yaks, causing blood to become concentrated and plasma TP levels to rise. Plasma TC concentration is commonly used to evaluate lipid metabolism and diagnose certain diseases (23, 24). We observed an increase in plasma TC concentration post-transport, likely due to the damage and dysfunction of cell membranes caused by lipid peroxidation, which affects the metabolism of lipoproteins and thus indirectly affects the TC in plasma. Plasma levels of LDH and CK are considered biomarkers of muscle injury (25, 26). Fang et al. (27) reported that transport induced an increase in plasma LDH concentration and changes in other blood indicators, suggesting the occurrence of oxidative stress. Alcalde et al. (28) also reported that road transportation, irrespective of the duration, consistently increased plasma CK levels in suckling goat kids, suggesting significant stress and muscle damage. Similarly, we observed elevated levels of LDH and CK in yak plasma post-transport, indicating potential health risks for yaks.
Stress during transport negatively affects the antioxidant system in animals, resulting in reduced antioxidant enzyme activity, which hinders the effective removal of free radicals and peroxide products from the body (29, 30). MDA is the end product of lipid peroxidation, shows a positive correlation between its plasma concentration and the severity of oxidative stress (31). Transport leads to increased plasma MDA concentration, indicating the onset and progression of oxidative stress (32, 33), which is also supported by our study findings. We observed that increasing TAU levels reduced plasma MDA concentration in yaks after transport, mitigating transport stress. Han et al. (16) found that TAU alleviates oxidative damage by increasing gene expression of NRF2, GPX and HO-1. Seidel et al. (34) also found that TAU reduces cellular stress levels, possibly by upregulating iron-related proteins like iron storage protein, thereby inhibiting lipid peroxidation. Therefore, we speculated that increasing TAU levels alleviated transport stress in yaks by inhibiting lipid peroxidation, and the specific mechanism needs to be further explored. GSH-PX is crucial for cellular antioxidant defense as it eliminates ROS from the body. Han et al. (35) indicated that TAU supplementation increased the glutathione contents and the GSH-PX activity, alleviating the oxidative stress induced by LPS. In our study, at 0 and 10 days after transport, increased TAU supplementation elevated plasma GSH-PX activity, implying an enhanced antioxidant capacity in yaks. It is to be noted that the plasma antioxidant capacity differences among treatments diminished over time after transport, possibly because the antioxidant function of TAU was activated in majority cases when exposed to oxidative stress risk.
During oxidative stress, the body generates a surplus of ROS, which activate the inflammasome and trigger the release of numerous pro-inflammatory cytokines, leading to cellular damage (36). Transport increased the concentration of proinflammatory cytokines like TNF-α and IL-1β in animal plasma (37, 38). However, in our study, transport did not change the concentration of IL-1β, IL-6 and TNF-α, while decreased the concentration of anti-inflammatory factors like IL-10, which also means that the health status of yaks may be potentially damaged. In addition, we found that increasing TAU supplementation decreased plasma IL-1β concentrations at 30 day after transport and TNF-α concentrations at 0 day after transport. TAU has been demonstrated to mitigates the adverse effects of LPS by dampening pro-inflammatory responses and oxidative stress (39). Zheng et al. (40) reported that TAU deficiency aggravated the DSS induced inflammatory model in mice, while TAU supplementation reduced proinflammatory factors levels like TNF-α and IL-6 by inhibiting the TLR4/NF-κB pathway. Han et al. (35) also indicated that TAU supplementation decreased the expression of IL-1β, TNF-α, and IL-6 genes, thereby resisting the LPS-induced inflammatory response in broilers. The release of pro-inflammatory cytokines accompanies the onset of inflammation, and over time, the pathogen is cleared by the body, and anti-inflammatory cytokines then come into play to repair the damaged tissue (41). TAU has a positive effect on the formation of the immune system of body (42). Zhou et al. (43) reported that TAU increases IL-10 concentration in weaned piglets and enhances immunity against weaning stress and inflammation. Elevated plasma concentrations of IL-4 and IL-10 in yaks were observed following transport when RPG and TAU were combined at high levels, indicating enhanced immunity, yet these levels stabilized after 30 days of feeding. It is likely that TAU exerts an anti-inflammatory effect during transport stress occurs, but the influence wanes when transport stress diminishes or even disappears.
The COR is an important glucocorticoid, and its concentrations serve as a crucial index for assessing stress responses, with their dynamic changes being closely tied to stress progression (44). Lee et al. (45) reported that road transport increased the plasma COR concentration of dairy cows. In our study, the concentrations of COR and LPS in yak plasma increased after transport, indicating the occurrence of transport stress. We also observed that the increase of TAU supplementation decreased the COR concentrations in yaks throughout the experiment, which suggested that TAU could mitigate transport stress in yaks, with a lasting beneficial effect (30 d). LPS stimulates the immune system, thereby triggering inflammation. He et al. (46) reported that transport impaired morphology and tissue in jejunum, induced stress responses, and elevated LPS concentrations. In our study, the LPS concentration of yaks decreased before and after transport with the increase of TAU addition, which was beneficial to the health of yaks after transport.
5 Conclusion
Transport induces oxidative stress in yaks, which can lead to reduced body weight and health impairment. TAU mitigates the adverse effects of transport on yak weight. Increasing TAU levels enhanced antioxidant capacity and immunity of yaks after transport, alleviating their oxidative stress, but this beneficial effect was weakened with time after transport. RPG had less effect on transport stress than TAU. Considering both cost and efficacy, the recommended dietary inclusion of RPG for yaks is 1%, with a TAU supplement of 15 g/d to mitigate transport stress. This work presents an effective strategy for mitigating transport stress, which is of great significance for off-site fattening of grazing yaks in the cold season.
Data availability statement
The raw data supporting the conclusions of this article will be made available by the authors, without undue reservation.
Ethics statement
The animal study was approved by the Experimental Animal Committee of the Animal Nutrition Institute at Sichuan Agricultural University. The study was conducted in accordance with the local legislation and institutional requirements.
Author contributions
XW: Conceptualization, Data curation, Formal analysis, Investigation, Methodology, Supervision, Writing – original draft, Writing – review & editing. KZ: Formal analysis, Investigation, Methodology, Supervision, Writing – review & editing. SZ: Supervision, Writing – review & editing. JZ: Supervision, Writing – review & editing. MC: Supervision, Writing – review & editing. LL: Supervision, Writing – review & editing. YC: Supervision, Writing – review & editing. HY: Supervision, Writing – review & editing. BZ: Supervision, Writing – review & editing. CS: Supervision, Writing – review & editing. YZ: Supervision, Writing – review & editing. RT: Supervision, Writing – review & editing. BX: Methodology, Project administration, Resources, Supervision, Validation, Writing – review & editing.
Funding
The author(s) declare that financial support was received for the research, authorship, and/or publication of this article. This study was supported by the National Key R&D Program of China (grant no: 2021YFD1600202).
Acknowledgments
The authors thank Shanghai Meinong Biotechnology Co., (Shanghai, China) for providing the rumen-protected glucose used in this study.
Conflict of interest
The authors declare that the research was conducted in the absence of any commercial or financial relationships that could be construed as a potential conflict of interest.
Publisher’s note
All claims expressed in this article are solely those of the authors and do not necessarily represent those of their affiliated organizations, or those of the publisher, the editors and the reviewers. Any product that may be evaluated in this article, or claim that may be made by its manufacturer, is not guaranteed or endorsed by the publisher.
References
1. Liu, H, Jiang, H, Hao, L, Cao, X, Degen, A, Zhou, J, et al. Rumen bacterial community of grazing lactating yaks (Poephagus grunniens) supplemented with concentrate feed and/or rumen-protected lysine and methionine. Animals. (2021) 11:2425. doi: 10.3390/ani11082425
2. Xu, T, Xu, S, Hu, L, Zhao, N, Liu, Z, Ma, L, et al. Effect of dietary types on feed intakes, growth performance and economic benefit in Tibetan sheep and yaks on the Qinghai-Tibet plateau during cold season. PLoS One. (2017) 12:e0169187. doi: 10.1371/journal.pone.0169187
3. Zhang, L, Wang, Z, Zhou, P, Fu, L, Zhang, L, Xu, C, et al. Vitamin E supplementation improves post-transportation systemic antioxidant capacity in yak. PLoS One. (2022) 17:e0278660. doi: 10.1371/journal.pone.0278660
4. Minka, NS, and Ayo, JO. Physiological responses of food animals to road transportation stress. Afr J Biotechnol. (2009) 8:849. doi: 10.5555/20103333849
5. Nwunuji, TP, Mayowa, OO, Yusoff, SM, Bejo, SK, Salisi, S, and Mohd, EAW. The ameliorative effect of ascorbic acid on the oxidative status, live weight and recovery rate in road transport stressed goats in a hot humid tropical environment. Anim Sci J. (2014) 85:611–6. doi: 10.1111/asj.12174
6. Parker, AJ, Dobson, GP, and Fitzpatrick, LA. Physiological and metabolic effects of prophylactic treatment with the osmolytes glycerol and betaine on Bos indicus steers during long duration transportation. J Anim Sci. (2007) 85:2916–23. doi: 10.2527/jas.2006-193
7. Alfaro, GF, Novak, TE, Rodning, SP, and Moisá, SJ. Preconditioning beef cattle for long-duration transportation stress with rumen-protected methionine supplementation: a nutrigenetics study. PLoS One. (2020) 15:e0235481. doi: 10.1371/journal.pone.0235481
8. Zhao, H, Tang, X, Wu, M, Li, Q, Yi, X, Liu, S, et al. Transcriptome characterization of short distance transport stress in beef cattle blood. Front Genet. (2021) 12:616388. doi: 10.3389/fgene.2021.616388
9. Wang, YP, Cai, M, Hua, DK, Zhang, F, Jiang, LS, Zhao, YG, et al. Metabolomics reveals effects of rumen-protected glucose on metabolism of dairy cows in early lactation. Anim Feed Sci Technol. (2020) 269:114620. doi: 10.1016/j.anifeedsci.2020.114620
10. McCarthy, CS, Dooley, BC, Branstad, EH, Kramer, AJ, Horst, EA, Mayorga, EJ, et al. Energetic metabolism, milk production, and inflammatory response of transition dairy cows fed rumen-protected glucose. J Dairy Sci. (2020) 103:7451–61. doi: 10.3168/jds.2020-18151
11. Lohrenz, A, Duske, K, Schneider, F, Nürnberg, K, Losand, B, Seyfert, HM, et al. Milk performance and glucose metabolism in dairy cows fed rumen-protected fat during mid lactation. J Dairy Sci. (2010) 93:5867–76. doi: 10.3168/jds.2010-3342
12. Zhang, X, Li, X, Wu, J, Jiao, J, He, Z, Tan, Z, et al. Rumen-protected glucose supplementation in transition dairy cows shifts fermentation patterns and enhances mucosal immunity. Anim Nutr. (2021) 7:1182–8. doi: 10.1016/j.aninu.2021.08.002
13. Wen, C, Li, F, Zhang, L, Duan, Y, Guo, Q, Wang, W, et al. Taurine is involved in energy metabolism in muscles, adipose tissue, and the liver. Mol Nutr Food Res. (2019) 63:e1800536. doi: 10.1002/mnfr.201800536
14. Izquierdo, JM. Taurine as a possible therapy for immunosenescence and inflammaging. Cell Mol Immunol. (2024) 21:3–5. doi: 10.1038/s41423-023-01062-5
15. Surai, PF, Earle-Payne, K, and Kidd, MT. Taurine as a natural antioxidant: from direct antioxidant effects to protective action in various toxicological models. Antioxidants. (2021) 10:1876. doi: 10.3390/antiox10121876
16. Han, HL, Zhang, JF, Yan, EF, Shen, MM, Wu, JM, Gan, ZD, et al. Effects of taurine on growth performance, antioxidant capacity, and lipid metabolism in broiler chickens. Poult Sci. (2020) 99:5707–17. doi: 10.1016/j.psj.2020.07.020
17. Lendrawati, L, Priyanto, R, Jayanegara, A, Manalu, W, and Desrial, D. Effect of different transportation period on body weight loss, hematological and biochemical stress responses of sheep. J Indones Trop Anim Agric. (2020) 45:115–23. doi: 10.14710/jitaa.45.2.115-123
18. González, LA, Schwartzkopf-Genswein, KS, Bryan, M, Silasi, R, and Brown, F. Factors affecting body weight loss during commercial long haul transport of cattle in North America. J Anim Sci. (2012) 90:3630–9. doi: 10.2527/jas.2011-4786
19. Watanabe, M, Ito, T, and Fukuda, A. Effects of taurine depletion on body weight and mouse behavior during development. Meta. (2022) 12:631. doi: 10.3390/metabo12070631
20. Kim, J, Beak, S, Ahn, S, Moon, BS, Kim, BS, Lee, SJ, et al. Effects of taurine and ginseng extracts on energy metabolism during exercise and their anti-fatigue properties in mice. Nutr Res Pract. (2022) 16:33–45. doi: 10.4162/nrp.2022.16.1.33
21. Lin, Y, and Lu, R. Dietary taurine supplementation enhances growth and nutrient digestibility in giant grouper Epinephelus lanceolatus fed a diet with soybean meal. Aquac Rep. (2020) 18:100464. doi: 10.1016/j.aqrep.2020.100464
22. Mufarrej, SA, Haidary, IA, Kraidees, MA, Hussein, MF, and Metwally, HM. Effect of chromium dietary supplementation on the immune response and some blood biochemical parameters of transport-stressed lambs. Asian Australas J Anim Sci. (2008) 21:671–6. doi: 10.5713/ajas.2008.70135
23. Cabiati, M, Fontanini, M, Giacomarra, M, Politano, G, Randazzo, E, Peroni, D, et al. Screening and identification of putative long non-coding RNA in childhood obesity: evaluation of their transcriptional levels. Biomedicines. (2022) 10:529. doi: 10.3390/biomedicines10030529
24. Liao, F, He, W, Jiang, C, Yin, C, Guo, G, Chen, X, et al. A high LDL-C to HDL-C ratio predicts poor prognosis for initially metastatic colorectal cancer patients with elevations in LDL-C. Onco Targets Ther. (2015) 8:3135–42. doi: 10.2147/OTT.S90479
25. Xu, C, Lv, J, You, S, Zhao, Q, Chen, X, and Hu, X. Supplementation with oat protein ameliorates exercise-induced fatigue in mice. Food Funct. (2013) 4:303–9. doi: 10.1039/C2FO30255A
26. Brancaccio, P, Lippi, G, and Maffulli, N. Biochemical markers of muscular damage. Clin Chem Lab Med. (2010) 48:757–67. doi: 10.1515/CCLM.2010.179
27. Fang, D, Mei, J, Xie, J, and Qiu, W. The effects of transport stress (temperature and vibration) on blood biochemical parameters, oxidative stress, and gill histomorphology of pearl gentian groupers. Aust Fish. (2023) 8:218. doi: 10.3390/fishes8040218
28. Alcalde, MJ, Suárez, MD, Rodero, E, Álvarez, R, Sáez, MI, and Martínez, TF. Effects of farm management practices and transport duration on stress response and meat quality traits of suckling goat kids. Animal. (2017) 11:1626–35. doi: 10.1017/S1751731116002858
29. Xia, C, Duan, C, Chen, C, Yang, X, Zhang, Y, Liu, Y, et al. The effects of electrolytic multivitamins and neomycin on antioxidant capacity and intestinal damage in transported lambs. Animals. (2024) 14:824. doi: 10.3390/ani14060824
30. Bi, C, Yin, J, Yang, W, Shi, B, and Shan, A. Effects of dietary γ-aminobutyric acid supplementation on antioxidant status, blood hormones and meat quality in growing-finishing pigs undergoing transport stress. J Anim Physiol Anim Nutr. (2020) 104:590–6. doi: 10.1111/jpn.13280
31. Shi, F, Ma, C, Ji, C, Li, M, Liu, X, and Han, Y. Serum lipid oxidative stress products as risk factors are the candidate predictive biomarkers for human abdominal aortic aneurysms. Clin Appl Thromb Hemost. (2020) 26:1076029620932226. doi: 10.1177/1076029620932226
32. Tozlu Çelik, H, Aslan, FA, Us Altay, D, Kahveci, ME, Konanç, K, Noyan, T, et al. Effects of transport and altitude on hormones and oxidative stress parameters in sheep. PLoS One. (2021) 16:e0244911. doi: 10.1371/journal.pone.0244911
33. Zou, Y, Xiang, Q, Wang, J, Wei, H, and Peng, J. Effects of oregano essential oil or quercetin supplementation on body weight loss, carcass characteristics, meat quality and antioxidant status in finishing pigs under transport stress. Livest Sci. (2016) 192:33–8. doi: 10.1016/j.livsci.2016.08.005
34. Seidel, U, Lüersen, K, Huebbe, P, and Rimbach, G. Taurine enhances iron-related proteins and reduces lipid peroxidation in differentiated C2C12 myotubes. Antioxidants. (2020) 9:1071. doi: 10.3390/antiox9111071
35. Han, H, Zhang, J, Chen, Y, Shen, M, Yan, E, Wei, C, et al. Dietary taurine supplementation attenuates lipopolysaccharide-induced inflammatory responses and oxidative stress of broiler chickens at an early age. J Anim Sci. (2020) 98:skaa311. doi: 10.1093/jas/skaa311
36. Glorieux, C, Sandoval, JM, Fattaccioli, A, Dejeans, N, Garbe, JC, Dieu, M, et al. Chromatin remodeling regulates catalase expression during cancer cells adaptation to chronic oxidative stress. Free Radic Biol Med. (2016) 99:436–50. doi: 10.1016/j.freeradbiomed.2016.08.031
37. Masmeijer, C, Devriendt, B, Rogge, T, van Leenen, K, De Cremer, L, Van Ranst, B, et al. Randomized field trial on the effects of body weight and short transport on stress and immune variables in 2-to 4-week-old dairy calves. J Vet Intern Med. (2019) 33:1514–29. doi: 10.1111/jvim.15482
38. Ren, Y, Men, X, Yu, Y, Li, B, Zhou, Y, and Zhao, C. Effects of transportation stress on antioxidation, immunity capacity and hypoxia tolerance of rainbow trout (Oncorhynchus mykiss). Aquac Rep. (2022) 22:100940. doi: 10.1016/j.aqrep.2021.100940
39. Liu, Y, Li, F, Zhang, L, Wu, J, Wang, Y, and Yu, H. Taurine alleviates lipopolysaccharide-induced liver injury by anti-inflammation and antioxidants in rats. Mol Med Rep. (2017) 16:6512–7. doi: 10.3892/mmr.2017.7414
40. Zheng, J, Zhang, J, Zhou, Y, Zhang, D, Guo, H, Li, B, et al. Taurine alleviates experimental colitis by enhancing intestinal barrier function and inhibiting inflammatory response through TLR4/NF-κB signaling. J Agric Food Chem. (2024) 72:12119–29. doi: 10.1021/acs.jafc.4c00662
41. Zong, Y, Chen, T, Dong, H, Zhu, L, and Ju, W. Si-Ni-san prevents reserpine-induced depression by inhibiting inflammation and regulating CYP450 enzymatic activity. Front Pharmacol. (2020) 10:1518. doi: 10.3389/fphar.2019.01518
42. Piao, J, Meng, F, Fang, H, Piao, F, Jin, B, Li, M, et al. Effect of taurine on thymus differentiation of dex-induced immunosuppressive mice. Taurine. (2019) 11:381–90. doi: 10.1007/978-981-13-8023-5_36
43. Zhou, M, Wu, Z, Deng, D, Wang, B, Zhou, X, Zhou, B, et al. Effects of taurine on the growth performance, diarrhea, oxidative stress and intestinal barrier function of weanling piglets. Front Vet Sci. (2024) 11:1436282. doi: 10.3389/fvets.2024.1436282
44. Chase, CC Jr, Randel, RD, Riley, DG, Coleman, SW, and Phillips, WA. Evaluation of tropically adapted straight bred and crossbred beef cattle: cortisol concentration and measures of temperament at weaning and transport. J Anim Sci. (2017) 95:5253–62. doi: 10.2527/jas2017.1924
45. Lee, J, Beak, S, Hong, SJ, Jeong, IH, Yoo, SP, Lee, JO, et al. Effects of stress after road transportation and oral administration of chromium and meloxicam on plasma cortisol concentrations and behavior in dairy calves. Anim Biosci. (2022) 35:503–10. doi: 10.5713/ab.21.0321
Keywords: rumen-protected glucose, taurine, yak, transport, stress response
Citation: Wang X, Zhao K, Zhao S, Zhou J, Cao M, Lu L, Chen Y, Yang H, Zhang B, Shao C, Zhao Y, Tang R and Xue B (2024) Effects of dietary rumen-protected glucose level and taurine supplementation on weight change and oxidative stress state of yaks after transport. Front. Vet. Sci. 11:1492747. doi: 10.3389/fvets.2024.1492747
Edited by:
Sadarman Sadarman, State Islamic University of Sultan Syarif Kasim Riau, IndonesiaReviewed by:
Yukun Sun, Northeast Agricultural University, ChinaLiyang Zhang, Henan Agricultural University, China
Xu Hongjian, Hebei Agricultural University, China
Copyright © 2024 Wang, Zhao, Zhao, Zhou, Cao, Lu, Chen, Yang, Zhang, Shao, Zhao, Tang and Xue. This is an open-access article distributed under the terms of the Creative Commons Attribution License (CC BY). The use, distribution or reproduction in other forums is permitted, provided the original author(s) and the copyright owner(s) are credited and that the original publication in this journal is cited, in accordance with accepted academic practice. No use, distribution or reproduction is permitted which does not comply with these terms.
*Correspondence: Bai Xue, MTM1OTJAc2ljYXUuZWR1LmNu
†These authors have contributed equally to this work